- 1Key Laboratory of Experimental Marine Biology, Institute of Oceanology, Chinese Academy of Sciences, Qingdao, China
- 2Laboratory for Marine Drugs and Bioproducts of Qingdao National Laboratory for Marine Science and Technology, Qingdao, China
- 3University of Chinese Academy of Sciences, Beijing, China
- 4State Key Laboratory of Microbial Technology, Shandong University, Qingdao, China
A highly selective copper-catalyzed trifluoromethylation of indoles is reported with the assistance of a removable directing group. This protocol provides an easy and rapid method to various 2-position-selective trifluoromethylated heteroarenes including indoles, pyrroles, benzofuran, and acetanilide. What is more, the reaction takes place at ambient conditions without any external ligand or additive.
Introduction
The introduction of trifluoromethyl (CF3) groups into heteroarenes enjoys a privileged role in medicinal chemistry, since it can substantially alter their properties, such as metabolic stability, lipophilicity and ability to penetrate the blood-brain barrier (Shimizu and Hiyama, 2005; Schlosser, 2006; Hagmann, 2008; Boechat and Bastos, 2010; Nie et al., 2011; Wang et al., 2014; Gouverneur and Seppelt, 2015). It has a great potential in the development of new pharmaceutical chemicals (Scheme 1). Thus, the trifluoromethylation of heteroarenes has received tremendous attentions (Sato et al., 2010; Furuya et al., 2011; Tomashenko and Grushin, 2011; Liu et al., 2013; Le et al., 2018). On the other hand, indoles represent ubiquitous structural motifs found in biologically active natural products and pharmaceutical compounds (Lee et al., 2015; Chripkova et al., 2016; Sravanthi and Manju, 2016; Goyal et al., 2018; Kaur et al., 2019). In this regard, direct trifluoromethylation of indoles offers an attractive alternative to the workers in the field of medicinal chemistry and biochemistry.
However, direct trifluoromethylation at the C2-position of indoles under radical trifluoromethylation conditions is quite difficult because of the lack of reaction selectivity at the C2/C3-position and the high reactivity of the CF3 radical (Scheme 2A; Nagib and MacMillan, 2011). Recently, directing group (DG) has emerged as a powerful tool to achieve regioselective C2-H functionalization of indoles (Nishino et al., 2012; Zhou et al., 2013; Yu et al., 2014). For example, Shi group and Punji group, respectively, achieved trifluoroethylation and difluoroalkylation of indoles at C2 position by introducing a directing group at the N-center of indoles (Scheme 2B; Yan et al., 2017; Soni et al., 2018). Also, Sodeoka group, Cho group, and Shi group accomplished trifluoromethylation of indoles at the C2 position with Togni's reagent, CF3I and CF3SO2Na respectively. However, a substituent at C3 was identified as a crucial factor for the selective trifluoromethylation at C2 (Scheme 2C; Shimizu et al., 2010; Iqbal et al., 2012; Shi et al., 2018).
In this context, we envisioned that with the aid of a readily removable N-protecting group, trifluoromethyl group can be introduced to C2 position, which is complementary to the previous work. Herein, we report a copper-catalyzed C2-H trifluoromethylation of N-Boc (t-butyloxy) indoles with CF3SO2Na under ambient conditions in the absence of any external ligand or additive (Scheme 2D). Notably, the key to our success is the installation of a suitable Boc director on the indole nitrogen atom.
Results and Discussion
To begin, we chose N-Boc indole (1a) as model substrate. To our delight, when the reaction mixture of N-Boc indole (1a, 0.50 mmol), 2 (1.5 mmol), TBHP (t-butyl hydroperoxide, 70% solution in H2O, 2.5 mmol) and CuSO4 (10 mol%) in DMA (dimethylacetamide, 3 mL) was stirred at 85°C in air for 1 h, 22% yield of C2-trifluoromethylation product 3a was obtained (Table 1, entry 1). Trace amount of product could be detected with other solvents, such as DCM (dichloromethane), toluene and THF (tetrahydrofuran) (entries 2-4). The yield could be increased to 46% when acetone was used, and it was elevated to 54% by using MeCN (acetonitrile) (entries 5-6). Subsequently, various metal catalysts were selected. To our delighted, the yield was increased to 65% by employing CuSO4•5H2O as catalyst (entry 7). Meanwhile, other catalysts such as FeCl3, FeCl2, Cu(OTf)2, and CuCl were screened. Unfortunately, no positive results were obtained (entries 8-11). In addition, the reaction was completely shut down in the absence of metal catalysts (entry 12). Finally, the desired product 3a was obtained in 86% isolated yield when the solvent was reduced to 1 mL (entry 13). The reaction showed low reactivity at room temperature (entry 14). Afterward, the efficiency of different directing groups was investigated. And no desired product was achieved when Ac, Ts and 2-pym were tried (entries 15-17). In addition, the use of the methyl group resulted in a marked decreased in selectivity and yield (entry 18).
With an optimized protocol in hand, the scope and limitation of the title reaction was explored (Scheme 3). With respect to the various indole derivatives, the reaction was found quite general and tolerated by various functional groups. A wide range of 2-trifluoromethylated products with substituent groups such as methyl (3b, 3i), methoxy (3c), acetyl (3d), esters (3e, 3j, 3k), and halogen (3f-3h, 3l-3o) at 4-, 5- and 6-position of indole were produced in moderate to good yields. In particular, halides, such as F, Cl, and Br, were well tolerated, affording the desired 2-trifluoromethylated products (3f-3h and 3l-3o) in good yields of 67–89%. However, C7-substituted indoles are not reactive under the optimized reaction conditions, which is presumed due to the steric hindrance. In addition, owing to the strong electron-withdrawing property, the indoles containing cyanide and nitro are not reactive.
