- Molecular Design and Synthesis, Department of Chemistry, KU Leuven, Leuven, Belgium
Herein, we present the first synthetic methodologies toward non-symmetrical 5,5′-C, C-linked bi-1,2,3-triazoles starting from 5-formyl-1,2,3-triazole via two related pathways. In a first reaction, 5-formyl-1,2,3-triazole is successfully reacted with a variety of nitroalkanes and organic azides in a one-pot three-component fashion resulting in tetra-ortho-substituted bi-1,2,3-triazoles. In the second, closely related reaction, 5-formyl-1,2,3-triazole is initially converted with nitromethane to the corresponding nitroalkene, and then subsequently oxidatively cyclized with a number of organic azides toward 4-nitro substituted non-symmetrical tetra-ortho-substituted 5,5′-bi-1,2,3-triazoles. The scope of both reactions and furtherr post-functionalizations are examined, and the atropisomeric properties of the obtained bi-1,2,3-triazoles are evaluated.
Introduction
The synthesis of bi-1,2,3-triazole derivatives is scarcely reported, and hence their characteristics and potential applications in e.g., enantioselective synthesis, are strongly underexplored (Zheng et al., 2015; Dawood et al., 2018). The bi-1,2,3-triazoles that have been reported to date are divided into three groups in recent reviews, i.e., symmetrical and unsymmetrical 4,4′-bi-1,2,3-triazoles, and symmetrical 5,5'-bi-1,2,3-triazoles (Zheng et al., 2015; Dawood et al., 2018). For the latter type, the only used method is a modified copper(I)-catalyzed azide-alkyne cycloaddition reaction first reported by Burgess in 2007 (Angell and Burgess, 2007). Via this way, only symmetrical bi-1,2,3-triazoles can be obtained (Angell and Burgess, 2007; Oladeinde et al., 2010; González et al., 2011; Kwon et al., 2012; Zheng et al., 2012, 2015; Wang et al., 2014; Hoyo et al., 2015; Brassard et al., 2016; Etayo et al., 2017; Dawood et al., 2018; Li et al., 2018; Singh et al., 2018). Interestingly, Péricas et al. reported the preparation of 5,5′-bi-1,2,3-triazoles derived from an enantiopure and sterically encumbered propargylamine, but the target compound was only obtained in a poor yield of 8% (Etayo et al., 2017). These 5,5′-bi-1,2,3-triazoles exhibited a relatively high conformational stability (113–117 kJ/mol at 75°C in toluene-d8), and were successfully applied in the scandium-catalyzed addition of indoles to isatin. Other axially chiral N, N-dimethylpropargylamine-derived bi-1,2,3-triazoles showed fast rotation at room temperature (79.5 kJ/mol at 25°C in n-hexane/ethanol) (Etayo et al., 2017). This was the first study of the conformational stability of 5,5′-bi-1,2,3-triazoles. Nonetheless, this field remains strongly underexplored. The lack of investigation in this area could be ascribed to the complicated syntheses of both starting materials (functionalized alkynes) and bi-1,2,3-triazoles themselves, and hence the cumbersome introduction of functional groups interesting for various applications (Zheng et al., 2015; Dawood et al., 2018). In regard with our current interests in sterically encumbered fully substituted (atropisomeric) 1,2,3-triazoles (Thomas et al., 2014, 2016; Vroemans et al., 2018; Krasniqi and Dehaen, 2019), we report what are to the best of our knowledge the first pathways toward novel unsymmetrically tetra-ortho-substituted 5,5′-bi-1,2,3-triazoles from easily accessible starting materials. The anticipated highly sterically hindered bi-1,2,3-triazoles could be decorated with various functional groups which can be introduced in a straightforward manner from ortho, ortho'(1,4-)-disubstituted 5-formyl-1,2,3-triazoles, and nitroalkane derivatives.
