- 1Department of Nanopharmaceutical Sciences, Nagoya Institute of Technology, Nagoya, Japan
- 2Institute of Advanced Fluorine-Containing Materials, Zhejiang Normal University, Jinhua, China
The defluorosilylation of aryl fluorides to access aryl silanes was achieved under transition-metal-free conditions via an inert C–F bond activation. The defluorosilylation, mediated by silylboronates and KOtBu, proceeded smoothly at room temperature to afford various aryl silanes in good yields. Although a comparative experiment indicated that Ni catalyst facilitated this transformation more efficiently, the transition-metal-free protocol is advantageous from a green chemistry perspective.
Introduction
Organofluorine compounds have been critical over the past few decades in pharmaceutical (Inoue et al., 2020), agrochemical (Ogawa et al., 2020), functional materials (Hiyama, 2000; Babudri et al., 2007; Berger et al., 2011; Liu et al., 2017; Liu et al., 2019) and polymer (Améduri et al., 2020) industries. The progress of synthetic technologies exemplified by fluorination (Rozen et al., 1996; Shibata et al., 2007; Furuya et al., 2011; Campbell et al., 2015; Ni et al., 2015; Lee et al., 2016; Zhu et al., 2018) and trifluoromethylation (Ma et al., 2004; Shibata et al., 2008; Shibata et al., 2010; Merino et al., 2014; Liu et al., 2015; Charpentier et al., 2015; Alonso et al., 2015; Xiao et al., 2021) reactions has expressively supported such success and prosperity of organofluorine compounds. One of the most attractive properties of organofluorine compounds is their durability, represented by Teflon®, induced by the most vital bond energy of the C–F bond in carbon chemistry (Uneyama et al., 2006; Luo et al., 2007; Amii et al., 2009). However, their robustness has often caused severe persistent environmental toxicity, such as the super-greenhouse effect by fluorocarbons (McCulloch et al., 2003; Velders et al., 2007; Shine and Sturges, 2007; Sovacool et al., 2021) and the bioaccumulation of perfluorooctanoic acid (PFOA) and perfluorooctanesulfonic acid (PFOS) (Vierke et al., 2012; Stanifer et al., 2018; Chen et al., 2019; Li et al., 2020). Given this limitation, recent attention has been focused on the activation and cleavage of remarkably inert C–F bonds of organofluorine molecules, creating a new field of research in fluorine chemistry (Stahl et al., 2013; Ahrens et al., 2015; Shen et al., 2015; Eisenstein et al., 2017; Hamel et al., 2018).
In 2018, we reported a significant achievement on the C–F bond cleavage of aryl fluorides via defluorosilylation using silylboronates (R3SiBPin) in the presence of potassium tert-butoxide (KOtBu) and a catalytic amount of Ni. The C–F bond cleavage occurred via the five-centered transition state via a π-nickel complex and a non-classical oxidative pathway (Scheme 1A); (Cui et al., 2018). Notably, we also found that the C–F bond activation did not require an Ni catalyst in the case of alkyl fluorides. The defluorosilylation of alkyl fluorides proceeded smoothly with R3SiBPin exclusively in the presence of KOtBu. A highly nucleophilic, silyl anionic species directly reacts with alkyl fluorides via a concerted SN2 process (Scheme 1B). The defluorosilylation reaction was then successfully reported by several groups (Gao et al., 2019; Liu et al., 2019; Kojima et al., 2019; Mallick et al., 2019; Coates et al., 2019; Lim et al., 2020; Sheldon et al., 2020). In 2019, Martin and co-workers reported the lithium-promoted defluorosilylation of organic fluorides, in which lithium bis(trimethylsilyl)amide (LiHMDS) and dimethyl ether (DME) cooperated well to activate the inert C–F bond (Scheme 1C); (Liu et al., 2019). In the same year, Uchiyama and co-workers also reported a transition-metal-free defluorosilylation of fluoroarenes using PhMe2SiBPin and sodium tert-butoxide (NaOtBu) (Kojima et al., 2019). In situ generated silyl anion species enabled the direct defluorosilylation of