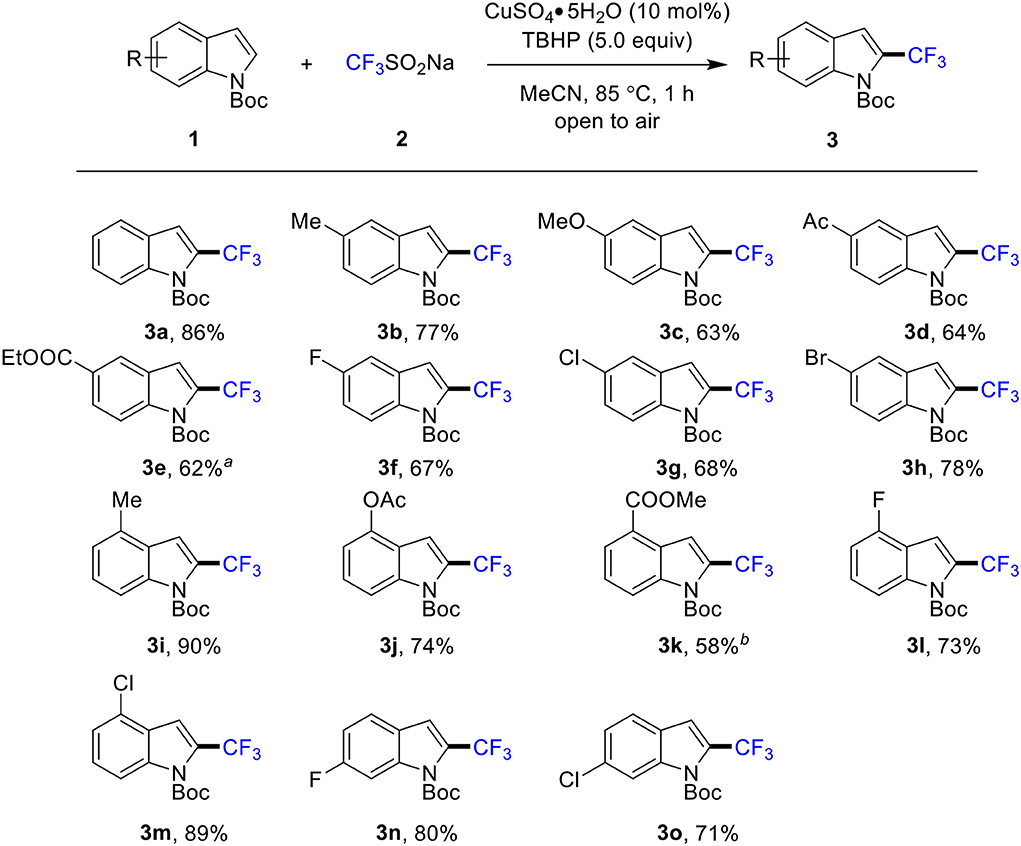
Scheme 3. Scope of indoles. Conditions: 1 (0.5 mmol), 2 (1.5 mmol), CuSO4•5H2O (10 mol %), MeCN (1.0 mL), 85°C, 1 h, in air. Isolated yield. a 12 h. b 5 h.
To extend the substrate scope of the above reaction, we proceeded to study the trifluoromethylation of other aromatics under the optimized reaction conditions. As shown in Scheme 4, pyrroles reacted smoothly to afford the corresponding 2-trifluoromethylated pyrroles (4a-4d) in good yields. Conventionally, direct deprotonation of benzofuran takes place at the most acidic C2 position (Larbi et al., 2017; Wang et al., 2018). Following C2 deprotonation, we obtained 2-trifluoromethylated benzofuran 4e in 88% through a radical addition mechanism. Notably, benzothiophene was also examined, but only a trace amount of product 4f was detected. Acetanilide, a drug to relieve pain or reduce fever, was also used for the synthesis of 4g. Additionally, we tried other “indole-like” compounds, but the products (4h-4k) were not gained.
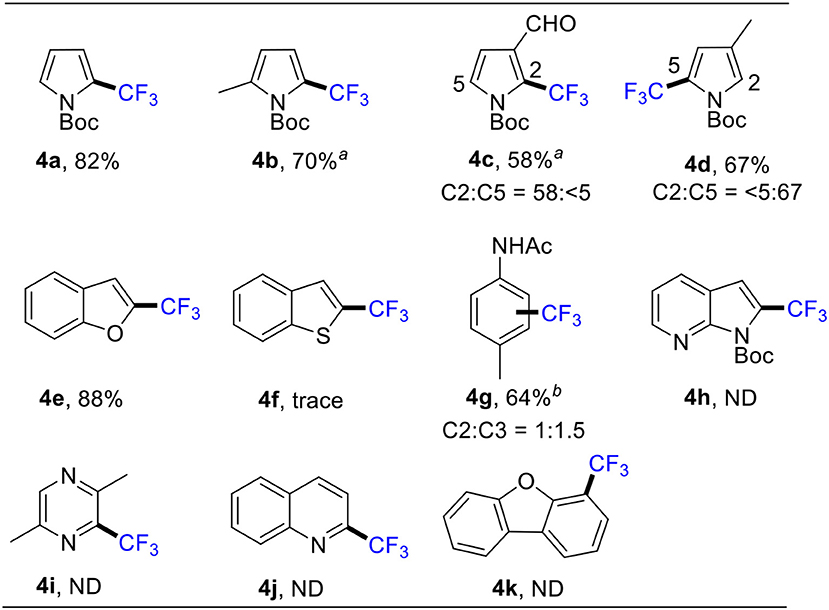
Scheme 4. Scope of other heteroarenes. Conditions: 1 (0.5 mmol), 2 (1.5 mmol), CuSO4•5H2O (10 mol %), MeCN (1.0 mL), 85°C, 1 h, in air. Isolated yield. a NMR yield. b Using 6 equiv of 2 and 10 equiv of TBHP, 12h.
Mechanism
The radical scavenger experiments were conducted to gain some insights into the mechanism of this reaction (Scheme 5). When radical inhibitors such as TEMPO (2,2,6,6-tetramethyl-1-piperidinyloxy) and BHT (butylated hydroxytoluene) were added, the reaction was suppressed to a great extent. Also, 19F NMR analysis showed that radical trapping product TEMPO/BHT-CF3 was formed dominantly. Therefore, we speculated that the high C2 selective is due to the formation of a five membered metallacycle at the C2 position through N-Boc-directed C-H activation (Sandtorv, 2015; Yang et al., 2016).
