- 1State Key Laboratory of Supramolecular Structure and Materials, Jilin University, Changchun, China
- 2State Key Laboratory on Integrated Optoelectronics, Key Laboratory of Advanced Gas Sensors, College of Electronic Science and Engineering, Jilin University, Changchun, China
To suppress efficiency roll-off induced by triplet–triplet annihilation (TTA) and singlet–triplet annihilation (STA) in thermally activated delayed fluorescence (TADF) based organic light emitting diodes (OLEDs) is still a challenge. This issue was efficiently addressed by generating dual delayed fluorescence in the emitting layer of OLEDs. A novel TADF compound, PXZ-CMO, featuring a D-A structure was designed and synthesized. By dispersing the emitter into different hosts, devices G1 (MCP host) and G2 (DPEPO host) with identical configurations were carefully fabricated, which showed similar maximum EQE/CE of 12.1%/38.2 cd A−1 and 11.8%/33.1 cd A−1, respectively. Despite severe efficiency roll-off in device G2 with only 6.4% EQE remaining at a luminance of 1,000 cd m−2, a remarkably reduced efficiency roll-off was attained in device G1, retaining EQE as high as 10.4% at the same luminance of 1,000 cd m−2. The excellent device performance with reduced roll-off in device G1 should result from the dual delayed fluorescence in the emitting layer, which possesses great advantages in achieving dynamic and adaptive exciton distribution for radiation acceleration and quench suppression.
Introduction
Recently, thermally activated delayed fluorescence (TADF) materials based on pure organic aromatic molecules have drawn great attention for their nature to achieve 100% exciton utilization in organic light emitting diodes (OLEDs) (Uoyama et al., 2012; Tao et al., 2014; Zhang et al., 2014a; Kaji et al., 2015; Liu et al., 2015, 2017; Cho et al., 2016; Data et al., 2016; Li et al., 2016; Chen et al., 2017). TADF-OLEDs with high external quantum efficiencies (EQEs) of over 20% have been reported within the visible light spectrum region. However, they still suffer from severe efficiency roll-offs and often exhibited quite low EQEs at a brightness of over 1,000 cd m−2, which is the required value for the practical application (Wang et al., 2014; Lin et al., 2016; Rajamalli et al., 2016; Huang et al., 2017; Wu et al., 2018; Zeng et al., 2018). For most of TADF emitters this is a great issue, which can induce the increase of power consumption, reduction of device lifetime, and limitation of their extensive applications. For TADF-OLEDs, the electrically generated triplet excitons contribute to emission through reverse intersystem crossing (RISC) process (Figure 1A), which can result in the transformation from triplet (T1) to singlet (S1) and sequential single exited state radiation transition.
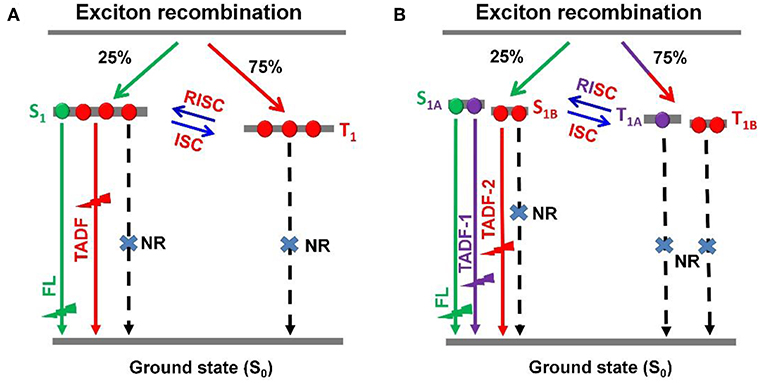
Figure 1. Schematic representations of RISC processes of emitters with (A) single delayed excited state and (B) dual delayed excited states.
To accomplish the RISC process, a relatively long time is essential. Therefore, in emitting layer (EML), the accumulation of T1 excitons is unavoidable, leading to intense T1-T1 annihilation (TTA), and S1-T1 annihilation (STA). The TTA and STA can result in remarkable efficiency roll-off. In principle, the efficiency roll-off can be suppressed by reducing delayed lifetime and concentration of T1 excitons. It was demonstrated that the TADF emitters with shorter delayed lifetimes displayed relatively smaller efficiency roll-offs (Numata et al., 2015; Lee et al., 2016, 2017). On the other hand, to promote both of phosphorescence and delayed fluorescence in OLEDs could also suppress the efficiency roll-off (Zhang et al., 2016a; Wei et al., 2017; Yu et al., 2017). To dilute the T1 excitons within an EML, a scheme for establishing the single molecule based dual- or multi-T1 excited states with different lifetimes and similar emission maxima was proposed (Figure 1B). It is rational to expect a breakthrough in EL performance based on this dual delayed fluorescence mechanism from both S1 and T1 states, which theoretically possesses great advantages in achieving dynamic and adaptive exciton distribution for radiation acceleration and quench suppression. However, so far, the construction of TADF systems with dual- or multi-T1 excited states still remains a great challenge.
