- 1Department of Chemical Sciences, University of Catania, Catania, Italy
- 2Faculty of Chemistry and Geosciences, University of Vilnius, Vilnius, Lithuania
- 3I.N.S.T.M. - Consorzio Interuniversitario Nazionale per la Scienza e Tecnologia dei Materiali, University of Catania, Catania, Italy
A novel uranyl salen-bis-porphyrin complex, in which two porphyrin subunits and salen moiety were directly linked, was synthesized for the recognition of tetrabutylammonium (TBA) amino acids. This uranyl salen complex, due to the presence of porphyrins with their fluorescence properties, represents the first example of a luminescence of uranyl salen complexes. UV/Vis measurements indicate the formation of 1:1 host–guest complexes, whereas UV-vis and fluorescence studies revealed that this complex acts as a receptor for the enantiomeric recognition of α-aminoacids derivatives, with high association constants and an excellent enantiomeric discrimination between the two enantiomers of phenylalanine–TBA.
Introduction
Salen ligands are a class of molecules that have been widely explored in the field of supramolecular chemistry. The most fascinating and promising use of salen derivatives is due to their chiral complexes with numerous metals. The salen structure, due to two contiguous stereogenic carbon atoms in the diimine bridge, creates a chiral pocket which can coordinate a metal cation (via imine nitrogen and phenolic oxygen atoms). Salen ligands rose to prominence thanks to the pioneering work of Jacobsen and Katsuki that paved the way to one of the most well-designed protocol for the enantioselective epoxidation of unfunctionalized alkenes catalyzed by chiral manganese salen complexes (Jacobsen, 1993; Katsuki, 2000; Yoon and Jacobsen, 2003; La Paglia Fragola et al., 2012; Trusso Sfrazzetto et al., 2015; Ballistreri et al., 2016, 2018; Zammataro et al., 2019). Furthermore, salen ligands are structures of great value in homogeneous catalysis (Katsuki, 1995; Jacobsen, 2000; Cozzi, 2004; McGarrigle and Gilheany, 2005; Baleizao and Garcia, 2006; Wezenberg and Kleij, 2008; Whiteoak et al., 2012).
In recent years, our research group exploited these chiral salen-metal complexes as enantiomeric receptors for chiral guests. In fact, depending on the metal ion and different substituents in the aromatic ring of salen framework, these salen-metal complexes can be used as efficient enantioselective catalysts and highly sensitive chemosensors (D'Urso et al., 2014; Puglisi et al., 2017, 2018, 2019). In particular, chiral uranyl salen complexes have proved to be excellent receptors for amino acid salts (Amato et al., 2007, 2010, 2011; Ballistreri et al., 2010; Pappalardo et al., 2012a), since the uranyl metal center, acting as a Lewis acid, possesses an equatorial fifth position able to coordinate one molecule of carboxylate anion (Ballistreri et al., 2012; Brancatelli et al., 2013). These synthetic enantioselective receptors could help to better understand the mechanisms of drugs action; processes that are involved in immunological responses and processes of the storage of genetic information. Besides, slightly modification of their structures could lead to chemosensors, which due to their simple use, relatively low cost and high sensitivity are particularly significant in the chemical analysis.
Taking into account the salen ligand applications in the field of catalysis and enantiomeric recognition, previous studies have inspired us to extend current research on the synthesis of salen receptors comprising porphyrin macrocycles which, with their high stability and fluorescence properties, could greatly extend the use of salen ligands as chemosensors. Porphyrins, due to their rigid molecular structure, tunable substituents, large skeleton dimensions, and additional metallation sites in the core, are very attractive macrocycles for their applications in many technological fields (Beletskaya et al., 2009; Drain et al., 2009). In our strategy, the rational combination of porphyrin derivatives with chiral uranyl-salen ligands in one structure, would lead to chemosensors that possess unprecedented luminescence properties, that till now were precluded in uranyl-salen complexes due to the presence of uranyl metal center. Here we report on the synthesis of a novel uranyl salen-bis-porphyrin complex, in which two porphyrin subunits and the salen ligand are directly connected, and the enantiomeric recognition properties of this receptor toward selected α-aminoacids derivatives assessed by UV-vis and fluorescence measurements (Figure 1).
Materials and Methods
General Experimental Methods
The NMR experiments were carried out at 27°C on a Varian UNITY Inova 500 MHz spectrometer (1H at 499.88 MHz, 13C NMR at 125.7 MHz) equipped with pulse field gradient module (Z axis) and a tuneable 5 mm Varian inverse detection probe (ID-PFG). ESI mass spectra were acquired on a API 2000– ABSciex using CH3OH (positive ion mode). A JASCO V-560 UV-Vis spectrophotometer equipped with a 1 cm path-length cell was used for the UV-Vis measurements. Luminescence measurements were carried out using a Cary Eclipse Fluorescence spectrophotometer with resolution of 0.5 nm, at room temperature. The emission was recorded at 90° with respect to the exciting line beam using 10:10 slit-widths for all measurements. All chemicals were reagent grade and were used without further purification.