fluoroarenes (Scheme 1D). In 2021, we have continuously reported the catalyst-free carbosilylation of alkenes using R3SiBPin and organic fluorides, including aryl and alkyl fluorides, via selective C–F bond activation (Zhou et al., 2021). The substrate-scope showed slightly better yields when the reaction was performed in the presence of an Ni-catalyst, although we noticed that the effect of Ni-catalyst was not significant (Scheme 1E). While the results of Uchiyama and co-workers (Scheme 1D); (Kojima et al., 2019) and our recent results (Scheme 1E); (Zhou et al., 2021) indicate that Ni-catalyst is not necessary for their transformations, the conditions are not precisely the same such as bases, solvents and reaction times, which is difficult to conclude the Ni-effect. We thus decided to carefully re-examine our original work of defluorosilylation of aryl fluorides in 2018 (Scheme 1A); (Cui et al., 2018) by the same conditions, R3SiBPin in the presence of KOtBu, with or without an Ni-catalyst. We disclose herein the improved-catalyst-free conditions for silylboronate-mediated defluorosilylation of aryl fluorides. A wide variety of aryl fluorides 1 having a substitution at the aromatic ring were smoothly converted into the corresponding aryl silanes 3 in good yields by R3SiBPin 2 (2.0 equiv) in the presence of KOtBu (3.0 equiv) in a mixed solvent system (c-hex/THF = 1/2) at room temperature. Heteroaromatic fluorides 1 are also accepted by the same conditions to provide heteroaromatic silanes 3 in good yields. We also carried out the same reactions under Ni-catalysis. While the yields under the catalyst-free conditions were lower than those under Ni-catalysis, the transition-metal-free system is advantageous from the perspective of green chemistry (Scheme 1F).
Results and Discussion
To start the optimization, we selected 4-fluorobiphenyl (1a) and silylboronate Et3SiBpin (2a) as model substrates to examine the defluorosilylation reaction. Based on our earlier reported conditions of the Ni-catalyzed defluorinative silylation of aryl fluorides 1 [Et3SiBpin (1.5 equiv), KOtBu (2.5 equiv), 10 mol% Ni(cod)2 in cyclohexane (c-hex)/THF (1/2, v/v) at room temperature], we carried out the reaction of 1a with 2a under the conditions mentioned above but without Ni-catalyst. All the optimizations were carried out on a 0.1 mmol scale of 1a. The expected biphenyl-4-yl-triethylsilane (3aa) was observed in 65% 1H NMR yield after 8 h (entry 1, Table 1). To compare Uchiyama’s reaction conditions (Kojima et al., 2019) (NaOtBu, THF), replacing KOtBu with NaOtBu, gave 58% yield of 3aa (entry 2). Other bases such as LiOtBu or KOMe resulted in no reaction (entries 3 and 4). The conditions by Martin (Liu et al., 2019) (LiHMDS, DME) were also attempted but using our solvent system (c-hex/THF = 1/2, v/v), but no reaction resulted (entry 5). Interestingly, KHMDS facilitated this defluorosilylation reaction by affording 3aa in 27% yield (entry 6). We subsequently attempted the reaction in a single solvent of c-hex, THF, or diglyme to investigate the effect of solvent. The mixed solvent system, c-hex/THF (entry 1), was more effective than others (entries 7–9). We next varied the amounts of 2a and KOtBu (entries 10 and 11) and found that 2.0 equiv of 2a and 3.0 equiv of KOtBu were the optimum amounts to afford 3aa in 74% yield (56% isolated yield; entry 11). To re-ascertain the effect of Ni(COD)2, we investigated the reaction using these optimized conditions (entry 11) but in the presence of Ni catalyst. The defluorosilylation reaction performed more efficiently under the optimal conditions with Ni(COD)2 to give 3aa in 83% yield (65% isolated yield; entry 12), while 1a remained (detected by crude 19F NMR). These comparative results thus convinced us that Ni(COD)2 accelerates the present defluorinative transformation, while the transition-metal-free variant (entry 11) is advantageous from a green chemistry perspective.