To achieve TADF, the exited states generally have intramolecular-charge-transfer (ICT) characteristic and the molecules are composed of spatially separated donor (D) and acceptor (A) moieties. Upon transformation from ground state to excited state, the molecules undergo internal electron transfer from D to A, which is usually accompanied by molecular conformation change resulting a new dipolar state to stabilize the exited state. It was demonstrated that the above process could be dominated by the environment polarity when the TADF molecules were doped in some matrixes (Grabowski et al., 2003; Aydemir et al., 2015, 2017; Data et al., 2016). Upon a kind of TADF molecules are doped into a host, the molecules may exist under different polarity and rigidity circumstances, which can induce different excited states. Additionally, the D-A type molecules containing pseudo-planar fragments, such as xanthone (XO), 9,9-dimethyl-9,10-dihydroacridine (DMAC), and phenoxazine (PXZ), have the potential to adopt different molecular configurations (Zhang et al., 2016b, 2017; Wang et al., 2017). Therefore, by dispersing the emitters in suitable hosts with distinct polarity and steric hindrance, dual- or multi-T1 excited states may be generated from one kind of TADF molecules (Méhes et al., 2014; Zhang et al., 2014b). To evaluate our hypothesis, 4H-chromen-4-one (CMO) and phenoxazine (PXZ) were selected as the electron A and D moieties, respectively, to construct a target TADF molecule PXZ-CMO (Figure 2A). 1,3-bis(carbazol-9-yl)benzene (MCP) with weak polarity and bis(2-(diphenylphosphino)phenyl)ether oxide (DPEPO) with strong polarity were employed as the hosts to prepare the emitting layer of OLEDs, respectively (Lee et al., 2014). In this contribution, we successfully develop an effective strategy to reduce the efficiency roll-off of TADF-OLEDs based on the dual delayed fluorescence in the emitting layer.
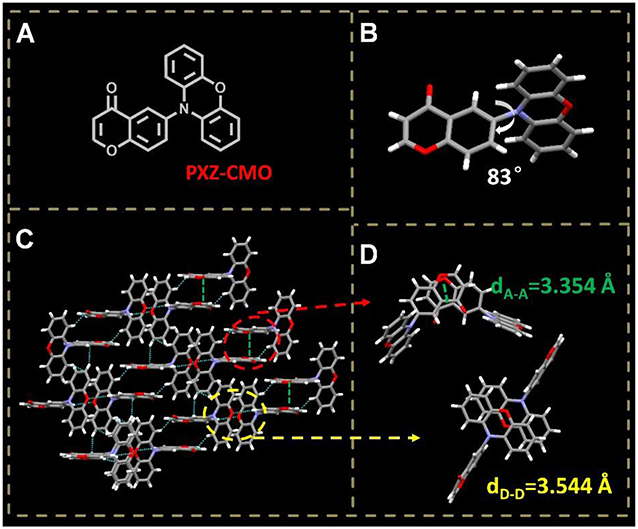
Figure 2. (A) Molecular structure of PXZ-CMO. (B) Single crystal structure of PXZ-CMO: twisted molecular structure. (C,D) Intermolecular interactions and molecular packing mode.
Results and Discussion
Synthesis, Crystal and Photophysical Properties
A TADF emitter 6-(10H-phenoxazin-10-yl)-4H-chromen-4-one, namely PXZ-CMO, was synthesized via Buchwald-Hartwig cross coupling between 6-bromo-4H-chromen-4-one and PXZ with high yields (Scheme S1) (Wolfe et al., 1998; Littke et al., 2002; Hooper et al., 2003; Fu, 2008). The single-crystal X-ray diffraction analyses demonstrated that both the PXZ and CMO moieties adopt perfect planar π-conjugated structure feature. The D and A planes are linked together by a single bond, which is beneficial to the regulation of molecular conformation. The crystal is generated based on moderate intermolecular π···π stacking interactions (acceptor···acceptor contacts) accompanied by noncovalent bonds such as C-H···O, O = C···H-C, and C-H···π interactions (Figures 2C,D), which can enhance the charge transfer ability (Wang et al., 2013). Since the highly twisted structure with large dihedral angle of 83° between D and A planes, the highest occupied molecular orbital (HOMO) and the lowest unoccupied molecular orbital (LUMO) of PXZ-CMO are mainly localized on the D and A moieties (Figure 2B and Figure S1), respectively. Therefore, the energy gap (ΔEST) between the lowest singlet excited state (S1) and triplet excited state (T1) is as small as 0.02 eV, which was calculated based on spectroscopic data in Figure S2. Obviously, the ΔEST value is small enough to promote the reverse intersystem crossing (RISC) from T1 to S1 (Yang et al., 2017).
The UV–vis absorption and emission spectra of PXZ-CMO in various solvents with different polarity were shown in Figure S3 and the photophysical data were summarized in Table S1. The strong absorption band at around 310 nm can be attributed to the π-π* transition of the PXZ, and the other weak and broad absorption band at longer wavelengths from 345 to 420 nm can be assigned to the ICT absorption from D (PXZ) to A (CMO). PXZ-CMO emission spectra displayed a significant solvatochromic phenomenon and the emission maxima displayed red-shift from 398 nm in toluene to 524 nm in dichloromethane. The largely solvatochromic red-shift indicated a typical ICT feature of PXZ-CMO, suggesting a large change of dipole moment in the electronically excited state (Guo et al., 2014; Wang et al., 2015; Li et al., 2017). Moreover, emissions that originated from local excited (LE) states could also be observed in toluene and THF solutions, which further demonstrated a weak electronic coupling between PXZ and CMO units. The transient PL decays for PXZ-CMO were measured in dilute solutions under nitrogen atmosphere (Figure S4). Unlike the behavior of conventional TADF molecules, its long-lived TADF emission was completely quenched by non-radiative decay in solution and only the prompt components with nanosecond-scale lifetimes were observed (Zhang et al., 2012, 2014c). In solid state, PXZ-CMO displayed a prompt lifetime of 93 ns and a delayed lifetime of 1.6 μs (Figure S5). The PXZ-CMO solid exhibited photoluminescence quantum yield (PLQY) of 27.6% and emission maximum of 518 nm (Table S1 and Figure S6). Thermogravimetric analysis (TGA) and differential scanning calorimetry (DSC) measurements revealed that PXZ-CMO possessed a good thermal stability with a thermal decomposition temperature (Td5, corresponding to 5% weight loss) of 261°C and a melting point (Tm) of 187°C (Figure S7). The HOMO (−5.95 eV) and LUMO (−3.35 eV) energy levels of PXZ-CMO were obtained from the onsets of the oxidation and reduction curves (Figure S8).
