- 1School of Chemical Engineering of Guizhou Minzu University, Guiyang, China
- 2Key Laboratory of Rubber-Plastics of Ministry of Education/Shandong Province (QUST), School of Polymer Science and Engineering, Qingdao University of Science and Technology, Qingdao, China
For polymer semiconductors, the packing ability and molecular weight of polymers play a very critical role in their optoelectronic properties and carrier transport properties. In this work, two polymers, named linear and branch, are designed and synthesized with donor–acceptor (D-A) structure, based on diketopyrrolopyrrole as an electron acceptor and carbazole as an electron donor, and applied these two polymers in organic field-effect transistors. Linear and branch have similar conjugated backbones but different molecular weights and alkyl chains. The effects of molecular weight and molecular aggregation ability on the carrier transfer efficiency are investigated. As a result, linear exhibits better aggregation ability, but due to its smaller molecular weight than branch molecule, the hole transfer efficiency of linear (1.1 × 10−2 cm2 V −1 s−1) is slightly lower than that of branch (2.3 × 10−2 cm2 V −1 s−1). This work proves that molecular weight is more important than molecular aggregation ability when designing organic field-effect transistors for polymer semiconductors.
Introduction
In the past few decades, organic conjugated polymers have been widely used in the field of field-effect transistors due to their flexible molecular structures and good solubility (Guo et al., 2013; Moon et al., 2018; Michael, 2021). In the late 1980s, the first organic field-effect tube made of polythiophene was developed for the first time (Cui et al., 2018; Zou et al., 2019). This kicked off the development of organic field-effect transistors (OFETs). After decades of development, OFETs have made great progress (Pan et al., 2018; Ran et al., 2020; Raveendran and Namboothiry, 2020).
At the beginning of this century, a wide variety of OFET materials sprung up like mushrooms after a rain. In the process of improving device performance, a large number of conjugated polymer materials have been developed. The molecular design of high-performance conjugated polymers is based on the concepts of molecular orbital energetics and crystal engineering, enabling efficient control of frontier orbital energy levels and π orbital overlap (Nahid et al., 2016; Moon et al., 2018; Qu et al., 2021; Ozdemir et al., 2017; Ozdemir et al., 2020). Therefore, in the past research, there are a lot of strategies to explore the structure of polymer main chain and side chain, such as the main chain π-conjugated orbital extension, main chain planarity improvement, and side chain and main chain hydrogen bonding engineering (Wang et al., 2018; Cheon et al., 2022; Kang et al., 2022). It was found that polymers with branched side chains can effectively increase the solubility of polymers. However, branched chains might affect the π-π stacking properties of molecules, which is not conducive to the transfer of carriers; thus, the application of branch alkyl chains in conjugated polymers remains to be further investigated (Liu et al., 2017; Pie et al., 2017).
In this work, we designed a series of DPP-CZ-based conjugated polymers with octane and 2,2,4-trimethylpentane focusing on the effects of branched vs. linear and molecular weight on polymer device performance. The aggregation and crystallization properties of the materials were investigated by XRD. Although the electrochemical properties of the polymers were very similar, their device properties showed some differences due to different molecular weights.
Result and discussion
Synthesis and properties of DPP-based polymers
Two polymers, named linear and branch (Figure 1A), are synthesized by the Suzuki coupling reaction through diketopyrrolopyrrole (DPP)-based monomers as electron acceptor units and carbazole groups with different alkyl chains as electron donor units (Supplementary Schemes S5, S6). In the process of synthesizing the polymer, the molecular weight of the polymer is controlled by changing the length of the polymerization time. The main chains of the two polymers are fixed so as to compare the molecular weight and the alkyl side chain of the polymer in the construction of high-performance organic field-effect transistors. The molecular weight (Mw) values of linear and branch polymers were 12 and 22 kDa, and the PDI is 1.7 and 1.9, respectively.
