- 1Centro de Química Estrutural, Instituto Superior Técnico, Universidade de Lisboa, Lisbon, Portugal
- 2Área Departamental de Engenharia Química, Instituto Superior de Engenharia de Lisboa, Instituto Politécnico de Lisboa, Lisbon, Portugal
- 3National Laboratory for Molecular Sciences, Center for Molecular Science, Institute of Chemistry, Chinese Academy of Sciences, Beijing, China
The 1D Cu(II) coordination polymers [Cu3(L1)(NO3)4(H2O)2]n (1) and [Cu2(H2L2)(NO3)(H2O)2]n(NO3)n (2) have been synthesized using the aroylhyrazone Schiff bases N'1,N'2-bis(pyridin-2-ylmethylene)oxalohydrazide (H2L1) and N'1,N'3-bis(2-hydroxybenzylidene)malonohydrazide (H4L2), respectively. They have been characterized by elemental analysis, infrared (IR) spectroscopy, UV-Vis spectroscopy, electrospray ionization mass spectrometry (ESI-MS), single crystal X-ray diffraction and variable temperature magnetic susceptibility measurements (for 2). The ligand (L1)2− coordinates in the iminol form in 1, whereas the amide coordination is observed for (H2L2)2− in 2. Either the ligand bridge or the nitrate bridge in 2 mediates weak antiferromagnetic coupling. The catalytic performance of 1 and 2 has been investigated toward the solvent-free microwave-assisted oxidation of a secondary alcohol (1-phenylethanol used as model substrate). At 120°C and in the presence of the nitroxyl radical 2,2,6,6-tetramethylpiperydil-1-oxyl (TEMPO), the complete conversion of 1-phenylethanol into acetophenone occurs with TOFs up to 1,200 h−1.
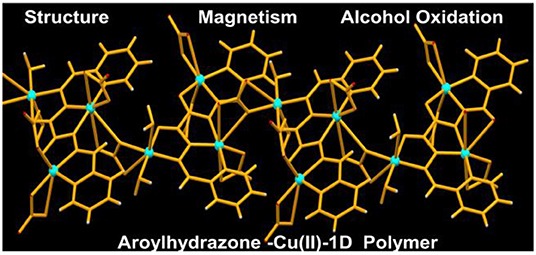
Graphical Abstract. Two new aroylhydrazone Cu(II) 1D coordination polymers [Cu3(L1)(NO3)4(H2O)2]n (1) and [Cu2(H2L2)(NO3)(H2O)2]n·(NO3)n (2) (H2L1 = N'1,N'2-bis(pyridin-2-ylmethylene)oxalohydrazide and H4L2 = N'1,N'3-bis(2-hydroxybenzylidene)malonohydrazide) have been structurally characterized. Their magnetic properties and the catalytic activity toward the solvent-free microwave-assisted oxidation of 1-phenylethanol have been explored.
Introduction
Transition metal complexes derived from multidentate Schiff base ligands received high significance due to their wide dimension of applications in the areas of molecular magnetism (Benelli and Gatteschi, 2002; Sutradhar et al., 2012, 2013, 2014b, 2015a, 2018; Cho et al., 2016; Andruh, 2018), crystal engineering (Dong et al., 2000; Kitaura et al., 2004; Andruh et al., 2009), supramolecular chemistry (Pradeep and Das, 2013; El-Bindary et al., 2016; Dwivedi et al., 2018), catalysis (Sutradhar et al., 2013, 2014b, 2015a,b,c, 2016a,b,c, 2017, 2018, 2019), etc. Molecular magnetism is one of the significant domains determining magneto-structural correlations to design magnetic materials (Benelli and Gatteschi, 2002; Sutradhar et al., 2012, 2013, 2014b, 2015a, 2018; Cho et al., 2016; Andruh, 2018). Single molecule magnets (SMMs) (Karotsis et al., 2010; Glaser et al., 2015; Maniaki et al., 2018) exhibit a large spin ground-state (S) value and large negative magnetic anisotropy and are used in potential high-density information storage devices and quantum computers (Leuenberger and Loss, 2001). Therefore, many efforts have been devoted to rationally establish polynuclear metal complexes and/or clusters elucidating molecular magnetism (Benelli and Gatteschi, 2002; Karotsis et al., 2010; Sutradhar et al., 2012, 2013, 2014b, 2015a, 2018; Glaser et al., 2015; Cho et al., 2016; Andruh, 2018; Maniaki et al., 2018). Coordination polymers and molecular clusters are particular classes of compounds that can display novel magnetic properties including high ground-state spin values and single molecule magnetism behavior (Zheng et al., 2014; Journaux et al., 2018; Yue and Gao, 2019).
The coordination behavior of Cu(II) is versatile, flexible and magnetically attractive. Many Cu(II) complexes are widely explored for magnetic studies focused on the synthesis of polynuclear complexes and/or clusters and their possible application in molecular magnetism (Benelli and Gatteschi, 2002; Sutradhar et al., 2013, 2015a, 2018; Andruh, 2018). Several attempts have been carried out to synthesize polynuclear Cu(II) complexes by designing suitable flexidentate Schiff base ligands to fabricate magnetic properties (Lu et al., 2013; Sutradhar et al., 2013, 2015a, 2018). Moreover, some multinuclear copper complexes show good catalytic performances toward the mild peroxidative oxidation of alkanes and alcohols into more valuable organic products (Pombeiro, 2013, 2019; Sutradhar et al., 2013, 2015a, 2018; Kopylovich et al., 2015) thus contributing to one of the most challenging subjects of modern chemistry. However, such examples are still limited and the study requires further exploration.
Aroylhydrazone Schiff bases are versatile in terms of functionalities, coordination properties and chelating abilities (Sutradhar et al., 2013, 2015a,b,c, 2016a,b,c, 2017, 2018). In continuation of our work in the fields of catalysis and magnetic studies, herein we report the syntheses of two 1D coordination polymers using two different multidentate N,O donor aroylhydrazone Schiff bases. The magnetic properties of one of them (complex 2) are studied using variable temperature magnetic susceptibility measurements. These 1D polymers are also tested as catalysts toward the microwave assisted peroxidative oxidation of alcohols under mild conditions for the future development of an environment benign catalytic system.
Experimental
All synthetic work was performed in air. Commercially available reagents and solvents were used as received, without further purification or drying. Cu(NO3)2·2.5H2O was used as metal source for the synthesis of the complexes.
