- School of Chemistry and Chemical Engineering, Nantong University, Nantong, China
Indocyanine green (ICG) is a classical near-infrared (NIR) photothermal reagent that can be employed in clinical medical detection. Under neutral conditions, ICG can adsorb NIR light effectively for photothermal (PTT) and photodynamic (PDT) therapy. However, ICG is easily degraded in weak acid environments, which seriously restricts its application. In this work, a cationic water-soluble pillar[5]arene (WP5) was selected as the stabilizing agent for ICG. Thanks to the host-guest interaction between WP5 and alkyl sulfonate, the stability and the photothermal conversion efficiency of ICG increased remarkably upon addition of WP5 as investigated by UV-vis spectrum and photothermal studies. Furthermore, an in vitro study showed higher efficiency of WP5&ICG in killing cancer cells in a shorter treatment time than the free ICG. Hence, it is hopeful that WP5 can be a new type of supramolecular host in enhancing the stability and photothermal conversion efficiency of photosensitizers.
Introduction
In today’s society, cancer is one of the world’s most concerning health problems to humans, and the main means of cancer treatment are operation, radiotherapy, and chemotherapy (Issels, 2008; Mcguire, 2016; Song et al., 2015). Operative treatment is highly controllable, but it is limited to large-area tumor tissue removal. Radiotherapy and chemotherapy cause many normal cells to die during the treatment because of their indiscriminate attack on human cells, which can cause side effects and relapse. In recent years, photothermotherapy has attracted tremendous attention due to the fact it can induce tumor cell necrosis at specific sites with minimal invasion and human side effects (Liu et al., 2019; Zheng et al., 2021). Near-infrared radiation (NIR) is particularly beneficial for photothermotherapy because near-infrared light penetrates deeply through tissues, and endogenous biomolecules absorb fewer photons and cause less cell damage in this wavelength range (Cen et al., 2020).
Indocyanine green (ICG) is a kind of low-toxicity photothermal reagent, which has a characteristic absorption peak in the near-infrared region, and its maximum emission wavelength is about 800 nm (Sheng et al., 2013). ICG is widely used in medical diagnosis, such as blood volume, liver function, ophthalmologic angiography, etc. ICG can effectively absorb near-infrared light and convert it into singlet oxygen and heat. Combined with the excellent tissue penetrating ability of near-infrared light and little effect on the tissue itself, ICG can be used in photothermal therapy (PTT) and photodynamic therapy (PDT) (Shafirstein et al., 2012). However, ICG is easily aggregated in aqueous solution, which affects its photothermal conversion and singlet oxygen generation efficiency. In addition, ICG also decomposes rapidly under illumination, especially in weak acid environments, which limits the application prospect of ICG in tumor therapy.
Pillar[5]arenes (Ogoshi et al., 2008; Xue et al., 2012; Ogoshi et al., 2019), composed of hydroquinone or its derivatives bridged by–CH2– in the 2,5-positions, are a smart type of macro-cyclic hosts after crown ethers (Yoo et al., 2019; Zhou et al., 2017), cyclodextrins (Lai et al., 2017), calixarenes (Li et al., 2020), and cucurbiturils (Jiang et al., 2020; Chernikova and Berdnikova, 2020). The preparation and modification of pillar[5]arene is convenient and efficient, which make them outstanding affinity hosts for selectively guests (Chao et al., 2020; Duan et al., 2020; Hao et al., 2020; Sheng et al., 2020; Xu et al., 2020; Guo et al., 2020a; Guo et al., 2020b; Cao et al., 2021; Chen et al., 2021; Shin et al., 2021). Considering the convenient synthesis of pillar[n]arenes and their rich host-guest properties, functional materials based on pillar[n]arenes have been widely studied and applied in various fields, such as drug delivery systems (Cai et al., 2021; Yao et al., 2017; Xiao et al., 2019), molecular machines (Han et al., 2020; Dong et al., 2014; Wan et al., 2020), trans-membrane channels (Chen et al., 2013; Strilets et al., 2020), and supramolecular polymeric materials (Xiao et al., 2018; Jie et al., 2020; Zhang et al., 2020). For example, Prof. Huang’s group found that anticancer drug tamoxifen could form a stable complex with water-soluble pillar[6]arene, which will enhance the solubility and bioactivity of tamoxifen (Shangguan et al., 2017). Although pillar[n]arene has been widely applied in the biological field (Yao et al., 2016; Song et al., 2021), the application of pillar[n]arene to improve the stability of photosensitizers has not been investigated. Herein, a cationic water-soluble pillar[5]arene (WP5) was selected to form a complex with indocyanine green (ICG). Due to fact that alkyl sulfonate can be entrapped in the cavity of WP5, ICG was stabilized with its photothermal conversion efficiency increased. We hope this host-guest strategy can be applied in other photosensitizers to prove their stability and activity.