With the optimized reaction conditions in hand (entry 11, Table 1), we next examined the feasibility of this transition-metal-free defluorosilylation reaction (Table 2). All the reactions were carried out on a 0.2 mmol scale of 1. As shown, various aromatic fluorides were examined under catalyst-free conditions. We efficiently converted a wide range of fluoroarenes 1 into corresponding defluorosilylation products 3 in good yield. It was found that any position (o-, m-, or p-) in the aromatic substitution of 1 was viable, affording the corresponding products 3 (3aa: 59%; 3ba: 51%; 3ca: 26%; 3da: 40%; 3ea: 55%) in acceptable to good yields (26–59%) under the catalyst-free conditions. We next repeated the same substrate scope in the presence of Ni(COD)2 (entry 12, Table 1) and the yield of products 3aa–3ea improved considerably (3aa: 86%; 3ba: 82%; 3ca: 74%; 3da: 70%; 3ea: 79%). Thus, these differences clearly show the efficiency of Ni(COD)2. Previous results with Ni(COD)2 are also indicated in Table 1 to ascertain the advantage of the Ni catalyst. Besides, the aryl fluorides 1f–1h with an electron-rich substitution were well-tolerated in this defluorosilylation reaction in moderate yield (3fa: 46%; 3ga: 45%; 3ha: 39%). Several substituted aryl silanes (3ia–3na) were also successfully obtained in moderate yield under identical conditions and a variety of functional groups such as OMe (1j), OMOM (1k), OPh (1l), NMe2 (1m) and 1H-pyrrole (1n) were well tolerated. The nitrogen-containing hetero-aromatic fluorides 1o–1q were successfully converted to the corresponding silanes 3. For example, 5-fluoro-2-phenylpyridine (1o) and 1H-indole derivatives (1p and 1q), which possess an active C–H bond, were well-tolerated and smoothly underwent the selective defluorosilylation process to afford desired products (3oa: 43%; 3pa: 37%; 3qa: 42%). Notably, 1,2-difluorobenzene (1r) was efficiently mono-silylated in good yield (3ra: 62%). Sterically