C, H, and N elemental analyses were carried out by the Microanalytical Service of Instituto Superior Técnico. Infrared spectra (4,000–400 cm−1) were recorded on a Bruker Vertex 70 (Bruker Corporation, Ettlingen, Germany) instrument in KBr pellets; wavenumbers are in cm−1. The 1H NMR spectra were recorded at room temperature on a Bruker Avance II + 400.13 MHz (UltraShieldTM Magnet, Rheinstetten, Germany) spectrometer. The UV–Vis absorption spectra of methanol solutions of 1 and 2 (ca. 2 × 10−5M) in 1.00 cm quartz cells were recorded at room temperature on a Lambda 35 UV–Vis spectrophotometer (Perkin–Elmer) by scanning the 200–1000 nm region at a rate of 240 nm min−1. Tetramethylsilane was used as the internal reference and the chemical shifts are reported in ppm. Mass spectra were run in a Varian 500-MS LC Ion Trap Mass Spectrometer (Agilent Technologies, Amstelveen, The Netherlands) equipped with an electrospray (ESI) ion source. For electrospray ionization, the drying gas and flow rate were optimized according to the particular sample with 35 p.s.i. nebulizer pressure. Scanning was performed from m/z 100 to 1,200 in methanol solution. The compounds were observed in the positive mode (capillary voltage = 80–105 V). The catalytic tests were performed under microwave (MW) irradiation using a focused Anton Paar Monowave 300 microwave (Anton Paar GmbH, Graz, Austria) fitted with a rotational system and an IR temperature detector, using a 10 mL capacity reaction tube with a 13 mm internal diameter. Gas chromatographic (GC) measurements were carried in a FISONS Instrument GC 8000 series gas chromatograph with a capillary DB-WAX column (30 m x 0.32 mm), a FID detector, helium as the carrier gas and using the Jasco-Borwin v.1.50 software. The magnetic susceptibility measurements were carried out on a polycrystalline samples with a Quantum Design MPMS-XL5 SQUID magnetometer in the temperature range of 2–300 K and at an applied field of 2000 Oe. Diamagnetic corrections were estimated from Pascal's constants for all constituent atoms (Kahn, 1993).
Synthesis of the N'1,N'2-bis(pyridin-2-ylmethylene)oxalohydrazide (H2L1)
The aroylhydrazone Schiff base pro-ligand H2L1 (Scheme 1) was prepared by using a similar method reported for the synthesis of N'1,N'3-bis(2-hydroxybenzylidene)malonohydrazide (H4L2) (Sutradhar et al., 2014a) upon condensation of the oxalyl dihydrazide with pyridine-2-aldehyde.
Yield: 86%. Anal. Calcd for H2L1 C14H12N6O2: C, 56.75; H, 4.08; N, 28.36. Found: C, 56.68; H, 4.04; N, 28.29. IR (KBr pellet, cm−1): 3131ν(NH), 1681 ν(C=O) 1185 ν(N–N). 1H NMR (DMSO-d6, δ): 12.67 (s, 2H, NH), 7.47 (s, 2H, -CH=N), 8.67–7.89 (m, 8H, C5H4N). UV–Vis max (MeOH, nm (ε, LM−1 cm−1)): 656 (305), 378 (16,478), 285 (23,434), 237 (32,108). ESI-MS(+): m/z 297 [M+H]+ (100%).
Synthesis of [Cu3(L1)(NO3)4(H2O)2]n (1)
0.930 g Cu(NO3)2·2.5H2O (4.0 mmol) was added to a 25 mL methanolic suspension of H2L1 (0.302 g, 1.02 mmol). The resultant mixture was stirred at room temperature for 20 min and a dark green solution was obtained. The solution was then filtered and the solvent was allowed to evaporate slowly at room temperature. After 2 d, single crystals suitable for X-ray diffraction were isolated, washed 3 times with cold methanol and dried over silica gel.
Yield: 0.523 g (68%, with respect to Cu). Anal. Calcd for C14H14Cu3N10O16: C, 21.87; H, 1.84; N, 18.22. Found: C, 21.83; H, 1.81; N, 18.17. IR (KBr; cm−1): 3448 ν(OH), 1610 ν(C=N), 1382 ν(NO3), 1252 ν(C–O)enolic and 1154 ν(N–N). UV–Vis λmax (MeOH, nm (ε, LM−1 cm−1)): 752 (309), 820 (286), 390 (16,248), 282 (22,632), 254 (31,818). ESI-MS(+): m/z 770 [M+H]+ (100%).
Synthesis of [Cu2(H2L2)(NO3)(H2O)2]n(NO3)n (2)
To a 25 mL methanol solution of H4L2 (0.340 g, 1.00 mmol), a 20 mL methanol solution of Cu(NO3)2·2.5H2O (0.670 g, 3.0 mmol) was added and the reaction mixture was stirred for 20 min at room temperature. The resultant dark green solution was filtered and the filtrate was kept in open air. Dark green single crystals suitable for X-ray diffraction analysis were isolated after 2 days. Crystals were washed 3 times with cold ethanol and dried over silica gel.
Yield: 0.450 g (72%, with respect to Cu). Anal. Calcd for C17H18Cu2N6O12 (2): C, 32.65; H, 2.90; N, 13.44. Found: C, 32.60; H, 2.86; N, 13.39. IR (KBr; cm−1): 3432 ν(OH), 2874 ν(NH), 1607 ν(C=O), 1384 ν(NO3), and 1154 ν(N–N). UV–Vis λmax (MeOH, nm (ε, LM−1 cm−1)): 756 (296), 359 (14,436), 344 (18,322), 326 (16,862). ESI-MS(+): m/z 581 [(M-NO3)+H2O]+ (100%).
X-Ray Measurements
Crystals of 1 were unstable at room temperature and without solvent. A good quality single crystal of 1 in the mother liquor was mounted in a capillary tube, sealed and measured at the temperature of ca. 150 K. A crystal of 2 was immersed in cryo-oil, mounted in a Nylon loop and measured at the temperature of 296 K. Intensity data were collected using a Bruker AXS PHOTON 100 diffractometer with graphite monochromated Mo-Kα (λ 0.71073) radiation. Data were collected using omega scans of 0.5° per frame and full sphere of data were obtained. Cell parameters were retrieved using Bruker SMART (Bruker, 2012) software and refined using Bruker SAINT (Bruker, 2012) on all the observed reflections. Absorption corrections were applied using SADABS (Bruker, 2012). Structures were solved by direct methods by using SHELXS (Sheldrick, 2000) and refined with SHELXL 2018 (Sheldrick, 2015). Calculations were performed using WinGX version 2014.1 (Farrugia, 2012). All non-hydrogen atoms were refined anisotropically. The H-atoms bonded to carbons were included in the model at geometrically calculated positions and refined using a riding model. Uiso(H) were defined as 1.2Ueq of the parent aromatic and methylene groups and 1.5Ueq of the parent methyl ones. The hydrogen atoms attached to O were located in the difference Fourier map and refined with their isotropic thermal parameter set at 1.5 times the average thermal parameter of the parent oxygen atom. There was disordered solvent in the structure of 2 that could not be modeled (232 electrons in a void of 471 Å3). Platon Squeeze (Spek, 2009) was used to remove that electron density. Least square refinements with anisotropic thermal motion parameters for all the non-hydrogen atoms and isotropic for the remaining atoms were employed. Crystal structures data are provided in the Supplementary Material.