Based on the analysis of the aforementioned results and previous reports, a plausible mechanism was proposed in Scheme 6 (Langlois et al., 1991; Ji et al., 2011; Zhang et al., 2018; Khan et al., 2019). Initially, the t-butoxy radical, generated from copper metal, reacts with CF3 to provide •CF3SO2. This transient intermediate disproportionates, releasing SO2 and •CF3 B. Meanwhile, the copper catalyst was introduced to ensure the formation of a five membered metallacycle A at the C2 position. Subsequently, chelation-assisted C-H metalation of 1a A reacts with B to form C as a key intermediate. After reductive elimination the product 3a was formed and copper(II) catalyst regenerated.
Conclusion
In conclusion, we have developed a direct C2-H trifluoromethylation of indoles with the assistance of a removable directing group under ambient conditions. This transformation exhibits high regioselectivity, functional group tolerance and provide a practical method to various trifluoromethylated heteroarenes including indoles, pyrroles, benzofuran, and acetanilide. What is more, control experiments testified that a radical mechanism may be involved in the reaction.
Materials and Methods
General
1H NMR spectra were recorded on Bruker 500 MHz spectrometer and the chemical shifts were reported in parts per million (δ) relative to internal standard TMS (0 ppm) for CDCl3. The peak patterns are indicated as follows: s, singlet; d, doublet; dd, doublet of doublet; t, triplet; m, multiplet; q, quartet. The coupling constants, J, are reported in Hertz (Hz). 13C NMR spectra were obtained at Bruker 126 MHz and referenced to the internal solvent signals (central peak is 77.0 ppm in CDCl3). The NMR yield was determined by 1H NMR using CH2Br2 as an internal standard. APEX II (Bruker Inc.) was used for ESI-HRMS. Flash column chromatography was performed over silica gel 200–300. All reagents were weighed and handled in air at room temperature. All chemical reagents were purchased from Alfa, Acros, Aldrich, and TCI, J&K and used without further purification.
General Procedure and Characterization Data for Product 3, 4
To a mixture of N-Boc indole 1 (0.5 mmol), CF3SO2Na 2 (1.5 mmol) and CuSO4•5H2O (10 mol%), MeCN (1.0 mL) was added in air at room temperature. tert-Butyl hydroperoxide (TBHP, 70% solution in H2O, 2.5 mmol) was dropped into the mixture in air at room temperature. The resulting mixture was stirred at 85°C in air for 1 h. Once the mixture was cooled to room temperature, the solvent was removed under reduced pressure. The crude product was purified by flash column chromatography on silica gel (petroleum ether/CH2Cl2) to give product 3 or 4 as colorless oil. The NMR spectra of synthesized compounds are depicted in Supplementary Material.
Tert-Butyl 2-(Trifluoromethyl)-1H-Indole-1-Carboxylate (3a) (Xu et al., 2011; Arimori and Shibata, 2015) (123 mg, 86%)
Isolated by flash column chromatography (petroleum ether/CH2Cl2 = 50:1, Rf = 0.3). 1H NMR (500 MHz, CDCl3) δ 8.28 (d, J = 8.5 Hz, 1H), 7.62 (d, J = 7.9 Hz, 1H), 7.45 (t, J = 7.9 Hz, 1H), 7.30 (t, J = 7.5 Hz, 1H), 7.14 (s, 1H), 1.68 (s, 9H). 13C NMR (126 MHz, CDCl3) δ 148.57, 137.70, 126.98, 126.93 (q, J = 38.9 Hz), 126.46, 123.51, 122.77 (q, J = 266.6 Hz), 121.99, 116.04, 113.43 (q, J = 5.0 Hz), 85.43, 27.86. 19F NMR (470 MHz, CDCl3) δ−58.15. HRMS (ESI) caculated for C9H5NF3 [M-Boc]−, 184.0374; found: 184.0380.
Tert-Butyl 5-Methyl-2-(Trifluoromethyl)-1H-Indole-1-Carboxylate (3b) (115 mg, 77%)
Isolated by flash column chromatography (petroleum ether/CH2Cl2 = 50:1, Rf = 0.3). 1H NMR (500 MHz, CDCl3) δ 8.14 (d, J = 8.7 Hz, 1H), 7.39 (s, 1H), 7.27 (d, J = 7.5 Hz, 1H), 7.06 (s, 1H), 2.45 (s, 3H), 1.67 (s, 9H). 13C NMR (126 MHz, CDCl3) δ 148.61, 135.95, 133.07, 128.52, 126.83 (q, J = 40.4 Hz), 126.65, 121.63, 120.78 (q, J = 266.1 Hz), 115.67, 113.19 (q, J = 5.1 Hz), 85.20, 27.86, 21.17. 19F NMR (470 MHz, CDCl3) δ−58.15. HRMS (ESI) caculated for C10H7NF3 [M-Boc]−, 198.0531; found: 198.0536.
Tert-Butyl 5-Methoxy-2-(Trifluoromethyl)-1H-Indole-1-Carboxylate (3c) (99 mg, 63%)
Isolated by flash column chromatography (petroleum ether/CH2Cl2 = 50:1, Rf = 0.3). 1H NMR (500 MHz, CDCl3) δ 8.17 (d, J = 9.2 Hz, 1H), 7.08 – 7.05 (m, 2H), 7.03 (d, J = 2.4 Hz, 1H), 3.86 (s, 3H), 1.66 (s, 9H). 13C NMR (126 MHz, CDCl3) δ 156.25, 148.52, 132.44, 127.24 (q, J = 38.6 Hz), 127.17, 120.68 (q, J = 266.4 Hz), 116.98, 116.53, 113.14 (q, J = 5.1 Hz), 103.45, 85.26, 55.63, 27.86. 19F NMR (470 MHz, CDCl3) δ−58.24. HRMS (ESI) caculated for C10H7ONF3 [M-Boc]−, 214.0480; found: 214.0485.
Tert-Butyl 5-Acetyl-2-(Trifluoromethyl)-1H-Indole-1-Carboxylate (3d) (105 mg, 64%)
Isolated by flash column chromatography (petroleum ether/ ethyl acetate = 50:1, Rf = 0.3). 1H NMR (500 MHz, CDCl3) δ 8.34 (d, J = 8.9 Hz, 1H), 8.25 (d, J = 1.2 Hz, 1H), 8.06 (dd, J = 9.0, 1.7 Hz, 1H), 7.22 (s, 1H), 2.67 (s, 3H), 1.68 (s, 9H). 13C NMR (126 MHz, CDCl3) δ 197.39, 148.14, 140.21, 132.93, 128.42 (q, J = 38.9 Hz), 126.85, 126.23, 123.23, 122.52 (q, J = 266.9 Hz), 116.06, 113.85 (q, J = 5.0 Hz), 86.31, 27.78, 26.68. 19F NMR (470 MHz, CDCl3) δ−58.38. HRMS (ESI) caculated for C11H7ONF3 [M-Boc]−, 226.0480; found: 226.0485.