Electroluminescence Performance
To evaluate the electroluminescent (EL) performance of PXZ-CMO, we fabricated multi-layer OLEDs with a structure of [ITO/HATCN (5 nm)/NPB (60 nm)/MCP (5 nm)/EML (30 nm)/TSPO1 (5 nm)/TPBi (30 nm)/LiF (0.5 nm)/Al (150 nm)] (Figure 3). ITO (indium-tin oxide) and LiF/Al (lithium fluoride/aluminum) were used as anode and cathode, respectively. HATCN (1,4,5,8,9,11-hexaazatriphenylene hexacarbonitrile), NPB (1,4-bis[(1-naphthylphenyl)amino]-biphenyl), and TPBi (1,3,5-tris(Nphenylbenzimidazol-2-yl)benzene) were selected as hole-injection (HIL), hole-transporting (HTL) and electron-transporting layers (ETL), respectively. MCP (T1 = 2.90 eV) and TSPO1 (diphenyl-4-triphenylsilylphenyl-phosphine oxide with high T1 (3.36 eV) were acted as the exciton-blocking layers (Kim and Lee, 2014). The doped thin films of PXZ-MCO (dopant) in solid hosts of MCP or DPEPO (T1 = 3.10 eV) with different dopant concentrations from 5 to 30 wt% were employed as the emitting layers (EMLs). Table 1 presents the key EL parameters of PXZ-CMO based OLEDs with various dopant concentrations (5, 15, 25 wt% PXZ-CMO doped in MCP and 10, 15, 20, 30 wt% PXZ-CMO doped in DPEPO). Obviously, the DPEPO-based devices all showed more severe efficiency roll-offs than that of the MCP-based ones. When 15 and 20 wt% dopant concentrations were employed, the MCP- and DPEPO-based devices displayed the best performances with the highest external quantum efficiencies (EQEs) of 12.1 and 11.8%, respectively. The photoluminescence quantum yields (PLQYs) of film A (15 wt% PXZ-CMO:MCP) and film B (20 wt% PXZ-CMO:DPEPO) were 48.7% and 35.0% (Table S1), respectively. Thus, almost all T1 excitons were up-converted into S1 excitons and utilized for electroluminescence, resulting in high EQEs. The finally optimized EMLs are 15 wt% PXZ-CMO:MCP (Device G1) and 20 wt% PXZ-CMO:DPEPO (Device G2). The current density–voltage–luminance (J–V–L), EQE–luminance (EQE–L), current efficiency–luminance (CE–L) characteristics, and EL spectra of devices G1 and G2 were present in Figure 4. Devices G1 and G2 exhibited emission maxima at 524 and 517 nm with corresponding Commission Internationale de l'E'clairage (CIE) color coordinates of (0.28, 0.55) and (0.30, 0.48), respectively (Figure 3D). Additionally, the EL spectra demonstrated negligible change with increasing driving voltages (Figure S9). Devices G1 and G2 showed comparable highest EQE values, however, the efficiency roll-off of device G1 was remarkably small compared with that of device G2. Under a high luminance of 1,000 cd m−2, G1 maintained its EQE at 10.4%, while the EQE of G2 reduced to 6.4%.
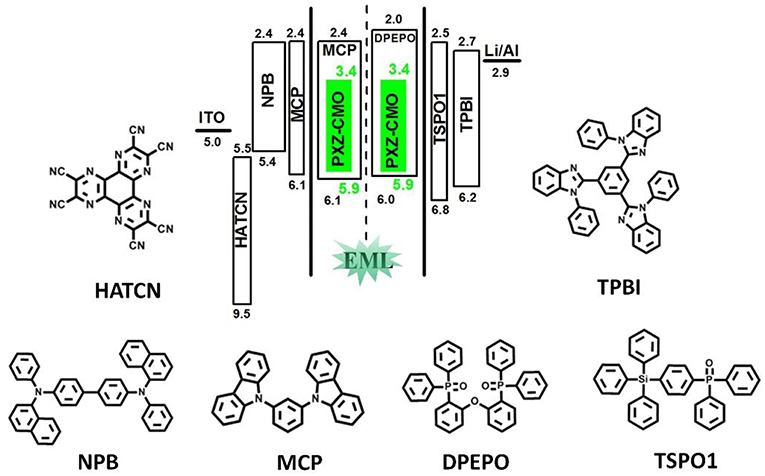
Figure 3. The energy-level diagram of the four devices and chemical structures of the used materials.
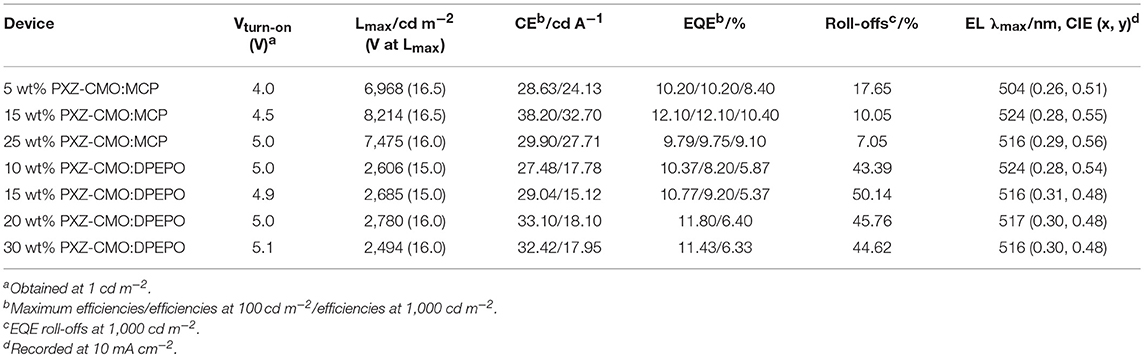
Table 1. Summary of the EL data of OLEDs based on PXZ-CMO doped in MCP/DPEPO with different doping concentrations.
