- State Key Laboratory Breeding Base of Green Pesticide and Agricultural Bioengineering, Key Laboratory of Green Pesticide and Agricultural Bioengineering, Ministry of Education, State-Local Joint Laboratory for Comprehensive Utilization of Biomass, Center for R&D of Fine Chemicals, Guizhou University, Guiyang, China
With the great adjustment of world industrialization and the continuous improvement of energy consumption requirements, the selective conversion of biomass-based platform molecules to high-value chemicals and biofuels has become one of the most important topics of current research. Catalysis is an essential approach to achieve energy-chemical conversion through the “bond breaking-bond formation” principle, which opens a broad world for the energy sector. Single-atom catalysts (SACs) are a new frontier in the field of catalysis in recent years, and exciting achievements have been made in biomass energy chemistry. This mini-review focuses on catalytic conversion of biomass-based levulinic acid (LA) to γ-valerolactone (GVL) over SACs. The current challenges and future development directions of SACs-mediated catalytic upgrading of biomass-based LA to produce value-added GVL, and the preparation and characterization of SACs are analyzed and summarized, aiming to provide theoretical guidance for further development of this emerging field.
Introduction
With advances in research and industrial technology, biomass is now increasingly deemed as one of the most valuable renewable resources that can be converted into a variety of platform molecules, fine chemicals, biofuels, and solid biochars (Li et al., 2015; Liu et al., 2015; Li et al., 2017; Li H et al., 2019; Li H et al., 2020; Meng and Li, 2021; Meng et al., 2022). γ-Valerolactone (GVL) is an important platform molecule and green solvent, which can be used to produce liquid fuels, polymers, intermediates for fine chemicals synthesis, and seasonings (Wright and Palkovits, 2012; Zhang, 2016; Ye et al., 2020). Catalytic hydrogenation of biomass-based levulinic acid (LA) is one of the most effective methods to produce GVL, which has attracted increasing attention in recent years. Homogeneous catalysts can be uniformly dispersed in the catalytic system, which can effectively promote LA hydrogenation to produce GVL. However, due to the high boiling point of GVL (207–208°C), the product/catalyst separated by distillation is not economical, resulting in a homogeneous system not suitable for the target production of GVL (Zhang, 2016). Therefore, the large-scale production of GVL almost certainly depends on heterogeneous catalysts, because heterogeneous catalysts are easier to be separated from nonvolatile GVL (Zhang, 2016; Zhang et al., 2019).
Metal sites play an important role in the hydrogenation, intramolecular (trans) esterification or dehydration of LA to obtain GVL (Dutta et al., 2019). Therefore, improving the utilization rate of metal atoms is the key step to reduce the production cost and obtain high efficiency in the whole catalytic process. Single-atom catalysts (SACs) are supported catalysts containing only isolated active centers (Chen et al., 2018; Ji et al., 2020), in which a strong interaction or considerable charge transfer between highly dispersed single metal atoms and solid supports. This unique structure allows single-metal atoms of the SACs to have a desired electronic structure and carry specific electrical charges different from those of conventional metal nanoparticles (Ding et al., 2019; Xue et al., 2022). It seems one of the most promising materials for rational utilization of metal resources and atomic economy. In theory, all the involved metal atoms could behave as homogeneously as homogeneous catalysts, with an atomic efficiency of 100% (Ding et al., 2019). SACs have shown excellent catalytic performance, with respect to activity, selectivity, and stability, in catalytic conversion of various biomass-derived feedstocks into target value-added chemicals (De et al., 2022).
Some excellent reviews have depicted the catalytic production of GVL from biomass-based platform molecule LA in different perspectives (Wright and Palkovits, 2012; Zhang, 2016; Ye et al., 2020), while SACs applied in this field have not been comprehensively discussed till now. This mini-review focuses on the preparation and characterization of SACs and their application in the conversion of LA to GVL. The advantages and disadvantages of SACs, and the catalytic activity of LA over SACs are introduced emphatically.