Results and Discussion
The common precursor that was envisaged for the synthesis of the desired tetra-ortho-substituted bi-1,2,3-triazoles 8 and 9 is 5-formyl-1,2,3-triazole 4, which itself can be easily prepared either from the commercially available methyl 4-methoxyacetoacetate 1 (Scheme 1; NMR spectra of all novel compounds are displayed in the Supplementary Material) or ethyl 4,4-diethoxy-3-oxobutanoate (L'abbé and Dehaen, 1988; Pokhodylo et al., 2018). The aldehyde moiety serves as a versatile tool which can be employed in our in-house developed three-component reaction (Thomas et al., 2014), or can be converted to nitroalkene derivative 5 (Nomland and Hills, 2008) which can subsequently undergo the copper-catalyzed oxidative [3+2]-cycloaddition reaction reported by the group of Chen (Chen et al., 2015). Hence, we commenced by synthesizing starting materials 4 and 5, which was initiated by performing the Dimroth reaction with methyl 4-methoxyacetoacetate 1 and phenyl azide 2, and not starting from ethyl 4,4-diethoxy-3-oxobutanoate which needs to be prepared itself. The desired 5-methoxybenzyl analog 3 was easily obtained on multigram scale via subsequent precipitation and recrystallization. Next, aldehyde 4 was obtained via the photochemical conversion of the methyl ether with bromine in 65% yield. For the second procedure, i.e., the oxidative [3+2]-cycloaddition reaction, 5-formyl-1,2,3-triazole 4 was converted into nitroalkene derivative 5. With both aldehyde 4 and nitroalkene 5 available on gram scale, these could now be employed as starting materials in the subsequent synthesis of unsymmetrical tetra-ortho-substituted 5,5′-bi-1,2,3-triazoles 8 and 9.
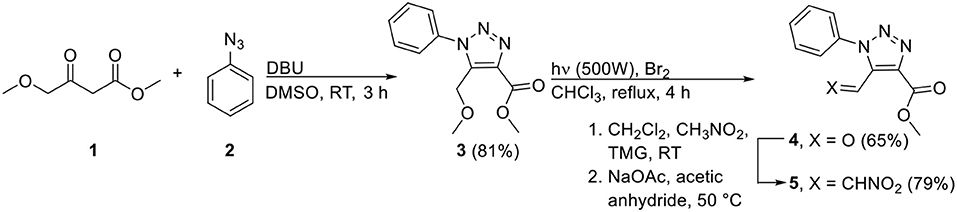
Scheme 1. Synthesis of 5-formyl- and 5-nitrovinyl-appended 1,2,3-triazoles 4 and 5 (TMG = 1,1,3,3-tetramethylguanidine).
Next, the three-component reaction was applied as described by our group on 5-formyl-1,2,3-triazole 4 with a variety of nitroalkanes 6a-d and organic azides 2, 7a-e (Figure 1). Firstly, various alkyl and aryl azides were employed with aldehyde 4 and ethyl nitroacetate 6a as model substrates. Good to excellent yields (56–87%) of bi-1,2,3-triazoles 8 were obtained for alkyl azides 7a-d. Interestingly, enantiopure chiral alkyl azide (R)-7b yielded a 2.25:1-diastereomeric mixture, which was successfully separated via silica gel chromatography into single diastereomers 8ba and 8bb. In general, the use of aryl azides 2 and 7e involved an extended reaction time and slightly decreased yields (21–36%) compared to alkyl azides, and the reaction even failed to afford any product when electron-deficient 4-nitrophenyl azide was employed. Secondly, other functional groups were introduced by varying nitroalkanes 6a-6d. Benzoyl- and phenylsulfonyl-appended bi-1,2,3-triazoles 8h and 8i, respectively, were both prepared in 54% yield. The direct three-component reaction toward bromo-derivative 8g seemed cumbersome, in which the formation of the intermediate bromonitroalkene did not proceed. Nevertheless, 8g was isolated in 64% via a two-pot procedure without intermediate purification of the in situ generated bromonitroalkene derivative.