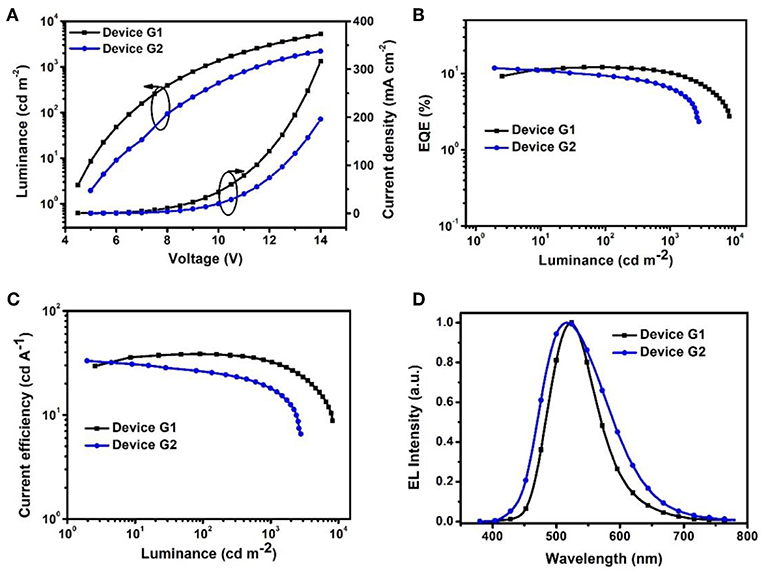
Figure 4. (A) Current density–voltage–luminance (J–V–L). (B) EQE vs. luminance curves. (C) Current efficiency vs. luminance curves. (D) EL spectra measured at 10 mA cm−2.
Carrier Transport and Transient PL Spectra Properties
Firstly, we evaluate the carrier transport properties of PXZ-CMO doped in MCP and DPEPO. The single-carrier devices with the structures of [ITO/NPB (10 nm)/15% PXZ-CMO:MCP or 20% PXZ-CMO:DPEPO (80 nm)/NPB (10 nm)/Al (100 nm)] for the hole-only device and [ITO/TPBI (10 nm)/15% PXZ-CMO:MCP or 20% PXZ-CMO:DPEPO (80 nm)/TPBI (10 nm)/LiF (1 nm)/Al (100 nm)] for the electron-only device were fabricated. The NPB and TPBI layers are used to prevent the electron and hole injection from the cathode and anode, respectively. As depicted in Figure S10, both hole and electron current density of 15% PXZ-CMO:MCP are higher than that of 20% PXZ-CMO:DPEPO at the same driving voltage, suggesting better ability for conducting both electrons and holes. However, both two doped films exhibit balanced carrier transport property, indicating that the different efficiency roll-off behaviors of devices G1 and G2 should not be attributed to be the difference of the carrier transport. To further understand the reasons of the different efficiency roll-off behaviors of devices G1 and G2, detail photophysical investigations of PXZ-CMO:MCP and PXZ-CMO:DPEPO doped films were performed and detailed data were summarized in Table S2. As shown in Figures 5, 6, the delayed PL emissions all presented similar spectral distributions as that of the prompt PL emissions, implying that the delayed and prompt fluorescence of these films originated from the same emissive singlet states (Kim et al., 2018). Upon fitting the transient PL decays of PXZ-CMO:MCP doped films, a clear third-order exponential decay could be found, revealing the presence of two delayed fluorescence processes with characteristic time constants of around 1.1 and 9.1 μs, respectively. Moreover, for PXZ-CMO:MCP films, the integral ration of the relatively shorter delayed lifetime remarkably decreased and the longer delayed lifetime showed a reverse tendency with increasing the doped concentration. While the PXZ-CMO:DPEPO doped films showed only one delayed lifetime (around 6.5 μs), testifying the typical TADF feature with a single delayed fluorescence process. In films with the doped concentration of over 5 wt%, the PXZ-CMO molecules should not adopt mono-dispersed state and assemble into small aggregates, which could be proved by the obvious spectral redshift of the green emission in both MCP- and DPEPO-based films as the doping concentration is increased (Figure 5). Since MCP is a weakly polar host, DPEPO is a strongly polar host, and the polarity of PXZ-CMO molecule with D-A structural feature is also very strong. Therefore, from the perspective of polarity, PXZ-CMO and DPEPO are similar with each other, while PXZ-CMO and MCP are different. For the PXZ-CMO aggregates within MCP matrix, the PXZ-CMO molecules should be in strong polarity environment (the inside of aggregates) or weak polarity environment (the surface of aggregates). The different polarity environment can not only induce different excited sate characteristics (two kinds of delayed lifetimes in MCP-based films), but also increase the distance between these two T1 states to prevent the TTA process, which occurs by a diffusion-limited, short-range electron exchange process (Dexter mechanism) (O'Brien et al., 2009). Differently, in PXZ-CMO:DPEPO doped films, both the dopant and host molecules possess strong polarity, therefore only one delayed lifetime was observed, similar to the PXZ-CMO solids. In addition, the singlet CT state in a polar matrix of DPEPO will be more stabilized than that of MCP, resulting in a theoretically smaller ΔEST values as well as faster RISC rates (shorter TADF lifetimes) in DPEPO-based films. However, according to the formula (Zhang et al., 2014c), the average lifetime of film A (6.5 μs) is actually similar to that of PXZ-CMO:DPEPO doped films (5.2–7.9 μs) at 300 K. This phenomenon indicates that not only the polarity of the host but also some potential interactions (such as π-π interactions) between the emitter and the host affect the RISC rate (Cai et al., 2017), because MCP has the potential π-π interaction groups of carbazole, while DPEPO doesn't. Thus, the dual delayed fluorescence in the MCP-based films played an important role in realizing the slow efficiency roll-offs.