Experiment Section
Synthesis of Cationic Water-Soluble Pillar[5]arene
As shown in Scheme 1 hydroquinone (5.0 g, 0.045 mol), 1,2-dibromoethane (34.2 g, 0.182 mol), and K2CO3 (12 g, 0.09 mol) were added in 250 ml of acetone. The mixture was stirred at 60°C for 24 h under an N2 atmosphere. When the inorganic solid was removed, the obtained solvent was evaporated and the residue was purified by chromatography on silica gel (petroleum ether/CH3COOCH2CH3, v/v 50:1) to give A as a white crystal. 1H NMR (400 MHz, CDCl3) as shown in Supplementary Figure S1: δ 6.86 (s, 4H, ArH), 4.25 (t, J = 6.3 Hz, 4H, -CH2-), 3.62 (t, J = 6.3 Hz, 4H, -CH2-). Then A (5.0 g), paraformaldehyde (3.0 g), and 2.25 ml of BF3•Et2O were added to 50 ml of ClCH2CH2Cl and stirred at 25°C until the reaction was finished. Then the saturated NaHCO3 solution was added into the mixture. The mixture was separated and the organic phase was collected. The solvent was evaporated and the residue was purified by chromatography on silica gel (petroleum ether/CH3COOCH2CH3, v/v 25:1) to give B as a white solid. 1H NMR (400 MHz, CDCl3) as shown in Supplementary Figure S2: δ 6.91 (s, 10H, ArH), 4.23 (dd, J = 6.3, 5.1 Hz, 20H, -CH2-), 3.84 (s, 10H, -CH2-), 3.63 (t, J = 5.7 Hz, 20H, -CH2-). At last, B (1.68 g, 1.00 mmol) and trimethylamine (2.36 g, 40.0 mmol) were stirred into 50 ml of dry toluene overnight under reflux. The reaction solvent was evaporated and the residue was recrystallized with CH3CH2OH (1.72 g, yield: 87.3%). 1H NMR (400 MHz, D2O), as shown in Supplementary Figure S3, δ 6.92 (s, 10 H, ArH), 4.49–4.32 (m, 20 H), 3.89–3.54 (m, 30 H), 3.33 (m, 90 H, N-CH3). 13C NMR (101 MHz, D2O) as shown in Supplementary Figure S4, δ: 148.98, 129.59, 116.42, 115.27, 65.49, 64.94, 63.31, 62.71, 54.04, 29.33. MS (m/z): HRMS (ESI) Calcd. for C85H150Br8N10O10 ([M – 2Br]2+): 1055.2461, found: 1055.2716 (Supplementary Figure S5). Elemental analysis: N, 6.15%; C, 44.86%; H, 6.59%.
Materials
All reagents and solvents were commercially available in analytical grade and used as received. Further purification and drying by standard methods were employed and the solvents and reagents were distilled prior to use when necessary. All evaporations of organic solvents were carried out with a rotary evaporator in conjunction with a water aspirator.
Methods
NMR spectroscopy: 1H and 13C NMR spectra were recorded with an Aviance III 400 MHz or 600 MHz liquid-state NMR spectrometer.
ESI-MS spectroscopy: Electrospray ionization mass spectra (ESI-MS) were measured by Agilent 6520 Q-TOF-MS.
Fluorescence spectroscopy: Fluorescence spectra were recorded on a Shimadzu HITACHI F-4500 spectrophotometer.
Cell viability: Human cervical cancer cells (HeLa cells) were incubated in Dulbecco’s modified Eagle’s medium (DMEM). The medium was supplemented with 10% fetal bovine serum and 1% penicillin-streptomycin. HeLa cells were seeded in 96-well plates (5 × 104 cell ml−1, 0.1 ml per well) for 24 h at 37°C in 5% CO2. Then the cells were incubated with WP5⊃ICG for 24 h. The relative cellular viability was determined by the MTT assay.
Confocal laser scanning microscopy: HeLa cells were seeded in 6-well plates (5 × 104 cell ml−1, 2 ml per well) for 24 h at 37°C in 5% CO2. The cells were incubated with the corresponding solution for 4 h. Then the medium was removed, and the cells were washed with phosphate buffer solution three times. Finally, the cells were subjected to observation by a confocal laser scanning microscope.
Photothermal conversion: For measuring the photothermal conversion performances of ICG and WP5⊃ICG, an 808 nm NIR laser was delivered through a quartz cuvette containing an aqueous dispersion (3 ml) of the sample with different concentrations (0–200 μg/ml), and the light source was an external adjustable power (1 W/cm2) 808-nm semiconductor laser device (LR-MFJ-808/1W, Changchun Femtosecond Technology Co. Ltd., China). The temperature was monitored by a thermometer and recorded once every 30 s. The temperature signals also recorded at different time intervals (0–10 min) were analyzed with FL-IR tools systems.
Results and Disscussion
Host-Guest Interaction
As WP5 presents 10 quaternary ammonium cations on its macrocyclic framework, it can form a complex with anionic guests efficiently. The host-guest property of WP5 with anionic guests was investigated in detail, which revealed that WP5 displayed high affinities for sodium dodecyl sulfonate in aqueous solution. As shown in Figure 1, when WP5 and sodium dodecyl sulfate (C) were dissolved in water, the host-guest complex was formed. The 1H NMR spectra of an aqueous solution of WP5 (16.00 mM) and C (16.00 mM) showed that the complex was in fast exchange on the 1H NMR time scale and an upfield shift had taken place for Ha and Hb on guest C after complexation (Figure 1A). Furthermore, the overlapped signal corresponding to Hal was obviously split into five separate peaks (Figure 1A, right).