demanding o-substituted substrates 1s and 1t were also transformed into the corresponding products 3sa and 3ta under Ni-free conditions in 26 and 12% yields, respectively. Ni-catalyst conditions improved both cases to 67% (3sa) and 35% (3ta). Furthermore, other silyl boronates such as PhMe2SiBpin (2b) and tBuMe2SiBPin (2c) were also investigated instead of 2a to yield the corresponding silylated products 3ab and 3ac in 36 and 51% yield, respectively. In all cases, the Ni catalyst-based protocol (Cui et al., 2018) has a substantial yield advantage in this defluorosilylation reaction, while both conditions did not entirely consume the staring materials 1. The substrates (1u and 1v) having electron-withdrawing group were not suitable, which is the limitation of this transformation.
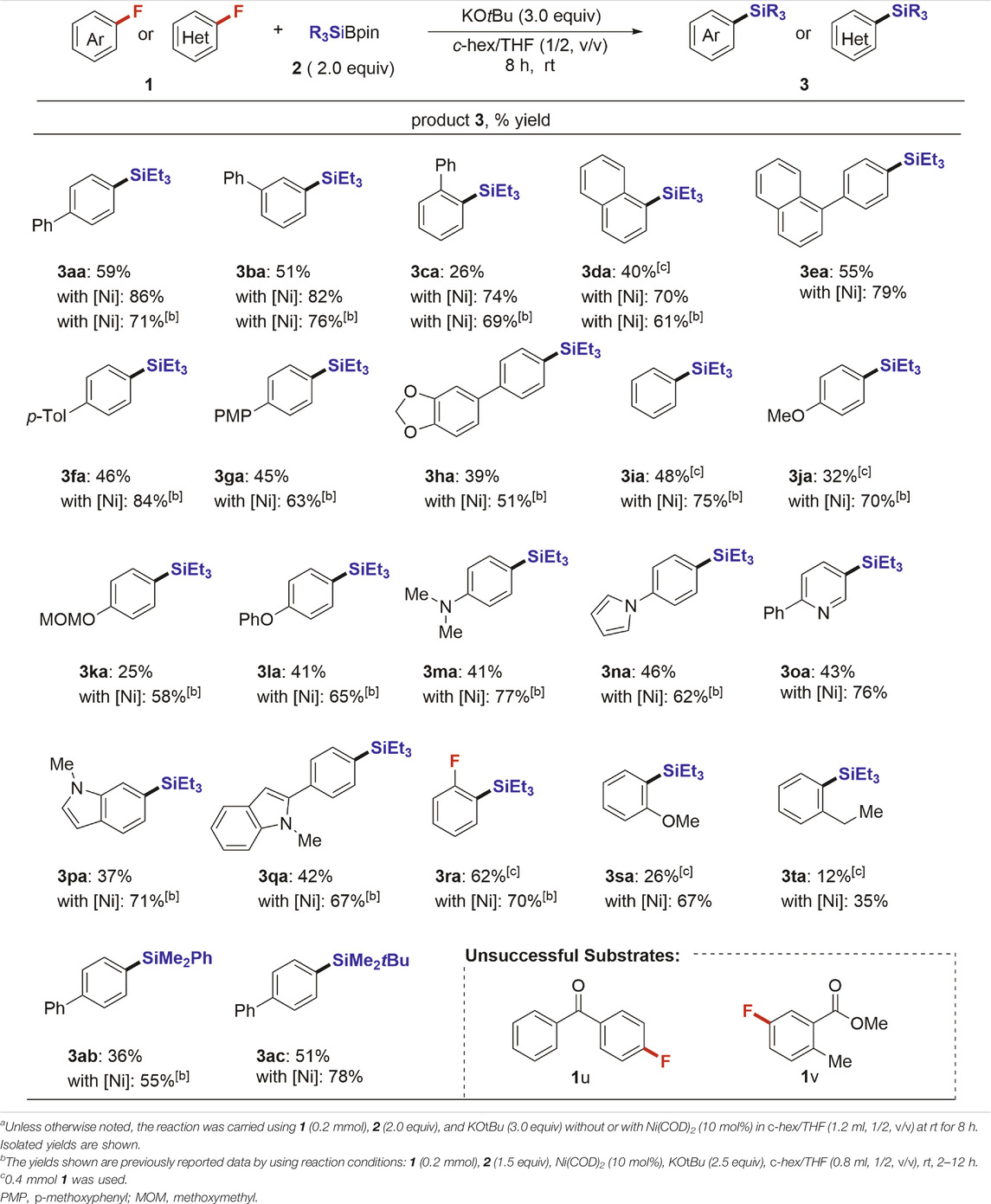
TABLE 2. Substrate scope of the defluorosilylation strategiesa.
Based on our previous work of defluorosilylation of alkyl fluorides 1 with R3SiBPin 2 mediated by a potassium base (Cui et al., 2018), the defluorosilylation of aryl fluorides mediated by a lithium base (Martin) (Liu et al., 2019) and by a sodium base (Uchiyama) (Kojima et al., 2019), the reaction should proceed the nucleophilic attack of the silyl anion involving a concerted SNAr process. A schematic reaction of the catalyst-free defluorosilylation process is presented in Scheme 2 by considering our previous work and Uchiyama’s elegant DFT calculations (Kojima et al., 2019). First, R3SiBPin 2 reacts with tBuOK to provide potassium silyl anion species C complexed with tBuO-BPin via A and B (Cui et al., 2018; Jain et al., 2018; Zhou et al., 2021). C approaches the aryl fluoride 1 to form the intermediate I. A concerted SNAr reaction happens with the attack of the boron center of tBuO-BPin by another tBuOK via a transition state II with the key C–F bond cleavage to furnish the aryl silanes 3 with the formation of KF and D, K+[tBuO2BPin]-.