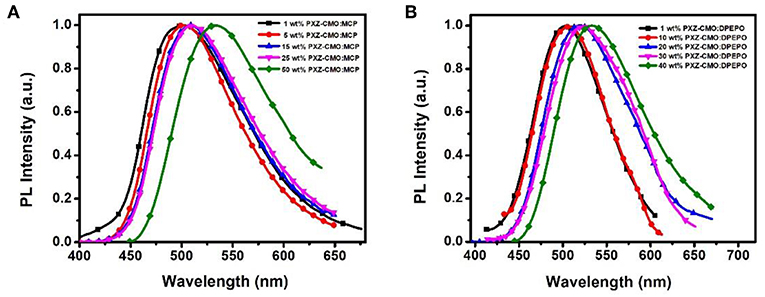
Figure 5. The normalized PL spectra of films based on PXZ-CMO doped in (A) MCP and (B) DPEPO with different doping concentrations.
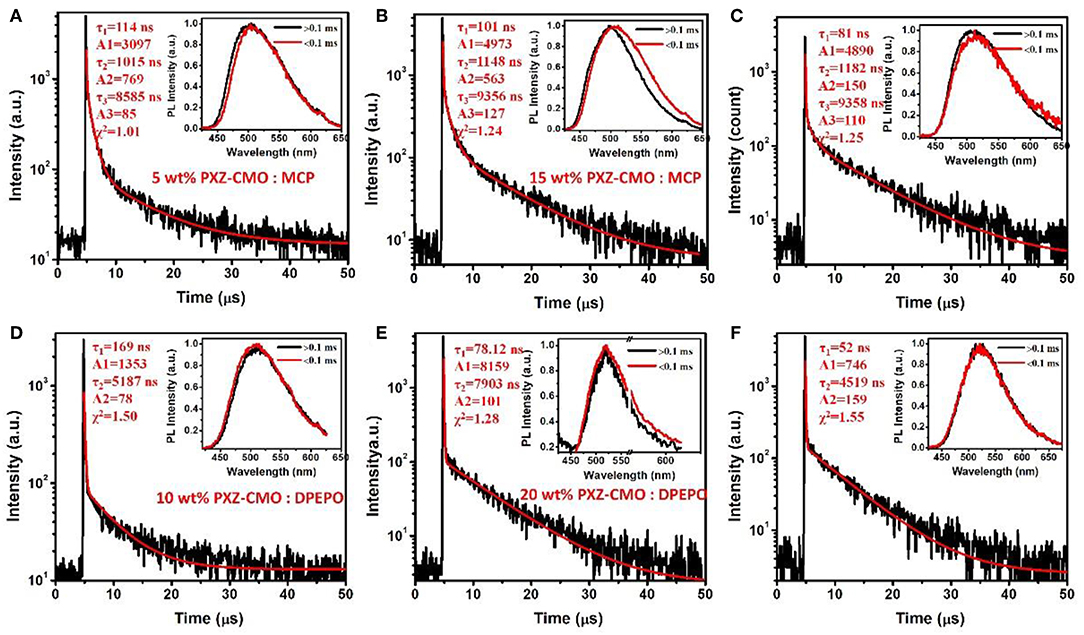
Figure 6. Transient decay spectra of films: (A) 5 wt% PXZ-CMO:MCP, (B) 5 wt% PXZ-CMO:MCP, (C) 25 wt% PXZ-CMO:MCP, (D) 10 wt% PXZ-CMO:DPEPO, (E) 20 wt% PXZ-CMO:DPEPO, and (F) 30 wt% PXZ-CMO:DPEPO at 300 K. Red curves are three or double exponential fitting data. Inset: Emission spectra of TADF components measured by HORIBA Scientific FluoroMax-4 spectrofluorometer.
The temperature dependent photopgysical properties of doped films A (15 wt% PXZ-CMO:MCP) and B (20 wt% PXZ-CMO:DPEPO) were presented in Figure 7 and Tables S3, S4. Within the temperature region from 78 to 325 K, film A displayed two delayed fluorescence lifetimes at around 1.0 (τd1) μs and 5.0–12.0 (τd2) μs, respectively. The integral ratio of the longer τd2 gradually improved upon temperature increasing, suggesting a typical TADF feature (Uoyama et al., 2012; Kaji et al., 2015). For the shorter τd1, the integral ratio displayed slight improvement upon temperature increasing from 78 to 175 K and then obviously decreased upon temperature increasing from 175 to 325 K. Therefore, it is possible that certain conformation transformation mechanism may be included in the emission spectra of film A at high temperature region (above 200 K). In particular, it still exhibited two delayed lifetimes at 77 K, which further suggested the presence of two T1 excited states of film A (Table S3). For film B, the delayed fluorescence was observed when the temperature was improved to 200 K and the delayed lifetime exhibited successive decrease tendency. Therefore, in DPEPO matrix, the triple exited state of PXZ-CMO may be easily quenched and the TADF behavior was only observed at relatively high temperature, which can provide enough energy to promote the RISC process. Thus, for device G1, the existence of dual delayed fluorescence makes it possible to decrease the concentrations of every kind of T1 excited state and suppress the TTA processes to realize an extremely low efficiency roll-off in TADF-OLED. In addition, the delayed lifetimes (τd) began to decrease when the dopant concentration ≥30 wt% in both MCP- and DPEPO-based films (Figure S11), which could be attributed to that the TTA induced excited state quenching is stronger in the high dopant concentration films. To further verify the molecular structure of PXZ-CMO, 1H and 13C NMR spectroscopies were measured (Figures S12, S13).
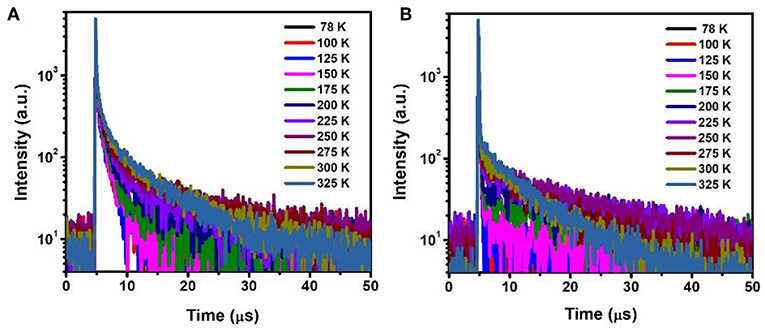
Figure 7. Temperature dependence of time decay characteristics for (A) film A and (B) film B in the range of 78–325 K.