General Procedure for the Synthesis of TBA Amino Acid Derivates (Ballistreri et al., 2010)
An aqueous solution of tetrabutylammonium hydroxide (40% w/w, 13 mmol) was added to an aqueous suspension of the desired amino acid (13 mmol). The resultant reaction mixture was heated at 60°C for 2 h. Water was removed in vacuo at 80°C. The residue was dissolved in CH2Cl2 (10 mL), filtered and the solvent was evaporated in vacuo to afford in high yield the desired product.
Procedure for UV-vis and Fluorescence Titrations
Two stock solutions of host and guest (1.0 × 10−3 M) in dry chloroform were prepared. From these, different solutions with different ratio receptor/guest (host concentration = 1.0 × 10−6 M) were prepared, and UV-vis and emission spectra were recorded at 25°C. Fluorescence titrations were carried out using λex = 350 nm in dry chloroform, recording at λem = 650 and 715 nm. With this data treatment, the apparent binding affinities of receptor with amino acid guests were estimated using HypSpec (version 1.1.33) (Pappalardo et al., 2012b), a software designed to extract equilibrium constants from potentiometric and/or spectrophotometric titration data. HypSpec starts with an assumed complex formation scheme and uses a least-squares approach to derive the spectra of the complexes and the stability constants. χ2-test (chi-square) was applied, where the residuals follow a normal distribution (for a distribution approximately normal, the χ2-test value is around 12 or less). In all of the cases, χ2 ≤ 10 were found, as obtained by 3 independent measurement sets.
Synthesis of Compound 4 (Johansson et al., 2003)
5.0 g (0.0300 mol) of compound 2 (Dalla Cort et al., 2006) and 5.97 g of potassium phthalimide 3 (0.0322 mol) were placed in a 250 mL round-bottom flask. The reagents were dissolved in 100 mL of DMF. The reaction mixture was allowed to stir at room temperature for 48 h and then was heated at 55°C for 4 h. The reaction mixture was cooled down to room temperature and diluted with 250 mL of EtOAc and washed with water (3 × 200 mL). The organic layer was dried over anhydrous Na2SO4, filtered and evaporated. The product was purified by silica gel column chromatography (CHCl3) to afford 3.37 g (40 % yield) of compound 4. 1H NMR (500 MHz, CDCl3): δ = 10.99 (s, 1H); 9.88 (s, 1H); 7.86-7.84 (m, 2H); 7.73-7.71 (m, 2H); 7.67 (s, 1H); 7.65-7.63 (dd, J = 8.5 Hz, J = 2.0 Hz, 1H); 6.95 (d, J = 8.5 Hz, 1H); 4.82 (s, 2H).
Synthesis of Compound 5
0.662 g (2.36 mmol) of compound 4 and 0.0171 g (0.036 mmol) of tetrabutylammonium tribromide were placed in a 20 mL round-bottom flask. In this flask 3.60 mL of 1,3-propanediol and 3.90 mL of triethyl orthoformate were added. The reaction mixture was allowed to stir at room temperature for 48 h, then diluted with 45 mL of EtOAc. The organic solution was washed with water (3 × 20 mL), dried over anhydrous Na2SO4, filtered and evaporated. The product was purified by silica gel column chromatography (cyclohexane/EtOAc 3:1) affording 0.732 g of white compound 5 (92% yield). 1H NMR (500 MHz, CDCl3): δ = 7.85 (s, 1H); 7.83–7.81 (m, 2H); 7.70–7.68 (m, 2H); 7.36–7.34 (dd, J = 8.5 Hz, J = 2.5 Hz, 1H); 7.28 (s, 1H); 6.84 (d, J = 8.5 Hz, 1H); 5.61 (s, 1H); 4.74 (s, 2H); 4.30–4.27 (m, 2H); 4.02–3.96 (m, 2H); 2.30– 2.20 (m, 1H); 1.52-1.48 (m, 1H). 13C (125 MHz, CDCl3): δ = 25.6, 31.4, 40.9, 67.2, 102.9, 117.4, 122.1, 123.2, 127.6, 128.6, 131.3, 132.1, 133.9, 154.9, 168.0.
Synthesis of Compound 6
0.440 g (1.298 mmol) of compound 5 and 0.704 mL (0.713 mmol) of hydrazine monohydrate in 30 mL of EtOH were added in a round-bottomed flask of 50 mL. The reaction was heated at 90°C and monitored by TLC. After 20 min, the heating was interrupted. The reaction mixture was cooled down to room temperature and solvent was evaporated. The obtained white precipitate was diluted in 30 mL of water. The aqueous solution was extracted with CHCl3 (2 × 20 mL) which was previously passed through Al2O3 layer. The CHCl3 solution was dried over anhydrous Na2SO4, filtered and evaporated. Compound 6 (0.154 g, 57%) was obtained as a yellow oil. 1H NMR (500 MHz, CDCl3): δ = 7.18–7.16 (dd, J = 8.5 Hz, J = 2.0 Hz, 1H); 7.14 (s, 1H); 6.87–6.85 (d, J = 8.5 Hz, 1H); 5.65 (s, 1H); 4.33-4.30 (m, 2H); 4.04–3.99 (m, 2H); 3.77 (s, 2H); 2.32–2.30 (m, 1H); 1.54–1.50 (m, 1H). 13C NMR (125 MHz, CDCl3): 26.6; 45.7; 67.4; 102.4; 117.1; 122.2; 126.3; 129.2; 134.3; 154.0.