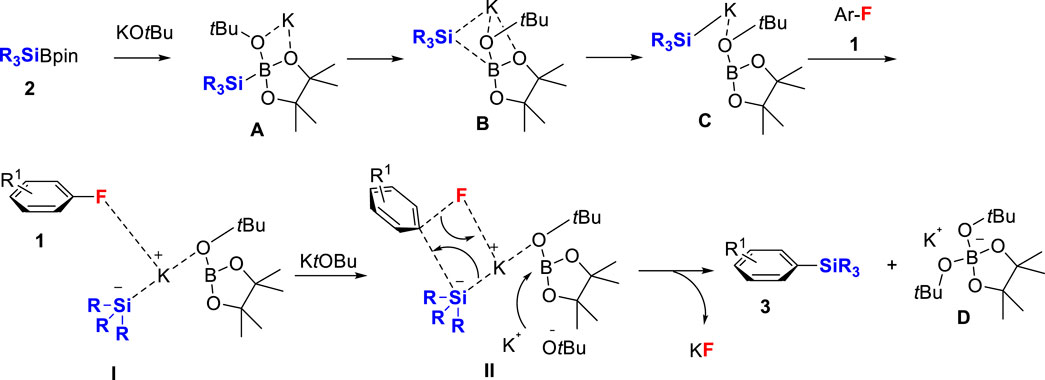
SCHEME 2. A schematic of the reaction process of catalyst-free defluorosilylation of aryl fluorides 1 with R3SiBPin 2 in the presence of tBuOK.
Conclusion
In summary, we reported a feasible transition-metal-free method for synthesizing aryl silanes 3 through the defluorosilylation of aryl fluorides 1 by using silylboronates R3SiBPin 2 and KOtBu. Furthermore, we compared our new results with a previous report on the success of Ni-catalyzed defluorosilylation of fluoroarenes. Thus, we concluded that the transformation of aryl fluorides into corresponding aryl silanes via a C−F bond cleavage can be achieved even in the absence of Ni(COD)2, but in relatively lower yields than those of the Ni-catalyzed protocol, due to different reaction mechanisms. A further extension of this methodology is currently underway.
Data Availability Statement
The original contributions presented in the study are included in the article/Supplementary Material, further inquiries can be directed to the corresponding author.
Author Contributions
NS conceived the concept. JZ and ZZ optimized the reaction conditions and surveyed the substrate scope. NS directed the project. NS and JZ prepared the manuscript.
Funding
This work was supported by JSPS KAKENHI grant 21H01933 (KIBAN B, NS).
Conflict of Interest
The authors declare that the research was conducted in the absence of any commercial or financial relationships that could be construed as a potential conflict of interest.
The reviewer SF declared a past co-authorship with one of the authors NS to the handling Editor.
Publisher’s Note
All claims expressed in this article are solely those of the authors and do not necessarily represent those of their affiliated organizations, or those of the publisher, the editors and the reviewers. Any product that may be evaluated in this article, orclaim that may be made by its manufacturer, is not guaranteed or endorsed by the publisher.
Supplementary Material
The Supplementary Material for this article can be found online at: https://www.frontiersin.org/articles/10.3389/fchem.2021.771473/full#supplementary-material
References
Ahrens, T., Kohlmann, J., Ahrens, M., and Braun, T. (2015). Functionalization of Fluorinated Molecules by Transition-Metal-Mediated C-F Bond Activation to Access Fluorinated Building Blocks. Chem. Rev. 115, 931–972. doi:10.1021/cr500257c
Alonso, C., Martínez de Marigorta, E., Rubiales, G., and Palacios, F. (2015). Carbon Trifluoromethylation Reactions of Hydrocarbon Derivatives and Heteroarenes. Chem. Rev. 115, 1847–1935. doi:10.1021/cr500368h
Améduri, B. (2020). The Promising Future of Fluoropolymers. Macromol. Chem. Phys. 221, 1900573–1900586. doi:10.1002/macp.201900573
Amii, H., and Uneyama, K. (2009). C−F Bond Activation in Organic Synthesis. Chem. Rev. 109, 2119–2183. doi:10.1021/cr800388c
Babudri, F., Farinola, G. M., Naso, F., and Ragni, R. (2007). Fluorinated Organic Materials for Electronic and Optoelectronic Applications: the Role of the Fluorine Atom. Chem. Commun. 43, 1003–1022. doi:10.1039/B611336B