Typical Procedures and Product Analysis for Catalysis
The catalytic microwave-assisted (MW) peroxidative oxidation of 1-phenylethanol was undertaken in a focused Anton Paar Monowave 300 reactor equipped with a rotational system and an IR temperature detector. To a cylindrical pyrex tube (10 mL), 2.5 mmol alcohol, 5 μmol catalyst 1 or 2 (0.2 mol% vs. substrate) and 70% aqueous solution of tert-BuOOH (5 mmol) were added. The tube was sealed and placed in the microwave reactor under stirring and irradiation (5 or 20 W) at 80 or 120°C respectively, for 0.5 h. In the end, the reaction mixture was cooled to room temperature, 150 μL of benzaldehyde (internal standard) and 2.5 mL of MeCN (for substrate and organic products extraction) were added. The reaction mixture was stirred for 10 min and in the end a sample (1 μL) was taken from the organic phase and analyzed by GC using a FISONS gas chromatograph (GC 8000) with a FID detector and a capillary column (DB-WAX, column length: 30 m; internal diameter: 0.32 mm) (He as the carrier gas) and the Jasco-Borwin v.1.50 software. The samples were injected at 240°C. Initially the temperature was held at 120°C for 1 min, then elevated 10°C/min up to 200°C and maintained at this temperature for 1 min. Assignment of product peaks was done by comparison with chromatograms of pure commercial samples.
Results and Discussion
Syntheses and Characterization
The aroylhydrazone Schiff base N'1,N'2-bis(pyridin-2-ylmethylene)oxalohydrazide (H2L1) and N'1,N'3-bis(2-hydroxybenzylidene)malonohydrazide (H4L2) have been used to synthesize Cu(II) coordination polymers. Reactions of Cu(NO3)2·2.5H2O with those Schiff bases result in the formation of the 1D coordination polymers [Cu3(L1)(NO3)4(H2O)2]n (1) and [Cu2(H2L2)(NO3)(H2O)2]n(NO3)n (2). In 1 and 2 the coordination forms of the ligands are different (Scheme 1). Generally, in the presence of a base, aroylhydrazone coordinates to the metal center via the iminol (enol) form. The amide (keto) form is typically observed when reacting with a metal salt of the weak conjugated base of strong acid, e.g., nitrate, chloride or sulfate (Sutradhar et al., 2015a, 2016a). The treatment of H2L1 with Cu(NO3)2·2.5H2O yields the 1D polymer 1 with the iminol (enol) coordination form of the ligand without addition of a base. However, this is probably due to the presence of the basic pyridine moiety in H2L1 which helps to deprotonate the enolic hydrogen of another molecule during complex formation. In accord, the ligand exhibits the usual amide (keto) coordination in polymer 2, where no such basic moiety is present in the ligand.
All the characteristic bands of the corresponding coordinated tridentate anionic ligand are found in the IR spectra of 1 and 2, viz., 3,448, 1,610, 1,252, and 1,154 ν(N–N) cm−1 for 1 and 3,432, 2,874, 1,607, 1,384, and 1,154 cm−1 for 2. In addition, the presence of nitrate was found at ca. 1,382 cm−1. In the UV-Vis spectra, both complexes 1 and 2 exhibit intense ligand to metal charge transfer transitions (LMCT) in the range of 254–390 nm (Figures S1,S2). In 1, less intense absorption bands at 752 and 820 nm are due to d–d transitions attributable to 2B1g → 2A1g and 2B1g → 2E1g transitions, suggesting a distorted octahedral geometry (Figure S1) (Sutradhar et al., 2016b; Sureshbabu et al., 2019). In the case of 2 a less intense absorption band is observed at 756 nm (Figure S2) in accord with square pyramidal coordination geometries at the Cu(II) centers (Sutradhar et al., 2016b; Sureshbabu et al., 2019), which is in agreement with the structures obtained by single crystal X-ray analysis. The ESI-MS spectrum of compound 1, in methanol solution (see experimental section), displays the molecular ion peak at m/z = 770 [1+H]+ (100%). For 2, the peak at m/z 581 (100%) suggests the absence of the non-coordinated nitrate ion and the addition of one water molecule. The magnetic properties and catalytic activity toward solvent-free microwave-assisted oxidation of 1-phenylethanol of 1 and 2 are discussed in the following sections.
Description of the Crystal Structures
Single crystals suitable for X-ray analysis were isolated upon slow evaporation of a methanolic solution of 1 or 2 at room temperature. Crystals of 1 are unstable at room temperature and loose crystallinity in the absence of the mother liquor. A special precaution was taken (mentioned in experimental section) to analyze the structure. Crystallographic data for 1 and 2 are summarized in Table S1 and selected dimensions (bond angle and lengths) are presented in Table S2. Figures 1, 2 represent the structures of the respective complexes.
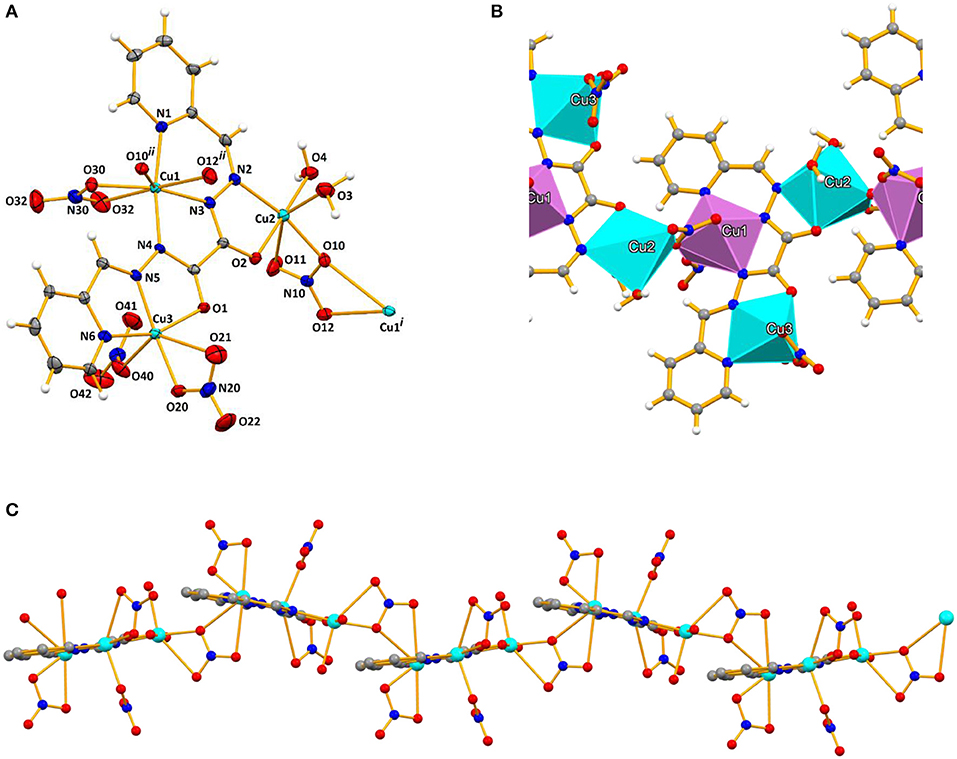
Figure 1. (A) Ellipsoid plot of 1 with partial atom numbering scheme, (B) a fragment of its 1D polymeric chain with the metals in polyhedral representations (coordination number six represented in blue, and seven in violet), and (C) a view of the smooth wave-like nature of the chain. Symmetry operation to generate equivalent atoms: (i) 1.5–x, –y,1/2+z; (ii) 1.5–x, –y, −1/2+z.
