- 1Jiangsu Engineering Technology Research Centre for Functional Textiles, Jiangnan University, Wuxi, China
- 2Key Laboratory of Eco-textiles, Ministry of Education, Jiangnan University, Wuxi, China
Broad solar light harvesting and fast photoinduced electron–hole migration are two critical factors for the catalytic capacity of photocatalytic system. In this study, novel visible light–driven carbon dot–TiO2 nanosheet (CD-TN) photocatalysts are successfully prepared by loading CDs on the surface of TNs through the hydrothermal method. The microstructure, chemical components, and optical properties of the prepared samples are characterized via X-ray diffraction, Fourier transform infrared spectroscopy, transmission electron microscopy, UV-visible diffuse reflectance spectroscopy, and X-ray photoelectron spectroscopy analysis. Congo red (CR), rhodamine B (RhB), and tetracycline (TC) are selected as pollutants to assess the catalytic performance of CD-TNs. As expected, the removal efficiencies of CD-TNs for CR, RhB, and TC are 94.6% (120 min), 97.2% (150 min), and 96.1% (60 min), respectively, obviously higher than that of pure TNs. The enhanced degradation efficiency of CD-TNs is predominantly ascribed to the merits of CDs (excellent up-conversion property and electron transfer property). Moreover, according to the several degradation cycles, CD-TNs possess the excellent stability, having removed 93.3% of CR after 120 min irradiation.
Introduction
Increasing awareness of aquatic contamination and environment crisis have spurred explosive research on solar energy conversion and utilization (Li et al., 2017a; Hu et al., 2018; Kayaalp et al., 2019). Wastewater is mostly generated from the spillage of a broad range of organic contaminants, which are extensively present in industrial, agricultural, and household applications (Zhang et al., 2017a; Wang et al., 2017; Shen et al., 2021). Organic reagents, closely linked with industry, are typical by-product of the rapid development of human society (Basith et al., 2018; Sordello et al., 2018). Not only effective contamination degradation but also appropriate applications of the purified water should benefit to the remediation of wastewater. However, due to their complex chemical structure, it is difficult to damage these pollutants, which are intentionally chosen to resist sunlight and oxidation by microorganisms (Zhang et al., 2017a; Saitow et al., 2018). For this reason, removal and lowering of toxicity of pollution effluents from wastewater is impending (Fan et al., 2015; Gao et al., 2018).
Organic pollutants removal through photocatalytic technology has spurred intense research in recent years, since it offers an environmentally friendly route to transfer solar energy into chemical energy for reducing the excessive pollution (Li et al., 2018a; Lu et al., 2018; Zewu et al., 2018; Liu et al., 2019a). The common photocatalyst is TiO2 for its fascinating features and relatively high photocatalytic capacity (Liu et al., 2019b). A bare TiO2, howbeit, is excited only under UV light, which attributes to its large bandgap (about 3.2 eV) (Zhang et al., 2016a). Thus, enormous efforts have been devoted to exploring the reaction processes and developing methods to improve the photocatalytic activity and efficiency of TiO2 (Liu et al., 2019b; Lee et al., 2019). As expected, huge breakthroughs have emerged in the process of preparation, functionalization, and modification of TiO2-based photocatalysts to enhance the utilization of solar spectrum (∼50% of solar light) for practical applications (Collado et al., 2018; She et al., 2019). In general, modifying TiO2 with appropriate dopant not only suppresses the photo-induced e+-h- recombination rate but also increases more visible light capacity that is absent with pure TiO2. Various strategies have been investigated to advance activities and applications of TiO2, including elements doping (Han et al., 2019), surface modification (Fu et al., 2017), semiconductor heterojunction (Zhen-Dong et al., 2018), and so on (Park et al., 2019).
Carbon materials are impressive candidates due to their striking optical and superior electron-transfer properties (Wang et al., 2016), including carbon dots (CDs; Zhang et al., 2016b; Chen et al., 2017; Sang et al., 2017), carbon nanotube (Li et al., 2017b), graphene (Xia et al., 2019; Xin et al., 2019), and graphene quantum dots (Zhi et al., 2019). Among them, carbon dots with size below 10 nm possess unique merits and have attracted widespread interests to improve the photocatalytic performance of catalysts (Cheng et al., 2016; Zhang et al., 2017b). The introduction of CDs in photocatalysts can extend the absorbance of sunlight and prompt electron transfer and decomposition of photoinduced electron–hole pairs, hence enhance the photocatalytic property of catalysts (Li and Zhang, 2018).
In this study, CD-TN photocatalysts were synthesized via the hydrothermal method and were applied to remove the three different pollutants (CR, RhB, and TC) under visible light irradiation. The morphology structures, chemical states, and optical properties of the prepared photocatalysts were investigated in detail. The photocatalytic performance of the as-prepared photocatalysts was tested by the decomposition of CR, RhB, and TC, which are toxic to human health under visible light irradiation. Additionally, the stability of CD-TN photocatalysts was also evaluated by five successive cycles.