Conclusion
In summary, a TADF compound PXZ-CMO featuring a D-A structure was designed and synthesized. The PXZ-CMO:MCP doped films displayed two delayed fluorescence lifetimes at around 1.1 and 9.1 μs, respectively. The PXZ-CMO:MCP films were employed as emitting layer to fabricate high performance TADF-OLEDs that exhibited low efficiency roll-offs. The MCP matrix with weak polarity can promote the generation of dual delayed fluorescence for PXZ-CMO molecules. The achievement of dual delayed fluorescence can dilute the concentration of T1 excited states and suppress the TTA processes in the emitting layer, which is beneficial to the reduction of efficiency roll-off. Therefore, this study provided an efficient approach to achieve the high-efficiency TADF-OLEDs with low efficiency roll-offs.
Author Contributions
YZ, CL, and YW proposed the idea of this manuscript and analyzed the experiment results. YZ and ZL contributed to the synthesis of the materials and the fabrication of the OLEDs. YW wrote the paper.
Funding
This work was supported by the National Basic Research Program of China (2015CB655003), the National Natural Science Foundation of China (91333201 and 51803069), China Postdoctoral Science Foundation (2018M630319 and 2018T110244), Program for JLU Science and Technology Innovative Research Team and Jilin Province-University Program (SXGJSF2017-3).
Conflict of Interest Statement
The authors declare that the research was conducted in the absence of any commercial or financial relationships that could be construed as a potential conflict of interest.
Supplementary Material
The Supplementary Material for this article can be found online at: https://www.frontiersin.org/articles/10.3389/fchem.2019.00302/full#supplementary-material
References
Aydemir, M., Haykir, G., Türksoy, F., Gümüş, S., Dias, F. B., and Monkman, A. P. (2015). Synthesis and investigation of intra-molecular charge transfer state properties of novel donor–acceptor–donor pyridine derivatives: the effects of temperature and environment on molecular configurations and the origin of delayed fluorescence. Phys. Chem. Chem. Phys. 17, 25572–25582. doi: 10.1039/C5CP03937A
Aydemir, M., Xu, S., Chen, C., Bryce, M. R., Chi, Z., and Monkman, A. P. (2017). Photophysics of an asymmetric donor–acceptor–donor′ TADF molecule and reinterpretation of aggregation-induced TADF emission in these materials. J. Phys. Chem. C 121, 17764–17772. doi: 10.1021/acs.jpcc.7b06299
Cai, M., Song, X., Zhang, D., Qiao, J., and Duan, L. (2017). π-π stacking: a strategy to improve the electron mobilities of bipolar hosts for TADF and phosphorescent devices with low efficiency roll-off. J. Mater. Chem. C 5, 3372–3381. doi: 10.1039/C7TC00733G
Chen, J.-X., Liu, W., Zheng, C.-J., Wang, K., Liang, K., Shi, Y.-Z., et al. (2017). Coumarin-Based thermally activated delayed fluorescence emitters with high external quantum efficiency and low efficiency roll-off in the devices. ACS Appl. Mater. Interfaces 9, 8848–8854. doi: 10.1021/acsami.6b15816
Cho, Y. J., Jeon, S. K., and Lee, J. Y. (2016). Molecular engineering of high efficiency and long lifetime blue thermally activated delayed fluorescent emitters for vacuum and solution processed organic light emitting diodes. Adv. Optical Mater. 4, 688–693. doi: 10.1002/adom.201500634
Data, P., Pander, P., Okazaki, M., Takeda, Y., Minakata, S., and Monkman, A. P. (2016). Dibenzo[a,j]phenazine-cored donor–acceptor–donor compounds as green-to-red/NIR thermally activated delayed fluorescence organic light emitters. Angew. Chem. Int. Ed. 55, 5739–5744. doi: 10.1002/anie.201600113
Fu, G. C. (2008). The development of versatile methods for palladium-catalyzed coupling reactions of aryl electrophiles through the use of P(t-Bu)3 and PCy3 as ligands. Acc. Chem. Res. 41, 1555–1564. doi: 10.1021/ar800148f
Grabowski, Z. R., Rotkiewicz, K., and Rettig, W. (2003). Structural changes accompanying intramolecular electron transfer: focus on twisted intramolecular charge-transfer states and structures. Chem. Rev. 103, 3899–4032. doi: 10.1021/cr940745l
Guo, Z.-H., Jin, Z.-X., Wang, J.-Y., and Pei, J. (2014). A donor–acceptor–donor conjugated molecule: twist intramolecular charge transfer and piezochromic luminescent properties. Chem. Commun. 50, 6088–6090. doi: 10.1039/c3cc48980a
Hooper, M. W., Utsunomiya, M., and Hartwig, J. F. (2003). Scope and mechanism of palladium-catalyzed amination of five-membered heterocyclic halides. J. Org. Chem. 68, 2861–2873. doi: 10.1021/jo0266339
Huang, J., Nie, H., Zeng, J., Zhuang, Z., Gan, S., Cai, Y., et al. (2017). Highly efficient nondoped OLEDs with negligible efficiency roll-off fabricated from aggregation-induced delayed fluorescence luminogens. Angew. Chem. Int. Ed. 56, 12971–12976. doi: 10.1002/anie.201706752
Kaji, H., Suzuki, H., Fukushima, T., Shizu, K., Suzuki, K., Kubo, S., et al. (2015). Purely organic electroluminescent material realizing 100% conversion from electricity to light. Nat. Commun. 6:8476. doi: 10.1038/ncomms9476