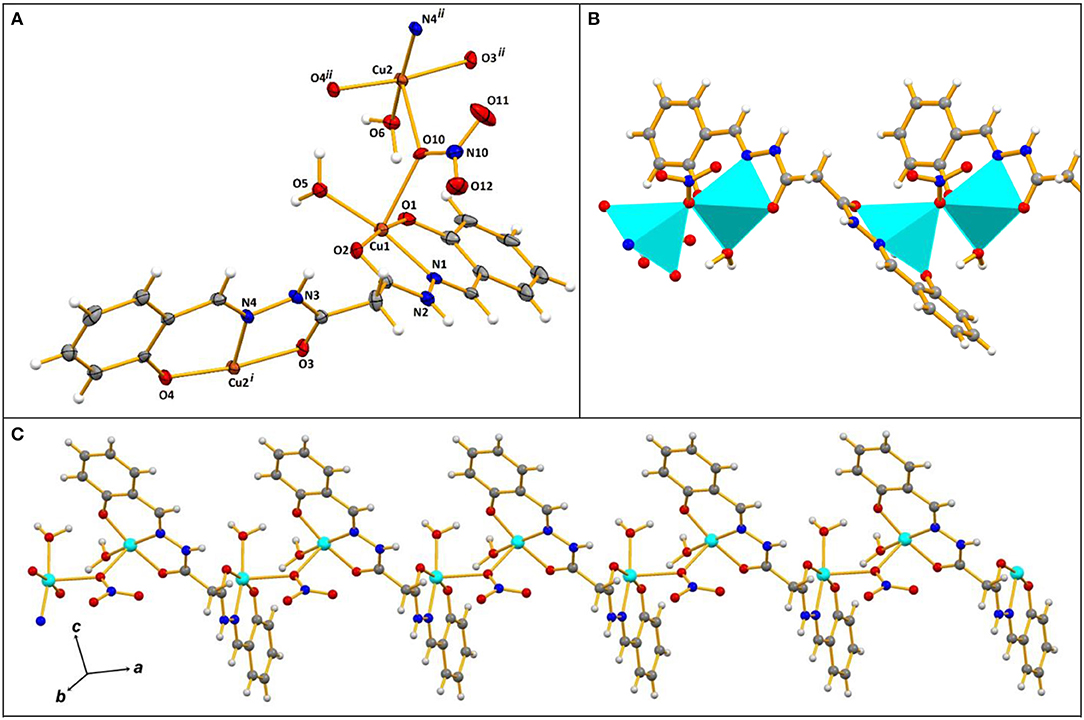
Figure 2. (A) Ellipsoid plot of 2 with partial atom numbering scheme; (B) a fragment of 1D polymeric chain with the metals in polyhedral representation; (C) a view of the 1D chain. The nitrate counter-ions are omitted for clarity. Symmetry operations to generate equivalent atoms: (i) 1+x, y, z; (ii) −1+x, y, z.
The multidentate ligand (L1)2− in 1 is planar and chelates to three Cu(II) ions through the NNN, the NNO and the NO pockets affording one 6-membered and four 5-membered metallacycles (Figure 1A). The copper cations are slightly displaced (maximum of 0.197 Å) toward the same side of the least-square plane of the organic ligand. Despite the planarity of (L1)2−, its derived 1D coordination polymer has a smoth wave-like shape imposed by the coordination mode of one of the nitrate anions behaving as bridging 1κOO':2κOO” donors to Cu1 and Cu2; the least-square planes of adjacent (L1)2− ligands make angles of 19.84°. The remaining nitrate ligands work as bidentate (to Cu1 and to Cu3) and as monodentate (to Cu3) ligands. The two water molecules are coordinated to the same copper cation (Cu2). The metal ions assume coordination numbers six (Cu2 and Cu3) and seven (Cu1), with the coordination polyhedron of Cu3 (Figure 1B) having no contact atom with the other polyhedra; those of Cu1 and Cu2 share an Onitrate atom. The intrachain Cu…Cu distances through the azine bridges are of 4.736 and 4.794 Å, and of 4.451 Å through the nitrate bridge. The minimum interchain Cu…Cu distance is of 5.692 Å.
The asymmetric unit of 2 contains two Cu(II) ions, one (H2L2)2−, one nitrate and two water ligands, and a nitrate counter-ion. The organic ligand in 2 behaves as a hexadentate chelator acting as an NOO donor for each of the Cu(II) ions, therefore giving rise to two six- and two five-membered metallacycles (Figure 1A). Bridging monodentate nitrate anions connect the {Cu2(H2L2)}2− moieties and generate a 1D polymeric chain running along the crystallographic a axis (Figure 1C). The copper cations adopt square pyramidal geometries (τ5 = 0.04 for Cu1 and 0.05 for Cu2) and share the Onitrate atom that stands in the axial position (Figures 2A,C). In view of the binding mode of the nitrates, the shortest Cu…Cu distance of 4.028 Å is along such ligands, while that along the hydrazone group exclusively (6.469 Å) is much longer than in 1 (see above). Resulting from the presence of a central methylene group in (H2L2)2− the ligand is highly twisted at this level, with the least-square planes of the two phenyl(methylene)acetohydrazide moieties making angles of 84.85°.
Magnetic Properties
The dc magnetic susceptibility of 2 was measured under 2000 Oe field (Figure 3). The room temperature χT value of 0.775 cm3 K mol−1 is a little larger than 0.750 cm3 K mol−1 calculated for two isolated Cu2+ ions. The χT value for 2 decreases gently with decreasing temperature in the range of 300–25 K, and then decreases sharply. The magnetic data at 50–300 K follow the Curie-Weiss law, with C = 0.785 cm3.K.mol−1 and Θ = −3.20 K (Figure S3). The small negative Θ value suggests existence of weak antiferromagnetic interaction in 2.
