- 1School of Chemistry and Chemical Engineering, Jinggangshan University, Ji'an, China
- 2State Key Laboratory of Materials Processing and Die & Mold Technology, School of Materials Science and Engineering, Huazhong University of Science and Technology, Wuhan, China
A novel radical reaction of monometallofullerene Y@C2v(9)-C82 with N-arylbezamidine (1) is successfully conducted through catalysis with silver carbonate. The high-performance liquid chromatographic and mass spectrum results demonstrate that the reaction is highly regioselective to afford only one monoadduct (2) with an imidazoline group added on C82 cage, and computations through density functional theory reveal the addition group is attached to a specific [5, 6]-bond (C20-C76) near the Y atom. Furthermore, the analysis of prymidalization angle of the carbon atoms demonstrates the geometry of carbon cage is in favor of the regioselective formation of isomer (20, 76).
Introduction
Exohedral chemical functionalization of fullerenes has a great significance toward their potential applications in photovoltaic and biomedical fields. To date, a large number of reactions, including but not limit to Bingle–Hirsch reactions, Prato reactions, Diels–Alder reactions, and radical reactions, have been successfully performed to modulate their chemical and physical properties (Hirsch and Brettreich, 2005). Among them, of particular interest are the radical reactions of fullerenes mediated by transition-metal salts (Tzirakis and Orfanopoulos, 2013), and many catalyzers, such as Mn(OAc)3, Fe(ClO4)3 (Zhang et al., 2003; Wang et al., 2004; Li et al., 2010a,b, 2012; Liu et al., 2011), Pb(OAc)4 (Chai and Lautens, 2009), Cu(OAc)2 (Wang and Li, 2005), CoCl2 (Lu S. et al., 2011), NiCl2 (Constable et al., 2012), CuCl2 (Yang et al., 2013; Sharma et al., 2018), and FeCl3 (Hashiguchi et al., 2013, 2014), have been utilized to produce radicals of fullerenes to promote the radical reactions taking advantage of their excellent catalytic activities. Because of the high efficiency in constructing multiple new chemical bonds, thus leading to derivatives with various structures in one step, this class of reactions becomes more and more important in functionalization of fullerenes.
On the other hand, endohedral metal doping of the fullerene cages can generate a novel class of hybrid molecules named endohedral metallofullerenes (EMFs) (Lu et al., 2012; Popov et al., 2013; Yang et al., 2017; Bao et al., 2018). The interaction between the internal metallic unit and fullerene carbon cages renders the chemistry of EMFs more complicated but also intriguing compared to that of empty fullerenes (Lu X. et al., 2011; Maeda et al., 2016). As a result, the amount of metal-mediated reactions of EMFs lags far behind those of fullerenes and the regioselectivity is just satisfactory with the generation of two or more isomers. Typically, Gu et al. synthesize a series of water-soluble multiadducts of Tb@C82 with Cu(MeCN)4PF6 as a catalyst in 2002 (Feng et al., 2002), and subsequently Dorn et al. utilized manganese(III) acetate to trigger the radical reaction of Sc3N@C80 and obtained two isomers methano monoadducts in 2007 (Feng et al., 2002; Shu et al., 2007). The rare reports about this kind of reactions can be understood by considering the direct reaction of EMFs with metallic salts such as CuCl2, NiCl2, and FeCl3, which forms solid precipitate instead of target reactants (Stevenson et al., 2009, 2014; Stevenson and Rottinger, 2013; Wang et al., 2017), and thus it is still of high interest and challenge to seek for the catalyst with appropriate activity and the regioselectivity for the metal-mediated reactions of EMFs.
In this work, we found that silver carbonate is an efficient catalyst to promote the reaction of Y@C2v(9)-C82 with N-arylbenzamidine. Remarkably, the reaction regioselectively affords only one derivative as revealed by high-performance liquid chromatography (HPLC) and mass spectrometry (MS), and the density functional theory calculations predict the addition is preferentially occurred on a specific [5, 6]-bond with large prymidalization angle near the Y atom.