Synthesis of Compound 8
0.394 g (0.598 mmol) of compound 7 (Gaware et al., 2017) were dissolved in 20 mL of anhydrous DMF, in a round-bottomed flask. To this stirred solution 0.273 g (0.718 mmol) of HATU was added, and the resulting mixture was left to stir under N2 atmosphere at room temperature for 20 min. Then, solution of 0.130 g (0.623 mmol) of compound 6 in 5 mL of anhydrous DMF was poured into the reaction mixture, which was stirred under N2 atmosphere and room temperature, for other 40 min. Finally, 0.105 mL (0.623 mmol) of N'N-diisopropylethylamine was added to the reaction mixture. The reaction was carried out in N2 atmosphere for 70 h. After this period, the reaction mixture was evaporated. The resulting precipitate was dissolved in 30 mL of CH2Cl2. The organic solution was washed with water (3 × 40 mL), dried over anhydrous Na2SO4 filtered and evaporated. Compound 8 was purified by silica gel column chromatography (CH2Cl2/MeOH 100:2) affording 80 mg (16% yield) of a purple solid. 1HNMR (500 MHz, CDCl3): δ = 8.85–8.61 (m, 8H); 8.29–8.26 (d, J = 8.0 Hz, 2H); 8.24–8.18 (m, 6H); 8.11–8.05 (d, J = 8.0 Hz, 2H); 7.90 (s, 1H); 7.78–7.72 (m, 9H); 7.37–7.33 (dd, J = 8.5 Hz, J = 2.0 Hz, 1H); 7.31 (d, J = 2.0 Hz, 1H); 6.96–6.93 (d, J = 8.5 Hz, 1H); 6.57 (s, 1H); 5.70 (s, 1H); 4.64 (d, 2H); 4.38–4.32 (m, 2H); 4.20–4.02 (m, 2H); 2.35–2.25 (m, 1H); 1.52-1.48 (m, 1H); −2.81 (s, 2H). 13C (125 MHz, CDCl3): δ = 25.7, 29.7, 43.9, 67.5, 102.9, 117.8, 118.5, 120.3, 125.3, 126.5 (x3), 126.7 (x3), 127.5 (x3) 127.7 (x3), 127.9, 129.2, 130.6, 131.7, 132.1, 134.4 (x2), 134.5, 134.6, 142.0, 142.7, 145.6, 150.3, 155.0.
Synthesis of Compound 9
0.080 g (0.094 mmmol) of compound 8 were dissolved in 1.74 mL of TFA, in a round-bottomed flask, and the resulting mixture was allowed to stir at room temperature for 3 h. Then, 10 mL of diethyl ether were added to the organic solution affording a green precipitate, that was filtered and crystallized using MeOH, to give 50 mg (67% yield) of a red solid. 1HNMR (500 MHz, CDCl3): δ = 11.04 (s, 1H); 9.96 (s, 1H); 8.96–8.86 (m, 8H); 8.33–8.29 (d, J = 8.0 Hz, 2H); 8.24–8.19 (m, 6H); 8.26–8.22 (d, J = 8.0 Hz, 2H); 7.80–7.72 (m, 9H); 7.70 (d, J = 2.0 Hz, 1H); 7.68–7.64 (dd, J = 8.5 Hz, J = 2.0 Hz, 1H); 7.05 (d, J = 8.5 Hz, 1H); 6.77 (t, 1H); 4.76 (d, 2H); −2.77 (s, 2H). 13C (125 MHz, CDCl3): δ = 43.0, 118.0, 118.1, 120.4, 121.2, 125.0, 125.2, 126.5, 126.7, 127.5, 127.8, 128.5, 129.0, 131.4, 132.1, 132.2, 132.6, 132.8, 132.9, 134.4, 134.5, 134.6, 136.6, 136.7, 142.0, 142.8, 149.5, 150.1, 150.3, 150.4, 167.2, 196.3. MS (ESI): m/z = 790.2 [M + H]+.