Kim, B. S., and Lee, J. Y. (2014). Phosphine oxide type bipolar host material for high quantum efficiency in thermally activated delayed fluorescent device. ACS Appl. Mater. Interfaces 6, 8396–8400. doi: 10.1021/am501301g
Kim, D.-H., D'Aléo, A., Chen, X.-K., Sandanayaka, A. D. S., Yao, D., Zhao, L., et al. (2018). High-efficiency electroluminescence and amplified spontaneous emission from a thermally activated delayed fluorescent near-infrared emitter. Nat. Photonics 12, 98–104. doi: 10.1038/s41566-017-0087-y
Lee, D. R., Choi, J. M., Lee, C. W., and Lee, J. Y. (2016). Ideal molecular design of blue thermally activated delayed fluorescent emitter for high efficiency, small singlet–triplet energy splitting, low efficiency roll-off, and long lifetime. ACS Appl. Mater. Interfaces 8, 23190–23196. doi: 10.1021/acsami.6b05877
Lee, J., Aizawa, N., and Yasuda, T. (2017). Isobenzofuranone- and chromone-based blue delayed fluorescence emitters with low efficiency roll-off in organic light-emitting diodes. Chem. Mater. 29, 8012–8020. doi: 10.1021/acs.chemmater.7b03371
Lee, S. Y., Yasuda, T., Yang, Y. S., Zhang, Q., and Adachi, C. (2014). Luminous butterflies: efficient exciton harvesting by benzophenone derivatives for full-color delayed fluorescence OLEDs. Angew. Chem. Int. Ed. 53, 6402–6406. doi: 10.1002/anie.201402992
Li, C., Duan, R., Liang, B., Han, G., Wang, S., Ye, K., et al. (2017). Deep-red to near-infrared thermally activated delayed fluorescence in organic solid films and electroluminescent devices. Angew. Chem. Int. Ed. 56, 11525–11529. doi: 10.1002/anie.201706464
Li, Y., Li, X.-L., Chen, D., Cai, X., Xie, G., He, Z., et al. (2016). Design strategy of blue and yellow thermally activated delayed fluorescence emitters and their all-fluorescence white OLEDs with external quantum efficiency beyond 20%. Adv. Funct. Mater. 26, 6904–6912. doi: 10.1002/adfm.201602507
Lin, T.-A., Chatterjee, T., Tsai, W.-L., Lee, W.-K., Wu, M.-J., Jiao, M., et al. (2016). Sky-blue organic light emitting diode with 37% external quantum efficiency using thermally activated delayed fluorescence from spiroacridine-triazine hybrid. Adv. Mater. 28, 6976–6983. doi: 10.1002/adma.201601675
Littke, A. F., Schwarz, L., and Fu, G. C. (2002). Pd/P(t-Bu)3: a mild and general catalyst for stille reactions of aryl chlorides and aryl bromides. J. Am. Chem. Soc. 124, 6343–6348. doi: 10.1021/ja020012f
Liu, M., Komatsu, R., Cai, X., Sasabe, H., Kamata, T., Nakao, K., et al. (2017). Introduction of twisted backbone: a new strategy to achieve efficient blue fluorescence emitter with delayed emission. Adv. Optical Mater. 5:1700334. doi: 10.1002/adom.201700334
Liu, X.-K., Chen, Z., Zheng, C.-J., Liu, C.-L., Lee, C.-S., Li, F., et al. (2015). Prediction and design of efficient exciplex emitters for high-efficiency, thermally activated delayed-fluorescence organic light-emitting diodes. Adv. Mater. 27, 2378–2383. doi: 10.1002/adma.201405062
Méhes, G., Goushi, K., Potscavage, W. J., and Adachi, C. (2014). Influence of host matrix on thermally-activated delayed fluorescence: effects on emission lifetime, photoluminescence quantum yield, and device performance. Org. Electron. 15, 2027–2037. doi: 10.1016/j.orgel.2014.05.027
Numata, M., Yasuda, T., and Adachi, C. (2015). High efficiency pure blue thermally activated delayed fluorescence molecules having 10H-phenoxaborin and acridan units. Chem. Commun. 51, 9443–9446. doi: 10.1039/C5CC00307E
O'Brien, J. A., Rallabandi, S., Tripathy, U., Paige, M. F., and Steer, R. P. (2009). Efficient S2 state production in ZnTPP–PMMA thin films by triplet–triplet annihilation: Evidence of solute aggregation in photon upconversion systems. Chem. Phys. Lett. 475, 220–222. doi: 10.1016/j.cplett.2009.05.039
Rajamalli, P., Senthilkumar, N., Gandeepan, P., Huang, P.-Y., Huang, M.-J., Ren-Wu, C.-Z., et al. (2016). A new molecular design based on thermally activated delayed fluorescence for highly efficient organic light emitting diodes. J. Am. Chem. Soc. 138, 628–634. doi: 10.1021/jacs.5b10950
Tao, Y., Yuan, K., Chen, T., Xu, P., Li, H., Chen, R., et al. (2014). Thermally activated delayed fluorescence materials towards the breakthrough of organoelectronics. Adv. Mater. 26, 7931–7958. doi: 10.1002/adma.201402532
Uoyama, H., Goushi, K., Shizu, K., Nomura, H., and Adachi, C. (2012). Highly efficient organic light-emitting diodes from delayed fluorescence. Nature 492, 234–238. doi: 10.1038/nature11687
Wang, H., Xie, L., Peng, Q., Meng, L., Wang, Y., Yi, Y., et al. (2014). Novel thermally activated delayed fluorescence materials–thioxanthone derivatives and their applications for highly efficient OLEDs. Adv. Mater. 26, 5198–5204. doi: 10.1002/adma.201401393
Wang, K., Zhao, F., Wang, C., Chen, S., Chen, D., Zhang, H., et al. (2013). High-performance red, green, and blue electroluminescent devices based on blue emitters with small singlet–triplet splitting and ambipolar transport property. Adv. Funct. Mater. 23, 2672–2680. doi: 10.1002/adfm.201202981