1-(Tert-Butyl) 5-Ethyl 2-(Trifluoromethyl)-1H-Indole-1,5-Dicarboxylate (3e) (111 mg, 62%)
Isolated by flash column chromatography (petroleum ether/ ethyl acetate = 50:1, Rf = 0.3). 1H NMR (500 MHz, CDCl3) δ 8.36 (d, J = 0.9 Hz, 1H), 8.32 (d, J = 8.9 Hz, 1H), 8.13 (dd, J = 8.9, 1.5 Hz, 1H), 7.20 (s, 1H), 4.41 (q, J = 7.1 Hz, 2H), 1.68 (s, 9H), 1.43 (t, J = 7.1 Hz, 3H). 13C NMR (126 MHz, CDCl3) δ 166.43, 148.21, 140.16, 128.23 (q, J = 39.3 Hz), 127.97, 126.15, 125.99, 124.32, 120.45 (q, J = 266.5 Hz), 115.83, 113.70 (q, J = 5.0 Hz), 86.18, 61.08, 27.79, 14.36. 19F NMR (470 MHz, CDCl3) δ−58.35. HRMS (ESI) caculated for C12H9F3NO2 [M-Boc]−, 256.0585; found: 256.0591.
Tert-Butyl 5-Fluoro-2-(Trifluoromethyl)-1H-Indole-1-Carboxylate (3f) (102 mg, 67%)
Isolated by flash column chromatography (petroleum ether/CH2Cl2 = 50:1, Rf = 0.3). 1H NMR (500 MHz, CDCl3) δ 8.26 (dd, J = 9.2, 4.5 Hz, 1H), 7.28 – 7.25 (m, 1H), 7.18 (td, J = 9.2, 2.5 Hz, 1H), 7.09 (s, 1H), 1.67 (s, 9H). 13C NMR (126 MHz, CDCl3) δ 160.30, 158.38, 148.34, 134.09, 128.30 (q, J = 39.1 Hz), 120.47 (q, J = 266.5 Hz), 117.41 (d, J = 8.9 Hz), 115.22 (d, J = 25.0 Hz), 112.84 (q, J = 4.9 Hz), 107.07 (d, J = 23.8 Hz), 85.80, 27.81. 19F NMR (470 MHz, CDCl3) δ−58.42,−119.41 (td, J = 8.5, 4.7 Hz). HRMS (ESI) caculated for C9H4NF4 [M-Boc]−, 202.0280; found: 202.0285.
Tert-Butyl 5-Chloro-2-(Trifluoromethyl)-1H-Indole-1-Carboxylate (3g) (109 mg, 68%)
Isolated by flash column chromatography (petroleum ether/CH2Cl2 = 50:1, Rf = 0.3). 1H NMR (500 MHz, CDCl3) δ 8.22 (d, J = 9.0 Hz, 1H), 7.58 (d, J = 2.0 Hz, 1H), 7.39 (dd, J = 9.0, 2.0 Hz, 1H), 7.06 (s, 1H), 1.67 (s, 9H). 13C NMR (126 MHz, CDCl3) δ 148.22, 136.05, 129.18, 128.10 (q, J = 39.3 Hz), 127.49, 127.28, 121.36, 120.43 (q, J = 266.5 Hz), 117.28, 112.45 (q, J = 5.1 Hz), 85.98, 27.80. 19F NMR (470 MHz, CDCl3) δ−58.39. HRMS (ESI) caculated for C9H4NClF3 [M-Boc]−, 217.9984; found: 217.9990.
Tert-Butyl 5-Bromo-2-(Trifluoromethyl)-1H-Indole-1-Carboxylate (3h) (142 mg, 78%)
Isolated by flash column chromatography (petroleum ether/CH2Cl2 = 50:1, Rf = 0.3). 1H NMR (500 MHz, CDCl3) δ 8.17 (d, J = 9.0 Hz, 1H), 7.75 (d, J = 1.7 Hz, 1H), 7.53 (dd, J = 9.0, 1.9 Hz, 1H), 7.06 (s, 1H), 1.67 (s, 9H). 13C NMR (126 MHz, CDCl3) δ 148.20, 136.41, 129.92, 128.03, 127.95 (q, J = 39.2 Hz), 124.48, 120.39 (q, J = 266.5 Hz), 117.62, 116.75, 112.32 (q, J = 5.0 Hz), 86.01, 27.80. 19F NMR (470 MHz, CDCl3) δ−58.37. HRMS (ESI) caculated for C9H4NBrF3 [M-Boc]−, 261.9479; found: 261.9485.
Tert-Butyl 4-Methyl-2-(Trifluoromethyl)-1H-Indole-1-Carboxylate (3i) (135 mg, 90%)
Isolated by flash column chromatography (petroleum ether/CH2Cl2 = 50:1, Rf = 0.3). 1H NMR (500 MHz, CDCl3) δ 8.10 (d, J = 8.5 Hz, 1H), 7.34 (t, J = 7.9 Hz, 1H), 7.18 (s, 1H), 7.09 (d, J = 7.2 Hz, 1H), 2.54 (s, 3H), 1.67 (s, 9H). 13C NMR (126 MHz, CDCl3) δ 148.63, 137.60, 131.57, 127.10, 126.31 (q, J = 38.9 Hz), 126.22, 123.81, 120.85 (q, J = 266.3 Hz), 113.51, 111.86 (q, J = 5.1 Hz), 85.33, 27.85, 18.23. 19F NMR (470 MHz, CDCl3) δ−57.97. HRMS (ESI) caculated for C10H7NF3 [M-Boc]−, 198.0531; found: 198.0536.