Materials and Methods
The solvent toluene was freshly distilled with sodium prior to usage. The reagent Ag2CO3 was obtained commercially, and N-arylbenzamidine was synthesized according to a previous report, and the structure was determined through 1H NMR (Supplementary Figure 5) (Koutentis and Mirallai, 2010). Y@C2v(9)-C82 was produced with arc-discharge method and isolated with HPLC. Analytical and preparative HPLC measurements were conducted on LC SPD-16 and LC 908 machines (Japan Analytical Industry Co., Ltd.), respectively. Matrix-assisted laser desorption/ionization time-of-flight (MALDI-TOF) MS was measured on a MICROFLEX spectrometer (Bruker Daltonics Inc., Germany), using 1,1,4,4-tetraphenyl-1,3-butadiene as matrix in a positive ion linear mode.
Y@C2v(9)-C82 and all possible isomers formed by the addition of 1 on non-equivalent C-C bonds, were constructed and optimized at HF level of theory with 3-21G for C, H, and N atoms and LANL2DZ for Y. Among them, low-lying isomers were chosen out and reoptimized using B3LYP (Lee et al., 1988; Becke, 1993) functional with 6-31G(d) for non-metals and SDD for Y. All computations were performed with Gaussian 09 Program (Frisch et al., 2013), and numbering of carbons in C2v(9)-C82 cage was given in Supplementary Figure 1 according to CAGE code (Brinkmann et al., 2010).
Results and Discussion
In a typical reaction, 5.0 mg (4.7 μmol) of Y@C2v(9)-C82 and 18.4 mg (20 eq) of N-arylbenzamidine (1) were dissolved in 25 mL of anhydrous toluene, and 13.0 mg (10 eq) of Ag2CO3 was added into the solution, and then the mixture was heated at reflux under argon (Figure 1A). The reaction process was monitored through analytical HPLC, and the profiles are shown in Figure 1B. At the beginning of the reaction, two peaks corresponding to the solvent and pristine metallofullerene were detected at 3.6 and 35.4 min, respectively. A new peak at 12.9 min increased along with the decreasing amount of metallofullerene, and the reaction was terminated because the peak almost keeps constant after 12 h. The above HPLC results demonstrate that the reaction possesses moderate reactivity and high selectivity. Besides, some silver salts such as silver nitrate, silver acetate, and silver trifluoroacetate, were applied to replace the catalyst silver carbonate; however, the results monitored by HPLC show no product was detected, but decreasing amount of the pristine metallofullerene, which is probably because Y@C2v(9)-C82 directly reacts and forms precipitates with these salts, impeding their mediated reaction between metallofullerene and 1.
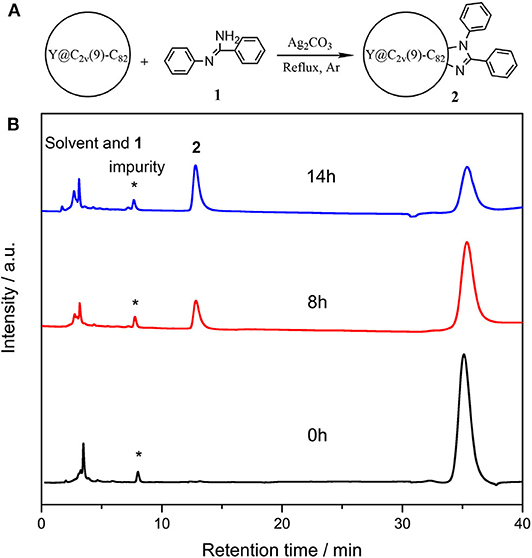
Figure 1. (A) Scheme of the reaction o of Y@C2v(9)-C82 with 1 and (B) analytical HPLC profiles of the reaction mixture of Y@C2v(9)-C82 and 1 probed at different time. HPLC condition: Buckyprep column (Φ4.6 mm × 250 mm); 20 μL injection volume; 1.0 mL/min toluene flow; room temperature; 330 nm detecting wavelength.
The reaction mixture was then concentrated and subjected to further HPLC separations (Supplementary Figure 1), and 3.4 mg of pure compound 2 as black solids was obtained, and 2 mg of Y@C2v(9)-C82 was recollected. A large portion (up to 95%) of consumed EMF was converted to 2, even much excess amounts of 1 were added, indicating the high regioselectivity of this catalytic reaction. The purified 2 is characterized through the MALDI-TOF MS, and the result shows only one peak at m/z 1267 was detected (Figure 2). It demonstrates that a group with 194 of molecular weight was added on the carbon cage, which is similar to the results of the reaction of C60 with 1 affording an imidazoline monoadduct (He et al., 2013). Consequently, it is speculated that 2 should be an imidazoline monoadduct too.