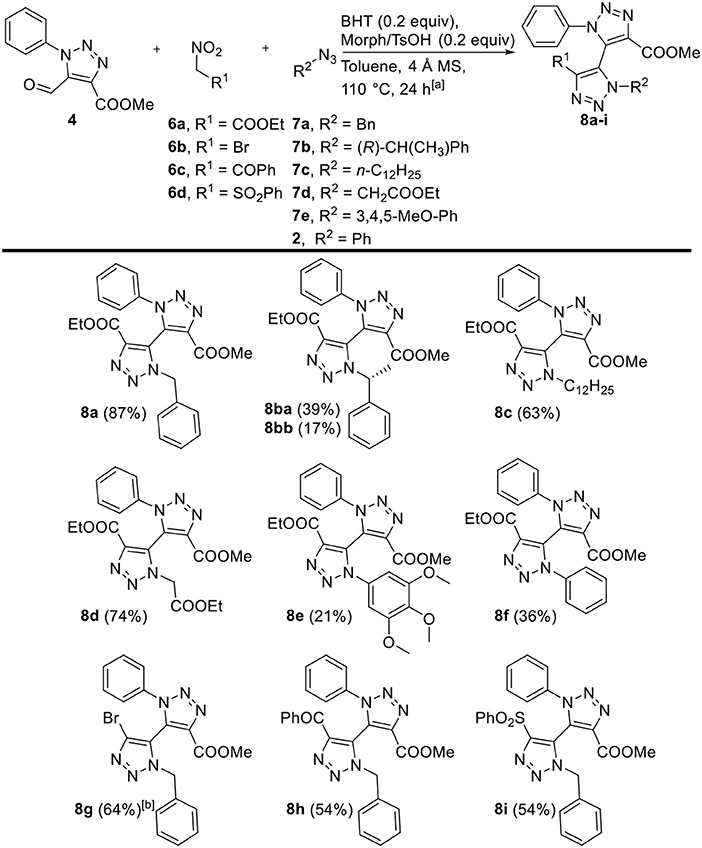
Figure 1. Scope of three-component reaction toward tetra-ortho-substituted 5,5'-bi-1,2,3-triazoles 8a-i, variations with respect to 5-formyl-1,2,3-triazole 4, nitroalkanes 6a–d, and organic azides 2, 7a–e. [a] Reaction conditions: 4 (1 equiv), nitroalkane 6a–d (1.3 equiv), organic azide 2, 7a–e (2 equiv), BHT (0.2 equiv), Morph/TsOH (0.2 equiv), 4 Å MS (50 mg), dry toluene (0.2 mL), 110°C. [b] Reaction conditions: 4 (1 equiv), bromonitromethane (1.2 equiv), TMG (0.1 equiv), dry DCM (0.4 mL); then, NaOAc (1 equiv), acetic anhydride (10 equiv), 50°C; then, 7a (1.5 equiv), BHT (0.1 equiv) and PTSA·H2O (0.1 equiv), dry toluene (1 mL), 110°C.
The introduction of the nitro moiety on the 4-position of 1,2,3-triazoles is highly interesting in view of further transformations, yet tedious to accomplish with the three-component reaction since this would require the use of the hazardous dinitromethane at elevated temperatures. Hence, the copper-catalyzed oxidative [3+2]-cycloaddition reaction with 5-nitrovinyl-appended 1,2,3-triazole 5 serves as a complementairy pathway toward highly interesting 4-nitro substituted non-symmetrical tetra-ortho-substituted 5,5'-bi-1,2,3-triazoles. 5-Nitrovinyl-appended 1,2,3-triazole 5 was subjected to the oxidative [3+2]-cycloaddition conditions with different alkyl and aryl azides 2, 7a-e (Figure 2). Derivatives 9a-d, obtained from alkyl azides 7a-d, were obtained in moderate to good yields (51–70%). Again, the use of (R)-7b yielded a diastereomeric mixture of 1.77:1, which were successfully separated via silica gel chromatography and furnished both isolated diastereomers 9ba and 9bb. The use of aryl azides 2 and 7e furnished derivatives 9e and 9f, whilst again 4-nitrophenyl azide did not furnish any desired product. In general, via this second pathway solely nitro derivatives can be obtained, and the crude reaction mixtures are cleaner than the ones obtained from the three-componentreaction with only the HNO2-eliminated derivatives as minor side products.