Catalytic Conversion of LA to GVL
LA is an important platform molecule, which can be easily and economically produced from lignocellulose via a simple and high-yield acid-catalyzed hydrolysis process (Figure 1) (Rackemann and Doherty, 2011; Ruppert et al., 2012; Khan et al., 2018). The condensation ability of LA is related to hydrogenation, and the generation of GVL may be realized through the hydrogenation of unsaturated carbon-carbon or carbon-oxygen bonds. Therefore, GVL can be synthesized by hydrogenation of LA through following two reaction mechanisms. 1) Hydrogenation of ketone group of LA to produce unstable intermediate 4-hydroxy-pentanoic acid (HPA), and subsequent dehydration followed by an intramolecular esterification, resulting in the ring closure to yield GVL. 2) LA is directly dehydrated to produce α-angelica lactone and then hydrogenated to produce GVL (Zhang, 2016; Dutta et al., 2019; Ye et al., 2020). In these two pathways, the hydrogenation step depends on the metal active sites of the catalyst, and the dehydration and cyclization steps are affected by the acidic conditions of the reaction system (Galletti et al., 2012; Lin et al., 2018; Cai et al., 2019). In this regard, improving the utilization rate of metal atoms is a key step to reducing the cost and obtaining high efficiency for the overall catalytic process.
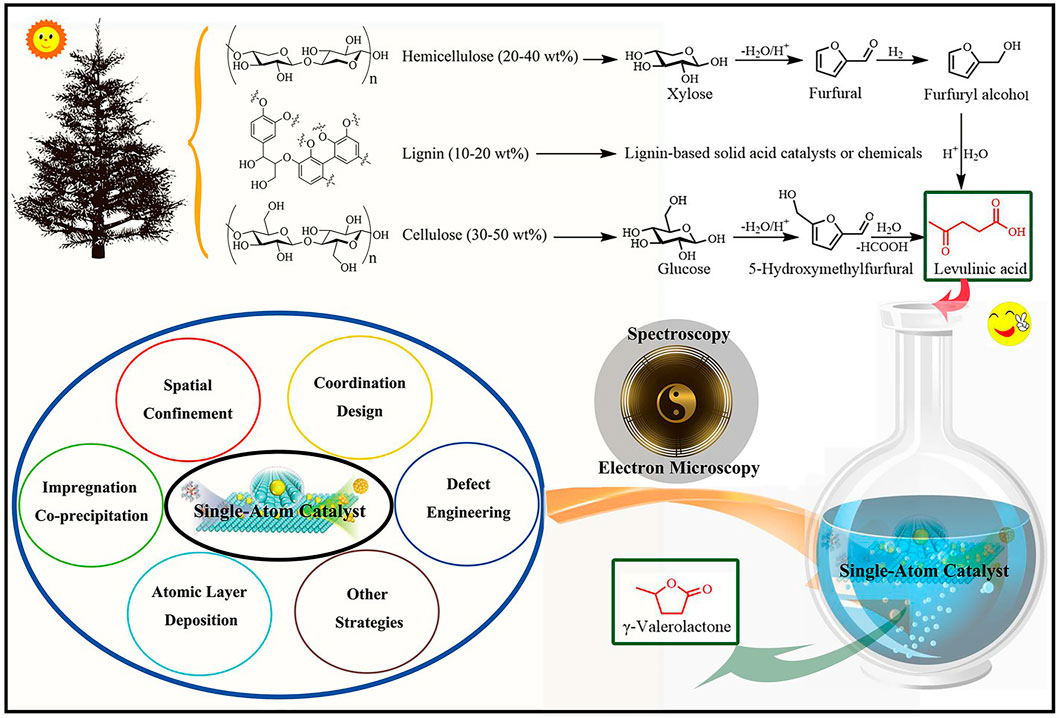
FIGURE 1. The schematic of reductive upgrading of biomass-based LA (levulinic acid) to GVL (γ-valerolactone) over single-atom catalysts.