Experimental
Chemicals
Tetrabutyl titanate (TBOT), citric acid (CA), CR, and TC were purchased from Aladdin Reagent Co., Ltd. (China). Hydrofluoric acid (HF), sodium hydroxide (NaOH), and RhB were provided by Sinopharm Chemical Reagent Co., Ltd. (China). Ammonium hydroxide (NH4OH) was purchased from J&K Scientific Ltd. Deionized (DI) water used in the experiments was obtained using an ULUPURE pure water/water system.
Synthesis of TiO2 Nanosheets
Initially, 3 ml of HF was mixed with 25 ml of TBOT and stirred for 30 min. Thereafter, the mixture was transferred to a Teflon-lined autoclave and maintained at 180°C for 24 h. After that, the obtained powders were filtered and washed with 0.1 M NaOH solution and DI water to remove the unreacted F−. The white products were dried at 60°C for 12 h under vacuum.
Synthesis of CDs
1.051 g of CA was dissolved into 25 ml of DI water. NH4OH was added into the above solution dropwise under stirring to adjust the pH value to 6. Then, the mixture was placed into a Teflon-lined autoclave and maintained for 4 h under 160°C. Then, the solution was filtrated by centrifugation and purified by dialysis (Mw = 3,500). Afterward, the CD solution was collected and freeze-dried for further use.
Synthesis of Carbon Dot/TiO2 Nanosheets
First, 1.051 g of citric acid was added into 25 ml of DI water to form a uniform solution. The pH value of solution was adjusted to six by NH4OH. Next, 0.4 g of TiO2 was added and sonicated for 1 h. The obtained suspension was transferred into a Teflon-lined autoclave and maintained at 160°C for 4 h. The precipitates were collected, followed by washing with 0.1 M of NaOH and DI water. The final products were dried in an oven at 60°C. Following the same process, photocatalysts prepared with different amount of CA (0.1, 0.15, 0.2, 0.25, and 0.3 M) were denoted as 0.1CD-TNs, 0.15CD-TNs, 0.2CD-TNs, 0.25CD-TNs, and 0.3CD-TNs, respectively.
Characterization
X-ray diffraction (XRD) carves of samples were recorded using X-ray diffraction (XRD, Bruker AXS) with Cu Kα radiation. Fourier transform infrared spectrum (FTIR) results were characterized by a Thermo Nicolet IS10 spectrometer scanning from 4,000 to 500 cm−1. The microstructure of prepared samples was observed by transmission electron microscopy (TEM) and high-resolution transmission electron microscopy (HR-TEM) using a JEM-2010, JEOL instrument. UV-vis diffuse reflectance spectra (DRS) patterns were performed on a UV-3600 (Agilent, Cary300) instrument with BaSO4 as a reference. Photoluminescence (PL) spectra of liquid samples were performed by using an F-2500 spectrophotometer (Hitachi). X-ray photoelectron spectroscopy (XPS) data was achieved on a Thermo ESCALAB 250XI device. The degraded intermediates and final products of TC were detected via LC-MS (Waters, United States) using a BEH C18 column (1.7 um, 2.1*150 mm).
Electrochemical impedance spectroscopy (EIS) measurements were performed with a CHI-760 E electrochemical workstation (Shanghai Chenhua Instrument Co., Ltd, China), with a frequency range from 100 kHz to 0.1 Hz and an amplitude of the modulation signal was 5 mV. Photocurrent tests were measured using a conventional three-electrode system with 0.5 M of Na2SO4 as the electrolyte solution, Ag/AgCl as the reference electrode, and Pt wire as the counter electrode. The working electrode was prepared by spreading a slurry of the as-prepared photocatalyst onto the fluorine-doped tin oxide (FTO) glass.
For this study, congo red (CR), rhodamine B (RhB), and tetracycline (TC) were selected as water-soluble organic pollutants for photocatalytic degradation experiments. Visible light irradiation was provided by a 500 W (XO-CHX-ID) Xe lamp with a cutoff filter (λ≥ 420 nm) and the light intensity in the reactant center was around 25.6 mW/cm2. In a typical degradation procedure, 40 mg of catalyst was added into 40 ml of aqueous pollutants solution (CR, 120 mg L−1; RhB, 20 mg L−1; or TC, 80 mg L−1), and the solution was stirred for 30 min to acquire adsorption-desorption equilibrium. A small amount of the degraded solution was taken out at fixed intervals and centrifuged to remove the catalyst, then the UV-vis measurements were recorded for the supernatant.
Results and Discussion
The typical TEM images of prepared TNs, CDs, and 0.2CD-TNs are shown in Figure 1. As shown in Figures 1A,B, TNs present a rectangular sheet-like structure and a lattice fringe of crystal, which can possess a large specific surface area. The TEM image of CDs (Figure 1C) displays a quasi-spherical morphology. Figure 1D shows that CDs disperse on the surface of TNs for 0.2CD-TNs, suggesting the coupling of CDs on TNs.