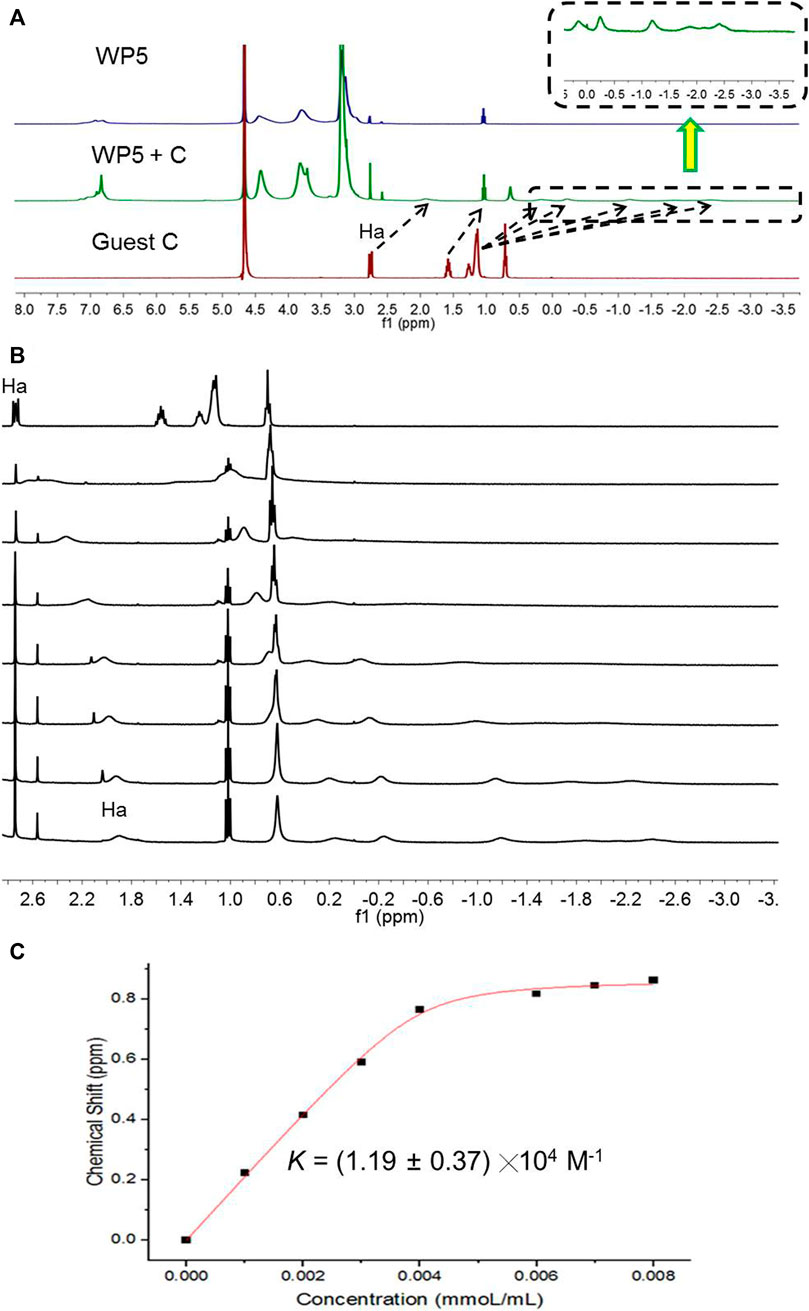
FIGURE 1. (A) 1H NMR spectra (D2O, 293 K, 400 MHz) of WP5 (16.00 mM), (B) WP5 (16.00 mM) + C (16.00 mM), and C (16.00 mM). (C) 1H NMR spectra (D2O, 293 K, 400 MHz) of C at a concentration of 16 mM with different concentrations (mM) of WP5. (C) The chemical shift changes of Ha on C upon addition of WP5.
The above results showed that the linear guest C penetrated into the cavity of WP5 to form a [2]pseudorotaxane, the anion head of C was close to the trimethylammonium groups of WP5, and the Hal in the middle of alkyl chain lay in the cavity of pillar[5]arene, the H at the tail of C was outside the cavity (Figure 1A). The driving force for the formation of the complex was hydrophobic and electrostatic interactions. The hydrophobic cavity of WP5 could hold the hydrophobic alkyl chain of C and the cationic trimethylammonium groups of WP5 could bind the anionic sulfonate group of C via electrostatic interaction seen through 2D Nuclear Overhauser Effect Spectroscopy (NOESY). As shown in Supplementary Figure S6, the hydrogens of the alkyl chain on C were close to the pillar[5]arene platform because Hal showed a strong correlation with Hph, indicating that the alkyl chain was in close proximity to the cavity.