Based on the above experimental results and previous reports about metal-mediated fullerene reactions (He et al., 2013; Aghabali et al., 2016; Chao et al., 2016), a plausible reaction mechanism for this silver carbonate-catalyzed reaction is proposed in Figure 3. At first, N-arylbenzimidamide 1 directly reacts with Ag+, which generates 3, and then a radical species 4 is obtained through homolytic cleavage of the nitrogen silver bond. Second, the radical addition of Y@C2v(9)-C82 will produce intermediate fullerenyl radical 5, which may also be formed by the homolytic addition of 3 to metalofullerene, and then the intramolecular cyclization of 5 produces radical species 6. Finally, the intermediate 6 is oxidated by Ag+ and loses the extra H+, affording the imidazoline derivative 2.
Theoretical calculations were conducted to further determine the accurate structure and addition site of 2. There are in total 35 non-equivalent bonds in the cage when C2v symmetry was taken into consideration, and all the corresponding isomers of 2 named according to the addition bonds were optimized, and the relative energies are given in Supplementary Table 1. Three low-lying isomers were reoptimized using B3LYP functional with SDD basis for Y and 6-31G(d) for non-metal atoms; more accurate relative energies are obtained and given in Figure 4. The results show the energy of isomer (20, 76) is far lower than those of other isomers (63, 77) and (56, 41) with 5.9 and 7.6 kcal/mol, respectively, indicating the reaction should prefer to occur at the [5, 6]-bond near the internal metal atom. Besides relative energies, stability of isomer (20, 76) can also be disclosed by inspection on its spin density and singly occupied molecular orbital (SOMO), which show the spin density and SOMO of isomer (20, 76) are not concentrated on some specific carbons but evenly distributed over the whole cage (Supplementary Figure 3).
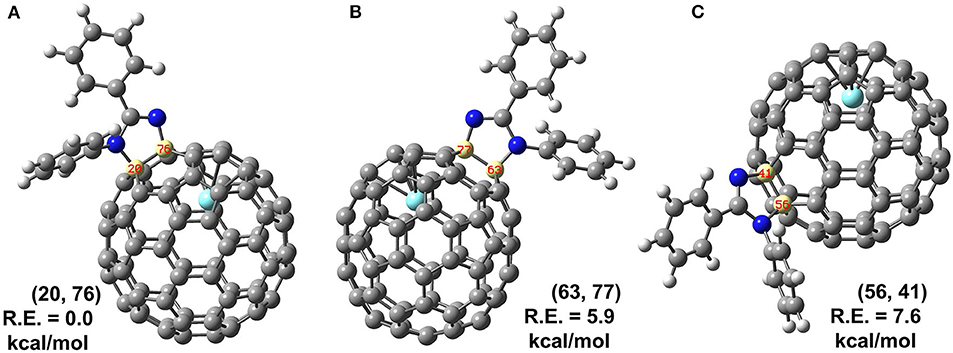
Figure 4. (A–C) Structures and relative energies (R.E., in kcal/mol) of isomers (20, 76), (63, 77) and (56, 41) predicated by B3LYP method. Isomers are labeled by a pair of numbers to indicate which carbons are attached by 1.
Furthermore, the kinetic stability of isomer (20, 76) can also be rationalized from the pyramidalization angles of carbon atoms on the C2v(9)-C82 cage, which are strongly dependent on the cage geometry. In general, the addition reactions of fullerenes preferentially occur at the carbon atoms with relative high spin population and/or large POAV [the p-orbital axis vector (θΔπ − 90°)] values. The B3LYP predicated spin density of Y@C2v(9)-C82 and spin population condensed on each carbon atom are given in Supplementary Figure 4. The spin density distributed over the cage quite evenly and spin population of carbon atoms are also relatively small (ranges from −0.027 to 0.082), which is in agreement with the previous report (Bao et al., 2016). As a consequence, there are no carbon atoms of Y@C2v(9)-C82 possessing the distinct advantage of radical to regioselectively react with 1. In contrast, as can be seen in Figure 5, the carbon atoms located near the Y atom possess evidently higher POAV values than others. In fact, the carbon atom C76 has largest POAV value up to 11.6°, and thus, C76 is certainly more reactive than other cage carbons to release its steric strain. After linking with C76, the adjacent carbon atom C20 has higher POAV value (9.7) than the other two adjacent carbon atoms C75 (5.3) and C77 (5.3), and thus, the addition group makes a second bond with C20 to form a [5, 6] monoadduct. Accordingly, the above results reveal the geometry of the carbon cage plays a role on the regioselective formation of the isomer (20, 76).