Synthesis of Salen-Bis-Porphyrin Ligand 10
In a round bottom flask, to a solution of 27.6 mg of salicylic-porphyrin 9 (0.0349 mmol) in absolute EtOH (10 mL) was added (1R,2R)-(+)-1,2-diphenylethylendiamine (3.7 mg, 0.0175 mmol). The reaction was stirred for 48 h at room temperature and monitored by TLC (CH2Cl2/CH3OH, 100:2). The reaction was quenched by evaporation of the solvent under reduced pressure, and salen-bis-porphyrin ligand 10 was purified by PLC (CH2Cl2/CH3OH, 100:2) to afford 24 mg of a red/purple solid compound (78% yield). 1HNMR (500 MHz, CDCl3): δ = 14.41 (bs, 2H); 8.86-8.74 (m, 16H); 8.30 (s, 2H); 8.25–8.07 (m, 20H); 7.77–7.66 (m, 20H); 7.29–7.15 (m, 12H); 6.97 (d, J = 8.5 Hz, 2H); 6.83 (t, 2H); 4.73 (s, 2H); 4.56 (d, 4H); −2.78 (s, 4H). 13C (125 MHz, CDCl3): δ = 43.3, 80.1, 117.2 (x2), 118.47, 118.53, 120.3 (x2), 120.5, 125.4 (x2), 126.3, 126.7 (x2), 127.7 (x3), 127.8 (x2), 128.4, 128.6, 131.0, 132.3, 133.5, 134.4 (x3), 134.5 (x2), 134.6, 139.0, 141.9, 142.0, 145.6, 160.4, 166.2, 167.4. MS (ESI): m/z = 1760.4 [M + H]+, m/z = 891.0 [M + Na]2+.
Synthesis of Uranyl Salen-Bis-Porphyrin Complex 1
To a solution of 10 (22 mg, 0.0125 mmol) dissolved in absolute ethanol (10 mL) was added uranyl acetate (5.3 mg, 0.0125 mmol). The reaction was stirred overnight at room temperature, and the resulting solid was filtered and dried to yield 27 mg of uranyl salen-bis-porphyrin complex 1 as a red powder (98% yield). 1HNMR (500 MHz, DMSO-d6): δ = 9.49 (s, 2H); 9.33 (s, 2H) 8.82–8.76 (m, 16H); 8.32–8.20 (m, 20H); 7.83–7.80 (m, 20H); 7.74–7.59 (m, 8H); 7.21 (t, 2H); 7.15 (m, 2H); 7.05 (d, 2H); 6.35 (s, 2H); 4.67–4.57 (m, 4H); −2.93 (s, 4H). 13C (125 MHz, DMSO-d6): δ = 42.2, 79.5, 119.0 (x2), 120.1, 120.2 (x2), 120.3, 122.7, 125.9 (x3), 126.6 (x2), 127.0 (x3), 127.2 (x2), 127.4 (x3), 127.5, 128.1, 128.2, 133.7, 133.9, 134.2 (x3), 135.2, 141.1, 141.6, 144.0, 166.0, 168.6, 171.2. MS (ESI): m/z = 1058 [M+2EtOH+2H]2+; m/z = 1077 [M+2EtOH+Na+H]2+.
Results and Discussion
Target uranyl salen-bis-porphyrin complex 1 was synthesized in seven steps starting from 5-Cl-methyl-salycilaldheyde 2 (Dalla Cort et al., 2006; Saffar-Telur, 2015) as shown in Scheme 1. In the first step potassium phtalimide was treated with compound 2 to yield compound 4 (40%), which was then reacted with 1,3-propandiol to afford the acetal intermediate 5 (92%). Conversion of the phthalimido moiety into an amino group by treatment with hydrazine, under standard Gabriel conditions, yielded the compound 6 (57%). The condensation reaction between compound 6 and 5-(4-Carboxyphenyl)-10,15,20-triphenylporphyrin 7 (Gaware et al., 2017) which was activated using HATU (Gangemi et al., 2015), afforded the porphyrin derivative 8 (16%), which was then treated with TFA to remove the acetal moiety and yield the salicylic-porphyrin 9 (67%). Condensation of 9 with the (1R,2R)-(+)-1,2-diphenylethylendiamine yielded salen ligand 10 (78%), which was finally converted into the corresponding salen complex 1 (98 %) by uranyl acetate. The proposed structures for this new chiral uranyl–salen complex and all the intermediates compounds are consistent with the 1H and 13C NMR spectroscopy data as well as the ESI mass spectrometry data (see Supplementary Material).
The UV-vis spectrum of uranyl salen-bis-porphyrin complex 1 dissolved in CHCl3 solution shows an intense Soret band centered at 419 nm (ε = 6,26·105 M−1 cm−1) and four Q-bands (515 nm; 550 nm; 590 nm; 646 nm) (Figure 2). The UV-vis spectrum is similar to the 5-(4-Methoxycarbonylphenyl)-10,15,20-triphenylporphyrin (TPPCOOMe, the precursor of 5-(4-Carboxyphenyl)-10,15,20-triphenylporphyrin 7) in the same solvent (Rong et al., 2012), suggesting that the insertion of the salen-UO2 does not change the spectroscopic behavior of the porphyrin. Furthermore, there is no evidence of aggregation even at higher concentration (2.5 μM), as confirmed by UV-Vis and fluorescence data. In addition, the luminescence measurements are in accordance with literature data for similar porphyrins, with two main emission bands centered at 650 and 715 nm (λex 350 nm), respectively (Figure 2).