We then added the 1H NMR titration of WP5 into the aqueous solution of C (16.00 mM) to investigate the ability of the WP5 complex with C (Figure 1B). As shown in Figure 1B, the proton NMR signal related to Ha shifted upfield considerably with the increase of the concentration of WP5. When the mole ratio of WP5/C was 1, the Ha protons of C shifted upfield about 0.90 ppm while Hal protons on C shifted upfield even more. However, when the concentration of WP5 was higher than 16.00 mM, chemical shifts of Ha protons were almost unchanged, indicating the formation of a 1: 1 complex between WP5 and C in water. The association constant (Ka) of WP5⊃C was calculated to be (1.19 ± 0.37) ╳ 104 M−1 by using a nonlinear curve-fitting analysis (Figure 1C).
Stability and Photothermal Conversion Investigation
After confirming the host-guest interaction between WP5 and C, we used WP5 to enhance the stability and photothermal conversion efficiency of ICG in water. From the UV-vis spectra, we found that ICG exhibited a strong absorption peak in the wavelength range of 600–900 nm (Figure 2A), which indicated that ICG could absorb near-infrared light well, and provided the possibility for photothermal therapy. Then we investigated the stability of ICG under illumination in water. After being irradiated with 808 nm light (1 W/cm2) for 30 min, the absorption of the pure ICG solution both in neutral (Figure 2A) or weak acidic (Figure 2B) environments was decreased significantly, indicating that ICG is unstable and easily degraded under illumination, especially in acid conditions. However, for the WP5⊃ICG group, the absorption peak of ICG decreased less under the same conditions, indicating that the host-guest complexation could protect ICG from degradation under light conditions (Figure 2C). We further studied the photothermal conversion efficiency of ICG in the aqueous solution. As shown in Figure 2D, with the increase of acid, the photothermal conversion efficiency of ICG decreased. But when forming the WP5⊃ICG complex, even at pH = 5.5, the temperature rose higher than that of pure ICG (Figure 2D). Infrared thermal images confirmed that the WP5⊃ICG complex could prove the photothermal conversion efficiency of ICG (Supplementary Figure S7). Importantly, by measuring the temperature change of ICG and WP5⊃ICG under the 808 nm laser and pH = 5.5 for six on/off laser cycles, we found that with each cycle, the increase in temperature dropped sharply for pure ICG (Figure 2E), but for WP5⊃ICG, it still increased by about 70% of the maximum temperature after six cycles (Figure 2F). These experimental results show that ICG is more easily degraded by light in weak acid conditions, and after forming a host-guest complex with WP5, WP5 can protect ICG and improve its photothermal conversion efficiency and stability.
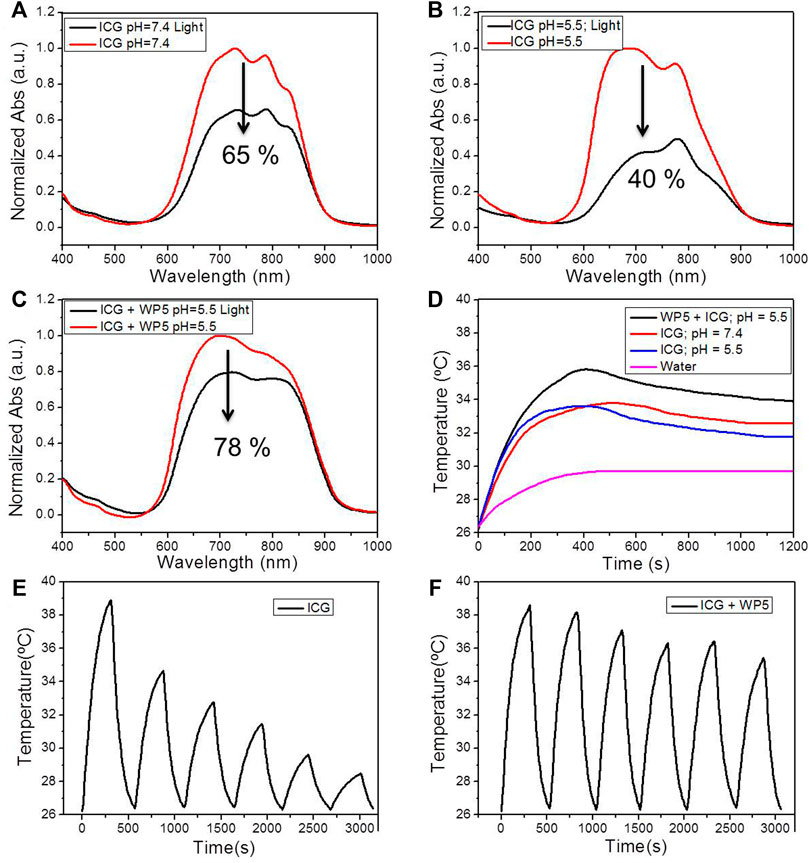
FIGURE 2. (A) UV-vis spectra of ICG (10 μg/ml) before and after irradiating with 808 nm light for 5 min when pH = 7.4. (B) UV-vis spectra of ICG (10 μg/ml) before and after irradiating with 808 nm light for 5 min when pH = 5.5. (C) UV-vis spectra of ICG (10 μg/ml) + WP5 before and after irradiating with 808 nm light for 5 min when pH = 5.5. (D) Photothermal conversion behavior of water, ICG (pH = 5.5), ICG (pH = 7.4), and WP5⊃ICG (pH = 5.5) under 808 nm laser irradiation (1.0 W cm−2). (E) Temperature variations of ICG (pH = 5.5) under 808 nm laser irradiation over six cycles of heating/cooling. (F) Temperature variations of WP5⊃ICG (pH = 5.5) under 808 nm laser irradiation over six cycles of heating/cooling.