In recent years, SACs have opened up a vast field of catalysis because of their advantages of high atomic utilization rate, high activity, high stability, and high selectivity. Many different active phases, including noble and non-noble metals, have been tested to catalyze the conversion of LA to GVL (Yan et al., 2013; Yuan et al., 2013; Mai et al., 2014). Ruthenium-based catalysts remain preferred because they are usually the most active and selective catalysts. Using metal-organic framework (MOF) NH2-MIL-125 as the precursor, Zhang et al. prepared Ru single-atom catalyst (Ru/TiO2@CN) by loading anatase and rutile mixed-phase TiO2 on nitrogen-doped amorphous carbon through high-temperature calcination (Zhang et al., 2021). At room temperature, the conversion of LA and selectivity of GVL could reach 100% through hydrogenation and dehydration reactions. The turnover frequency (TOF) of the catalyst was about 35 times higher than that of the industrial Ru/C catalyst, also with superior selectivity toward GVL. In addition, the mechanism study showed that LA is first converted to HPA through the hydrogenation process, and then HPA is rapidly dehydrated to GVL. The stability of 0.85 wt% Ru/ZrO2@C catalyst prepared by wet impregnation method for the conversion of LA to GVL was significantly improved (Cao et al., 2017). ZrO2@C support, with a nano t-ZrO2 (3.3 nm) structure embedded in amorphous carbon, was obtained by thermal decomposition of UiO-66 material (Zr-MOF). The catalytic performance of Ru/ZrO2@C in LA-to-GVL conversion was tested under 10 bar H2 at 150°C in an aqueous solution, and compared with that of commercial 5 wt% Ru/C. Both catalysts could achieve the full conversion of LA and the quantitative yield of GVL, while 5 wt% Ru/C exhibited poor deactivation resistance after the first operation. Inductively coupled plasma optical emission spectroscopy (ICP), X-ray photoelectron spectroscopy (XPS), high-resolution transmission electron microscopy (HR-TEM), aberration-corrected scanning transmission electron microscopy (AC-STEM), temperature-programmed reduction (TPR), and physical adsorption data showed that the rapid deactivation of Ru/C was mainly due to the leaching of Ru and the loss of surface area caused by carbon deposition in micropores (Cao et al., 2017). In contrast, the self-made Ru/ZrO2@C catalyst could be repeatedly recycled in water and high proton aqueous solution, with no significant decrease in catalytic performance. Yang et al. reported a single-atom catalyst (SAC) with a core-shell structure synthesized by core-shell bistability strategy using amine-modified Ru1/Fe3O4 as the core and periodic mesoporous organic silicon (PMO) as the shell (Yang et al., 2021). The Ru atom (0.76 wt%) was inserted into the oxygen vacancy of Fe3O4 spheres and stabilized by the amine group of 1,6-hexanediamine. Hollow PMO spheres are hydrophobic, which provides a strong barrier for the core of internal Ru1/Fe3O4, while the mesopores of the shell (4.2 nm) together with the cavity enhance the porosity of the catalyst. The conversion rate of LA was up to 99% with the GVL selectivity of up to 98.9%, while the high catalyst stability was still maintained after seven cycles. Regulating the electronic structure of metal catalysts can improve the catalyst performance, but it is very challenging work to regulate the electronic structure of atom-level catalysts. Fortunately, a RuCo single-atom alloy (SAA) catalyst was recently reported, in which precisely tailored electron-rich Ru atoms were confined to Co lattices, and ZIF-67 containing Ru was prepared by pyrolysis (Shao et al., 2021). The experimental study and calculation simulation showed that its activity was derived from the intrinsic active site of RuCo SAA. It was further illustrated that the electron-rich Ru single atom promoted the adsorption of C=O/H2 and the dissociation of H2 to H atom, especially beneficial to hydrogenation of the unsaturated γ-C of LA, which is the rate-determining step of LA hydrogenation. The more Ru content, the better the reaction activity of RuCo is for LA-to-GVL, and the TOF value could reach 3500 h−1, which is 27 times higher than that of commercial 5 wt% Ru/C catalyst. Han et al. also reported a strategy using notched polyoxomtalate (N-POM) to immobilize Ru atoms, which could prevent the aggregation of Ru during pyrolysis and obtain atom-dispersed Ru catalysts anchored onto uniform subsupporter WOx clusters on carbon-nitrogen (Ru1@WOx/CN) (Han et al., 2021). The synthesized Ru1@WOx/CN catalyst had good catalytic activity for the hydrogenation of LA to GVL under solvent-free conditions (99% conversion, and 100% selectivity). Properly regulating the active center of the Ru electronic structure can promote the formation and dehydration of HPA intermediates to form GVL. The N-POM strategy is also excellent in preparing a series of atom-dispersed noble metal atoms, which provides an opportunity to find SACs. The stability of the catalyst in polar solvents is also a very important indicator in the catalytic upgrading of the biomass platform molecules. The activity, selectivity, and stability of Ru-based catalysts supported on TiO2, ZrO2, and C in the conversion of GVL from LA at 30 bar H2 and 150°C were investigated (Ftouni et al., 2016). All the tested catalysts showed good GVL yield in fresh use, but only Ru/ZrO2 catalyst could maintain high yield in multiple cycles. Surprisingly, the widely used Ru/TiO2 catalyst showed rapid deactivation after the first catalytic test. The characterization structure showed that the partial deactivation of Ru was attributed to the reduction of Ti support and the coating of Ru nanoparticles, namely the interaction of harmful strong metal supports, rather than the sintering or coking of Ru. In contrast, the Zr support showed no signs of activity reduction after five cycles and had high morphological and structural stability. It is worth noting that in the fresh Ru/ZrO2 catalyst, even if the Ru loading is 1 wt%, Ru can still be completely dispersed on the fresh catalyst, and some Ru nanoparticles can be observed after recycling. Further studies on Ru/ZrO2 catalysts showed that the dioxane was easily replaced by milder solvents, including GVL itself. Recently, in addition to the SACs constructed with Ru as the active center, which shows excellent catalytic performance in the research system of LA-to-GVL conversion, Ir-based SACs have also come into the vision of researchers. Cao et al. first reported an ultra-stable Ir-based SAC (0.6 wt% Ir@ZrO2@C) (Cao et al., 2019). In polar and proton reaction media (pH = 3 and pH = 1) under harsh conditions (T = 155°C, PH2 = 40 bar), 2.7 wt% Ir/C and 0.6 wt% Ir/ZrO2 nanocatalysts showed significant deactivation in recycling experiments, mainly due to the leaching of Ir into acidic reaction media. In contrast, Ir@ZrO2@C SAC catalyst showed advantages in selectivity and unprecedented stability toward GVL, which could be recycled continuously seven times (pH = 3) and six times (pH = 1) in aqueous solution without deactivation and metal sintering or leaching. It is thus can be observed that the in-situ synthesis process that limits the entry of a single atom into the metal-organic framework by space proves to be an effective method for preparing acid-resistant solid catalysts.