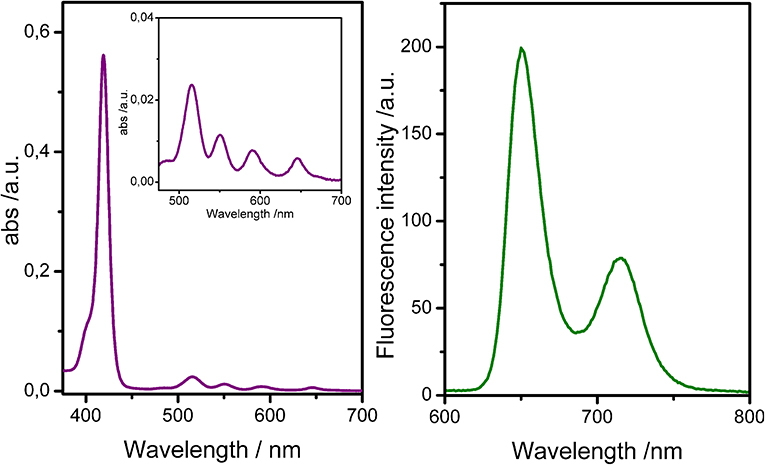
Figure 2. UV-Vis spectra (Left) and fluorescence spectra (Right) of uranyl salen-bis-porphyrin complex 1 in CHCl3 (1 μM).
After proving the luminescent properties of uranyl salen-bis-porphyrin complex 1, enantioselective recognition properties were evaluated by UV-vis and fluorescence measurements in chloroform, in particular for fluorescence titrations, following the emission changes at these two emission wavelengths of porphyrin moiety (650 nm and 715 nm, by using λex 350 nm). Unfortunately, the fluorescence titrations showed a small intensity variation with all the selected amino acid guests except for the L-tryptophan derivative, which led to a poor data fitting. For that reasons, binding constant values between uranyl salen-bis-porphyrin complex 1 and amino acid derivatives were determined by UV-vis titrations, following a decrease of the absorption at 419 nm upon addition of increasing aliquots of guests. A representative example of UV-vis titration and the fluorescence titration of uranyl salen-bis-porphyrin complex 1 with L-Trp-TBA are shown in Figure 3.
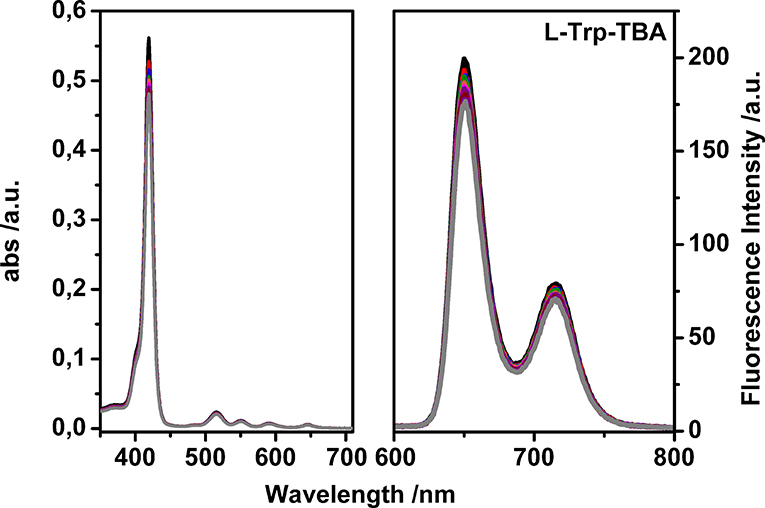
Figure 3. Representative UV-vis titration (Left) and fluorescence titration (Right) of uranyl salen-bis-porphyrin complex 1 with L-Trp-TBA (λex = 350 nm in dry chloroform).
Table 1 reports the pertinent binding constant values with selected amino acid derivatives, the detection limit observed (DL) and the corresponding enantiomeric excess. In all cases, binding constant values have been calculated using 1:1 stoichiometry, suggested by Job's plots (see Supplementary Material).
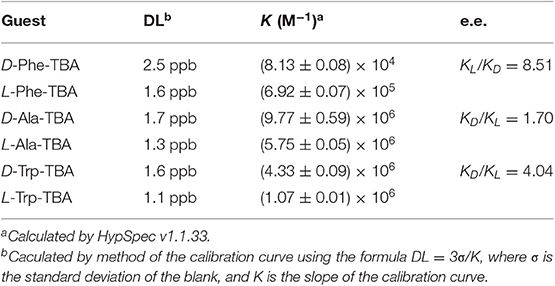
Table 1. Binding constant values K (M−1) with selected amino acid derivatives, detection limit observed (DL), and enantiomeric excess calculated by UV-vis titrations in dry chloroform at 25°C.
Notably, due to the presence of the porphyrin moieties, receptor 1 is able to detect the amino acid guests at low concentration. In fact, 1 μM solution of uranyl salen-bis-porphyrin complex 1 is able to detect amino acid derivatives at very low concentrations (ppb).