Cell Viability (MTT) Assay
The above results suggest that WP5⊃ICG can be used as an excellent photothermo-therapeutic agent in the weak acidic microenvironment of tumor tissue. So we cultured HeLa cells with ICG and WP5⊃ICG at a certain concentration, and irradiated them with 808 nm of near-infrared light for different lengths of time. The relative activity of HeLa cells was measured by an MTT assay. The treated cells were double-stained with calcein AM/propidium iodide (PI) to differentiate the living cells from the dead cells. As shown in Figure 3A, ICG and WP5⊃ICG did not show cytotoxicity with increasing concentration in the absence of light, but both ICG and WP5⊃ICG showed cytotoxicity in the presence of near-infrared light. Moreover, the cytotoxicity of WP5⊃ICG was stronger than that of ICG. The cytotoxicity of ICG was found to be unchanged after 2 min of laser irradiation, indicating that ICG was degraded in tumor tissue after 2 min of laser irradiation, no further light conversed into heat to kill cancer cells. However, for WP5⊃ICG, the cytotoxicity continued to increase over time, suggesting that with the protection of WP5, ICG can continuously and steadily transform light into heat to kill cancer cells. The fluorescence imaging data of the cells were consistent with the relative cytotoxicity studies. As shown in Figure 3B, the green color represents living cells, and the red represents dead cells. We can clearly see that all the cancer cells died when treated with the WP5⊃ICG + light group while for ICG + light, only 60% of cancer cells died. In conclusion, the complex formed by WP5 and ICG cannot only improve the photothermal conversion efficiency of ICG, but also greatly improve its stability, which makes WP5⊃ICG a promising photothermal therapeutic agent for cancer.
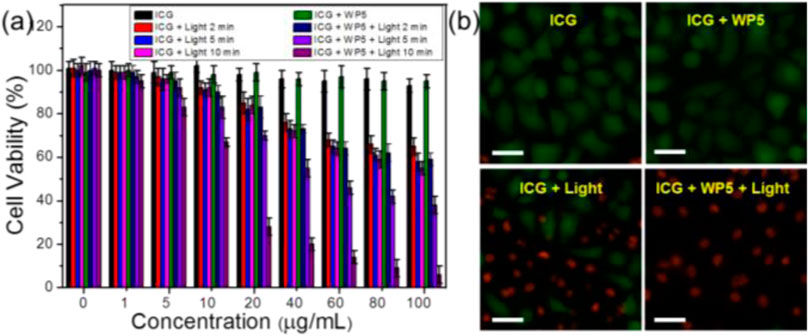
FIGURE 3. (A) Cell viabilities of HeLa cells treated with different groups with 808 nm laser irradiation (1 W/cm2). Error bars were based on the standard error of mean (n = 4). (B) Fluorescence images of calcein AM (live cells, green) and PI (dead cells, red) co-stained HeLa cells after different treatments (ICG = 100 μg/ml, irradiated for 10 min).
Conclusion
A trimethylammonium functionalized cationic water-soluble pillar[5]arene (WP5) was designed and synthesized. It was found that WP5 and linear guest C could form a stable host-guest complex by 1H NMR. As ICG has two alkyl sulfonates, the host-guest interaction between WP5 and ICG cannot only inhibit the π-π stacking between ICG molecules, but can also improve the photothermal conversion efficiency of ICG in water. Moreover, ICG can also be protected by WP5 to reduce its degradation rate under light conditions and improve its stability. Cell experiments showed that WP5⊃ICG has a better ability to kill cancer cells under near-infrared light than ICG in the weak acidic microenvironment of tumor tissue. Moreover, as WP5⊃ICG is more stable, it can kill cancer cells when exposed to light continuously. The method of improving the stability and conversion efficiency of photothermal reagents through the host-guest interaction provides a new idea for cancer therapy. Our following study will focus on animal experiments.
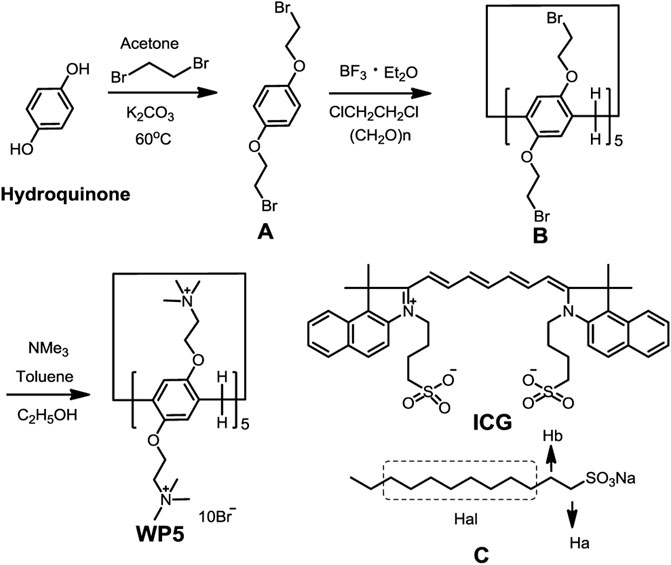
SCHEME 1. Synthetic route to cationic water-soluble pillar[5]arene and the chemical structures of ICG and model guest C.