Conclusion
In summary, a novel derivative 2 of monometallofullerene was synthesized via a highly regioselective reaction of 1 catalyzed by silver carbonate. Studies of MS and theoretical calculations disclose that an imidazoline group is attached to [5, 6]-bond near the metal atom forming a monoadduct. Additionally, the analysis of POAV values on carbon cages demonstrates that the geometry of carbon cage is conductive to regioselectively afford the isomer (20, 76). We believe the successful functionalization of metallofullerene mediated by transition metal will broaden the approach to chemistry of EMFs, which may find applications in photovoltaic and biomedical fields.
Data Availability Statement
All datasets generated for this study are included in the article/Supplementary Material.
Author Contributions
Experiments conceived and designed and the paper written by CP. Experiments and calculations performed by CP, JL, and PY. WW, XY, PL, JZ, and ZL analyzed the data. All authors contributed to the article and approved the submitted version.
Funding
This study was supported by National Nature Science Foundation of China NSFC (Nos. 21901090, 21802057, and 21965015) and Jiangxi Provincial Department of Education's Item of Science and Technology (No. 180590).
Conflict of Interest
The authors declare that the research was conducted in the absence of any commercial or financial relationships that could be construed as a potential conflict of interest.
Supplementary Material
The Supplementary Material for this article can be found online at: https://www.frontiersin.org/articles/10.3389/fchem.2020.593602/full#supplementary-material
References
Aghabali, A., Jun, S., Olmstead, M. M., and Balch, A. L. (2016). Silver(I)-mediated modification, dimerization, and polymerization of an open-cage fullerene. J. Am. Chem. Soc. 138, 16459–16465. doi: 10.1021/jacs.6b10394
Bao, L., Pan, C., Slanina, Z., Uhlik, F., Akasaka, T., and Lu, X. (2016). Isolation and crystallographic characterization of the labile isomer of Y@C82 cocrystallized with Ni(OEP): unprecedented dimerization of pristine metallofullerenes. Angew. Chem. Int. Ed. 55, 9234–9238. doi: 10.1002/anie.201604121
Bao, L., Peng, P., and Lu, X. (2018). Bonding inside and outside fullerene cages. Acc. Chem. Res. 51, 810–815. doi: 10.1021/acs.accounts.8b00014
Becke, A. D. (1993). Density-functional thermochemistry. III. The role of exact exchange. J. Chem. Phys. 98, 5648–5652. doi: 10.1063/1.464913
Brinkmann, G., Delgado-Friedrichs, O., Lisken, S., Peeters, A., and Van Cleemput, N. (2010). CaGe: a virtual environment for studying some special classes of plane graphs : an update. Match Commun. Math. Comput. Chem. 63, 533–552. doi: 10.1007/s10985-009-9124-6
Chai, D. I., and Lautens, M. (2009). Tandem Pd-catalyzed double C–C bond formation: effect of water. J. Org. Chem. 74, 3054–3061. doi: 10.1021/jo900053b
Chao, D., Liu, T.-X., Ma, N., Zhang, P., Fu, Z., Ma, J., et al. (2016). Silver(I)-mediated three-component annulation reaction of [60]fullerene, sulfonylhydrazones, and nitriles: leading to diverse disubstituted [60]fullerene-fused dihydropyrroles. Chem. Commun. 52, 982–985. doi: 10.1039/C5CC07218B
Constable, E. C., Zhang, G., Housecroft, C. E., and Zampese, J. A. (2012). Bucky-blocks: templating a coordination network with C60. CrystEngComm 14, 1770–1774. doi: 10.1039/C2CE06156B
Feng, L., Zhang, X. M., Yu, Z. P., Wang, J. B., and Gu, Z. N. (2002). Chemical modification of Tb@C82 by copper(I)-catalyzed cycloadditions. Chem. Mater. 14, 4021–4022. doi: 10.1021/cm025532o
Frisch, M. J., Trucks, G. W., Schlegel, H. B., Scuseria, G. E., Robb, M. A., Cheeseman, J. R., et al. (2013). Gaussion 09, Revision D. Wallingford, CT: Gaussian, Inc.