Enantiomeric recognition is very efficient with the L-Phe-TBA, which is recognized more than 8 times respect to the D-enantiomer (KL/KD = 8.51). A good enantioselectivity is also observed for the D- and L-Trp-TBA pair (KD/KL = 4.04). Moreover, for the L-Trp-TBA we were able to determine the binding constant value by fluorescence titration (K (M−1 = 2.63 ± 0.03 × 106), in good agreement with the value calculated by UV-vis titration. In particular, a decrease of the emission intensity has been observed, probably due to a photoinduced electron transfer mechanism (PET) (Trusso Sfrazzetto et al., 2016). Only the D- and L-Ala-TBA (KD/KL = 1.70) pair shows a slight selective recognition. With the smaller amino acid guest, coordination to the uranyl metal center appears less susceptible to the different configurations of the carbon atom stereocenter and then the molecular recognition is less selective. With aromatic amino acid derivatives, the possibility for the carboxylate anion to bind the fifth equatorial coordination site of the uranyl metal and, at the same time, to exploit CH-π interactions with the salen moiety and the porphyrin macrocycles of receptor 1 might be responsible for the strong observed enantioselectivity. Moreover, the strong recognition for the L-Phe-TBA enantiomer is in contrast with respect to those observed with our previous receptors having the same configuration of the chiral diamine bridge (R, R) (Ballistreri et al., 2010; Amato et al., 2011; Pappalardo et al., 2012a; Forte et al., 2015). Probably, the presence of the two porphyrin arms not only increase the limit of detection by the presence of a fluorescence signal, but also plays a fundamental role in the recognition event.
Conclusion
We have synthesized a new chiral uranyl salen complex bearing two porphyrin macrocycles and evaluated the enantiomeric recognition properties of this complex toward α-amino acid derivatives by UV-vis titrations. The presence of porphyrin arms lead to a receptor that possesses luminescence properties that are not quenched by the coordination with the uranyl cation, which decreases the fluorescence intensity in uranyl salen complexes. UV/Vis measurements and Job plots indicate the formation of 1:1 host–guest complexes. This receptor displays a very high selectivity toward amino acid derivatives, in particular for the two enantiomers of Phe-TBA. The two porphyrin macrocycles play a key role in the enantioselectivity interacting through CH–π with the aromatic moiety of aminoacids, leading to high binding affinities. Work is in progress in our laboratory to better understand the rules governing the interactions of this salen receptor with amino acid guests in order to design new host–guest systems that possess luminescence properties.
Data Availability Statement
All datasets generated for this study are included in the article/Supplementary Material.
Author Contributions
CG, UR, FC, and AP performed the synthesis of uranyl porphyrin-salen complex. GT acquired UV-vis and luminescence data. AP writing-original draft preparation. CG, UR, FC, GT, and AP writing-review and editing.
Funding
This work was supported by the University of Catania, Department of Chemical Sciences (Piano per la Ricerca 2016-2018–Linea Intervento 2).
Conflict of Interest
The authors declare that the research was conducted in the absence of any commercial or financial relationships that could be construed as a potential conflict of interest.
The reviewer SG declared a past co-authorship with one of the authors AP to the handling editor.
Supplementary Material
The Supplementary Material for this article can be found online at: https://www.frontiersin.org/articles/10.3389/fchem.2019.00836/full#supplementary-material
References
Amato, M. E., Ballistreri, F. P., D'Agata, S., Pappalardo, A., Tomaselli, G. A., Toscano, R. M., et al. (2011). Enantioselective molecular recognition of chiral organic ammonium ions and amino acids using cavitand–salen-based receptors. Eur. J. Org. Chem. 21, 5674–5680. doi: 10.1002/ejoc.201100955
Amato, M. E., Ballistreri, F. P., Gentile, S., Pappalardo, A., Tomaselli, G. A., and Toscano, R. M. (2010). Recognition of achiral and chiral ammonium salts by neutral ditopic receptors based on chiral salen-UO2 macrocycles. J. Org. Chem. 75, 1437–1443. doi: 10.1021/jo902328y