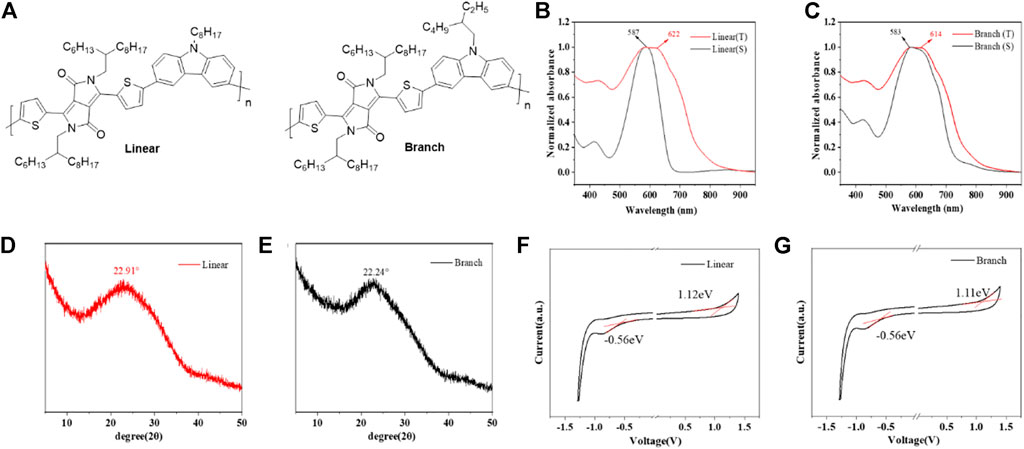
FIGURE 1. (A) Chemical structures of both linear and branch polymers. (B,C) UV/vis absorption spectra of both polymers in solution and thin film state. (D, E) XRD spectra of both polymers. (F,G) Cyclic voltammograms of both polymers (spin-coated thin films on the ITO glass). Electrolyte: 0.1 M tetrabutylammonium hexafluorophosphate in acetonitrile. Potential calculated versus ferrocene. Scan rate: 100 mV s−1. Temperature: room temperature.
Optical and electrochemical properties
Figures 1B and C show the UV-Vis absorption spectra of the two polymers in solution and film state, and their specific parameters are listed in Table 1. Since they have similar main chain structures, their UV absorptions also show very similar curves, but due to the difference in the alkyl chains, in contrast, linear has a red-shift of 15 nm in the absorption maximum from solution to thin film samples, while branch is 11 nm, indicating that linear molecules have slightly stronger aggregation ability, which was considered to be able to effectively improve the carrier transfer efficiency in past studies. The onset absorption of both polymer films starts at 775 nm, based on which the optical band gaps are calculated to be 1.60 eV for branch and 1.59 eV for linear, respectively. In order to further verify the effect of the alkyl chain on molecular aggregation ability and π-π stacking ability, XRD was tested as shown in Figures 1D and 3. Both molecules showed intermolecular co-facial π-π stacking peaks, located at 22.91° and 22.24°, corresponding to the d-spacing of 0.387 and 0.40 nm for linear and branch polymers, respectively.
As shown in Figures 1F and G, the two polymers exhibited very similar electrochemical profiles due to their identical backbone structural units. Both polymers showed similar oxidation onset potentials around 1.11–1.12 eV, based on which the highest occupied molecular orbital (HOMO) energy levels were calculated to be −5.92 eV and −5.91 eV for branch and linear polymers, respectively. Similar electrochemical profiles suggest that the two polymers can exhibit similar hole-injection abilities after being assembled into OFETs. This also proves that the difference between the two devices mainly comes from the difference in molecular weight and side chain. The electrochemical band gap is 1.67 eV for branch and 1.68 eV for linear, whose value is similar to the optical ones.
OFET
The charge transport properties of the two polymers were evaluated by fabricating the OFET devices of the materials with a bottom-gate and bottom-contact (BGBC) configuration on an n-type silicon wafer using a layer of 300 nm SiO2 as the dielectric material. The linear and branch polymer-based devices were fabricated via directly spin-casting the polymer solution in chloroform onto the OTS-treated silicon wafer with pre-patterned gold source and drain electrodes, which were further measured under vacuum conditions. The detailed device fabrication and testing procedures are described in the Supporting Information. As shown in Figure 2, both polymers exhibited p-type properties with the highest hole mobility (μh) estimated to be 1.1 × 10−2 cm2 V −1 s−1 (average μh: 9 × 10−3 cm2 V −1 s−1) and 2.3 × 10−2 cm2 V −1 s−1 (average μh: 1.9 × 10−3 cm2 V −1 s−1) for linear and branch obtained from eight different devices, respectively. Both polymers present a similar polymer backbone. In addition, compared to the branch polymer, the linear one exhibits improved aggregation and π-π stacking. Thus, high charge mobility is expected for the linear polymer. However, in this work, we found that the branch one presents a double value for hole transport mobility when compared to the linear one. This indicates that to obtain a high-performance semiconductor, molecular weight is more important than molecular aggregation ability for the molecular design concept. The current ratio of both OFET devices is in the range of 102–103.