Berger, R., Resnati, G., Metrangolo, P., Weber, E., and Hulliger, J. (2011). Organic Fluorine Compounds: a Great Opportunity for Enhanced Materials Properties. Chem. Soc. Rev. 40, 3496–3508. doi:10.1039/C0CS00221F
Campbell, M. G., and Ritter, T. (2015). Modern Carbon-Fluorine Bond Forming Reactions for Aryl Fluoride Synthesis. Chem. Rev. 115, 612–633. doi:10.1021/cr500366b
Charpentier, J., Früh, N., and Togni, A. (2015). Electrophilic Trifluoromethylation by Use of Hypervalent Iodine Reagents. Chem. Rev. 115, 650–682. doi:10.1021/cr500223h
Chen, H., Reinhard, M., Yin, T., Nguyen, T. V., Tran, N. H., and Yew-Hoong Gin, K. (2019). Multi-compartment Distribution of Perfluoroalkyl and Polyfluoroalkyl Substances (PFASs) in an Urban Catchment System. Water Res. 154, 227–237. doi:10.1016/j.watres.2019.02.009
Coates, G., Tan, H. Y., Kalff, C., White, A. J. P., and Crimmin, M. R. (2019). Defluorosilylation of Industrially Relevant Fluoroolefins Using Nucleophilic Silicon Reagents. Angew. Chem. Int. Ed. 58, 12514–12518. doi:10.1002/anie.201906825
Cui, B., Jia, S., TokunagaShibata, E. N., and Shibata, N. (2018). Defluorosilylation of Fluoroarenes and Fluoroalkanes. Nat. Commun. 9, 4393. doi:10.1038/s41467-018-06830-w
Eisenstein, O., Milani, J., and Perutz, R. N. (2017). Selectivity of C-H Activation and Competition between C-H and C-F Bond Activation at Fluorocarbons. Chem. Rev. 117, 8710–8753. doi:10.1021/acs.chemrev.7b00163
Furuya, T., Kamlet, A. S., and Ritter, T. (2011). Catalysis for Fluorination and Trifluoromethylation. Nature 473, 470–477. doi:10.1038/nature10108
Gao, P., Wang, G., Xi, L., Wang, M., Li, S., and Shi, Z. (2019). Transition‐Metal‐Free Defluorosilylation of Fluoroalkenes with Silylboronates. Chin. J. Chem. 37, 1009–1014. doi:10.1002/cjoc.201900310
Hamel, J.-D., and Paquin, J.-F. (2018). Activation of C-F Bonds α to C-C Multiple Bonds. Chem. Commun. 54, 10224–10239. doi:10.1039/C8CC05108A
Hiyama, T. (2000). “Biologically Active Organofluorine Compounds,” in Organofluorine Compounds. Editor H. Yamamoto (Springer-verlag Berlin Heidelberg), 137–182. doi:10.1007/978-3-662-04164-2_5
Inoue, M., Sumii, Y., and Shibata, N. (2020). Contribution of Organofluorine Compounds to Pharmaceuticals. ACS Omega 5, 10633–10640. doi:10.1021/acsomega.0c00830
Jain, P., Pal, S., and Avasare, V. (2018). Ni(COD)2-Catalyzed Ipso-Silylation of 2-Methoxynaphthalene: A Density Functional Theory Study. Organometallics 37, 1141–1149. doi:10.1021/acs.organomet.8b00046
Kojima, K., Nagashima, Y., Wang, C., and Uchiyama, M. (2019). In SituGeneration of Silyl Anion Species through Si−B Bond Activation for the Concerted Nucleophilic Aromatic Substitution of Fluoroarenes. ChemPlusChem 84, 277–280. doi:10.1002/cplu.201900069
Lee, J.-W., Oliveira, M. T., Jang, H. B., Lee, S., Chi, D. Y., Kim, D. W., et al. (2016). Hydrogen-bond Promoted Nucleophilic Fluorination: Concept, Mechanism and Applications in Positron Emission Tomography. Chem. Soc. Rev. 45, 4638–4650. doi:10.1039/C6CS00286B
Li, P., Oyang, X., Xie, X., Guo, Y., Li, Z., Xi, J., et al. (2020). Perfluorooctanoic Acid and Perfluorooctane Sulfonate Co-exposure Induced Changes of Metabolites and Defense Pathways in Lettuce Leaves. Environ. Pollut. 256, 113512. doi:10.1016/j.envpol.2019.113512