Amato, M. E., Ballistreri, F. P., Pappalardo, A., Sciotto, D., Tomaselli, G. A., and Toscano, R. M. (2007). Synthesis and conformational aspects of 20- and 40-membered macrocyclic mono and dinuclear uranyl complexes incorporating salen and (R)-BINOL units. Tetrahedron 63, 9751–9757. doi: 10.1016/j.tet.2007.07.014
Baleizao, C., and Garcia, H. (2006). Chiral salen complexes: an overview to recoverable and reusable homogeneous and heterogeneous catalysts. Chem. Rev. 106, 3987–4043. doi: 10.1021/cr050973n
Ballistreri, F. P., Gangemi, C. M., Pappalardo, A., Tomaselli, G. A., Toscano, R. M., and Trusso Sfrazzetto, G. (2016). (Salen)Mn(III) catalyzed asymmetric epoxidation reactions by hydrogen peroxide in water: a green protocol. Int. J. Mol. Sci. 17:1112. doi: 10.3390/ijms17071112
Ballistreri, F. P., Lombardo, G., Pappalardo, A., Punzo, F., Thompson, A., Tomaselli, G. A., et al. (2012). An integrated X-ray and molecular dynamics study of uranyl–salen structures and properties. Dalton Trans. 41, 1951–1960. doi: 10.1039/C1DT11758K
Ballistreri, F. P., Pappalardo, A., Toscano, R. M., Tomaselli, G. A., and Trusso Sfrazzetto, G. (2010). Heteroditopic chiral uranyl–salen receptor for molecular recognition of amino acid ammonium salts. Eur. J. Org. Chem. 20, 3806–3810. doi: 10.1002/ejoc.201000566
Ballistreri, F. P., Toscano, R. M., Amato, M. E., Pappalardo, A., Gangemi, C. M. A., Spidalieri, S., et al. (2018). A new Mn–salen micellar nanoreactor for enantioselective epoxidation of alkenes in water. Catalysts 8:129. doi: 10.3390/catal8040129
Beletskaya, I., Tyurin, V. S., Tsivadze, A. Y., Guilard, R., and Stern, C. (2009). Supramolecular chemistry of metalloporphyrins. Chem. Rev. 109, 1659–1713. doi: 10.1021/cr800247a
Brancatelli, G., Geremia, S., Notti, A., Pappalardo, A., and Trusso Sfrazzetto, G. (2013). Mono- and dinuclear uranyl(VI) complexes with chiral Schiff base ligand. Inorg. Chim. Acta 396, 25–29. doi: 10.1016/j.ica.2012.12.034
Cozzi, P. G. (2004). Metal–Salen Schiff base complexes in catalysis: practical aspects. Chem. Soc. Rev. 33, 410–421. doi: 10.1039/B307853C
Dalla Cort, A., Mandolini, L., Pasquini, C., and Schiaffino, L. (2006). A novel ditopic zinc-salophen macrocycle: a potential two-stationed wheel for [2]-pseudorotaxane. Org. Biomol. Chem. 4, 4543–4546. doi: 10.1039/b613705a
Drain, C. M., Varotto, A., and Radiovojevic, I. (2009). Self-organized porphyrinic materials. Chem. Rev. 109, 1630–1658. doi: 10.1021/cr8002483
D'Urso, A., Tudisco, C., Ballistreri, F. P., Condorelli, G. G., Randazzo, R., Tomaselli, G. A., et al. (2014). Enantioselective extraction mediated by a chiral cavitand–salen covalently assembled on a porous silicon surface. Chem. Commun. 50, 4993–4496. doi: 10.1039/C4CC00034J
Forte, G., D'Urso, A., Ballistreri, F. P., Toscano, R. M., Tomaselli, G. A., Trusso Sfrazzetto, G., et al. (2015). Enantiomeric recognition of α-amino acid derivatives by chiral uranyl-salen receptors. Tetrahedron Lett. 56, 2922–2926. doi: 10.1016/j.tetlet.2015.04.092
Gangemi, C. M. A., Randazzo, R., Fragal,à, M. E., Tomaselli, G. A., Ballistreri, F. P., Pappalardo, A., et al. (2015). Hierarchically controlled protonation/aggregation of a porphyrin-spermine derivative. New J. Chem. 39, 6722–6725. doi: 10.1039/C5NJ01264C
Gaware, V. S., Hakerud, M., Juzeniene, A., Høgset, A., Berg, K., and Másson, M. (2017). Endosome targeting meso-tetraphenylchlorin–chitosan nanoconjugates for photochemical internalization. Biomacromolecules 18, 1108–1126. doi: 10.1021/acs.biomac.6b01670
Jacobsen, E. N. (1993). “Asymmetric catalytic epoxidation of unfunctionalized olefins,” in Catalytic Asymmetric Synthesis, ed I. Ojima (New York, NY: VCH, 159–202.
Jacobsen, E. N. (2000). Asymmetric catalysis of epoxide ring-opening reactions. Acc. Chem. Res. 33, 421–431. doi: 10.1021/ar960061v
Johansson, A., Abrahamsson, M., Magnuson, A., Huang, P., Mårtensson, J., Styring, S., et al. (2003). Synthesis and photophysics of one mononuclear Mn(III) and one dinuclear Mn(III,III) complex covalently linked to a ruthenium(II) tris(bipyridyl) complex. Inorg. Chem. 42, 7502–7511. doi: 10.1021/ic0344822
Katsuki, T. (1995). Catalytic asymmetric oxidations using optically active (salen)manganese(III) complexes as catalysts. Coord. Chem. Rev. 140, 189–214. doi: 10.1016/0010-8545(94)01124-T
Katsuki, T. (2000). “Asymmetric oxidations and related reactions: asymmetric epoxidation of unfunctionalized olefins and related reactions,” in Catalytic Asymmetric Synthesis, 2nd Edn, ed I. Ojima (New York, NY: Wiley-VCH, 287–325.