Data Availability Statement
The original contributions presented in the study are included in the article/Supplementary Material, further inquiries can be directed to the corresponding authors.
Author Contributions
The authors contributed to the present paper as follows: YD and CW prepared all the compounds; BL and YY analyzed the data; and YD and YY wrote the paper.
Funding
This work was supported by the National Natural Science Foundation of China (21801139) and Natural Science Foundation of Jiangsu Province (BK20180942).
Conflict of Interest
The authors declare that the research was conducted in the absence of any commercial or financial relationships that could be construed as a potential conflict of interest.
Publisher’s Note
All claims expressed in this article are solely those of the authors and do not necessarily represent those of their affiliated organizations, or those of the publisher, the editors and the reviewers. Any product that may be evaluated in this article, or claim that may be made by its manufacturer, is not guaranteed or endorsed by the publisher.
Acknowledgments
We also thank Nantong University Analysis & Testing Center for characterization.
Supplementary Material
The Supplementary Material for this article can be found online at: https://www.frontiersin.org/articles/10.3389/fchem.2021.775436/full#supplementary-material
References
Cai, Y., Zhang, Z., Ding, Y., Hu, L., Wang, J., Chen, T., et al. (2021). Recent Development of Pillar[n]arene-Based Amphiphiles. Chin. Chem. Lett. 32, 1267–1279. doi:10.1016/j.cclet.2020.10.036
Cao, S., Zhou, L., Liu, C., Zhang, H., Zhao, Y., and Zhao, Y. (2021). Pillararene-based Self-Assemblies for Electrochemical Biosensors. Biosens. Bioelectron. 181, 113164. doi:10.1016/j.bios.2021.113164
Cen, M., Ding, Y., Wang, J., Yuan, X., Lu, B., Wang, Y., et al. (2020). Cationic Water-Soluble Pillar[5]arene-Modified Cu2-xSe Nanoparticles: Supramolecular Trap for ATP and Application in Targeted Photothermal Therapy in the NIR-II Window. ACS Macro Lett. 9, 1558–1562. doi:10.1021/acsmacrolett.0c00714
Chao, S., Lv, X., Ma, N., Shen, Z., Zhang, F., Pei, Y., et al. (2020). A Supramolecular Nanoprodrug Based on a Boronate Ester Linked Curcumin Complexing with Water-Soluble Pillar[5]arene for Synergistic Chemotherapies. Chem. Commun. 56, 8861–8864. doi:10.1039/D0CC04315J
Chen, J., Zhang, Y., Chai, Y., Meng, Z., Zhang, Y., Chen, L., et al. (2021). Synergistic Enhancement of the Emergency Treatment Effect of Organophosphate Poisoning by a Supramolecular Strategy. Chem. Sci. 12, 5202–5208. doi:10.1039/D1SC00426C
Chen, L., Si, W., Zhang, L., Tang, G., Li, Z.-T., and Hou, J.-L. (2013). Chiral Selective Transmembrane Transport of Amino Acids through Artificial Channels. J. Am. Chem. Soc. 135 (6), 2152–2155. doi:10.1021/ja312704e
Chernikova, E. Y., and Berdnikova, D. V. (2020). Cucurbiturils in Nucleic Acids Research. Chem. Commun. 56, 15360–15376. doi:10.1039/D0CC06583H
Dong, S., Yuan, J., and Huang, F. (2014). A Pillar[5]arene/imidazolium [2]rotaxane: Solvent- and Thermo-Driven Molecular Motions and Supramolecular Gel Formation. Chem. Sci. 5, 247–252. doi:10.1039/C3SC52481G
Duan, Q., Wang, L., Wang, F., Zhang, H., and Lu, K. (2020). Facile One-step Electrodeposition Preparation of Cationic Pillar[6]arene-Modified Graphene Films on Glassy Carbon Electrodes for Enhanced Electrochemical Performance. Front. Chem. 8, 430. doi:10.3389/fchem.2020.00430
Guo, H., Yan, X., Lu, B., Wang, J., Yuan, X., Han, Y., et al. (2020b). Pillar[5]arene-based Supramolecular Assemblies with Two-step Sequential Fluorescence Enhancement for Mitochondria-Targeted Cell Imaging. J. Mater. Chem. C 8, 15622–15625. doi:10.1039/D0TC04343E
Guo, H., Ye, J., Zhang, Z., Wang, Y., Yuan, X., Ou, C., et al. (2020a). Pillar[5]arene-Based [2]Rotaxane: Synthesis, Characterization, and Application in a Coupling Reaction. Inorg. Chem. 59, 11915–11919. doi:10.1021/acs.inorgchem.0c01752