Preparation and Characterization
The catalytic performance of SACs with a good structure and coordination environment is greatly improved, which is helpful for the identification of active sites and the study of catalytic mechanisms at an atomic level. Although SACs have made proud achievements in various catalytic fields such as thermal catalysis, photo-catalysis, electro-catalysis, and photoelectron-catalysis, the preparation of highly dispersed SACs still presents some challenges (Ji et al., 2020). Here, the synthesis strategies of SACs are summarized for a better understanding of the construction process of SACs to promote the application of SACs for LA-to-GVL (e.g., impregnation, co-precipitation, spatial confinement, coordination, and defect strategy) (Figure 1). As a typical preparation method, the impregnation and co-precipitation methods are of great significance for the preparation of SACs by controlling metal loadings and selecting suitable high surface area supports (Wei et al., 2014; Zhang X et al., 2018; Ye et al., 2019). For example, Ftouni et al. prepared two 1 wt% Ru catalysts supported on TiO2 and monoclinic ZrO2 by wet impregnation method (Ftouni et al., 2016). The spatial confinement strategy is to disperse single atoms in space using porous materials such as zeolites (Masoumifard et al., 2018; Liu L et al., 2020), metal-organic frameworks (MOFs) (Jiao and Jiang, 2019), and covalent organic frameworks (COFs) (Yi et al., 2018; Zhong et al., 2019). N-POM was used to confine Ru atoms to prepare highly dispersed Ru1@WOx/CN catalysts for producing GVL (Han et al., 2021). The coordination usually takes the target single atom as the coordination center, which then coordinates with the ligand containing lone pair electrons to form highly dispersed SACs. Ligands commonly used are polymers and polymer-derived materials with abundant heteroatoms (Pan et al., 2018; He et al., 2019), MOFs and their derivatives (Zhang et al., 2016; Yang et al., 2019), carbon-based materials (Li X et al., 2020), and C3N4 and graphdiyne (Tan et al., 2017). The defect strategy is to prepare materials with dispersed defect sites from relatively intact materials, followed by introducing single atoms into the defect sites to form highly dispersed SACs (Beniya and Higashi, 2019). These materials usually include oxides, hydroxides (Dvorak et al., 2016; Sun et al., 2019), graphene, and other deficiency-rich materials (Fei et al., 2019). The strategy of direct conversion of metal nanoparticles into SACs is to create suitable synthesis conditions to break the metal bond of nanoparticles and form a new bond between the single metal atom and the anchor chain of the support (Moliner et al., 2016). The “top-down” strategy is to directly convert metal block materials and metal oxide powers materials into SACs (Qu et al., 2018). Single-atom alloy strategy is dependent on the dispersion of metal single-atom sites into another metal nanomaterial (Greiner et al., 2018; Li M et al., 2019; Shao et al., 2021). Chemical etching strategies include direct etching of bulk metals and nanoparticles (Qiu et al., 2015), and indirect template-assisted etching into SACs (Han et al., 2017; Sun et al., 2018). Atomic layer deposition (ALD) technology has a good ability to control the deposition of single atom or cluster on the support, so it is one of the powerful methods to accurately prepare ideal single-atom materials (Sun et al., 2013; Zhang L et al., 2018). In addition, other preparation strategies that are not commonly used include photochemistry method (Karkas et al., 2016), electrochemistry method (Zhang et al., 2017), freezing-assisted method (Wei et al., 2017), microwave-assisted method (Bilecka and Niederberger, 2010), ball-milling method (Cui et al., 2016), ionic-liquid-assisted method (Xi and Sun, 2019), and so on.