Lim, S., Cho, H., Jeong, J., Jang, M., Kim, H., Cho, S. H., et al. (2020). Cobalt-catalyzed Defluorosilylation of Aryl Fluorides via Grignard Reagent Formation. Org. Lett. 22, 7387–7392. doi:10.1021/acs.orglett.0c02752
Liu, X., Xu, C., Wang, M., and Liu, Q. (2015). Trifluoromethyltrimethylsilane: Nucleophilic Trifluoromethylation and beyond. Chem. Rev. 115, 683–730. doi:10.1021/cr400473a
Liu, Q., Ni, C., and Hu, J. (2017). China's Flourishing Synthetic Organofluorine Chemistry: Innovations in the New Millennium. Natl. Sci. Rev. 4, 303–325. doi:10.1093/nsr/nwx058
Liu, X.-W., Zarate, C., and Martin, R. (2019). Base-Mediated Defluorosilylation of C(sp2 )−F and C(sp3 )−F Bonds. Angew. Chem. Int. Ed. 58, 2064–2068. doi:10.1002/anie.201813294
Liu, Y., Jiang, L., Wang, H., Wang, H., Jiao, W., Chen, G., et al. (2019). A Brief Review for Fluorinated Carbon: Synthesis, Properties and Applications. Nanotechnol. Rev. 8, 573–586. doi:10.1515/ntrev-2019-0051
Luo, Y.-R. (2007). “BDEs of C-Halogen Bonds,” in Comprehensive Handbook of Chemical Bond Energies. Y.-R. Luo, (ed) 1st ed. (Boca Raton, FL: CRC Press), 211–253. doi:10.1201/9781420007282-5
Ma, J.-A., and Cahard, D. (2004). Asymmetric Fluorination, Trifluoromethylation, and Perfluoroalkylation Reactions. Chem. Rev. 104, 6119–6146. doi:10.1021/cr030143e
Mallick, S., Xu, P., Würthwein, E.-U., and Studer, A. (2019). Silyldefluorination of Fluoroarenes by Concerted Nucleophilic Aromatic Substitution. Angew. Chem. Int. Ed. 58, 283–287. doi:10.1002/anie.201808646
McCulloch, A. (2003) Fluorocarbons in the Global Environment: a Review of the Important Interactions with Atmospheric Chemistry and Physics. Sciencej. Fluor. Chem. 315123, 180421–180529. doi:10.1016/S0022-1139(03)00105-2
Merino, E., and Nevado, C. (2014). Addition of CF3 across Unsaturated Moieties: a Powerful Functionalization Tool. Chem. Soc. Rev. 43, 6598–6608. doi:10.1039/C4CS00025K
Ni, C., Hu, M., and Hu, J. (2015). Good Partnership between Sulfur and Fluorine: Sulfur-Based Fluorination and Fluoroalkylation Reagents for Organic Synthesis. Chem. Rev. 115, 765–825. doi:10.1021/cr5002386
Ogawa, Y., Tokunaga, E., Kobayashi, O., Hirai, K., and Shibata, N. (2020). Current Contributions of Organofluorine Compounds to the Agrochemical Industry. iScience 23, 101467–110519. doi:10.1016/j.isci.2020.101467
Shibata, N., Ishimaru, T., Nakamura, S., and Toru, T. (2007). New Approaches to Enantioselective Fluorination: Cinchona Alkaloids Combinations and Chiral Ligands/metal Complexes. J. Fluorine Chem. 128, 469–483. doi:10.1016/j.jfluchem.2006.12.014
Sheldon, D. J., CoatesCrimmin, G. M. R., and Crimmin, M. R. (2020). Defluorosilylation of Trifluoromethane: Upgrading an Environmentally Damaging Fluorocarbon. Chem. Commun. 56, 12929–12932. doi:10.1039/D0CC04592F
Shen, Q., Huang, Y.-G., Liu, C., Xiao, J.-C., Chen, Q.-Y., and Guo, Y. (2015). Review of Recent Advances in CF Bond Activation of Aliphatic Fluorides. J. Fluorine Chem. 179, 14–22. doi:10.1016/j.jfluchem.2015.07.007
Shibata, N., Ishimaru, T., Nakamura, S., and Toru, T. (2007). New Approaches to Enantioselective Fluorination: Cinchona Alkaloids Combinations and Chiral Ligands/metal Complexes. J. Fluorine Chem. 128, 469–483. doi:10.1016/j.jfluchem.2006.12.014