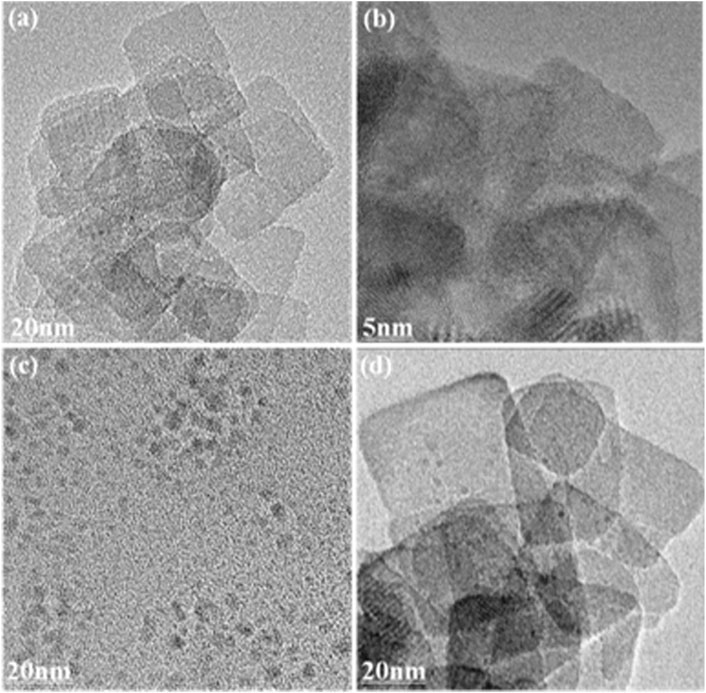
FIGURE 1. (A) TEM image of TNs; (B) enlarged TEM image of (A); TEM images of CDs (C); and 0.2CD-TNs (D).
The light-harvesting capacity of photocatalyst is a vital factor for its photocatalytic performance (Shi et al., 2018; Valenti et al., 2016). To investigate the role of CD loading on the light absorption of TNs, the UV-vis diffuse reflectance spectra (DRS) of as-prepared samples are compared (Figure 2). For pure TNs, an absorption edge at about 400 nm is observed, exhibiting the bandgap of TNs is 3.2 eV, which is in accordance with previous reports (Li et al., 2018b). Compared to TNs, there is no obvious difference in the UV absorption after CD introduction. However, CD-TN photocatalysts reveal an enhanced visible light absorption with the increasing CD content, which directly confirms that the doping of CDs on TNs improves the visible light–harvesting capacity.
The crystal phase of synthesized samples was measured by X-ray diffraction (XRD) (Figure 3). The spectrum of CDs shows an obvious broad peak at around 29.7°, which is contributed to (200) planes of graphitic carbon (Qu et al., 2016). All the characteristic peaks in TNs and CD-TN photocatalysts are identical to the crystal planes of anatase TiO2 (PDF No.00–021-1272). Notably, no characteristic peak for CDs is detected in CD-TN photocatalysts with the amount of CDs from 0.1 to 0.3, which may ascribe to the relatively limited CD loading and low diffraction intensity in the composites (Shi et al., 2017). The peaks of CD-TNs with different CDs are consistent with TNs without any change, indicating that there is no obvious influence on the structure of TNs by loading CDs.
The X-ray photoelectron spectroscopy (XPS) technique was conducted for CD-TNs to delve the valence band and the related elements, the results are illustrated in Figure 4. A survey scan (Figure 4A) depicts that the prepared CD-TN photocatalysts contain C, O, N, and Ti elements. Figure 4B shows the C1s spectrum, where the energies binding at 283.5, 284.9, 287.3, and 288.2 eV are attributed to C-Ti, C-C&C=C, C-O, and C=O, indicating the coexistence of CDs and TNs in the CD-TN catalysts. The Ti 2p spectrum shown in Figure 4C is deconvoluted into two signals: the bind energy observed at 457.4 eV is ascribed to Ti 2p1/2 and the peak at 463.1 eV comes from Ti 2p3/2. The O1s spectrum (Figure 4D) divided into three peaks at 528.6, 529.6, and 531.6 eV are corresponding to Ti-O, C-O, H-O, suggesting chemical interaction occurs between the two components.
Meanwhile, based on the Tauc plot equation, the bandgap energy of the 0.2CD-TNs is estimated. As illustrated in Figure 5, the bandgap energy of 0.2CD-TNs is 1.89 eV, implying that prepared 0.2CD-TN photocatalysts can generate active species for degrading pollutants under visible light irradiation in theory.
The FTIR spectra of samples are shown in Figure 6. Three peaks emerged at 3,426, 1,633, and 1,383 cm−1 in all samples correspond to the stretching of O-H, C=C, and C=O, signifying the good hydrophilicity of CDs (Que et al., 2017). The peaks at 3,218 and 1,406 cm−1 in the FTIR spectrum of CDs are assigned to N-H and C-N = bonds, suggesting the presence of carboxylic and amide groups on the surface of CDs. In the FTIR spectrum of 0.2CD-TNs, a stronger peak centered at 1,383 cm−1 can be ascribed to the C=O group, and a new peak emerged at 1,257 cm−1 is assigned to the C-O-C bond. Furthermore, the characteristic peaks of CDs and TNs are detected, demonstrating that CDs are successfully introduced onto TNs.
The photoresponse property of CDs is shown in Figure 7. When the excitation wavelength is 350 nm, the emission peak is located at about 440 nm. Significantly, CDs display bright fluorescence under UV-light irradiation, suggesting CDs possess the up-conversion property. CDs with the up-conversion property can absorb visible light and emit shorter wavelengths, which help CD-TNs utilize solar energy more efficiently.