Based on the crystal structure, complex 2 is an alternative copper(II) chain compound, so two J1 and J2 parameters are necessary to describe the magnetic interaction, which are mediated through the ligand bridge and the Onitrate bridge, respectively. The magnetic data could be fitted by MagPack software using a Cu14 cluster loop approximation (Figure 4). The Hamiltonian of the Cu14 cluster loop with alternative J1 and J2 magnetic coupling constants is as follows: H = −2J1(SCu1SCu2+SCu3SCu4+SCu5SCu6+SCu7SCu8+SCu9SCu10 + SCu11SCu12+SCu13SCu14)−2J2(SCu2SCu3+SCu4SCu5+SCu6SCu7 + SCu8SCu9+SCu10SCu11+SCu12SCu13+SCu14SCu1). The best fitting gave J1 = −1.84 cm−1, J2 = −4.35 cm−1, g = 2.10 and R = 1.6 × 10−4. Both J1 and J2 values are small, suggesting that the antiferromagnetic interaction in 2 is weak. The J2 value is more negative than that of J1, indicating that the antiferromagnetic interaction through the ligand bridge is weaker than that through the Onitrate bridge.
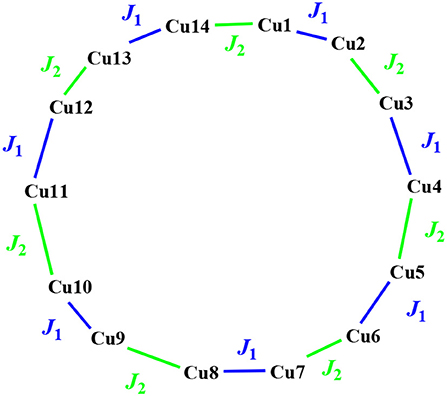
Figure 4. Magnetic topology of Cu14 cycle in 2 (the copper numbers do not show crystallographically independent copper ions, but rather are used to distinguish them).
Catalytic Studies Toward Microwave (MW) Assisted Oxidation of a Secondary Alcohol Under Solvent-Free Conditions
Cu(II) complexes 1 and 2 have been investigated as catalysts for the homogeneous oxidation of a secondary alcohol, 1-phenylethanol, to the corresponding ketone using tert-butylhydroperoxide (tert-BuOOH, TBHP, aq. 70%, 2 eq.) as oxidizing agent. The oxidation reactions were performed typically at 80 or 120°C, low power (5 or 20 W) microwave irradiation (MW), 0.5 h reaction time without additional solvent (Scheme 2). Results are summarized in Table 1.
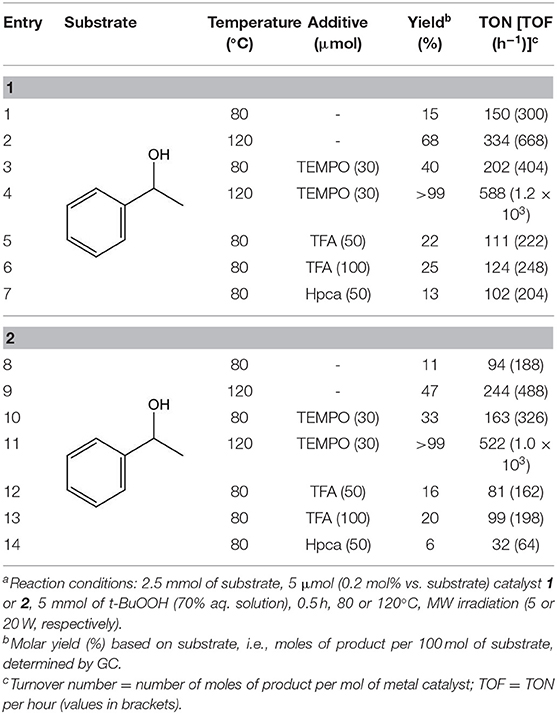
Table 1. Solvent-free MW-assisted oxidation of 1-phenyethanol using 1 and 2 as catalysts precursors (selected data)a.
Complexes 1 and 2 proved to be essential for the formation of acetophenone, since under similar reaction conditions and in the absence of these metal catalysts only traces of the desired product were detected. For catalytic tests performed only with the free ligand, no product was detected.
Under the studied conditions, the assays with copper catalysts 1 and 2 have shown comparable yields. For instance, for both 1 and 2 and using TBHP as oxidant at 120 °C, in the presence of TEMPO (2,2,6,6-tetramethylpiperydil-1-oxyl) radical, the almost complete conversion of 1-phenylethanol to the desired product is achieved after the short period of 30 min with a TON of 588 and 522, respectively (Table 1, entries 4 and 11, respectively).
For these encouraging results, the effect of temperature and the presence of TEMPO, a chemically stable nitroxyl radical which has emerged as a promoter for the metal catalyzed transformation of alcohols to the corresponding carbonyl products (Gamez et al., 2004; Sheldon and Arends, 2004; Figiel et al., 2007, 2009; Sheldon, 2008; Ahmad et al., 2009), seem to be determinant. Reactions carried out under the same reaction conditions but at 80°C and in the absence of any additive did not go beyond 15 and 11% in the presence of 1 and 2, respectively (Table 1, entries 1 and 8, respectively) whereas when carried out at 120°C it was possible to observe a significant increase in yield to 68 and 47% for 1 and 2, respectively (Table 1, entries 2 and 9, respectively). The combination of these two parameters, temperature at 120°C and presence of TEMPO radical (Figure 5), resulted in the complete conversion of 1-phenylethanol into acetophenone and TOFs up to 1200 h−1 (Table 1, entries 4 and 11, for 1 and 2, respectively).
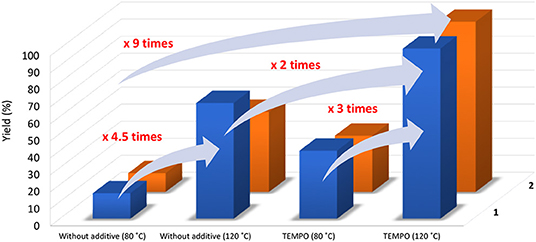
Figure 5. Influence of temperature and of presence of TEMPO additive on the yield of acetophenone from oxidation of 1-phenylethanol catalyzed by 1 and 2. Reaction conditions: 2.5 mmol of substrate, 5 μmol (0.2 mol% vs. substrate) catalyst 1 or 2, 5 mmol of t-BuOOH, 0.5 h, 80 or 120°C (5 or 20 W, respectively).
Considering the promoting effect of acid co-catalysts observed for other Cu-catalyzed oxidation systems (Sutradhar et al., 2016a,c, 2018), which are believed to accelerate the oxidation reactions by improving the oxidation properties of the complexes and by creating unsaturated metal centers (Sutradhar et al., 2015c), the influence of acidic additives on the acetophenone product yield was explored. The addition of trifluoroacetic acid (TFA) has a slight beneficial effect on both catalytic systems, resulting, for example, into a maximum yield of 25% (Table 1, entry 6), in the presence of 1 (at 80°C) (Figure 6). In contrary, the addition of the heteroaromatic 2-pyrazynecarboxylic acid (Hpca) has the opposite effect (Figure 6), i.e., a yield drop is observed when 50 μmol was used [n(acid)/n(catalyst 1 or 2) = 10] (Table 1, entries 7 and 14, for 1 and 2, respectively).