With continuous efforts in recent years, researchers have developed a variety of effective synthesis strategies, as discussed above. In connection to this, how to reveal the isolated reactive centers and overall structural information of SACs is also a very important link. In this mini-review paper, the structural characterization of isolated reactive centers in SACs (especially the Ru-based SACs for LA-to-GVL conversion) was disclosed by using advanced technologies such as electron microscopy and spectroscopy (Figure 1) (Xue et al., 2022). Transmission electron microscopy (TEM) with the atomic resolution has been developed as an effective method to study the detailed structural information of isolated reaction centers and their interactions with supports. The additional energy dispersive X-ray (EDX) detector of scanning transmission electron microscopy (STEM) can further provide element mappings, clarify the atomic dispersion of metal atoms on supports, and further evaluate the dispersion degree of single atoms. In addition, aberration-corrected high-angle annular dark-field scanning transmission electron microscopy (AC-HAADF-STEM) can easily confirm the existence of isolated reaction centers on the supports, as long as the metal atoms exhibit a much higher atomic number than the supporting elements (Lee et al., 2019; Chen et al., 2020). Although electron microscope images provide effective information to identify the structural information of the catalysts, it should be noted that there are some limitations in its application in structural characterization. Due to the limited electron penetration ability of microscopic technology, it is difficult to observe the isolated metal atoms modified in the bulk phase or cavities, rather than the surface structure. In addition, the electron microscope can only image the local structure, and cannot provide the overall structure information of the SACs (Liu J et al., 2020). Therefore, some additional spectral methods are needed to provide supplementary data and support the existence of isolated metal sites in SACs. For example, X-ray photoelectron spectroscopy (XPS) is widely used to reveal the surface valence structure of SACs. Compared with pure metal, the obvious change of binding energy may explain the oxidation state of isolated metal atoms and exclude the existence of nanoparticles (Han et al., 2021; Shao et al., 2021). In addition, X-ray absorption spectroscopy (XAS) is one of the most commonly used and powerful tools to characterize SACs, including X-ray absorption near-edge structure (XANES) spectroscopy and the extended X-ray absorption fine structure (EXAFS) (Pan et al., 2019; Qiao et al., 2022). XANES can provide local electronic state information of the detected elements, while EXAFS can provide a high-resolution coordination environment and local geometry details of isolated metal sites. As site-specific characterization techniques, Fourier transform infrared spectroscopy (FTIR) and Raman spectroscopy are also widely used to evaluate the existence of isolated metal sites (Li et al., 2016; Fang et al., 2018), because they have obvious displacement relative to clusters or nanoparticles. The theoretical calculation also is one of the most important research methods (Han et al., 2021), state density, wave function |Ψ|2, and modeling can verify each other with electron microscopy and spectroscopy. Taking together, the above characterization methods are conducive to revealing the role of SACs in the LA-to-GVL conversion system, which provides an effective basis for the visualization of the system in the future.
Conclusion and Perspectives
The over-exploitation of fossil resources has caused serious social and environmental problems, and the high-value conversion of renewable resources has gradually become a global research upsurge. This mini-review focuses on the conversion of LA to GVL over SACs, and also extends to the involved reaction mechanism (e.g., hydrogenation, cyclization, and dehydration), synthesis methods (e.g., impregnation, co-precipitation, spatial confinement, self-assembling, and defect sites), and structural characterization methods (e.g., electron microscopy, and spectroscopy) of SACs. Both SACs and traditional heterogeneous catalysts show high activity and selectivity in the catalytic conversion of LA to GVL. However, traditional heterogeneous catalysts tend to lose activity after reaction, while SACs show excellent stability. At present, the SACs in this field mainly use Ru species as the active sites. Therefore, the development of SCAs with cheap transition metals as the active sites is one of the major tasks for LA-to-GVL, which is conducive to reducing the catalyst cost and promoting the commercialization of this field. In addition, the development of cleaner and more sustainable catalytic systems for LA-to-GVL is also a hot topic for future research, such as single-atom electro- and photocatalytic systems. In conclusion, SACs have great potential in large-scale catalytic production of GVL from LA. Further development of facile preparation methods of SACs and eco-friendly catalytic processes, as well as elucidation of the single-atom active center is challenging but an unprecedented opportunity to promote the industrialization of SACs for biomass valorization.