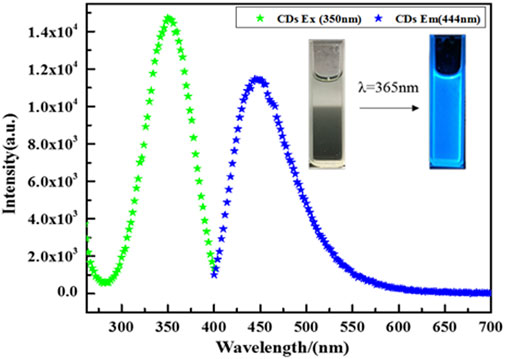
FIGURE 7. Upconverted PL spectra of CDs with excitation wavelength of 350 nm; inset: fluorescent picture of CDs under UV-light irradiation.
For photocatalyst activity, the separation and transfer of photo-induced electron–hole in photocatalyst is considerably significant (Zhang et al., 2017c). Transient photocurrent and EIS are typical measurements used to delve the electron–hole excitation effect. As illustrated in Figure 8A, the arc radius of 0.2CD-TNs is narrower than that of TNs, indicating lower charge transfer resistance for 0.2CD-TN photocatalysts, which accelerates the charge transport at the interface of 0.2CD-TNs. Moreover, a uniform and increased photocurrent can be captured under light (Figure 8B), which verifies that CDs can effectively promote the charge transfer and migration, demonstrating a higher capacity in photocatalytic degradation of the CD-TN photocatalysts than that of TNs.
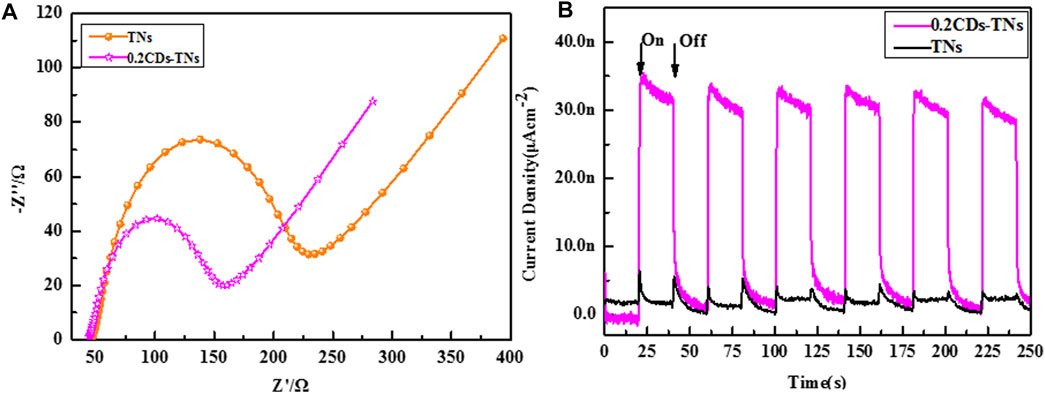
FIGURE 8. EIS curves (A) and transient photocurrent density (B) of TNs and 0.2CD-TNs under visible light irradiation.
The photocatalytic performance of prepared samples was tested via degradation of CR, RhB, and TC under simulated visible light irradiation (Figures 9A–C). It can be seen that in the presence of TNs, the concentration of CR, RhB, and TC changes negligibly under visible light. In contrast, CR, RhB, and TC are degraded remarkably in the same irradiation time in the presence of CD-TN photocatalysts. Additionally, the results reveal that the photocatalytic activity of CD-TNs greatly depends on the CD loading. The removal rate of CR is increased from 7.3 to 94.6% with the amount of CDs from 0 to 0.2 M. However, further increasing the amount of CDs from 0.2 to 0.3 M decreases the rate from 94.6 to 25.3%. Figures 9B,C depict the effect of CD-TNs on the removal of RhB and TC. The RhB degradation efficiency is measured to be around 97.2% under 150 min of irradiation in the presence of 0.2CD-TNs. Similarly, the TC degradation efficiency is achieved to be 96.1% for 0.2CD-TNs after 60 min of visible light irradiation. The experimental results also revealed that TC degrades fast because of the unstable fragments of TC (Supplementary Table S1, Supporting information). Among CD-TN catalysts, 0.2CD-TNs have the highest degradation efficiency, which probably contributes to that the high CD contents which will decrease catalytic active sites and enhance the charge carriers’ recombination rate (Giovannetti et al., 2015; Xie et al., 2018).
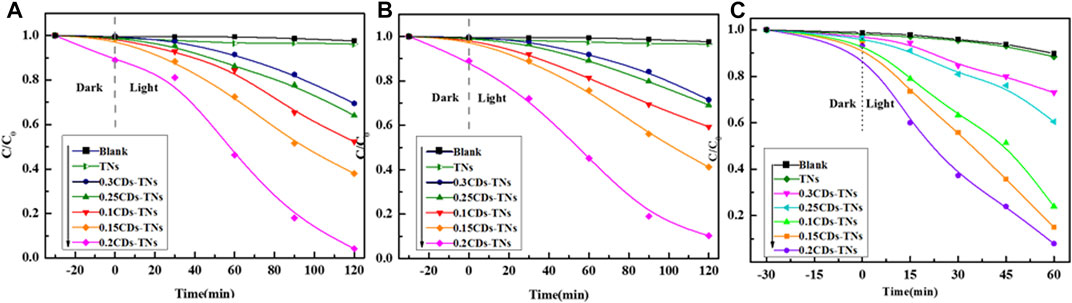
FIGURE 9. Photodegradation of (A) Congo red, 120 ppm, (B) RhB, 20 ppm, and (C) TC, 80 ppm by CD-TN composites with different contents of CDs under visible light.