Tert-Butyl 4-Acetoxy-2-(Trifluoromethyl)-1H-Indole-1-Carboxylate (3j) (127 mg, 74%)
Isolated by flash column chromatography (petroleum ether/ ethyl acetate = 100:1, Rf = 0.3). 1H NMR (500 MHz, CDCl3) δ 8.16 (d, J = 8.6 Hz, 1H), 7.43 (t, J = 8.2 Hz, 1H), 7.07 (dd, J = 7.9, 0.6 Hz, 1H), 7.05 (s, 1H), 2.40 (s, 3H), 1.67 (s, 9H). 13C NMR (126 MHz, CDCl3) δ 168.91, 148.30, 144.04, 138.98, 127.46, 127.17 (q, J = 39.0 Hz), 120.46 (q, J = 266.4 Hz), 120.07, 115.80, 113.96, 109.74 (q, J = 5.3 Hz), 85.88, 27.80, 20.96. 19F NMR (470 MHz, CDCl3) δ−58.27. HRMS (ESI) caculated for C11H7O2NF3 [M-Boc]−, 242.0429; found: 242.0434.
1-(Tert-Butyl) 4-Methyl 2-(Trifluoromethyl)-1H-Indole-1,4-Dicarboxylate (3k) (100 mg, 58%)
Isolated by flash column chromatography (petroleum ether/ ethyl acetate = 50:1, Rf = 0.3). 1H NMR (500 MHz, CDCl3) δ 8.54 (d, J = 8.5 Hz, 1H), 8.04 (dd, J = 7.6, 0.8 Hz, 1H), 7.85 (s, 1H), 7.50 (t, J = 8.2 Hz, 1H), 4.00 (s, 3H), 1.67 (s, 9H). 13C NMR (126 MHz, CDCl3) δ 166.68, 148.35, 138.28, 128.38 (q, J = 38.9 Hz), 126.41, 126.34, 126.14, 123.10, 120.72, 120.59 (q, J = 266.8 Hz), 113.77 (q, J = 5.4 Hz), 86.04, 52.13, 27.79. 19F NMR (470 MHz, CDCl3) δ−58.20. HRMS (ESI) caculated for C11H7O2NF3 [M-Boc]−, 242.0429; found: 242.0434.
Tert-Butyl 4-Fluoro-2-(Trifluoromethyl)-1H-Indole-1-Carboxylate (3l) (111 mg, 73%)
Isolated by flash column chromatography (petroleum ether/CH2Cl2 = 50:1, Rf = 0.3). 1H NMR (500 MHz, CDCl3) δ 8.06 (d, J = 8.6 Hz, 1H), 7.38 (td, J = 8.3, 5.6 Hz, 1H), 7.24 (s, 1H), 6.97 (t, J = 9.0, 1H), 1.67 (s, 9H). 13C NMR (126 MHz, CDCl3) δ 157.23, 155.23, 148.34, 139.54, 127.84 (d, J = 7.5 Hz), 127.01 (q, J = 39.1 Hz), 120.42 (q, J = 266.5 Hz), 112.11 (d, J = 4.0 Hz), 108.91 (q, J = 5.4 Hz), 108.51 (d, J = 17.9 Hz), 86.02, 27.80. 19F NMR (470 MHz, CDCl3) δ−58.31,−120.93 (q, J = 9.4 Hz). HRMS (ESI) caculated for C9H4NF4 [M-Boc]−, 202.0280; found: 202.0285.
Tert-Butyl 4-Chloro-2-(Trifluoromethyl)-1H-Indole-1-Carboxylate (3m) (142 mg, 89%)
Isolated by flash column chromatography (petroleum ether/CH2Cl2 = 50:1, Rf = 0.3). 1H NMR (500 MHz, CDCl3) δ 8.19 (d, J = 8.5 Hz, 1H), 7.36 (t, J = 8.1 Hz, 1H), 7.29 (dd, J = 7.8, 0.6 Hz, 1H), 7.27 (s, 1H), 1.67 (s, 9H). 13C NMR (126 MHz, CDCl3) δ 148.23, 138.29, 127.62, 127.46 (q, J = 35.8 Hz), 127.28, 125.45, 123.27, 120.46 (q, J = 266.3 Hz), 114.66, 111.37 (q, J = 5.3 Hz), 86.10, 27.80. 19F NMR (470 MHz, CDCl3) δ−58.30. HRMS (ESI) caculated for C9H4NClF3 [M-Boc]−, 217.9984; found: 217.9990.
Tert-Butyl 6-Fluoro-2-(Trifluoromethyl)-1H-Indole-1-Carboxylate (3n) (121 mg, 80%)
Isolated by flash column chromatography (petroleum ether/CH2Cl2 = 50:1, Rf = 0.3). 1H NMR (500 MHz, CDCl3) δ 8.02 (dd, J = 10.6, 1.8 Hz, 1H), 7.55 (dd, J = 8.5, 5.5 Hz, 1H), 7.10 (s, 1H), 7.06 (td, J = 8.7, 1.7 Hz, 1H), 1.67 (s, 9H). 13C NMR (126 MHz, CDCl3) δ 163.29, 161.36, 148.33, 122.93 (d, J = 10.1 Hz), 122.74, 120.51 (q, J = 266.1 Hz), 113.15 (q, J = 4.9 Hz), 112.38 (d, J = 24.6 Hz), 103.36 (d, J = 29.1 Hz), 102.90 (q, J = 28.5 Hz), 85.92, 27.81. 19F NMR (470 MHz, CDCl3) δ−58.26,−112.94 (td, J = 9.6, 5.6 Hz). HRMS (ESI) caculated for C9H4NF4 [M-Boc]−, 202.0280; found: 202.0285.
Tert-Butyl 6-Chloro-2-(Trifluoromethyl)-1H-Indole-1-Carboxylate (3o) (113 mg, 71%)
Isolated by flash column chromatography (petroleum ether/CH2Cl2 = 50:1, Rf = 0.3). 1H NMR (500 MHz, CDCl3) δ 8.35 (s, 1H), 7.53 (d, J = 8.4 Hz, 1H), 7.30 – 7.26 (m, 1H), 7.10 (s, 1H), 1.67 (s, 9H). 13C NMR (126 MHz, CDCl3) δ 148.22, 138.03, 133.12, 127.48 (q, J = 39.1 Hz), 124.87, 124.37, 122.72, 120.48 (q, J = 266.5 Hz), 116.37, 113.03 (q, J = 5.0 Hz), 86.07, 27.80. 19F NMR (470 MHz, CDCl3) δ−58.30. HRMS (ESI) caculated for C9H4NClF3 [M-Boc]−, 217.9984; found: 217.9990.