Author Contributions
YM organized and prepared this manuscript; YJ made preliminary revisions to the manuscript; DC and JH participated in literature collection and collation; HZ and HL supervised and contributed to reviewing the manuscript.
Funding
The study was funded by the National Natural Science Foundation of China (21908033), Guizhou Provincial S&T Project (ZK(2022)011, ZK(2022)141), the Fok Ying-Tong Education Foundation (161030), and GZU (Guizhou University) Found for Cultivation ((2020)73).
Conflict of Interest
The authors declare that the research was conducted in the absence of any commercial or financial relationships that could be construed as a potential conflict of interest.
Publisher’s Note
All claims expressed in this article are solely those of the authors and do not necessarily represent those of their affiliated organizations, or those of the publisher, the editors and the reviewers. Any product that may be evaluated in this article, or claim that may be made by its manufacturer, is not guaranteed or endorsed by the publisher.
References
Beniya, A., and Higashi, S. (2019). Towards Dense Single-Atom Catalysts for Future Automotive Applications. Nat. Catal. 2, 590–602. doi:10.1038/s41929-019-0282-y
Bilecka, I., and Niederberger, M. (2010). Microwave Chemistry for Inorganic Nanomaterials Synthesis. Nanoscale 2, 1358–1374. doi:10.1039/b9nr00377k
Cai, X., Xu, Q., Tu, G., Fu, Y., Zhang, F., and Zhu, W. (2019). Synergistic Catalysis of Ruthenium Nanoparticles and Polyoxometalate Integrated within Single UiO−66 Microcrystals for Boosting the Efficiency of Methyl Levulinate to γ-Valerolactone. Front. Chem. 7, 42. doi:10.3389/fchem.2019.00042
Cao, W., Lin, L., Qi, H., He, Q., Wu, Z., Wang, A., et al. (2019). In-situ Synthesis of Single-Atom Ir by Utilizing Metal-Organic Frameworks: An Acid-Resistant Catalyst for Hydrogenation of Levulinic Acid to γ-valerolactone. J. Catal. 373, 161–172. doi:10.1016/j.jcat.2019.03.035
Cao, W., Luo, W., Ge, H., Su, Y., Wang, A., and Zhang, T. (2017). UiO-66 Derived Ru/ZrO2@C as a Highly Stable Catalyst for Hydrogenation of Levulinic Acid to γ-valerolactone. Green. Chem. 19, 2201–2211. doi:10.1039/c7gc00512a
Chen, Y., Ji, S., Chen, C., Peng, Q., Wang, D., and Li, Y. (2018). Single-Atom Catalysts: Synthetic Strategies and Electrochemical Applications. Joule 2, 1242–1264. doi:10.1016/j.joule.2018.06.019
Chen, Y., Ji, S., Sun, W., Lei, Y., Wang, Q., Li, A., et al. (2020). Engineering the Atomic Interface with Single Platinum Atoms for Enhanced Photocatalytic Hydrogen Production. Angew. Chem. Int. Ed. 59, 1295–1301. doi:10.1002/anie.201912439
Cui, X., Xiao, J., Wu, Y., Du, P., Si, R., Yang, H., et al. (2016). A Graphene Composite Material with Single Cobalt Active Sites: A Highly Efficient Counter Electrode for Dye-Sensitized Solar Cells. Angew. Chem. Int. Ed. 55, 6708–6712. doi:10.1002/anie.201602097
De, S., Burange, A. S., and Luque, R. (2022). Conversion of Biomass-Derived Feedstocks into Value-Added Chemicals over Single-Atom Catalysts. Green. Chem. 24, 2267–2286. doi:10.1039/d1gc04285h
Ding, S., Hülsey, M. J., Pérez-Ramírez, J., and Yan, N. (2019). Transforming Energy with Single-Atom Catalysts. Joule 3, 2897–2929. doi:10.1016/j.joule.2019.09.015
Dutta, S., Yu, I. K. M., Tsang, D. C. W., Ng, Y. H., Ok, Y. S., Sherwood, J., et al. (2019). Green Synthesis of Gamma-Valerolactone (GVL) through Hydrogenation of Biomass-Derived Levulinic Acid Using Non-noble Metal Catalysts: A Critical Review. Chem. Eng. J. 372, 992–1006. doi:10.1016/j.cej.2019.04.199