Hashiguchi, M., Inada, H., and Matsuo, Y. (2013). Solution-phase synthesis of dumbbell-shaped C120 by FeCl3-mediated dimerization of C60. Carbon 61, 418–422. doi: 10.1016/j.carbon.2013.04.101
Hashiguchi, M., Ueno, T., and Matsuo, Y. (2014). FeCl3-mediated retro-reactions of fullerene derivatives to C60. Fullerenes Nanotubes Carbon Nanostruct. 22, 845–852. doi: 10.1080/1536383X.2012.742429
He, C.-L., Liu, R., Li, D.-D., Zhu, S.-E., and Wang, G.-W. (2013). Synthesis and functionalization of [60]Fullerene-fused imidazolines. Org. Lett. 15, 1532–1535. doi: 10.1021/ol400319w
Koutentis, P. A., and Mirallai, S. I. (2010). Reinvestigating the synthesis of N-arylbenzamidines from benzonitriles and anilines in the presence of AlCl3. Tetrahedron 66, 5134–5139. doi: 10.1016/j.tet.2010.04.103
Lee, C., Yang, W., and Parr, R. G. (1988). Development of the Colle-Salvetti correlation-energy formula into a functional of the electron density. Phys. Rev. B 37, 785–789. doi: 10.1103/PhysRevB.37.785
Li, F., Zhu, S., You, X., and Wang, G. (2012). Ferric perchlorate-promoted reaction of [60]fullerene with β-keto esters. Chin. Sci. Bull. 57, 2269–2272. doi: 10.1007/s11434-012-5103-5
Li, F.-B., You, X., and Wang, G.-W. (2010b). Synthesis of disubstituted [60]Fullerene-fused lactones: ferric perchlorate-promoted reaction of [60]Fullerene with malonate esters. Org. Lett. 12, 4896–4899. doi: 10.1021/ol102056k
Li, F.-B., Zhu, S., and Wang, G. (2010a). Manganese(III) acetate-mediated radical reaction of [60]fullerene with bromoacetic acid, 3-chloropropionic acid or 1-naphthylacetic acid. Chin. Sci. Bull. 55, 2909–2914. doi: 10.1007/s11434-010-4016-4
Liu, T.-X., Li, F.-B., and Wang, G.-W. (2011). Synthesis of [60]Fullerene-fused tetrahydronaphthalene and indane derivatives via a pathway switched by aluminum chloride. Org. Lett. 13, 6130–6133. doi: 10.1021/ol202645z
Lu, S., Jin, T., Bao, M., and Yamamoto, Y. (2011). Cobalt-catalyzed hydroalkylation of [60]Fullerene with active alkyl bromides: selective synthesis of monoalkylated fullerenes. J. Am. Chem. Soc. 133, 12842–12848. doi: 10.1021/ja204982w
Lu, X., Akasaka, T., and Nagase, S. (2011). Chemistry of endohedral metallofullerenes: the role of metals. Chem. Commun. 47, 5942–5957. doi: 10.1039/c1cc10123d
Lu, X., Feng, L., Akasaka, T., and Nagase, S. (2012). Current status and future developments of endohedral metallofullerenes. Chem. Soc. Rev. 41, 7723–7760. doi: 10.1039/c2cs35214a
Maeda, Y., Tsuchiya, T., Kikuchi, T., Nikawa, H., Yang, T., Zhao, X., et al. (2016). Effective derivatization and extraction of insoluble missing lanthanum metallofullerenes La@C2n (n = 36–38) with iodobenzene. Carbon 98, 67–73. doi: 10.1016/j.carbon.2015.10.088
Popov, A., Yang, S., and Dunsch, L. (2013). Endohedral fullerenes. Chem. Rev. 113, 5989–6113. doi: 10.1021/cr300297r
Sharma, S., Chakrahari, K. K., Saillard, J.-Y., and Liu, C. W. (2018). Structurally precise dichalcogenolate-protected copper and silver superatomic nanoclusters and their alloys. Acc. Chem. Res. 51, 2475–2483. doi: 10.1021/acs.accounts.8b00349
Shu, C., Cai, T., Xu, L., Zuo, T., Reid, J., Harich, K., et al. (2007). Manganese(III)-catalyzed free radical reactions on trimetallic nitride endohedral metallofullerenes. J. Am. Chem. Soc. 129, 15710–15717. doi: 10.1021/ja0768439