Tert-Butyl 2-(Trifluoromethyl)-1H-Pyrrole-1-Carboxylate (4a) (Nagib and MacMillan, 2011; Du et al., 2017) (96 mg, 82%)
Isolated by flash column chromatography (petroleum ether/CH2Cl2 = 50:1, Rf = 0.3). 1H NMR (500 MHz, CDCl3) δ 7.44 (d, J = 1.9 Hz, 1H), 6.73 (s, 1H), 6.19 (t, J = 3.2 Hz, 1H), 1.61 (s, 9H). 13C NMR (126 MHz, CDCl3) δ 125.78, 120.53 (q, J = 264.9 Hz), 117.76 (q, J = 4.6 Hz), 109.59, 85.62, 27.71. 19F NMR (470 MHz, CDCl3) δ−58.33. HRMS (ESI) caculated for C5H3NF3 [M-Boc]−, 134.0218; found: 134.0223.
Tert-Butyl 2-Methyl-5-(Trifluoromethyl)-1H-Pyrrole-1-Carboxylate (4b)
Isolated by flash column chromatography (petroleum ether/CH2Cl2 = 50:1, Rf = 0.3). 1H NMR (500 MHz, CDCl3) δ 6.59 (d, J = 3.5 Hz, 1H), 5.92 (d, J = 3.2 Hz, 1H), 2.44 (s, 3H), 1.60 (s, 9H). 13C NMR (126 MHz, CDCl3) δ 148.43, 137.08, 121.58 (q, J = 39.3 Hz), 120.77 (q, J = 264.5 Hz), 116.00 (q, J = 4.8 Hz), 109.96, 85.39, 31.60, 27.63. 19F NMR (470 MHz, CDCl3) δ−57.19. HRMS (ESI) caculated for C6H5NF3 [M-Boc]−, 148.0374; found: 148.0380.
Tert-Butyl 3-Formyl-2-(Trifluoromethyl)-1H-Pyrrole-1-Carboxylate (4c)
Isolated by flash column chromatography (petroleum ether/ ethyl acetate = 50:1, Rf = 0.3). 1H NMR (500 MHz, cdcl3) δ 10.18 (s, 1H), 7.43 (d, J = 3.3 Hz, 1H), 6.72 (d, J = 3.4 Hz, 1H), 1.63 (s, 9H). 13C NMR (126 MHz, CDCl3) δ 185.88 (q, J = 5.6 Hz), 171.10, 146.75, 125.86, 123.69 (q, J = 41.4 Hz), 120.46 (q, J = 267.8 Hz), 109.08, 87.39, 27.57. 19F NMR (470 MHz, CDCl3) δ−54.31. HRMS (ESI) caculated for C6H3ONF3 [M-Boc]−, 162.0167; found: 162.0172.
Tert-Butyl 4-Methyl-2-(Trifluoromethyl)-1H-Pyrrole-1-Carboxylate (4d) (83 mg, 67%)
Isolated by flash column chromatography (petroleum ether/CH2Cl2 = 50:1, Rf = 0.3). 1H NMR (500 MHz, CDCl3) δ 7.32 (s, 1H), 6.01 (s, 1H), 2.21 (s, 3H), 1.59 (s, 9H). 13C NMR (126 MHz, CDCl3) δ 147.67, 128.96 (q, J = 2.6 Hz), 124.61, 121.68 (q, J = 266.0 Hz), 119.54 (q, J = 4.4 Hz), 117.06 (q, J = 38.3 Hz), 113.52, 85.09, 27.69. 19F NMR (376 MHz, CDCl3) δ−54.63. HRMS (ESI) caculated for C6H5NF3 [M-Boc]−, 148.0374; found: 148.0380.
2-(Trifluoromethyl)Benzofuran (4e) (Liu and Shen, 2011) (82 mg, 88%)
Isolated by flash column chromatography (petroleum ether, Rf = 0.3). 1H NMR (500 MHz, CDCl3) δ 7.67 (d, J = 7.8 Hz, 1H), 7.58 (d, J = 8.0 Hz, 1H), 7.45 (t, J = 7.4 Hz, 1H), 7.34 (t, J = 7.2 Hz, 1H), 7.18 (s, 1H). 13C NMR (126 MHz, CDCl3) δ 155.13, 143.48 (q, J = 41.9 Hz), 126.90, 125.99, 123.95, 122.46, 119.31 (q, J = 266.5 Hz), 112.09, 108.09 (q, J = 3.1 Hz). 19F NMR (470 MHz, CDCl3) δ -64.87.
N-(4-Methyl-2-(Trifluoromethyl)Phenyl)Acetamide (4g) (Zou et al., 2019) (28 mg, 26%)
Isolated by flash column chromatography (petroleum ether/ ethyl acetate = 5:1, Rf = 0.3). 1H NMR (500 MHz, CDCl3) δ 7.95 (d, J = 8.1 Hz, 1H), 7.40 (s, 1H), 7.34 (d, J = 7.8 Hz, 2H), 2.36 (s, 3H), 2.19 (s, 3H). 13C NMR (126 MHz, CDCl3) δ 168.48, 134.75, 133.28, 132.45, 126.33 (q, J = 4.9 Hz), 125.20, 124.00 (q, J = 271.5 Hz), 120.65 (q, J = 29.4 Hz), 24.43, 20.79. 19F NMR (470 MHz, CDCl3) δ−60.67. HRMS (ESI) caculated for C10H11ONF3 [M+H]+, 218.0793; found: 218.0787.
N-(4-Methyl-3-(Trifluoromethyl)Phenyl)Acetamide (4g) (41 mg, 38%)
Isolated by flash column chromatography (petroleum ether/ ethyl acetate = 5:1, Rf = 0.3). 1H NMR (500 MHz, CDCl3) δ 7.64 (d, J = 10.7 Hz, 2H), 7.57 (s, 1H), 7.21 (d, J = 8.1 Hz, 1H), 2.42 (s, 3H), 2.17 (s, 3H). 13C NMR (126 MHz, CDCl3) δ 168.60, 135.70, 132.48, 132.26, 129.18 (q, J = 29.9 Hz), 124.13 (q, J = 272.4 Hz), 123.00, 117.40 (q, J = 5.9 Hz), 24.41, 18.70. 19F NMR (470 MHz, CDCl3) δ−61.97. HRMS (ESI) caculated for C10H11ONF3 [M+H]+, 218.0793; found: 218.0787.