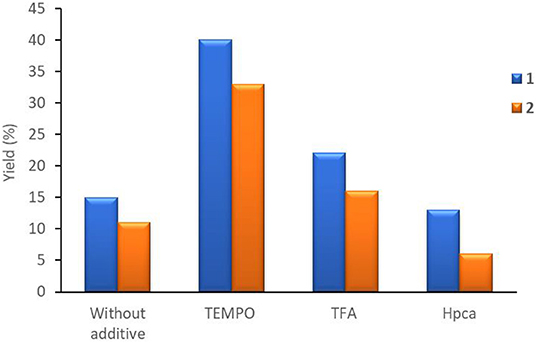
Figure 6. Influence of different additives on the yield of acetophenone from oxidation of 1-phenylethanol catalyzed by 1 and 2. Reaction conditions: 2.5 mmol of substrate, 5 μmol (0.2 mol% vs. substrate) catalyst 1 or 2, 5 mmol of t-BuOOH, 0.5 h, 80°C (5 W MW irradiation).
The promoting effect of TEMPO suggests the involvement of a radical mechanism which possibly involves the formation of t-BuOO and t-BuO radicals by a metal-assisted oxidation or reduction of t-BuOOH by a CuII or a CuI center, respectively (Gephart et al., 2012; Dronova et al., 2014), the latter behaving as an H-atom abstractor from the alcohol (Rothenberg et al., 1998; Mahdavi and Mardani, 2012; Frija et al., 2016; Sutradhar et al., 2016a,b, 2018; Ma et al., 2019).
Conclusions
In this study we have successfully synthesized two 1D Cu(II) coordination polymers using two different multidenate aroylhyrazone Schiff bases. The X-ray crystallographic study indicates that the two ligands show different coordination behaviors. The N'1,N'2-bis(pyridin-2-ylmethylene)oxalohydrazide (H2L1), having a basic pyridine moiety, coordinates in the iminol form, whereas the amide form is observed in the case of 2. Both the ligand bridge and the nitrate bridge in 2 mediate weak antiferromagnetic interaction. The effects of temperature and of the aditive TEMPO dramatically increase the catalytic efficiency of both Cu(II) compounds in the microwave-assisted oxidation of 1-phenylethanol to acetophenone under solvent-free conditions.
Our study concerns an attempt to design Cu(II) coordination polymers with interesting magnetic properties and also for use as catalyst toward the development of environmentally friendly alcohol oxidation catalytic systems.
Data Availability Statement
All datasets generated for this study are included in the article/Supplementary Material. The crystallographic datasets generated for this study can also be found in the Cambridge Crystallographic Data Center (https://www.ccdc.cam.ac.uk/structures/) under the identifiers 1945551 and 1945552.
Author Contributions
MS: overall planning, synthesis and characterization of catalysts, and manuscript writing. EA: catalytic studies and manuscript writing. TB: catalytic studies, GC analysis and manuscript writing. MG: single crystal X-ray diffraction analysis. C-ML: Magnetic studies and manuscript writing. AP: manuscript reading and correcting.
Funding
This work has been supported by the Fundação para a Ciência e Tecnologia (FCT) 2020-2023 multiannual funding to Centro de Quimica Estrutural. Authors are also grateful to the FCT (projects UID/QUI/00100/2020, PTDC/QUI-QIN/29778/2017, and PTDC/QEQ-QIN/3967/2014), Portugal, for financial support. MS acknowledges the FCT and IST for a working contract DL/57/2017 (Contract no. IST-ID/102/2018). C-ML acknowledges the funding from the National Natural Science Foundation of China (21871274).
Conflict of Interest
The authors declare that the research was conducted in the absence of any commercial or financial relationships that could be construed as a potential conflict of interest.
Acknowledgments
Authors are thankful to the Portuguese NMR Network (IST-UL Center) for access to the NMR facility and the IST Node of the Portuguese Network of mass-spectrometry for the ESI-MS measurements.
Supplementary Material
The Supplementary Material for this article can be found online at: https://www.frontiersin.org/articles/10.3389/fchem.2020.00157/full#supplementary-material
Data sheet 1. Crystal structures data.
References
Ahmad, J. U., Figiel, P. J., Räisänen, M. T., Leskelä, M., and Repo, T. (2009). Aerobic oxidation of benzylic alcohols with bis(3,5-di-tert-butylsalicylaldimine)copper(II) complexes. Appl. Catal. A 371, 17–21. doi: 10.1016/j.apcata.2009.09.011
Andruh, M. (2018). Heterotrimetallic complexes in molecular magnetism. Chem. Commun. 54, 3559–3577. doi: 10.1039/C8CC00939B
Andruh, M., Branzea, G. D., and Gheorghe, R., and Madalan, A. M. (2009). Crystal engineering of hybrid inorganic–organic systems based upon complexes with dissymmetric compartmental ligands. Cryst. Eng. Comm. 11, 2571–2584. doi: 10.1039/b909476h
Benelli, C., and Gatteschi, D. (2002). Magnetism of lanthanides in molecular materials with transition-metal ions and organic radicals. Chem. Rev. 102, 2369–2388. doi: 10.1021/cr010303r
Cho, Y. I., Ward, M. L., and Rose, M. J. (2016). Substituent effects of N4 schiff base ligands on the formation of fluoride-bridged dicobalt(II) complexes via B-F abstraction: structures and magnetism. Dalton. Trans. 45, 13466–13476. doi: 10.1039/C6DT02104B
Dong, Y.-B., Smith, M. D., and zur Loye, H.-C. (2000). New inorganic/organic coordination polymers generated from bidentate schiff-base ligands. Inorg. Chem. 39. 4927–4935. doi: 10.1021/ic0006504
Dronova, M. S., Bilyachenko, A. N., Yalymov, A. I., Kozlov, Y. N., Shul'pina, L. S., Korlyukov, A. A., et al. (2014). Solvent-controlled synthesis of tetranuclear cage-like copper(II) silsesquioxanes. Remarkable features of the cage structures and their high catalytic activity in oxidation with peroxides. Dalton Trans. 43, 872–882. doi: 10.1039/C3DT52508B
Dwivedi, N., Sunkari, S. S., Verma, A., and Saha, S. (2018). Molecular packing dependent solid state fluorescence response of supramolecular metal-organic frameworks: phenoxo-bridged trinuclear Zn(II) centered schiff base complexes with halides and pseudohalides. Cryst. Growth Des. 18, 5628–5637. doi: 10.1021/acs.cgd.8b00948