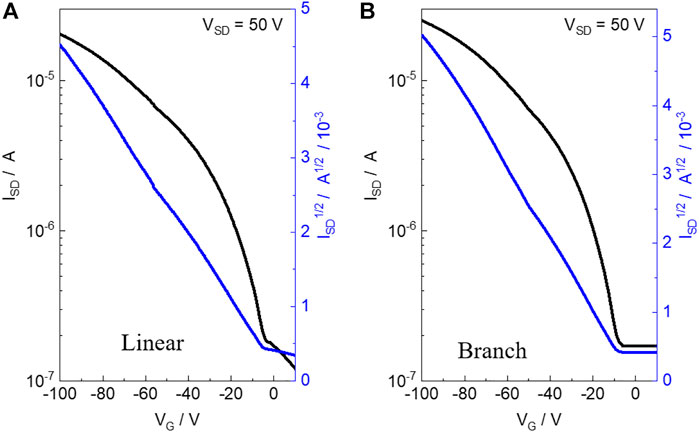
FIGURE 2. Transfer characteristics of the OFET devices based on linear polymer (A) and branch polymer (B).
Conclusion
In this work, two polymers, named linear and branch, are synthesized and characterized. These two polymers present almost the same polymer backbone and the chemical structures, except the side alkyl chains are linear or branch. The XRD as well as the optical absorption studies indicate that the linear polymer exhibits stronger aggregation than the branch one. Normally, strong aggregation is beneficial for charge transport between individual molecules. Thus, the linear polymer with high charge transport mobility is expected. However, the GPC measurement shows that the branch polymer has a high molecular weight, which might be due to the fact that the branch polymer exhibits improved solubility compared to linear ones. As a result, linear polymer presents lower hole transport mobility than the branch one, which indicates that molecular weight is more important than molecular aggregation ability when designing organic field-effect transistors for polymer semiconductors. This work paves the way for future molecular design to fabricate high-performing semiconductor polymer materials for a wide range of applications.
Data availability statement
The original contributions presented in the study are included in the article/Supplementary Material; further inquiries can be directed to the corresponding author.
Author contributions
DZ: investigation, formal analysis, data curation, writing—original draft preparation, and funding acquisition. DL: investigation, formal analysis, data curation, and writing—original draft preparation LG: data curation and formal analysis. JL: data curation and formal analysis. HZ: supervision, writing—reviewing and editing, project administration, and funding acquisition.
Funding
The authors acknowledge the support from Young Taishan Scholars under Grant 201909120, Natural Science Foundation of China (Grants: 52163001), Guizhou Province Science and Technology Plan Projects [Grants: CXTD(2021)005, GCC(2022)010-1, (2019)4022], Guiyang city expert workstation (Grant: ZJGZZ 2021-07), Baiyun District Science and Technology Plan Projects (Grants: (2020)28), and Polymer Composites Engineering Research Center of Guizhou Minzu University [Grant: GZMUGCZX (2021)01].
Conflict of interest
The authors declare that the research was conducted in the absence of any commercial or financial relationships that could be construed as a potential conflict of interest.
Publisher’s note
All claims expressed in this article are solely those of the authors and do not necessarily represent those of their affiliated organizations, or those of the publisher, the editors, and the reviewers. Any product that may be evaluated in this article, or claim that may be made by its manufacturer, is not guaranteed or endorsed by the publisher.