Han, Y., Nie, C.-Y., Jiang, S., Sun, J., and Yan, C.-G. (2020). Synthesis and Characterization of Bis-[1]rotaxanes via Salen-Bridged Bis-Pillar[5]arenes. Chin. Chem. LettersChem. Lett. 31, 725–728. doi:10.1016/j.cclet.2019.09.014
Hao, M., Sun, G., Zuo, M., Xu, Z., Chen, Y., Hu, X. Y., et al. (2020). A Supramolecular Artificial Light‐Harvesting System with Two‐Step Sequential Energy Transfer for Photochemical Catalysis. Angew. Chem. Int. Ed. 59, 10095–10100. doi:10.1002/anie.201912654
Issels, R. D. (2008). Hyperthermia Adds to Chemotherapy. Eur. J. Cancer 44, 2546–2554. doi:10.1016/j.ejca.2008.07.038
Jiang, T., Qu, G., Wang, J., Ma, X., and Tian, H. (2020). Cucurbiturils Brighten Au Nanoclusters in Water. Chem. Sci. 11, 3531–3537. doi:10.1039/D0SC00473A
Jie, K., Zhou, Y., Sun, Q., Li, B., Zhao, R., Jiang, D.-e., et al. (2020). Mechanochemical Synthesis of Pillar[5]quinone Derived Multi-Microporous Organic Polymers for Radioactive Organic Iodide Capture and Storage. Nat. Commun. 11, 1086. doi:10.1038/s41467-020-14892-y
Lai, W.-F., Rogach, A. L., and Wong, W.-T. (2017). Chemistry and Engineering of Cyclodextrins for Molecular Imaging. Chem. Soc. Rev. 46, 6379–6419. doi:10.1039/C7CS00040E
Li, L., Tuo, W., Zhu, Q., Sepehrpour, H., Yao, Y., Yan, C., et al. (2020). Resorcinarene Induced Assembly of Carotene and Lutein into Hierarchical Superstructures. J. Am. Chem. Soc. 142, 20583–20587. doi:10.1021/jacs.0c10901
Liu, Y., Bhattarai, P., Dai, Z., and Chen, X. (2019). Photothermal Therapy and Photoacoustic Imaging via Nanotheranostics in Fighting Cancer. Chem. Soc. Rev. 48, 2053–2108. doi:10.1039/C8CS00618K
Mcguire, S. (2016). World Cancer Report 2014. Geneva, Switzerland: World Health Organization, International Agency for Research on Cancer, WHO Press, 2015, Adv. Nutr., 7, 418–419. World Cancer Report 2014 (Geneva, Switzerland: World Health Organization, International Agency for Research on Cancer, WHO Press2015). doi:10.3945/an.116.012211
Ogoshi, T., Kakuta, T., and Yamagishi, T. a. (2019). Applications of Pillar[ N ]arene‐Based Supramolecular Assemblies. Angew. Chem. Int. Ed. 58, 2197–2206. doi:10.1002/anie.201805884
Ogoshi, T., Kanai, S., Fujinami, S., Yamagishi, T.-a., and Nakamoto, Y. (2008). para-Bridged Symmetrical Pillar[5]arenes: Their Lewis Acid Catalyzed Synthesis and Host-Guest Property. J. Am. Chem. Soc. 130, 5022–5023. doi:10.1021/ja711260m
Shafirstein, G., Bäumler, W., Hennings, L. J., Siegel, E. R., Friedman, R., Moreno, M. A., et al. (2012). Indocyanine green Enhanced Near-Infrared Laser Treatment of Murine Mammary Carcinoma. Int. J. Cancer 130, 1208–1215. doi:10.1002/ijc.26126
Shangguan, L., Chen, Q., Shi, B., and Huang, F. (2017). Enhancing the Solubility and Bioactivity of Anticancer Drug Tamoxifen by Water-Soluble Pillar[6]arene-Based Host-Guest Complexation. Chem. Commun. 53, 9749–9752. doi:10.1039/C7CC05305C
Sheng, X., Li, E., Zhou, Y., Zhao, R., Zhu, W., and Huang, F. (2020). Separation of 2-Chloropyridine/3-Chloropyridine by Nonporous Adaptive Crystals of Pillararenes with Different Substituents and Cavity Sizes. J. Am. Chem. Soc. 142 (13), 6360–6364. doi:10.1021/jacs.0c01274
Sheng, Z., Hu, D., Xue, M., He, M., Gong, P., and Cai, L. (2013). Indocyanine Green Nanoparticles for Theranostic Applications. Nano-micro Lett. 5, 145–150. doi:10.1007/bf03353743
Shin, M., Kim, S., Lee, E., Jung, J. H., Park, I.-H., and Lee, S. S. (2021). Pillar[5]-bis-trithiacrown: Influence of Host-Guest Interactions on the Formation of Coordination Networks. Inorg. Chem. 60 (8), 5804–5811. doi:10.1021/acs.inorgchem.1c00114
Song, N., Zhang, Z., Liu, P., Dai, D., Chen, C., Li, Y., et al. (2021). Pillar[5]arene‐Modified Gold Nanorods as Nanocarriers for Multi‐Modal Imaging‐Guided Synergistic Photodynamic‐Photothermal Therapy. Adv. Funct. Mater. 31, 2009924. doi:10.1002/adfm.202009924