Stevenson, S., Mackey, M. A., Pickens, J. E., Stuart, M. A., Confait, B. S., and Phillips, J. P. (2009). Selective complexation and reactivity of metallic nitride and oxometallic fullerenes with lewis acids and use as an effective purification method. Inorg. Chem. 48, 11685–11690. doi: 10.1021/ic9017147
Stevenson, S., and Rottinger, K. A. (2013). CuCl2 for the isolation of a broad array of endohedral fullerenes containing metallic, metallic carbide, metallic nitride, and metallic oxide clusters, and separation of their structural isomers. Inorg. Chem. 52, 9606–9612. doi: 10.1021/ic4013476
Stevenson, S., Rottinger, K. A., Fahim, M., Field, J. S., Martin, B. R., and Arvola, K. D. (2014). Tuning the selectivity of Gd3N cluster endohedral metallofullerene reactions with lewis acids. Inorg. Chem. 53, 12939–12946. doi: 10.1021/ic502024a
Tzirakis, M. D., and Orfanopoulos, M. (2013). Radical reactions of fullerenes: from synthetic organic chemistry to materials science and biology. Chem. Rev. 113, 5262–5321. doi: 10.1021/cr300475r
Wang, G.-W., and Li, F.-B. (2005). Cu(II) acetate- and Mn(III) acetate-mediated radical reactions of [60]fullerene with ketonic compounds. Org. Biomol. Chem. 3, 794–797. doi: 10.1039/b416756b
Wang, G.-W., Zhang, T.-H., Cheng, X., and Wang, F. (2004). Selective addition to [60]fullerene of two different radicals generated from Mn(III)-based radical reaction. Org. Biomol. Chem. 2, 1160–1163. doi: 10.1039/b317084e
Wang, Z., Omachi, H., and Shinohara, H. (2017). Non-chromatographic purification of endohedral metallofullerenes. Molecules 22:718. doi: 10.3390/molecules22050718
Yang, H.-T., Liang, X.-C., Wang, Y.-H., Yang, Y., Sun, X.-Q., and Miao, C.-B. (2013). CuCl2-Mediated reaction of [60]Fullerene with amines in the presence or absence of dimethyl acetylenedicarboxylate: preparation of fulleropyrroline or aziridinofullerene derivatives. J. Org. Chem. 78, 11992–11998. doi: 10.1021/jo402043p
Yang, S., Wei, T., and Jin, F. (2017). When metal clusters meet carbon cages: endohedral clusterfullerenes. Chem. Soc. Rev. 46, 5005–5058. doi: 10.1039/C6CS00498A
Keywords: metallofullerene, radical reaction, silver carbonate, functionalization, imidazoline
Citation: Li J, Yu P, Lai P, Zou J, Liu Z, Yi X, Wang W and Pan C (2020) Regioselective Radical Reaction of Monometallofullerene Y@C2v(9)-C82 With N-arylbenzamidine Mediated by Silver Carbonate. Front. Chem. 8:593602. doi: 10.3389/fchem.2020.593602
Received: 11 August 2020; Accepted: 01 September 2020;
Published: 20 October 2020.
Edited by:
Long Qie, Tongji University, ChinaReviewed by:
Yuanzhi Tan, Xiamen University, ChinaLi Xiaofang, Hunan University of Science and Technology, China
Copyright © 2020 Li, Yu, Lai, Zou, Liu, Yi, Wang and Pan. This is an open-access article distributed under the terms of the Creative Commons Attribution License (CC BY). The use, distribution or reproduction in other forums is permitted, provided the original author(s) and the copyright owner(s) are credited and that the original publication in this journal is cited, in accordance with accepted academic practice. No use, distribution or reproduction is permitted which does not comply with these terms.
*Correspondence: Changwang Pan, cGFuY2hhbmd3YW5nQGpnc3UuZWR1LmNu