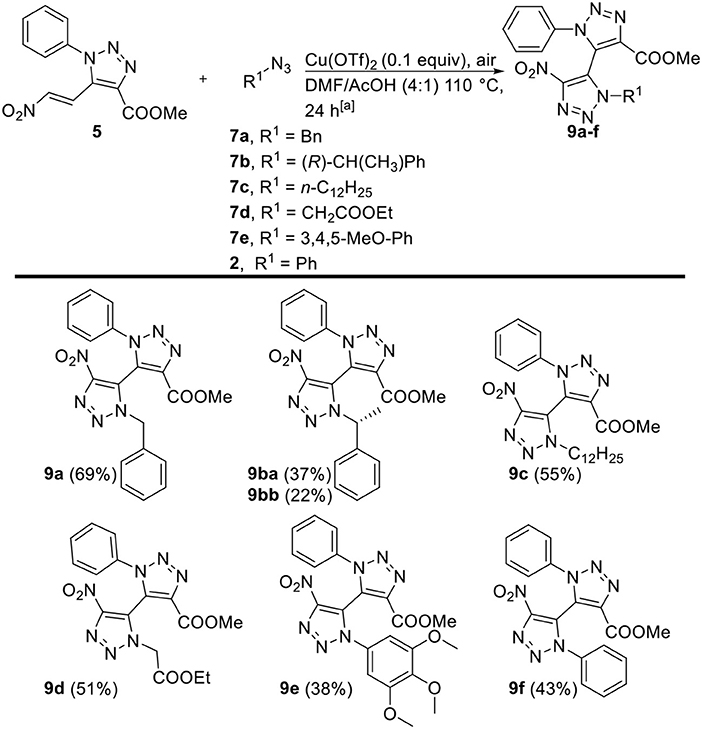
Figure 2. Scope of copper-catalyzed oxidative [3+2]-cycloaddition toward tetra-ortho-substituted 5,5′-bi-1,2,3-triazoles 9a–f, variations with respect to 5-nitrovinyl-1,2,3-triazole 5, and organic azides 2, 7a–e. [a] Reaction conditions: 5 (1 equiv), organic azides 2, 7a–e (1.5 equiv), Cu(OTf)2 (0.1 equiv), air, DMF/AcOH (2.5 mL, 4:1), 110°C.
Several of the obtained bi-1,2,3-triazoles now bear interesting functionalities that could serve as valuable starting point for further derivatization (Scheme 2). Bromo-derivative 8g was subjected to Buchwald-Hartwig amination conditions with pyrrolidine, yet surprisingly the reaction furnished hydrodehalogenated amide 10 in 60% yield. The reactivity of the ester moiety over the bromide of 8g was further exemplified in a substitution reaction with pyrrolidine, in which amide 11 constituting the bromide formed in excellent yields upon reaction at room temperature. Next, hydrolysis of the methyl ester moiety of 9a formed the highly versatile, yet possibly labile, carboxylic acid 12 in 92% yield. Hence, the carboxylic acid was in a next step subjected to heating in order to investigate its thermal stability. Carboxylic acid 12 displayed reasonable stability over the course of several weeks at room temperature, but at 140°C decarboxylation of 12 nicely furnished 13 in good yields (82%). Finally, the reduction of nitro derivative 9f was investigated. Under standard hydrogenation conditions using Pd/C, the versatile free 4-amino-appended derivative 14 was obtained in 44% yield, without the observation of ring-closed amide 15. Further cyclization toward bis-1,2,3-triazolo-fused 2-pyridone 15 was achieved under acidic conditions at 80°C, in which 15 was obtained in 76% yield.