Supplementary material
The Supplementary Material for this article can be found online at: https://www.frontiersin.org/articles/10.3389/fchem.2022.1008807/full#supplementary-material
References
Cheon, H. J., An, T. K., and Kim, Y. H. (2022). Diketopyrrolopyrrole (DPP)-Based polymers and their organic field-effect transistor applications: A review. Macromol. Res. 2 (30), 71–84. doi:10.1007/s13233-022-0015-y
Cui, H. N., Qiu, F., and Peng, J. (2018). Synthesis and properties of an all-conjugated polythiophene-polyselenophene diblock copolymer. Acta chimi. Sin. 9 (76), 691–700. doi:10.6023/A18040178
Guo, X., Baumgarten, M., and Mullen, K. (2013). Designing pi-conjugated polymers for organic electronics. Prog. Polym. Sci. 12 (38), 1832–1908. doi:10.1016/j.progpolymsci.2013.09.005
Kang, S. H., Lee, D., Choi, W., Oh, J. H., and Yang, C. (2022). Usefulness of polar and bulky phosphonate chain-end solubilizing groups in polymeric semiconductors. Macromolecules 11 (55), 4367–4377. doi:10.1021/acs.macromol.1c02628
Liu, D. P., Chu, Y. L., Wu, X. H., and Huang, J. (2017). Side-chain effect of organic semiconductors in OFET-based chemical sensors. Sci. China Mat. 10 (60), 977–984. doi:10.1007/s40843-017-9121-y
Michael, S. (2021). Development of conjugated polymers for organic flexible electronics. Org. Flex. Electron. 2021, 27–70. doi:10.1016/B978-0-12-818890-3.00002-3
Moon, Y., LeeKim, C, H., and Kim, Y. (2018). Light-insensitive organic field-effect transistors with n-type conjugated polymers containing Dinitrothiophene units. Adv. Electron. Mat. 4 (11), 1800375. doi:10.1002/aelm.201800375
Nahid, M. M., Gann, E., Thomsen, L., and McNeill, C. R. (2016). NEXAFS spectroscopy of conjugated polymers. Eur. Polym. J. 81, 532–554. doi:10.1016/j.eurpolymj.2016.01.017
Ozdemir, R., Ahn, K., Deneme, I., Zorlu, Y., Kim, D., Kim, M. G., et al. (2020). Engineering functionalized low LUMO [1]benzothieno[3, 2-b] [1]benzothiophenes (BTBTs): Unusual molecular and charge transport properties. J. Mat. Chem. C Mat. 43 (8), 15253–15267. doi:10.1039/d0tc02945a
Ozdemir, R., Choi, D., Ozdemir, M., Kwon, G., Kim, H., Sen, U., et al. (2017). Ultralow bandgap molecular semiconductors for ambient-stable and solution-processable ambipolar organic field-effect transistors and inverters. J. Mat. Chem. C Mat. 9 (5), 2368–2379. doi:10.1039/c6tc05079d
Pan, Y., Lv, A. F., and Chi, L. F. (2018). Analysis of influencing factors on air-stable organic field-effect transistors (OFETs). ms. 2 (24), 148–158. doi:10.5755/j01.ms.24.2.18197
Pei, M., Kim, J. H., On, S., Lee, H. K., Cho, K., Hwang, D. H., et al. (2017). Coplanar donor-acceptor semiconducting copolymers to achieve better conjugated structures: Side-chain engineering. Macromol. Chem. Phys. 16, 1700135. doi:10.1002/macp.201700135
Qu, D. S., Qi, T., and Huang, H. (2021). Acceptor-acceptor-type conjugated polymer semiconductors. J. Energy Chem. 59, 364–387. doi:10.1016/j.jechem.2020.11.019
Ran, H. J., Li, F., Zheng, R., Zhang, H. L., Xie, F. L., Jin, P. C., et al. (2020). Polarity change of OFETs based on Dithienocoronene Diimide (DTCDI)-Derived isomeric triads end-capped with Azulene. Dyes Pigm. 203, 110311. doi:10.1016/j.dyepig.2022.110311
Raveendran, R., and Namboothiry, M. A. G. (2022). Bias stress stability and hysteresis in elastomeric dielectric based solution processed OFETs. Mat. Res. Bull. 146, 111596. doi:10.1016/j.materresbull.2021.111596
Wang, Q., Dong, X., He, M., Li, M. M., Tian, H. K., Liu, J., et al. (2018). Polythiophenes with carboxylate side chains and vinylene linkers in main chain for polymer solar cells. Polymer 140, 89–95. doi:10.1016/j.polymer.2018.02.035
Keywords: organic semiconductor, conjugated (conducting) polymers, polymer, donor–acceptor conjugated polymers, charge transport mobilities
Citation: Zhang D, Liang D, Gu L, Li J and Zhang H (2022) DPP-based polymers with linear/branch side chain for organic field-effect transistors. Front. Chem. 10:1008807. doi: 10.3389/fchem.2022.1008807
Received: 01 August 2022; Accepted: 23 August 2022;
Published: 13 September 2022.
Edited by:
Yue Liu, Liaoning Technical University, ChinaReviewed by:
Yalong Wang, Hainan University, ChinaXu Qiu, Shandong University of Science and Technology, China
Zhifeng Deng, Shaanxi University of Technology, China
Copyright © 2022 Zhang, Liang, Gu, Li and Zhang. This is an open-access article distributed under the terms of the Creative Commons Attribution License (CC BY). The use, distribution or reproduction in other forums is permitted, provided the original author(s) and the copyright owner(s) are credited and that the original publication in this journal is cited, in accordance with accepted academic practice. No use, distribution or reproduction is permitted which does not comply with these terms.
*Correspondence: Haichang Zhang, haichangzhang@hotmail.com
†These authors have contributed equally to this work