The kinetic fitting plots of ln (C/C0) against reaction time t for all photocatalysts are shown in Figures 10A–C. The results show that the degradation reactions of CR, RhB, and TC by CD-TN photocatalysts fit to pseudo first order kinetics. The formula can be expressed as follows:
where k is the kinetic rate constant, C0 is the initial concentration of pollutants, and Ct is the concentration of pollutants at different degradation time t. The parameters of photocatalytic degradation of CR, RhB, and TC by different photocatalysts are summarized in Table 1. For CR degradation, the degradation activity of 0.2CD-TN photocatalysts with k = 0.01409 min−1 displays 26.09 times higher than that of pure TNs. Furthermore, the k value of 0.2CD-TNs is much larger than other CD-TN photocatalysts under visible light, illustrating its outstanding capacity in pollutants removal. Moreover, similar trend can be observed for RhB and TC, and the degradation capacity for the three pollutant follows the sequence of 0.2CD-TNs >0.1 CD-TNs >0.15CD-TNs >0.25CD-TNs >0.3CD-TNs. It is apparent that 0.2CD-TNs show the highest reaction rate constants of 0.01542 and 0.02546 min−1 for RhB and TC, respectively.
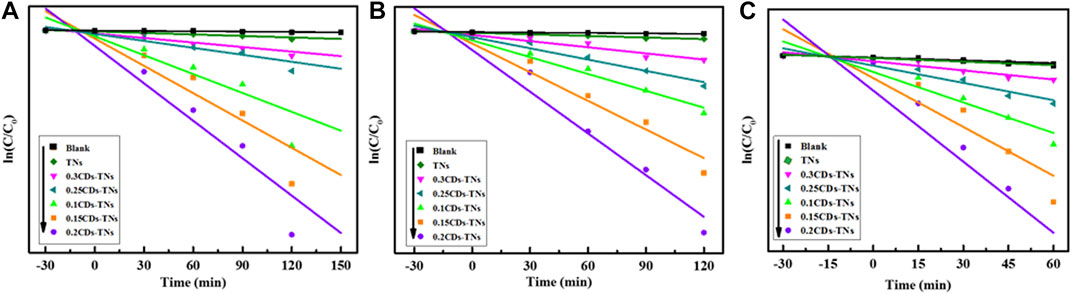
FIGURE 10. The degradation kinetics curves of (A) CR, (B) RhB, and (C) TC by CD-TN composites with different contents of CDs under visible light.
Besides, the BET surface area and total pore volume of 0.2CD-TNs are summarized. As shown in Table 2, the BET specific surface area and total pore volume of 0.2CD-TNs are 129.6286 m2/g and 0.244819 cm3/g, respectively, which can be attributed to the large surface area of TNs.
The prospective applicability of CD-TNs for the practical stability was measured by degrading CR on the basis of the recycling experiments. As shown in Figure 11, after five recycling measurements, the CR degradation rate still remains at about 93.3%, and the photocatalytic activity of 0.2CD-TNs has no loss during the degradation process, indicating that the 0.2CD-TNs exhibit an excellent structural stability. Moreover, the XRD patterns of samples after reaction are shown in Supplementary Figure S1. It can be seen that there are no noticeable changes in the crystal structure for CD-TNs before and after degrading, which further verifies the robust stability of CD-TNs.
In Table 3, the photocatalytic performance of CD-TNs is compared with other catalysts reported previously. Obviously, taking into account the different experimental parameters, CD-TNs exhibit outstanding photodegradation activity among these catalysts.
Conclusion
Practical photocatalysts (CD-TNs) were synthesized via the hydrothermal method. The results show that all the CD-TN photocatalysts exhibit higher photocatalytic efficiency for pollutants degradation under visible light irradiation than that of TNs. For these samples, 0.2CD-TN photocatalysts present the highest reaction rate and more than 90% pollutants are removed after visible light irradiation. This excellent photocatalytic activity in CD-TN photocatalysts can be contributed to the merits of CDs that not only extend the light absorption but also impede the recombination and promote the charge transfer. Consequently, the results demonstrate that the CD-TN photocatalytic process is a promising technology for wastewater remediation and purification.
Data Availability Statement
The original contributions presented in the study are included in the article/Supplementary Material; further inquiries can be directed to the corresponding authors.
Author Contributions
SS: conceptualization, investigation, data curation, and writing—original draft. RL, HW: review and editing. JF: supervision, writing—review and editing, and funding acquisition.
Funding
This work was financially supported by the National Natural Science Foundation of China (No. 31470509); the China Postdoctoral Science Foundation (No. 2019T120390); and Jiangsu Planned Projects for Postdoctoral research funds (No. 2018K018A).