Wang, K., Zheng, C.-J., Liu, W., Liang, K., Shi, Y.-Z., Tao, S.-L., et al. (2017). Avoiding energy loss on TADF emitters: controlling the dual conformations of D–A structure molecules based on the pseudoplanar segments. Adv. Mater. 29:1701476. doi: 10.1002/adma.201701476
Wang, S., Yan, X., Cheng, Z., Zhang, H., Liu, Y., and Wang, Y. (2015). Highly efficient near-infrared delayed fluorescence organic light emitting diodes using a phenanthrene-based charge-transfer compound. Angew. Chem. Int. Ed. 54, 13068–13072. doi: 10.1002/anie.201506687
Wei, X., Chen, Y., Duan, R., Liu, J., Wang, R., Liu, Y., et al. (2017). Triplet decay-induced negative temperature dependence of the transient photoluminescence decay of thermally activated delayed fluorescence emitter. J. Mater. Chem. C 5, 12077–12084. doi: 10.1039/C7TC04025C
Wolfe, J. P., Wagaw, S., Marcoux, J.-F., and Buchwald, S. L. (1998). Rational development of practical catalysts for aromatic carbon–nitrogen bond formation. Acc. Chem. Res. 31, 805–818. doi: 10.1021/ar9600650
Wu, T.-L., Huang, M.-J., Lin, C.-C., Huang, P.-Y., Chou, T.-Y., Chen-Cheng, R.-W., et al. (2018). Diboron compound-based organic light-emitting diodes with high efficiency and reduced efficiency roll-off. Nat. Photonics 12, 235–240. doi: 10.1038/s41566-018-0112-9
Yang, Z., Mao, Z., Xie, Z., Zhang, Y., Liu, S., Zhao, J., et al. (2017). Recent advances in organic thermally activated delayed fluorescence materials. Chem. Soc. Rev. 46, 915–1016. doi: 10.1039/C6CS00368K
Yu, L., Wu, Z., Zhong, C., Xie, G., Zhu, Z., Ma, D., et al. (2017). Pure organic emitter with simultaneous thermally activated delayed fluorescence and room-temperature phosphorescence: thermal-controlled triplet recycling channels. Adv. Optical Mater. 5:1700588. doi: 10.1002/adom.201700588
Zeng, W., Lai, H.-Y., Lee, W.-K., Jiao, M., Shiu, Y.-J., Zhong, C., et al. (2018). Achieving nearly 30% external quantum efficiency for orange–red organic light emitting diodes by employing thermally activated delayed fluorescence emitters composed of 1,8-naphthalimide-acridine hybrids. Adv. Mater. 30:1704961. doi: 10.1002/adma.201704961
Zhang, D., Duan, L., Li, C., Li, Y., Li, H., Zhang, D., et al. (2014a). High-efficiency fluorescent organic light-emitting devices using sensitizing hosts with a small singlet–triplet exchange energy. Adv. Mater. 26, 5050–5055. doi: 10.1002/adma.201401476
Zhang, J., Duan, C., Han, C., Yang, H., Wei, Y., and Xu, H. (2016a). Balanced dual emissions from tridentate phosphine-coordinate copper(i) complexes toward highly efficient yellow OLEDs. Adv. Mater. 28, 5975–5979. doi: 10.1002/adma.201600487
Zhang, Q., Kuwabara, H., Potscavage, W. J., Huang, S., Hatae, Y., Shibata, T., et al. (2014b). Anthraquinone-based intramolecular charge-transfer compounds: computational molecular design, thermally activated delayed fluorescence, and highly efficient red electroluminescence. J. Am. Chem. Soc. 136, 18070–18081. doi: 10.1021/ja510144h
Zhang, Q., Li, B., Huang, S., Nomura, H., Tanaka, H., and Adachi, C. (2014c). Efficient blue organic light-emitting diodes employing thermally activated delayed fluorescence. Nat. Photonics 8, 326–332. doi: 10.1038/nphoton.2014.12
Zhang, Q., Li, J., Shizu, K., Huang, S., Hirata, S., Miyazaki, H., et al. (2012). Design of efficient thermally activated delayed fluorescence materials for pure blue organic light emitting diodes. J. Am. Chem. Soc. 134, 14706–14709. doi: 10.1021/ja306538w
Zhang, Y., Ma, H., Wang, S., Li, Z., Ye, K., Zhang, J., et al. (2016b). Supramolecular structure-dependent thermally-activated delayed fluorescence (TADF) properties of organic polymorphs. J. Phys. Chem. C, 120, 19759–19767. doi: 10.1021/acs.jpcc.6b05537
Keywords: thermally activated delayed fluorescence, organic light emitting diodes, dual delayed fluorescence, efficiency roll-off, donor-acceptor
Citation: Zhang Y, Li Z, Li C and Wang Y (2019) Suppressing Efficiency Roll-Off of TADF Based OLEDs by Constructing Emitting Layer With Dual Delayed Fluorescence. Front. Chem. 7:302. doi: 10.3389/fchem.2019.00302
Received: 27 January 2019; Accepted: 15 April 2019;
Published: 30 April 2019.
Edited by:
Lian Duan, Tsinghua University, ChinaReviewed by:
Zuo-Quan Jiang, Soochow University, ChinaAlbert Moyano, University of Barcelona, Spain
Copyright © 2019 Zhang, Li, Li and Wang. This is an open-access article distributed under the terms of the Creative Commons Attribution License (CC BY). The use, distribution or reproduction in other forums is permitted, provided the original author(s) and the copyright owner(s) are credited and that the original publication in this journal is cited, in accordance with accepted academic practice. No use, distribution or reproduction is permitted which does not comply with these terms.
*Correspondence: Chenglong Li, Y2hlbmdsb25nbGlAamx1LmVkdS5jbg==
Yue Wang, eXVld2FuZ0BqbHUuZWR1LmNu