El-Bindary, A. A., El-Sonbati, A. Z., Diab, M. A., Ghoneim, M. M., and Serag, L. S. (2016). Polymeric complexes - LXII. Coordination chemistry of supramolecular schiff base polymer complexes—a review. J. Mol. Liq. 216, 318–329. doi: 10.1016/j.molliq.2015.12.113
Farrugia, L. J. (2012). WinGX and ORTEP for windows: an update. J. Appl. Cryst. 45, 849–854. doi: 10.1107/S0021889812029111
Figiel, P. J., Leskelä, M., and Repo, T. (2007). TEMPO-copper(II) diimine-catalysed oxidation of benzylic alcohols in aqueous media. Adv. Synth. Catal. 349, 1173–1179. doi: 10.1002/adsc.200600505
Figiel, P. J., Sibaouih, A., Ahmad, J. U., Nieger, M., Räisänen, M. T., Leskelä, M., et al. (2009). Aerobic oxidation of benzylic alcohols in water by 2,2,6,6-tetramethylpiperidine-1-oxyl (TEMPO)/copper(II) 2-N-arylpyrrolecarbaldimino complexes. Adv. Synth. Catal. 351, 2625–2632. doi: 10.1002/adsc.200900478
Frija, L. M. T., Alegria, E. C. B. A., Sutradhar, M., Cristiano, M. L. S., Ismael, A., Kopylovich, M. N., et al. (2016). Copper(II) and cobalt(II) tetrazole-saccharinate complexes as effective catalysts for oxidation of secondary alcohols. J. Mol. Cat. A Chem. 425, 283–290. doi: 10.1016/j.molcata.2016.10.023
Gamez, P., Arends, I. W. C. E., Sheldon, R. A., Reedijk, J. (2004). Room temperature aerobic copper–catalysed selective oxidation of primary alcohols to aldehydes. Adv. Synth. Catal. 346, 805–811. doi: 10.1002/adsc.200404063
Gephart, R. T., McMullin, C. L., Sapiezynski, N. G., Jang, E. S., Aguila, M. J. B., Cundari, T. R., et al. (2012). Reaction of CuI with dialkyl peroxides: CuII-alkoxides, alkoxy radicals, and catalytic C–H etherification. J. Am. Chem. Soc. 134, 17350–17353. doi: 10.1021/ja3053688
Glaser, T., Hoeke, V., Gieb, K., Schnack, J., Schroder, C., and Muller, P. (2015). Quantum tunneling of the magnetization in [(Mn6M)-M-III](3+) (M = Cr-III, Mn-III) SMMs: impact of molecular and crystal symmetry. Coord. Chem. Rev. 289, 261–278. doi: 10.1016/j.ccr.2014.12.001
Journaux, Y., Ferrando-Soria, J., Pardo, E., Ruiz-Garcia, R., Julve, M., Lloret, F., et al. (2018). Design of magnetic coordination polymers built from polyoxalamide ligands: a thirty year story. Eur. J. Inorg. Chem. 228–247. doi: 10.1002/ejic.201700984
Karotsis, G., Kenedy, S., Teat, S. J., Beavers, C. M., Fowler, D. A., Morales, J. J., et al. (2010). [] Calix[4]arene clusters as enhanced magnetic coolers and molecular magnets. J. Am. Chem. Soc. 132, 12983–12990. doi: 10.1021/ja104848m
Kitaura, R., Onoyama, G., Sakamoto, H., Matsuda, R., Noro, S-i., and Kitagawa, S. (2004). Immobilization of a metallo schiff base into a microporous coordination polymer. Angew. Chem. Int. Ed. 43. 2684–2687. doi: 10.1002/anie.200352596
Kopylovich, M. N., Ribeiro, A. P. C., Alegria, E. C. B., Martins, N. M. R., Martins, L. M. D. R. S., and Pombeiro, A. J. L. (2015). Catalytic oxidation of alcohols: recent advances. Adv. Organomet. Chem. 63, 91–174. doi: 10.1016/bs.adomc.2015.02.004
Leuenberger, M. N., and Loss, D. (2001). Quantum computing in molecular magnets. Nature 410, 789–793. doi: 10.1038/35071024
Lu, Z., Fan, T., Guo, W., Lu, Z., and Fan, C. (2013). Synthesis, structure and magnetism of three cubane Cu(II) and Ni(II) complexes based on flexible schiff-base ligands. Inorg. Chim. Acta. 400, 191–196. doi: 10.1016/j.ica.2013.02.030
Ma, Z., Wang, Q., Yang, H., Alegria, E. C. B. A., Guedes da Silva, M. F. C., Martins, L. M. D. R. S., et al. (2019). Synthesis and structure of copper complexes of a N6O4 macrocyclic ligand and catalytic application in alcohol oxidation. Catalysts 9:424. doi: 10.3390/catal9050424
Mahdavi, V., and Mardani, M. (2012). Selective oxidation of benzyl alcohol with tert-butylhydroperoxide catalysed via Mn (II) 2, 2-bipyridine complexes immobilized over the mesoporous hexagonal molecular sieves (HMS). J. Chem. Sci. 124, 1107–1115. doi: 10.1007/s12039-012-0307-4
Maniaki, D., Pilichos, E., Perlepes, S. P. (2018). Coordination clusters of 3d-metals that behave as single-molecule magnets (SMMs): synthetic routes and strategies. Front. Chem. 6:461. doi: 10.3389/fchem.2018.00461
Pombeiro, A. J. L. (2013). Advances in Organometallic Chemistry and Catalysis. Hoboken, NJ: Wiley, 15–25. doi: 10.1002/9781118742952.ch2
Pombeiro, A. J. L. (2019). “Alkane Functionalization: Introduction and Overview,” in Alkane Functionalization, eds A. J. L. Pombeiro and M. F. C. Guedes da Silva (Hoboken, NJ: Wiley), 1–15. doi: 10.1002/9781119379256.ch1
Pradeep, C. P., and Das, S. K. (2013). Coordination and supramolecular aspects of the metal complexes of chiral N-salicyl-beta-amino alcohol schiff base ligands: towards understanding the roles of weak interactions in their catalytic reactions. Coord. Chem. Rev. 257, 1699–1715. doi: 10.1016/j.ccr.2013.01.028
Rothenberg, G., Feldberg, L., Wiener, H., and Sasson, Y. J. (1998). Copper-catalyzed homolytic and heterolytic benzylic and allylic oxidation using tert-butyl hydroperoxide. Chem. Soc. Perkin Trans. 2, 2429–2434. doi: 10.1039/a805324c
Sheldon, R. A. (2008). E factors, green chemistry and catalysis: an odyssey. Chem. Commun. 29, 3352–3365. doi: 10.1039/b803584a
Sheldon, R. A., and Arends, I. W. C. E. (2004). Organocatalytic oxidations mediated by nitroxyl radicals. Adv. Synth. Catal. 346, 1051–1071. doi: 10.1002/adsc.200404110
Sheldrick, G. M. (2000). SADABS. Program for Empirical Absorption Correction. Göttingen: University of Göttingen.