Conflict of Interest
The authors declare that the research was conducted in the absence of any commercial or financial relationships that could be construed as a potential conflict of interest.
Publisher’s Note
All claims expressed in this article are solely those of the authors and do not necessarily represent those of their affiliated organizations, or those of the publisher, the editors, and the reviewers. Any product that may be evaluated in this article, or claim that may be made by its manufacturer, is not guaranteed or endorsed by the publisher.
Acknowledgments
The authors would like to thank the Shiyanjia lab for their professional tests support.
Supplementary Material
The Supplementary Material for this article can be found online at: https://www.frontiersin.org/articles/10.3389/fchem.2021.706343/full#supplementary-material
References
Basith, M. A., Ragib, A., Ishrat, Z., and Jalil, M. A. (2018). Enhanced Photocatalytic dye degradation and hydrogen Production ability of Bi25FeO40-rGO nanocomposite and mechanism insight. Scientific Rep. 8, 11090. doi:10.1038/s41598-018-29402-w
Chen, P., Wang, F., Chen, Z. F., Zhang, Q., Su, Y., Shen, L., et al. (2017). Study on the Photocatalytic mechanism and detoxicity of gemfibrozil by a sunlight-driven TiO2/carbon dots Photocatalyst: The significant roles of reactive oxygen species. Appl. Catal. B Environ. 204, 250–259.
Cheng, C., Lu, D., Shen, B., Liu, Y., Lei, J., Wang, L., et al. (2016). Mesoporous silica-based carbon dot/TiO 2 Photocatalyst for efficient organic Pollutant degradation. Microporous Mesoporous Mater. 226, 79–87. doi:10.1016/j.micromeso.2015.12.043
Collado, L., Reynal, A., Fresno, F., Barawi, M., Escudero, C., Perez-Dieste, V., et al. (2018). Unravelling the effect of charge dynamics at the Plasmonic metal/semiconductor interface for CO2 Photoreduction. Nat. Commun. 9 (1), 1–10. doi:10.1038/s41467-018-07397-2
Fan, Q., Huang, Y., Zhang, C., Liu, J., Piao, L., Yu, Y., et al. (2015). Superior nanoporous graphitic carbon nitride photocatalyst coupled with CdS quantum dots for photodegradation of RhB. Catalysis Today 264, 250–256.
Fu, J., Zhu, B., Jiang, C., Cheng, B., and Yu, J. (2017). Hierarchical Porous O doped gC3N4 with Enhanced Photocatalytic CO2 Reduction Activity. Small 13 (15), 1603938. doi:10.1002/smll.201603938
Gao, H., Yang, H., Xu, J., Zhang, S., and Li, J. (2018). Strongly Coupled g-C3N4 Nanosheets-Co3O4 Quantum Dots as 2D/0D Heterostructure Composite for Peroxymonosulfate Activation. Small 14 (31), 1801353. doi:10.1002/smll.201801353
Giovannetti, R., Amato, C. A. D., Zannotti, M., Rommozzi, E., Gunnella, R., Minicucci, M., et al. (2015). Visible light Photoactivity of Polypropylene coated Nano-TiO2 for dyes degradation in water. Scientific Rep. 5, 17801. doi:10.1038/srep17801
Han, Y., Yue, D., Kan, M., Wu, Y., Zeng, J., Bian, Z., et al. (2019). [Mo3S13]2− modified TiO2 coating on non-woven fabric for efficient Photocatalytic mineralization of acetone. Appl. Catal. B: Environ. 245, 190–196. doi:10.1016/j.apcatb.2018.12.060
Hu, J., Zhang, P., An, W., Liu, L., and Cui, W. (2018). In-situ Fe-doped g-C3N4 heterogeneous catalyst via Photocatalysis-Fenton reaction with enriched Photocatalytic Performance for removal of complex wastewater. Appl. Catal. B: Environ., 245.
Kayaalp, B., Lee, S., Klauke, K., Seo, J., Nodari, L., Kornowski, A., et al. (2019). Template-free mesoporous La0.3Sr0.7FexTi1-xO3±δ with superior oxidation catalysis Performance. Appl. Catal. B: Environ. 245, 536–545.
Lee, D., Kim, Y., Kwon, Y., Lee, J., Kim, T.-W., Noh, Y., et al. (2019). Boosting the electrocatalytic glycerol oxidation Performance with highly-dispersed Pt nanoclusters loaded on 3D graphene-like microporous carbon. Appl. Catal. B: Environ. 245, 555–568. doi:10.1016/j.apcatb.2019.01.022
Li, H., Duan, L., Shen, H., Zhang, Y., and Zhao, X. (2017). In situ synthesis and enhanced visible light Photocatalytic activity of C-TiO2 microspheres/carbon quantum dots. Ceramics Int. 43 (12), 8648–8654.
Li, J., Ma, Y., Ye, Z., Zhou, M., Wang, H., Ma, C., et al. (2017). Fast electron transfer and enhanced visible light Photocatalytic activity using multi-dimensional components of carbon quantum dots@3D daisy-like In2S3/single-wall carbon nanotubes. Appl. Catal. B Environ.