La Paglia Fragola, V., Lupo, F., Pappalardo, A., Trusso Sfrazzetto, G., Toscano, R. M., Ballistreri, F. P., et al. (2012). A surface-confined O = MnV(salen) oxene catalyst and high turnover values in asymmetric epoxidation of unfunctionalized olefins. J. Mater. Chem. 22, 20561–20561. doi: 10.1039/c2jm34847k
McGarrigle, E. M., and Gilheany, D. G. (2005). Chromium- and manganese-salen promoted epoxidation of alkenes. Chem. Rev. 105, 1563–1602. doi: 10.1021/cr0306945
Pappalardo, A., Amato, M. E., Ballistreri, F. P., Tomaselli, G. A., Toscano, R. M., and Trusso Sfrazzetto, G. (2012a). Pair of diastereomeric uranyl salen cavitands displaying opposite enantiodiscrimination of α-amino acid ammonium salts. J. Org. Chem. 77, 7684–7687. doi: 10.1021/jo301098d
Pappalardo, A., Ballistreri, F. P., Li Destri, G., Mineo, P. G., Tomaselli, G. A., Toscano, R. M., et al. (2012b). Supramolecular polymer networks based on calix[5]arene tethered poly(p-phenyleneethynylene). Macromolecules 45, 7549–7556. doi: 10.1021/ma3015239
Puglisi, R., Ballistreri, F. P., Gangemi, C. M. A., Toscano, R. M., Tomaselli, G. A., Pappalardo, A., et al. (2017). Chiral Zn–salen complexes: a new class of fluorescent receptors for enantiodiscrimination of chiral amines. New J. Chem. 41, 911–915. doi: 10.1039/C6NJ03592B
Puglisi, R., Mineo, P. G., Pappalardo, A., Gulino, A., and Trusso Sfrazzetto, G. (2019). Supramolecular detection of a nerve agent simulant by fluorescent Zn–salen oligomer receptors. Molecules 24:2160. doi: 10.3390/molecules24112160
Puglisi, R., Pappalardo, A., Gulino, A., and Trusso Sfrazzetto, G. (2018). Supramolecular recognition of CWAs simulant by metal-salen complexes: the first multi-topic approach. Chem. Commun. 54, 11156–11159. doi: 10.1039/C8CC06425C
Rong, Y., Chen, P., Wang, D., and Liu, M. (2012). Porphyrin assemblies through the air/water interface: effect of hydrogen bond, thermal annealing, and amplification of supramolecular chirality. Langmuir 28, 6356–6363. doi: 10.1021/la3005294
Saffar-Telur, A. (2015). Direct covalent attachment of Mn(III) salophen complex to the hydroxyapatite-encapsulated γ- Fe2O3 nanocrystallites: an efficient magnetic and reusable catalyst for oxidation of alcohols. RSC Adv. 5, 70577–70585. doi: 10.1039/C5RA08594B
Trusso Sfrazzetto, G., Millesi, S., Pappalardo, A., Toscano, R. M., Ballistreri, F. P., Tomaselli, G. A., et al. (2015). Olefin epoxidation by a (salen)Mn(III) catalyst covalently grafted on glass beads. Cat. Sci. Techn. 5, 673–679. doi: 10.1039/C4CY00831F
Trusso Sfrazzetto, G.;, Satriano, C.;, Tomaselli, G. A.;, and Rizzarelli, E. (2016). Synthetic fluorescent probes to map metallostasis and intracellular fate of zinc and copper. Coord. Chem. Rev. 311, 125–167. doi: 10.1016/j.ccr.2015.11.012
Wezenberg, S. J., and Kleij, A. W. (2008). Material applications for salen frameworks. Angew. Chem., Int. Ed. 47, 2354–2364. doi: 10.1002/anie.200702468
Whiteoak, C. J., Salassa, G., and Kleij, A. W. (2012). Recent advances with π-conjugated salen systems. Chem. Soc. Rev. 41, 622–631. doi: 10.1039/C1CS15170C
Yoon, T. P., and Jacobsen, E. N. (2003). Privileged chiral catalysts. Science 299, 1691–1693. doi: 10.1126/science.1083622
Keywords: porphyrins, salen ligands, uranyl complexes, luminescence, enantiomeric recognition
Citation: Gangemi CMA, Rimkaite U, Cipria F, Trusso Sfrazzetto G and Pappalardo A (2019) Enantiomeric Recognition of α-Aminoacids by a Uranyl Salen-Bis-Porphyrin Complex. Front. Chem. 7:836. doi: 10.3389/fchem.2019.00836
Received: 27 September 2019; Accepted: 18 November 2019;
Published: 03 December 2019.
Edited by:
Carmine Gaeta, University of Salerno, ItalyReviewed by:
Paula M. Marcos, University of Lisbon, PortugalSilvano Geremia, University of Trieste, Italy
Copyright © 2019 Gangemi, Rimkaite, Cipria, Trusso Sfrazzetto and Pappalardo. This is an open-access article distributed under the terms of the Creative Commons Attribution License (CC BY). The use, distribution or reproduction in other forums is permitted, provided the original author(s) and the copyright owner(s) are credited and that the original publication in this journal is cited, in accordance with accepted academic practice. No use, distribution or reproduction is permitted which does not comply with these terms.
*Correspondence: Andrea Pappalardo, YW5kcmVhLnBhcHBhbGFyZG9AdW5pY3QuaXQ=