Sheldrick, G. M. (2015). Crystal structure refinement with SHELXL. Acta Cryst. C71, 3–8. doi: 10.1107/S2053229614024218
Spek, A. L. (2009). Structure validation in chemical crystallography. Acta Cryst. D65, 148–155. doi: 10.1107/S090744490804362X
Sureshbabu, P., Junaid, Q. M., Upadhyay, C., Victoria, W., Pitchavel, V., Natarajan, S., and Sabiah, S. (2019). Di and tetranuclear Cu(II) complexes with simple 2-aminoethylpyridine: magnetic properties, phosphodiester hydrolysis, DNA binding/cleavage, cytotoxicity and catecholase activity. Polyhedron 164, 202–218. doi: 10.1016/j.poly.2019.02.015
Sutradhar, M., Alegria, E. C. B. A., Guedes da Silva, M. F. C., Liu, C.-M., Pombeiro, A. J. L. (2018). Peroxidative oxidation of alkanes and alcohols under mild conditions by di- and tetranuclear copper(II) complexes of bis(2-hydroxybenzylidene)isophthalohydrazide. Molecules 23:2699. doi: 10.3390/molecules23102699
Sutradhar, M., Alegria, E. C. B. A., Guedes da Silva, M. F. C., Martins, L. M. D. R. S., and Pombeiro, A. J. L. (2016b). Aroylhydrazone Cu(II) complexes in keto form: structural characterization and catalytic activity towards cyclohexane oxidation. Molecules 21:425. doi: 10.3390/molecules21040425
Sutradhar, M., Alegria, E. C. B. A., Mahmudov, K. T., Guedes da Silva, M. F. C., and Pombeiro, A. J. L. (2016a). Iron(III) and cobalt(III) complexes with both tautomeric (keto and enol) forms of aroylhydrazone ligands: catalysts for the microwave assisted oxidation of alcohols. RSC Adv. 6, 8079–8088. doi: 10.1039/C5RA25774C
Sutradhar, M., Alegria, E. C. B. A., Roy Barman, T., Scorcelletti, F., Guedes da Silva, M. F. C., and Pombeiro, A. J. L. (2017). Microwave-assisted peroxidative oxidation of toluene and 1-phenylethanol with monomeric keto and polymeric enol aroylhydrazone Cu(II) complexes. Mol. Catal. 439, 224–232. doi: 10.1016/j.mcat.2017.07.006
Sutradhar, M., Carrella, L. M., and Rentschler, E. (2012). A discrete μ4-oxido tetranuclear iron(III) cluster. Eur. J. Inorg. Chem. 2017, 4273–4278. doi: 10.1002/ejic.201200396
Sutradhar, M., Guedes da Silva, M. F. C., Pombeiro, A. J. L. (2014a). A new cyclic binuclear Ni(II) complex as a catalyst towards nitroaldol (Henry) reaction. Catal. Commun. 57, 103–106. doi: 10.1016/j.catcom.2014.08.013
Sutradhar, M., Kirillova, M. V., Guedes da Silva, M. F. C., Liu, C. -M., Pombeiro, A. J. L. (2013). Tautomeric effect of hydrazone schiff bases in tetranuclear Cu(II) complexes: magnetism and catalytic activity towards mild hydrocarboxylation of alkanes. Dalton. Trans. 42, 16578–16587. doi: 10.1039/c3dt52453a
Sutradhar, M., Martins, L. M. D. R. S., Carabineiro, S. A. C., Guedes da Silva, M. F. C., Buijnsters, J. G., Figueiredo, J. L., et al. (2016c). Oxidovanadium(V) complexes anchored on carbon materials as catalysts for the oxidation of 1-phenylethanol. ChemCatChem 8, 2254–2266. doi: 10.1002/cctc.201600316
Sutradhar, M., Martins, L. M. D. R. S., Guedes da Silva, M. F. C., Alegria, E. C. B. A., Liu, C.-M., and Pombeiro, A. J. L. (2014b). Mn(II,II) complexes: magnetic properties and microwave assisted oxidation of alcohols. Dalton Trans. 43, 3966–3977. doi: 10.1039/c3dt52774c
Sutradhar, M., Martins, L. M. D. R. S., Guedes da Silva, M. F. C., Mahmudov, K. T., Liu, C.-M., and Pombeiro, A. J. L. (2015a). Trinuclear Cu(II) structural isomers: coordination, magnetism, electrochemistry and catalytic activity toward oxidation of alkanes. Eur. J. Inorg. Chem. 2015, 3959–3969. doi: 10.1002/ejic.201500440
Sutradhar, M., Martins, L. M. D. R. S., Guedes da Silva, M. F. C., Pombeiro, A. J. L. (2015b). Vanadium complexes: recent progress in oxidation catalysis. Coord. Chem. Rev. 301–302, 200–239. doi: 10.1016/j.ccr.2015.01.020
Sutradhar, M., Martins, L. M. D. R. S., Guedes da Silva, M. F. C., Pombeiro, A. J. L. (2015c). Oxido vanadium complexes with tridentate aroylhydrazone as catalyst precursors for solvent-free microwave-assisted oxidation of alcohol. Appl. Cat. A Gen. 493, 50–57. doi: 10.1016/j.apcata.2015.01.005
Sutradhar, M., Roy Barman, T., Pombeiro, A. J. L., Martins, L. M. D. R. S. (2019). Catalytic activity of polynuclear vs. dinuclear aroylhydrazone Cu(II) complexes in microwave-assisted oxidation of neat aliphatic and aromatic hydrocarbons. Molecules 24:47. doi: 10.3390/molecules24010047
Yue, Q., Gao, E. Q. (2019). Azide and carboxylate as simultaneous coupler for magnetic coordination polymers. Coord. Chem. Rev. 382, 1–31. doi: 10.1016/j.ccr.2018.12.002
Keywords: Cu(II) complexes, coordination polymer, X-ray structure, magnetism, microwave assisted oxidation of alcohols
Citation: Sutradhar M, Alegria ECBA, Barman TR, Guedes da Silva MFC, Liu C-M and Pombeiro AJL (2020) 1D Copper(II)-Aroylhydrazone Coordination Polymers: Magnetic Properties and Microwave Assisted Oxidation of a Secondary Alcohol. Front. Chem. 8:157. doi: 10.3389/fchem.2020.00157
Received: 09 December 2019; Accepted: 21 February 2020;
Published: 06 March 2020.
Edited by:
Feng Luo, East China University of Technology, ChinaReviewed by:
Marta Elena Gonzalez Mosquera, University of Alcalá, SpainLuiz Fernando Cappa De Oliveira, Juiz de Fora Federal University, Brazil
Copyright © 2020 Sutradhar, Alegria, Barman, Guedes da Silva, Liu and Pombeiro. This is an open-access article distributed under the terms of the Creative Commons Attribution License (CC BY). The use, distribution or reproduction in other forums is permitted, provided the original author(s) and the copyright owner(s) are credited and that the original publication in this journal is cited, in accordance with accepted academic practice. No use, distribution or reproduction is permitted which does not comply with these terms.
*Correspondence: Manas Sutradhar, bWFuYXNAdGVjbmljby51bGlzYm9hLnB0; Armando J. L. Pombeiro, cG9tYmVpcm9AdGVjbmljby51bGlzYm9hLnB0