The newly prepared tetra-ortho-substituted 5,5'-bi-1,2,3-triazoles are fascinating structures in the viewpoint of obtaining novel 1,2,3-triazole-containing axially chiral derivatives. The presence of diastereotopic splitting displayed in the 1H NMR spectra of the synthesized products strongly indicates this existence of conformationally stable atropisomers (Figure 3). Hence, VT-NMR studies were conducted in tetrachloroethane-d4 for both 8a and 9a to have an estimate on the energy barrier to rotation for these novel bi-1,2,3-triazoles. At 25°C, both compounds show well-separated signals of the diastereotopic benzylic protons at 5.59 (J = 15.0 Hz) and 5.34 ppm (J = 15.0 Hz) for compound 8a, and at 5.67 (J = 14.9 Hz) and 5.42 ppm (J = 14.9 Hz) for compound 9a, both as clear AX spin systems. Moreover, compound 8a also displayed split signals of the diastereotopic methylene protons of the ester group at 4.18 (J = 10.9, 7.1 Hz) and 4.11 ppm (J = 10.9, 7.1 Hz). Subsequently, from the high temperature NMR spectra at 115°C, it can be observed, based on the chemical shifts, that the diastereotopic signals of both compounds 8a and 9a move closer to each other when the temperature rises, i.e., benzylic signals of 8a are positioned at 5.48 (J = 15.0 Hz) and 5.36 ppm (J = 15.0 Hz) at 115°C, the signals of the methylene protons of the ester are positioned at 4.25 (J = 10.8, 7.2 Hz) and 4.18 ppm (J = 10.8, 7.2 Hz) at 115°C, and for 9a the benzylic signals are positioned at 5.55 (J = 14.9 Hz) and 5.43 ppm (J = 15.0 Hz) at 115°C (Figure 3). Unfortunately, or fortunately from the perspective of forming conformationally stable atropisomers, no coalescence was observed for the diastereotopic signals when the maximum temperature of 115°C (388 K) was reached. Therefore, the corresponding rotational energy barriers ΔG of these atropisomeric compounds 8a and 9a could not be accurately calculated. Nevertheless, a minimal energy could be tentatively calculated by assuming coalescence at 388 K. Hence, the rotational energy barrier at this temperature for both 8a and 9a is at least 79.5 kJ/mol. In addition, the isolation of both diastereomers via silica gel chromatography was possible for 8ba and 8bb, and 9ba and 9bb. Regrettably, the sets of diastereomers showcased a rather limited rotational stability as they isomerized at room temperature over the course of a couple of days. In future work, the steric hindrance of the ortho-substituents should be increased to create more stable axially chiral compounds. Nevertheless, this is an encouraging result from the perspective of preparing atropisomeric unsymmetrically substituted bi-1,2,3-triazoles.
Conclusion
We report herein the synthesis toward unsymmetrically substituted 5,5'-bi-1,2,3-triazoles from 5-formyl-1,2,3-triazoles directly or indirectly via two distinct methodologies, i.e., a three-component reaction from 5-formyl-1,2,3-triazole, nitroalkanes, and organic azides yielding variously substituted 5,5'-bi-1,2,3-triazoles, and the copper-catalyzed oxidative [3+2]-cycloaddition reaction from 5-nitrovinyl-appended 1,2,3-triazole and organic azides resulting in 4-nitro-functionalized bi-1,2,3-triazoles. Both reactions displayed a versatile scope toward various alkyl and aryl azides, and via the use of both aforementioned procedures various attractive functional groups can be incorporated. Postfunctionalizations further emphasized their peculiar and interesting chemistries for future applications. Rotational stability tests showed promising characteristics as atropisomers, although the rotational barrier was still rather limited and could be further increased by implementing more sterically hindered ortho-substituents.
Data Availability Statement
All datasets generated for this study are included in the article/Supplementary Material.
Author Contributions
RV and TH carried out the experiments, analyzed the data, and wrote the manuscript. MV carried out some of the experiments and analyzed the data. WD directed the project and corrected the manuscript.
Conflict of Interest
The authors declare that the research was conducted in the absence of any commercial or financial relationships that could be construed as a potential conflict of interest.
Acknowledgments
We thank the KU Leuven for financial support. RV thanks the Fonds Wetenschappelijk Onderzoek—Vlaanderen (FWO) for a Ph.D. fellowship (1S13516N), WD thanks the FWO for a bilateral grant with Brazil (G0F6619N). Mass spectrometry was made possible by the support of the Hercules Foundation of the Flemish Government (grant 20100225-7).