Li, K., and Zhang, W.-D. (2018). Creating Graphitic Carbon Nitride Based Donor-π-Acceptor-π-Donor Structured Catalysts for Highly Photocatalytic Hydrogen Evolution. Small 14, 1703599. doi:10.1002/smll.201703599
Li, M., Wang, M., Zhu, L., Li, Y., Yan, Z., Shen, Z., et al. (2018). Facile microwave assisted synthesis of N-rich carbon quantum dots/dual-phase TiO2 heterostructured nanocomposites with high activity in CO2 Photoreduction. Appl. Catal. B: Environ. 231, 269–276. doi:10.1016/j.apcatb.2018.03.027
Li, Z., Zhi, Y., Shao, P., Xia, H., Li, G., Feng, X., et al. (2018). Covalent organic framework as an efficient, metal-free, heterogeneous Photocatalyst for organic transformations under visible light. Appl. Catal. B: Environ. 245, 334–342.
Liu, D., Chen, D., Li, N., and Xu, Q. (2019). ZIF-67-Derived 3D Hollow Mesoporous Crystalline Co3O4 Wrapped by 2D g-C3N4 Nanosheets for Photocatalytic Removal of Nitric Oxide. Small 15, e1902291.
Liu, Y., Wygant, B. R., Kawashima, K., Mabayoje, O., Hong, T. E., Lee, S.-G., et al. (2019). Facet effect on the Photoelectrochemical Performance of a WO3/BiVO4 heterojunction Photoanode. Appl. Catal. B: Environ. 245, 227–239. doi:10.1016/j.apcatb.2018.12.058
Lu, Y., Yin, W. J., Peng, K. L., Wang, K., Hu, Q., Selloni, A., et al. (2018). Self-hydrogenated shell Promoting Photocatalytic H2 evolution on anatase TiO2. Nat. Commun. 9, 2752. doi:10.1038/s41467-018-05144-1
Park, S. J., Das, G. S., Schütt, F., Adelung, R., Mishra, Y. K., Tripathi, K. M., et al. (2019). Visible-light Photocatalysis by carbon-nano-onion-functionalized ZnO tetrapods: degradation of 2,4-dinitrophenol and a Plant-model-based ecological assessment. Npg Asia Mater. 11 (1), 1–13. doi:10.1038/s41427-019-0107-0
Qu, A., Xie, H., Xu, X., Zhang, Y., Wen, S., and Cui, Y. (2016). High quantum yield graphene quantum dots decorated TiO2 nanotubes for enhancing Photocatalytic activity. Appl. Surf. ScienceApplied Surf. Sci. 375, 230–241. doi:10.1016/j.apsusc.2016.03.077
Que, Q., Xing, Y., He, Z., Yang, Y., Yin, X., and Que, W. (2017). Bi2O3/Carbon quantum dots heterostructured Photocatalysts with enhanced Photocatalytic activity. Mater. Lett. 209, 220–223. doi:10.1016/j.matlet.2017.07.115
Saitow, K. I., Wang, Y., and Takahashi, S. (2018). Mechano-synthesized orange TiO2 shows significant Photocatalysis under visible light. Scientific Rep. 8 (1), 1–10. doi:10.1038/s41598-018-33772-6
Sang, L., Lin, J., and Zhao, Y. (2017). Preparation of carbon dots/TiO 2 electrodes and their Photoelectrochemical activities for water splitting. Int. J. Hydrogen Energ. 42, 12122–12132. doi:10.1016/j.ijhydene.2017.01.228
She, X., Xu, H., Yu, Y., Li, L., Zhu, X., Mo, Z., et al. (2019). Accelerating Photogenerated Charge Kinetics via the Synergetic Utilization of 2D Semiconducting Structural Advantages and Nobleetalree Schottky Junction Effect. Small 15, 1804613.
Shen, S., Fu, J. J., and Wang, H. B. (2021). Unravelling the favorable Photocatalytic effect of hydrogenation Process on the novel g-C3N4-TiO2 catalysts for water Purification. Diamond Relat. Mater. 114, 108292. doi:10.1016/j.diamond.2021.108292
Shi, W., Guo, F., Wang, H., Han, M., Li, H., Yuan, S., et al. (2017). Carbon dots decorated the exposing high-reactive (111) facets CoO octahedrons with enhanced Photocatalytic activity and stability for tetracycline degradation under visible light irradiation. Appl. Catal. B: Environ. 219, 36–44. doi:10.1016/j.apcatb.2017.07.019
Shi, X., Fujitsuka, M., Kim, S., and Majima, T. (2018). Faster Electron Injection and More Active Sites for Efficient Photocatalytic H2 Evolution in g-C3N4/MoS2 Hybrid. Small 14, e1703277. doi:10.1002/smll.201703277
Sordello, F., Berruti, I., Gionco, C., Paganini, M. C., and Minero, C. (2018). Photocatalytic Performances of rare earth element-doped zinc oxide toward Pollutant abatement in water and wastewater. Appl. Catal. B: Environ. 245, 159–166.