Shibata, N., Mizuta, S., and Kawai, H. (2008). Recent Advances in Enantioselective Trifluoromethylation Reactions. Tetrahedron: Asymmetry 19, 2633–2644. doi:10.1016/j.tetasy.2008.11.011
Shibata, N., Matsnev, A., and Cahard, D. (2010). Shelf-stable Electrophilic Trifluoromethylating Reagents: a Brief Historical Perspective. Beilstein J. Org. Chem. 6. doi:10.3762/bjoc.6.65
Shine, K. P., and Sturges, W. T. (2007). CO2 is Not the only gas. Science 315, 1804-1805. doi:10.1126/science.1141677
Sovacool, B. K., Griffiths, S., Kim, J., and Bazilian, M. (2021). Climate Change and Industrial F-Gases: a Critical and Systematic Review of Developments, Sociotechnical Systems and Policy Options for Reducing Synthetic Greenhouse Gas Emissions. Renew. Sustain. Energ. Rev. 141, 110759–110813. doi:10.1016/j.rser.2021.110759
Stahl, T., Klare, H. F. T., and Oestreich, M. (2013). Main-Group Lewis Acids for C-F Bond Activation. ACS Catal. 3, 1578–1587. doi:10.1021/cs4003244
Stanifer, J. W., Stapleton, H. M., Souma, T., Wittmer, A., Zhao, X., and Boulware, L. E. (2018). Perfluorinated Chemicals as Emerging Environmental Threats to Kidney Health. Cjasn 13, 1479–1492. doi:10.2215/CJN.04670418
Velders, G. J. M., Andersen, S. O., Daniel, J. S., Fahey, D. W., and Mcfarland, M. (2007). The Importance of the Montreal Protocol in Protecting Climate. Proc. Natl. Acad. Sci. 104, 4814–4819. doi:10.1073/pnas.0610328104
Vierke, L., Staude, C., Biegel-Engler, A., Drost, W., and Schulte, C. (2012). Perfluorooctanoic Acid (PFOA) - Main Concerns and Regulatory Developments in Europe from an Environmental point of View. Environ. Sci. Eur. 24, 16–26. doi:10.1186/2190-4715-24-16
Xiao, H., Zhang, Z., Fang, Y., Zhu, L., and Li, C. (2021). Radical Trifluoromethylation. Chem. Soc. Rev. 50, 6308–6319. doi:10.1039/D1CS00200G
Zhou, J., Jiang, B., Fujihira, Y., Zhao, Z., Imai, T., and Shibata, N. (2021). Catalyst-free Carbosilylation of Alkenes Using Silyl Boronates and Organic Fluorides via Selective C-F Bond Activation. Nat. Commun. 12, 3749. doi:10.1038/s41467-021-24031-w
Keywords: defluorosilylation, transition-metal-free catalysis, C–F bond activation, silylboronate, nickel
Citation: Zhou J, Zhao Z and Shibata N (2021) Silylboronate-Mediated Defluorosilylation of Aryl Fluorides with or without Ni-Catalyst. Front. Chem. 9:771473. doi: 10.3389/fchem.2021.771473
Received: 06 September 2021; Accepted: 04 October 2021;
Published: 25 October 2021.
Edited by:
Iwao Ojima, Stony Brook University, United StatesReviewed by:
Ming-Yu Ngai, Stony Brook University, United StatesSantos Fustero, University of Valencia, Spain
Copyright © 2021 Zhou, Zhao and Shibata. This is an open-access article distributed under the terms of the Creative Commons Attribution License (CC BY). The use, distribution or reproduction in other forums is permitted, provided the original author(s) and the copyright owner(s) are credited and that the original publication in this journal is cited, in accordance with accepted academic practice. No use, distribution or reproduction is permitted which does not comply with these terms.
*Correspondence: Norio Shibata, bm96c2hpYmFAbml0ZWNoLmFjLmpw