Data Availability
All datasets generated for this study are included in the manuscript/Supplementary Files.
Author Contributions
XS, XianL, and DS constructed the workflow. XS synthesized and purified the compounds. XS and XiaoL performed the mass spectrometric analysis. XS completed the paper.
Funding
This work was supported by the Funded by National Natural Science Foundation of China (No. 81773586, 81703354), and Key research and development project of Shandong province (2016GSF201193, 2016ZDJS07A13, 2016GSF115002, 2016GSF115009), and Key Research Program of Frontier Sciences, CAS (QYZDB-SSW-DQC014), and the Project of Discovery, Evaluation and Transformation of Active Natural Compounds, Strategic Biological Resources Service Network Program of Chinese Academy of Sciences (ZSTH-026), and Shandong Provincial Natural Science Foundation for Distinguished Young Scholars (JQ201722), and National Program for Support of Top-notch Young Professionals, and Taishan scholar Youth Project of Shandong province.
Conflict of Interest Statement
The authors declare that the research was conducted in the absence of any commercial or financial relationships that could be construed as a potential conflict of interest.
Supplementary Material
The Supplementary Material for this article can be found online at: https://www.frontiersin.org/articles/10.3389/fchem.2019.00613/full#supplementary-material
References
Arimori, S., and Shibata, N. (2015). Catalytic trifluoromethylation of aryl- and vinylboronic acids by 2-cyclopropyl-1-(trifluoromethyl)benzo[b]thiophenium triflate. Org. Lett. 17, 1632–1635. doi: 10.1021/acs.orglett.5b00164
Boechat, N., and Bastos, M. M. (2010). Trifluoromethylation of carbonyl compounds. Curr. Org. Synth. 7, 403–413. doi: 10.2174/157017910792246081
Chripkova, M., Zigo, F., and Mojzis, J. (2016). Antiproliferative effect of indole phytoalexins. Molecules 21, 1626–1640. doi: 10.3390/molecules21121626
Du, Y., Pearson, R. M., Lim, C.-H., Sartor, S. M., Ryan, M. D., Yang, H., et al. (2017). Strongly reducing, visible-lightorganic photoredox catalysts as sustainable alternatives to precious metals. Chem. Eur. J. 23, 10962–10968. doi: 10.1002/chem.201702926
Furuya, T., Kamlet, A. S., and Ritter, T. (2011). Catalysis for fluorination and trifluoromethylation. Nature 473, 470–477. doi: 10.1038/nature10108
Gouverneur, V., and Seppelt, K. (2015). Introduction: fluorine chemistry. Chem. Rev. 115, 563–565. doi: 10.1021/cr500686k
Goyal, D., Kaur, A., and Goyal, B. (2018). Benzofuran and indole: promising scaffolds for drug development in Alzheimer's disease. Chem. Med. Chem. 13, 1275–1299. doi: 10.1002/cmdc.201800156
Hagmann, W. K. (2008). The many roles for fluorine in medicinal chemistry. J. Med. Chem. 51, 4359–4369. doi: 10.1021/jm800219f
Iqbal, N., Choi, S., Ko, E., and Cho, E. J. (2012). Trifluoromethylation of heterocycles via visible light photoredox catalysis. Tetrahedron Lett. 53, 2005–2008. doi: 10.1016/j.tetlet.2012.02.032
Ji, Y., Brueckl, T., Baxter, R. D., Fujiwara, Y., Seiple, I. B., Su, S., et al. (2011). Innate C-H trifluoromethylation of heterocycles. Proc. Natl Acad. Sci. U. S.A. 108, 14411–14415. doi: 10.1073/pnas.1109059108
Kaur, H., Singh, J., and Narasimhan, B. (2019). Indole hybridized diazenyl derivatives: synthesis, antimicrobial activity, cytotoxicity evaluation and docking studies. BMC Chem. 13, 65–82. doi: 10.1186/s13065-019-0580-0
Khan, R., Kumar, M., Kant, R., and Koley, D. (2019). Cu-catalyzed directed C7–H imidation of indolines via crossdehydrogenative coupling. Adv. Synth. Catal. 369, 3108–3113. doi: 10.1002/adsc.201900166
Langlois, B. R., Laurent, E., and Roidot, N. (1991). Trifluoromethylation of aromatic compounds with sodium trifluoromethanesulfinate under oxidative conditions. Tetrahedron Lett. 32, 7525–7528. doi: 10.1016/0040-4039(91)80524-A
Larbi, K. S., Djebbar, S., Soul,é, J.-F., and Doucet, H. (2017). Reactivity of benzofuran and benzothiophene in palladium-catalysed direct C2, C3-diarylations. J. Organomet. Chem. 843, 32–39. doi: 10.1016/j.jorganchem.2017.05.029
Le, C., Chen, T. Q., Liang, T., Zhang, P., and MacMillan, D. W. C. (2018). A radical approach to the copper oxidative addition problem: trifluoromethylation of bromoarenes. Science 360, 1010–1014. doi: 10.1126/science.aat4133
Lee, J.-H., Wood, T. K., and Lee, J. (2015). Roles of indole as an interspecies and interkingdom signaling molecule. Trends Microbiol. 23, 707–718. doi: 10.1016/j.tim.2015.08.001
Liu, H., Gu, Z., and Jiang, X. (2013). Direct trifluoromethylation of the C-H bond. Adv. Synth. Catal. 355, 617–626. doi: 10.1002/adsc.201200764
Liu, T., and Shen, Q. (2011). Copper-catalyzed trifluoromethylation of aryl and vinyl boronic acids with an electrophilic trifluoromethylating reagent. Org. Lett. 13, 2342–2345. doi: 10.1021/ol2005903
Nagib, D. A., and MacMillan, D. W. (2011). Trifluoromethylation of arenes and heteroarenes by means of photoredox catalysis. Nature 480, 224–228. doi: 10.1038/nature10647