Valenti, G., Boni, A., Melchionna, M., Cargnello, M., Nasi, L., Bertoni, G., et al. (2016). Co-axial heterostructures integrating Palladium/titanium dioxide with carbon nanotubes for efficient electrocatalytic hydrogen evolution. Nat. Commun. 7, 13549. doi:10.1038/ncomms13549
Wang, J., Li, G., Li, Z., Tang, C., Feng, Z., An, H., et al. (2017). A highly selective and stable ZnO-ZrO2 solid solution catalyst for CO2 hydrogenation to methanol. Sci. Adv. 3 (10), e1701290. doi:10.1126/sciadv.1701290
Wang, N., Yiting, J., Chen, M., Guo, T., and Yang, T. (2016). Green Preparation of carbon dots with Papaya as carbon source for effective fluorescent sensing of Iron (III) and Escherichia coli. Biosens. Bioelectron. The International Journal for the Professional Involved with Research, Technology and Applications of Biosensers and Related Devices,. 85, 68–75.
Xia, Z., Fang, J., Zhang, X., Fan, L., Barlow, A. J., Lin, T., et al. (2019). Pt nanoparticles embedded metal-organic framework nanosheets: A synergistic strategy towards bifunctional oxygen electrocatalysis. Appl. Catal. B: Environ. 245, 389–398. doi:10.1016/j.apcatb.2018.12.073
Xie, Z., Feng, Y., Wang, F., and Chen, D. (2018). Construction of carbon dots modified MoO3/g-C3N4 Z-scheme Photocatalyst with enhanced visible-light Photocatalytic activity for the degradation of tetracycline. Appl. Catal. B Environ. Int. J. Devoted Catalytic Sci. Its Appl. 229, 96–104.
Xin, X., Li, S.-H., Zhang, N., Tang, Z.-R., and Xu, Y.-J. (2019). 3D graphene/AgBr/Ag cascade aerogel for efficient Photocatalytic disinfection. Appl. Catal. B: Environ. 245, 343–350. doi:10.1016/j.apcatb.2018.12.066
Zewu, Z., Yuhang, M., Xiaohai, B., Qiong, W., Zusheng, H., Zhao, D., et al. (2018). Facile one-step synthesis of TiO2/Ag/SnO2 ternary heterostructures with enhanced visible light Photocatalytic activity. Scientific Rep. 8, 10532.
Zhang, A., Chen, J., Shu, J., Jiao, , and Yan, Z. (2016). Heng, Synthesis of Carbon Quantum Dots/TiO2 Nanocomposite for Photo-Degradation of Rhodamine B and Cefradine. Diamond & Related Materials 70, 137–144.
Zhang, B., Maimaiti, H., Zhang, D.-D., Xu, B., and Wei, M. (2017a). Preparation of coal-based C-Dots/TiO2 and its visible-light Photocatalytic characteristics for degradation of Pulping black liquor. J. Photochem. Photobiol. A: Chem. 345, 54–62. doi:10.1016/j.jphotochem.2017.05.031
Zhang, G., Wu, Z., Liu, H., Ji, Q., Qu, J., and Jinghong, L. (2017b). Photoactuation Healing of α-FeOOH@g-C3N4 Catalyst for Efficient and Stable Activation of Persulfate. Small. 13, 1702225.
Zhang, Q., Zhang, Y., Meng, Z., Tong, W., Yu, X., and An, Q. (2017c). Constructing the magnetic bifunctional graphene/titania nanosheet-based composite Photocatalysts for enhanced visible-light Photodegradation of MB and electrochemical ORR from Polluted water. Scientific Rep. 7, 12296. doi:10.1038/s41598-017-12504-2
Zhang, X., Dong, S., Zhou, X., and Dong, S. (2016). N-doped carbon quantum dots/TiO2 hybrid composites with enhanced visible light driven Photocatalytic activity toward dye wastewater degradation and mechanism insight. J. Photochem. Photobiol. A: Chem. 325, 104–115.
Zhen-Dong, L., Yuan-Cheng, X., Wen-Qian, C., Lin, L., and Wen-Hui, Q. (2018). The Influence of Carbon Nitride Nanosheets Doping on the Crystalline Formation of MIL-88B(Fe) and the Photocatalytic Activities. Small. 14, 1802045.
Keywords: carbon dots, visible light, photocatalytic degradation, congo red, stability
Citation: Shen S, Li R, Wang H and Fu J (2021) Carbon Dot–Doped Titanium Dioxide Sheets for the Efficient Photocatalytic Performance of Refractory Pollutants. Front. Chem. 9:706343. doi: 10.3389/fchem.2021.706343
Received: 07 May 2021; Accepted: 23 July 2021;
Published: 07 September 2021.
Edited by:
Svetlana Ivanova, University of Seville, SpainReviewed by:
Qizhao Wang, Chang’an University, ChinaVincenzo Vaiano, University of Salerno, Italy
Sebastián Murcia-López, Energy Research Institute of Catalonia, Spain
Copyright © 2021 Shen, Li, Wang and Fu. This is an open-access article distributed under the terms of the Creative Commons Attribution License (CC BY). The use, distribution or reproduction in other forums is permitted, provided the original author(s) and the copyright owner(s) are credited and that the original publication in this journal is cited, in accordance with accepted academic practice. No use, distribution or reproduction is permitted which does not comply with these terms.
*Correspondence: Hongbo Wang, d3h3YW5naGJAMTYzLmNvbQ==; Jiajia Fu, a2F0aHlmampAMTI2LmNvbQ==