Song, X., Chen, Q., and Liu, Z. (2015). Recent Advances in the Development of Organic Photothermal Nano-Agents. Nano Res. 8, 340–354. doi:10.1007/s12274-014-0620-y
Strilets, D., Fa, S., Hardiagon, A., Baaden, M., Ogoshi, T., and Barboiu, M. (2020). Biomimetic Approach for Highly Selective Artificial Water Channels Based on Tubular Pillar[5]arene Dimers. Angew. Chem. Int. Ed. 59, 23213–23219. doi:10.1002/anie.202009219
Wan, K., Gao, S.-C., Fang, X., Xu, M.-Y., Yang, Y., and Xue, M. (2020). Oxacalix[4]arene-bridged Pillar[5]arene Dimers: Syntheses, Planar Chirality and Construction of Chiral Rotaxanes. Chem. Commun. 56, 10155–10158. doi:10.1039/D0CC04375C
Xiao, T., Qi, L., Zhong, W., Lin, C., Wang, R., and Wang, L. (2019). Stimuli-responsive Nanocarriers Constructed from Pillar[n]arene-Based Supra-amphiphiles. Mater. Chem. Front. 3, 1973–1993. doi:10.1039/C9QM00428A
Xiao, T., Xu, L., Zhong, W., Zhou, L., Sun, X.-Q., Hu, X.-Y., et al. (2018). Advanced Functional Materials Constructed from Pillar[n]arenes. Isr. J. Chem. 58, 1219–1229. doi:10.1002/ijch.201800026
Xu, X., Jerca, V. V., and Hoogenboom, R. (2020). Structural Diversification of Pillar[ N ]arene Macrocycles. Angew. Chem. Int. Ed. 59, 6314–6316. doi:10.1002/anie.202002467
Xue, M., Yang, Y., Chi, X., Zhang, Z., and Huang, F. (2012). Pillararenes, A New Class of Macrocycles for Supramolecular Chemistry. Acc. Chem. Res. 45, 1294–1308. doi:10.1021/ar2003418
Yao, Y., Sun, Y., Yu, H., Chen, W., Dai, H., and Shi, Y. (2017). A Pillar[5]arene Based Gel from a Low-Molecular-Weight Gelator for Sustained Dye Release in Water. Dalton Trans. 46, 16802–16806. doi:10.1039/C7DT04001F
Yao, Y., Wang, Y., Zhao, R., Shao, L., Tang, R., and Huang, F. (2016). Improved In Vivo Tumor Therapy via Host-Guest Complexation. J. Mater. Chem. B 4, 2691–2696. doi:10.1039/C5TB02611C
Yoo, C., Dodge, H. M., and Miller, A. J. M. (2019). Cation-controlled Catalysis with crown Ether-Containing Transition Metal Complexes. Chem. Commun. 55, 5047–5059. doi:10.1039/C9CC00803A
Zhang, R., Yan, X., Guo, H., Hu, L., Yan, C., Wang, Y., et al. (2020). Supramolecular Polymer Networks Based on Pillar[5]arene: Synthesis, Characterization and Application in the Fenton Reaction. Chem. Commun. 56, 948–951. doi:10.1039/C9CC09155F
Zheng, Q., Liu, X., Zheng, Y., Yeung, K. W. K., Cui, Z., Liang, Y., et al. (2021). The Recent Progress on Metal-Organic Frameworks for Phototherapy. Chem. Soc. Rev. 50, 5086–5125. doi:10.1039/D1CS00056J
Keywords: indocyanine green, pillar[5]arene, host-guest interaction, cancer therapy, photothermal
Citation: Ding Y, Wang C, Lu B and Yao Y (2021) Enhancing the Stability and Photothermal Conversion Efficiency of ICG by Pillar[5]arene-Based Host-Guest Interaction. Front. Chem. 9:775436. doi: 10.3389/fchem.2021.775436
Received: 14 September 2021; Accepted: 05 October 2021;
Published: 29 October 2021.
Edited by:
Pavel Padnya, Kazan Federal University, RussiaReviewed by:
Tangxin Xiao, Changzhou University, ChinaBingbing Shi, Northwest Normal University, China
Nasim Chiniforush, Tehran University of Medical Sciences, Iran
Copyright © 2021 Ding, Wang, Lu and Yao. This is an open-access article distributed under the terms of the Creative Commons Attribution License (CC BY). The use, distribution or reproduction in other forums is permitted, provided the original author(s) and the copyright owner(s) are credited and that the original publication in this journal is cited, in accordance with accepted academic practice. No use, distribution or reproduction is permitted which does not comply with these terms.
*Correspondence: Bing Lu, MjAyMDAyOGx1YmluZ0BudHUuZWR1LmNu; Yong Yao, eWFveW9uZzE5ODZAbnR1LmVkdS5jbg==
†These authors have contributed equally to this work