Nie, J., Guo, H.-C., Cahard, D., and Ma, J.-A. (2011). Asymmetric construction of stereogenic carbon centers featuring a trifluoromethyl group from prochiral trifluoromethylated substrates. Chem. Rev. 111, 455–529. doi: 10.1021/cr100166a
Nishino, M., Hirano, K., Satoh, T., and Miura, M. (2012). Copper-mediated and copper-catalyzed cross-coupling of indoles and 1,3-azoles: double C-H activation. Angew. Chem. Int. Ed. 51, 6993–6997. doi: 10.1002/anie.201201491
Sandtorv, A. H. (2015). Transition metal-catalyzed C-H activation of indoles. Adv. Synth. Catal. 357, 2403–2435. doi: 10.1002/adsc.201500374
Sato, K., Tarui, A., Omote, M., Ando, A., and Kumadaki, I. (2010). Trifluoromethylation of organic compounds and related reactions. Synthesis 11, 1865–1882. doi: 10.1055/s-0029-1218745
Schlosser, M. (2006). CF3-bearing aromatic and heterocyclic building blocks. Angew. Chem. Int. Ed. 45, 5432–5446. doi: 10.1002/anie.200600449
Shi, X., Li, X., Ma, L., and Shi, D. (2018). Cu(II)-catalyzed oxidative trifluoromethylation of indoles with KF as the base. Catalysts 9, 278–291. doi: 10.3390/catal9030278
Shimizu, M., and Hiyama, T. (2005). Modern synthetic methods for fluorine-substituted target molecules. Angew. Chem. Int. Ed. 44, 214–231. doi: 10.1002/anie.200460441
Shimizu, R., Egami, H., Nagi, T., Chae, J., Hamashima, Y., and Sodeoka, M. (2010). Direct C2-trifluoromethylation of indole derivatives catalyzed by copper acetate. Tetrahedron Lett. 51, 5947–5949. doi: 10.1016/j.tetlet.2010.09.027
Soni, V., Sharma, D. M., and Punji, B. (2018). Nickel-catalyzed regioselective C(2)-H difluoroalkylation of indoles with difluoroalkyl bromides. Chem. Asian. J. 13, 2516–2522. doi: 10.1002/asia.201800504
Sravanthi, T. V., and Manju, S. L. (2016). Indoles — a promising scaffold for drug development. Eur. J. Pharm. Sci. 91, 1–10. doi: 10.1016/j.ejps.2016.05.025
Tomashenko, O. A., and Grushin, V. V. (2011). aromatic trifluoromethylation with metal complexes. Chem. Rev. 111, 4475–4521. doi: 10.1021/cr1004293
Wang, J., Sánchez-Rosell,ó, M., Aceña, J. L., Pozo, C., Sorochinsky, A. E., Fustero, S., et al. (2014). Fluorine in pharmaceutical industry: fluorine-containing drugs introduced to the market in the last decade (2001–2011). Chem. Rev. 114, 2432–2506. doi: 10.1021/cr4002879
Wang, Y., Yang, Y., Jie, K., Huang, L., Guo, S., and Cai, H. (2018). Copper-catalyzed C2 and C3 phosphonation of benzofuran and benzothiophene with trialkyl phosphites. ChemCatChem. 10, 716–717. doi: 10.1002/cctc.201701361
Xu, J., Luo, D.-F., Xiao, B., Liu, Z.-J., Gong, T.-J., Fu, Y., et al. (2011). Copper-catalyzed trifluoromethylation of aryl boronic acids using a reagent. Chem. Commun. 47, 4300–4302. doi: 10.1039/c1cc10359h
Yan, S.-Y., Zhang, Z.-Z., and Shi, B.-F. (2017). Nickel-catalyzed direct C–H trifluoroethylation of heteroarenes with trifluoroethyl iodide. Chem. Commun. 53, 10287–10290. doi: 10.1039/c7cc05532c
Yang, Y., Qiu, X., Zhao, Y., Mu, Y., and Shi, Z. (2016). Palladium-catalyzed C–H arylation of indoles at the C7 position. J. Am. Chem. Soc. 138, 495–498. doi: 10.1021/jacs.5b11569
Yu, D.-G., Gensch, T., Azambuja, F., Vásquez-Céspedes, S., and Glorius, F. (2014). Co(III)-Catalyzed C–H activation/formal SN-type reactions: selective and efficient cyanation, halogenation, and allylation. J. Am. Chem. Soc. 136, 17722–17725. doi: 10.1021/ja511011m
Zhang, H., Wang, H.-L., Luo, Y., Chen, C., Cao, Y., Chen, P., et al. (2018). Regioselective palladium-catalyzed C-H Bond trifluoroethylation of indoles: exploration and mechanistic insight. ACS Catal. 8, 2173–2180. doi: 10.1021/acscatal.7b03220
Zhou, B., Yang, Y., Lin, S., and Li, Y. (2013). Rhodium-catalyzed direct addition of indoles to N-sulfonylaldimines. Adv. Synth. Catal. 355, 360–364. doi: 10.1002/adsc.201200909
Keywords: C-H functionalization, trifluoromethylation, indoles, copper, radical reaction
Citation: Shi X, Li X, Li X and Shi D (2019) Ligand- and Additive-Free 2-Position-Selective Trifluoromethylation of Heteroarenes Under Ambient Conditions. Front. Chem. 7:613. doi: 10.3389/fchem.2019.00613
Received: 24 June 2019; Accepted: 22 August 2019;
Published: 06 September 2019.
Edited by:
Norio Shibata, Nagoya Institute of Technology, JapanReviewed by:
Yoshitaka Hamashima, University of Shizuoka, JapanTakashi Koike, Tokyo Institute of Technology, Japan
Thierry Billard, UMR5246 Institut de Chimie et Biochimie Moléculaires et Supramoléculaires (ICBMS), France
Copyright © 2019 Shi, Li, Li and Shi. This is an open-access article distributed under the terms of the Creative Commons Attribution License (CC BY). The use, distribution or reproduction in other forums is permitted, provided the original author(s) and the copyright owner(s) are credited and that the original publication in this journal is cited, in accordance with accepted academic practice. No use, distribution or reproduction is permitted which does not comply with these terms.
*Correspondence: Xiangqian Li, eHFsaUBxZGlvLmFjLmNu; Dayong Shi, c2hpZGF5b25nQHNkdS5lZHUuY24=