Dvořák, F., Farnesi Camellone, M., Tovt, A., Tran, N.-D., Negreiros, F. R., Vorokhta, M., et al. (2016). Creating Single-Atom Pt-Ceria Catalysts by Surface Step Decoration. Nat. Commun. 7, 10801. doi:10.1038/ncomms10801
Fang, X., Shang, Q., Wang, Y., Jiao, L., Yao, T., Li, Y., et al. (2018). Single Pt Atoms Confined into a Metal-Organic Framework for Efficient Photocatalysis. Adv. Mater. 30, 1705112. doi:10.1002/adma.201705112
Fei, H., Dong, J., Chen, D., Hu, T., Duan, X., Shakir, I., et al. (2019). Single Atom Electrocatalysts Supported on Graphene or Graphene-like Carbons. Chem. Soc. Rev. 48, 5207–5241. doi:10.1039/c9cs00422j
Ftouni, J., Muñoz-Murillo, A., Goryachev, A., Hofmann, J. P., Hensen, E. J. M., Lu, L., et al. (2016). ZrO2 Is Preferred over TiO2 as Support for the Ru-Catalyzed Hydrogenation of Levulinic Acid to γ-Valerolactone. ACS Catal. 6, 5462–5472. doi:10.1021/acscatal.6b00730
Galletti, A. M. R., Antonetti, C., De Luise, V., and Martinelli, M. (2012). A Sustainable Process for the Production of γ-valerolactone by Hydrogenation of Biomass-Derived Levulinic Acid. Green. Chem. 14, 688. doi:10.1039/c2gc15872h
Greiner, M. T., Jones, T. E., Beeg, S., Zwiener, L., Scherzer, M., Girgsdies, F., et al. (2018). Free-atom-like D States in Single-Atom alloy Catalysts. Nat. Chem 10, 1008–1015. doi:10.1038/s41557-018-0125-5
Han, Y., Dai, J., Xu, R., Ai, W., Zheng, L., Wang, Y., et al. (2021). Notched-Polyoxometalate Strategy to Fabricate Atomically Dispersed Ru Catalysts for Biomass Conversion. ACS Catal. 11, 2669–2675. doi:10.1021/acscatal.0c04006
Han, Y., Wang, Y.-G., Chen, W., Xu, R., Zheng, L., Zhang, J., et al. (2017). Hollow N-Doped Carbon Spheres with Isolated Cobalt Single Atomic Sites: Superior Electrocatalysts for Oxygen Reduction. J. Am. Chem. Soc. 139, 17269–17272. doi:10.1021/jacs.7b10194
He, X., He, Q., Deng, Y., Peng, M., Chen, H., Zhang, Y., et al. (2019). A Versatile Route to Fabricate Single Atom Catalysts with High Chemoselectivity and Regioselectivity in Hydrogenation. Nat. Commun. 10, 3663. doi:10.1038/s41467-019-11619-6
Ji, S., Chen, Y., Wang, X., Zhang, Z., Wang, D., and Li, Y. (2020). Chemical Synthesis of Single Atomic Site Catalysts. Chem. Rev. 120, 11900–11955. doi:10.1021/acs.chemrev.9b00818
Jiao, L., and Jiang, H.-L. (2019). Metal-Organic-Framework-Based Single-Atom Catalysts for Energy Applications. Chem 5, 786–804. doi:10.1016/j.chempr.2018.12.011
Kärkäs, M. D., Porco, J. A., and Stephenson, C. R. J. (2016). Photochemical Approaches to Complex Chemotypes: Applications in Natural Product Synthesis. Chem. Rev. 116, 9683–9747. doi:10.1021/acs.chemrev.5b00760
Khan, A. S., Man, Z., Bustam, M. A., Nasrullah, A., Ullah, Z., Sarwono, A., et al. (2018). Efficient Conversion of Lignocellulosic Biomass to Levulinic Acid Using Acidic Ionic Liquids. Carbohydr. Polym. 181, 208–214. doi:10.1016/j.carbpol.2017.10.064
Lee, B.-H., Park, S., Kim, M., Sinha, A. K., Lee, S. C., Jung, E., et al. (2019). Reversible and Cooperative Photoactivation of Single-Atom Cu/TiO2 Photocatalysts. Nat. Mater. 18, 620–626. doi:10.1038/s41563-019-0344-1
Li, H., Guo, H., Fang, Z., Aida, T. M., and Smith, R. L. (2020). Cycloamination Strategies for Renewable N-Heterocycles. Green. Chem. 22, 582–611. doi:10.1039/c9gc03655e