Supplementary Material
The Supplementary Material for this article can be found online at: https://www.frontiersin.org/articles/10.3389/fchem.2020.00271/full#supplementary-material
References
Angell, Y., and Burgess, K. (2007). Base dependence in copper-catalyzed huisgen reactions: efficient formation of bistriazoles. Angew. Chemie Int. Ed. 46, 3649–3651. doi: 10.1002/anie.200700399
Brassard, C. J., Zhang, X., Brewer, C. R., Liu, P., Clark, R. J., and Zhu, L. (2016). Cu(II)-catalyzed oxidative formation of 5,5′-bistriazoles. J. Org. Chem. 81, 12091–12105. doi: 10.1021/acs.joc.6b01907
Chen, Y., Nie, G., Zhang, Q., Ma, S., Li, H., and Hu, Q. (2015). Copper-catalyzed [3+2] cycloaddition/oxidation reactions between nitro-olefins and organic azides: highly regioselective synthesis of NO2-substituted 1,2,3-triazoles. Org. Lett. 17, 1118–1121. doi: 10.1021/ol503687w
Dawood, K. M., Abdel-Wahab, B. F., and Raslan, M. A. (2018). Synthesis and applications of bi- and bis-triazole systems. Arkivoc 2018, 179–215. doi: 10.24820/ark.5550190.p010.522
Etayo, P., Escudero-Adán, E. C., and Pericàs, M. A. (2017). 5,5′-Bistriazoles as axially chiral, multidentate ligands: synthesis, configurational stability and catalytic application of their scandium(III) complexes. Catal. Sci. Technol. 7, 4830–4841. doi: 10.1039/C7CY01518F
González, J., Pérez, V. M., Jiménez, D. O., Lopez-Valdez, G., Corona, D., and Cuevas-Yañez, E. (2011). Effect of temperature on triazole and bistriazole formation through copper-catalyzed alkyne–azide cycloaddition. Tetrahedron Lett. 52, 3514–3517. doi: 10.1016/j.tetlet.2011.05.002
Hoyo, A. M., del Latorre, A., Díaz, R., Urbano, A., and Carreño, M. C. (2015). Enantiopure helical ferrocene-triazole-quinone triads: synthesis and properties. Adv. Synth. Catal. 357, 1154–1160. doi: 10.1002/adsc.201400998
Krasniqi, B., and Dehaen, W. (2019). Synthesis of 1,2,3-Triazolo-fused allocolchicine analogs via intramolecular oxidative biaryl coupling. Org. Lett. 21, 5002–5005. doi: 10.1021/acs.orglett.9b01707
Kwon, M., Jang, Y., Yoon, S., Yang, D., and Jeon, H. B. (2012). Unusual Cu(I)-catalyzed 1,3-dipolar cycloaddition of acetylenic amides: formation of bistriazoles. Tetrahedron Lett. 53, 1606–1609. doi: 10.1016/j.tetlet.2012.01.069
L'abbé, G., and Dehaen, W. (1988). Synthesis and thermal rearrangement of 5-diazomethyl-1,2,3-triazoles. Tetrahedron 44, 461–469. doi: 10.1016/S0040-4020(01)85837-3.
Li, L., Huang, S., Shang, T., Zhang, B., Guo, Y., Zhu, G., et al. (2018). Medium rings bearing bitriazolyls: easily accessible structures with superior performance as Cu catalyst ligands. J. Org. Chem. 83, 13166–13177. doi: 10.1021/acs.joc.8b01899
Nomland, A., and Hills, I. D. (2008). 2-Quinolinecarboxaldehyde: an unusual partner in the Henry reaction and subsequent elimination. Tetrahedron Lett. 49, 5511–5514. doi: 10.1016/j.tetlet.2008.07.044
Oladeinde, O. A., Hong, S. Y., Holland, R. J., Maciag, A. E., Keefer, L. K., Saavedra, J. E., et al. (2010). “Click” reaction in conjunction with diazeniumdiolate chemistry: developing high-load nitric oxide donors. Org. Lett. 12, 4256–4259. doi: 10.1021/ol101645k
Pokhodylo, N. T., Shyyka, O. Y., and Obushak, M. D. (2018). Convenient synthetic path to ethyl 1-aryl-5-formyl-1H-1,2,3-triazole-4-carboxylates and 1-aryl-1,5-dihydro-4H-[1,2,3]triazolo[4,5-d]pyridazin-4-ones. Chem. Heterocycl. Compd. 54, 773–779. doi: 10.1007/s10593-018-2348-1
Singh, K., Sharma, G., Shukla, M., Kant, R., Chopra, S., Shukla, S. K., et al. (2018). Metal- and phenol-free synthesis of biaryl ethers: access to dibenzobistriazolo-1,4,7-oxadiazonines and vancomycin-like glyco-macrocycles as antibacterial agents. J. Org. Chem. 83, 14882–14893. doi: 10.1021/acs.joc.8b01631
Thomas, J., Jana, S., John, J., Liekens, S., and Dehaen, W. (2016). A general metal-free route towards the synthesis of 1,2,3-triazoles from readily available primary amines and ketones. Chem. Commun. 52, 2885–2888. doi: 10.1039/C5CC08347H
Thomas, J., John, J., Parekh, N., and Dehaen, W. (2014). A metal-free three-component reaction for the regioselective synthesis of 1,4,5-trisubstituted 1,2,3-triazoles. Angew. Chemie 126, 10319–10323. doi: 10.1002/ange.201403453
Vroemans, R., Tran, M. T. D., Sayed, M. G., Boodts, S., and Dehaen, W. (2018). Synthesis and characterization of novel axially chiral β-linked 1,2,3-triazolyl porphyrins. Dye. Pigment. 156, 61–66. doi: 10.1016/j.dyepig.2018.03.056
Wang, C.-Y., Zou, J.-F., Zheng, Z.-J., Huang, W.-S., Li, L., and Xu, L.-W. (2014). BINOL-linked 1,2,3-triazoles: an unexpected fluorescent sensor with anion–π interaction for iodide ions. RSC Adv. 4, 54256–54262. doi: 10.1039/C4RA09589H
Zheng, Z.-J., Wang, D., Xu, Z., and Xu, L.-W. (2015). Synthesis of bi- and bis-1,2,3-triazoles by copper-catalyzed Huisgen cycloaddition: a family of valuable products by click chemistry. Beilstein J. Org. Chem. 11, 2557–2576. doi: 10.3762/bjoc.11.276
Zheng, Z.-J., Ye, F., Zheng, L.-S., Yang, K.-F., Lai, G.-Q., and Xu, L.-W. (2012). Copper-catalyzed huisgen and oxidative huisgen coupling reactions controlled by polysiloxane-supported amines (AFPs) for the divergent synthesis of triazoles and bistriazoles. Chem. A Eur. J. 18, 14094–14099. doi: 10.1002/chem.201202472
Keywords: bi-1,2,3-triazole, atropisomer, nitro compounds, 1,2,3-triazoles, 1,3-dipolar cycloadditions
Citation: Vroemans R, Horsten T, Van Espen M and Dehaen W (2020) 5-Formyltriazoles as Valuable Starting Materials for Unsymmetrically Substituted Bi-1,2,3-Triazoles. Front. Chem. 8:271. doi: 10.3389/fchem.2020.00271
Received: 14 January 2020; Accepted: 19 March 2020;
Published: 15 April 2020.
Edited by:
Alexey Sukhorukov, N.D. Zelinsky Institute of Organic Chemistry (RAS), RussiaReviewed by:
Azim Ziyaei Halimehjani, Kharazmi University, IranHelio A. Stefani, University of São Paulo, Brazil
Copyright © 2020 Vroemans, Horsten, Van Espen and Dehaen. This is an open-access article distributed under the terms of the Creative Commons Attribution License (CC BY). The use, distribution or reproduction in other forums is permitted, provided the original author(s) and the copyright owner(s) are credited and that the original publication in this journal is cited, in accordance with accepted academic practice. No use, distribution or reproduction is permitted which does not comply with these terms.
*Correspondence: Wim Dehaen, d2ltLmRlaGFlbkBrdWxldXZlbi5iZQ==
†These authors have contributed equally to this work