Li, H., Li, Y., Fang, Z., and Smith, R. L. (2019). Efficient Catalytic Transfer Hydrogenation of Biomass-Based Furfural to Furfuryl Alcohol with Recycable Hf-Phenylphosphonate Nanohybrids. Catal. Today 319, 84–92. doi:10.1016/j.cattod.2018.04.056
Li, H., Saravanamurugan, S., Yang, S., and Riisager, A. (2015). Catalytic Alkylation of 2-Methylfuran with Formalin Using Supported Acidic Ionic Liquids. ACS Sustain. Chem. Eng. 3, 3274–3280. doi:10.1021/acssuschemeng.5b00850
Li, H., Zhao, W., and Fang, Z. (2017). Hydrophobic Pd Nanocatalysts for One-Pot and High-Yield Production of Liquid Furanic Biofuels at Low Temperatures. Appl. Catal. B: Environ. 215, 18–27. doi:10.1016/j.apcatb.2017.05.039
Li, M., Duanmu, K., Wan, C., Cheng, T., Zhang, L., Dai, S., et al. (2019). Single-atom Tailoring of Platinum Nanocatalysts for High-Performance Multifunctional Electrocatalysis. Nat. Catal. 2, 495–503. doi:10.1038/s41929-019-0279-6
Li, X., Rong, H., Zhang, J., Wang, D., and Li, Y. (2020). Modulating the Local Coordination Environment of Single-Atom Catalysts for Enhanced Catalytic Performance. Nano Res. 13, 1842–1855. doi:10.1007/s12274-020-2755-3
Li, Y., Wang, Z., Xia, T., Ju, H., Zhang, K., Long, R., et al. (2016). Implementing Metal-To-Ligand Charge Transfer in Organic Semiconductor for Improved Visible-Near-Infrared Photocatalysis. Adv. Mater. 28, 6959–6965. doi:10.1002/adma.201601960
Lin, Z., Luo, M., Zhang, Y., Wu, X., Fu, Y., Zhang, F., et al. (2018). Coupling Ru Nanoparticles and Sulfonic Acid Moieties on Single MIL-101 Microcrystals for Upgrading Methyl Levulinate into γ-valerolactone. Appl. Catal. A: Gen. 563, 54–63. doi:10.1016/j.apcata.2018.06.027
Liu, J., Li, H., Liu, Y.-C., Lu, Y.-M., He, J., Liu, X.-F., et al. (2015). Catalytic Conversion of Glucose to 5-hydroxymethylfurfural over Nano-Sized Mesoporous Al2O3-B2o3 Solid Acids. Catal. Commun. 62, 19–23. doi:10.1016/j.catcom.2015.01.008
Liu, J., Wu, H., Li, F., Feng, X., Zhang, P., and Gao, L. (2020). Recent Progress in Non‐Precious Metal Single Atomic Catalysts for Solar and Non‐Solar Driven Hydrogen Evolution Reaction. Adv. Sustain. Syst. 4, 2000151. doi:10.1002/adsu.202000151
Liu, L., Wang, N., Zhu, C., Liu, X., Zhu, Y., Guo, P., et al. (2020). Direct Imaging of Atomically Dispersed Molybdenum that Enables Location of Aluminum in the Framework of Zeolite ZSM‐5. Angew. Chem. Int. Ed. 59, 819–825. doi:10.1002/anie.201909834
Mai, E. F., Machado, M. A., Davies, T. E., Lopez-Sanchez, J. A., and Teixeira da Silva, V. (2014). Molybdenum Carbide Nanoparticles within Carbon Nanotubes as superior Catalysts for γ-valerolactone Production via Levulinic Acid Hydrogenation. Green. Chem. 16, 4092–4097. doi:10.1039/c4gc00920g
Masoumifard, N., Guillet-Nicolas, R., and Kleitz, F. (2018). Synthesis of Engineered Zeolitic Materials: From Classical Zeolites to Hierarchical Core-Shell Materials. Adv. Mater. 30, 1704439. doi:10.1002/adma.201704439
Meng, Y., and Li, H. (2021). Electrocatalytic Oxidation of Biomass-Derived 5-Hydroxymethylfurfural to 2,5-Furandicarboxylic Acid Coupled with H2 Evolution. Curr. Org. Chem. 25, 2810–2814. doi:10.2174/1385272825666210927101643
Meng, Y., Yang, S., and Li, H. (2022). Electro‐ and Photocatalytic Oxidative Upgrading of Bio‐based 5‐Hydroxymethylfurfural. ChemSusChem e202102581. doi:10.1002/cssc.202102581
Moliner, M., Gabay, J. E., Kliewer, C. E., Carr, R. T., Guzman, J., Casty, G. L., et al. (2016). Reversible Transformation of Pt Nanoparticles into Single Atoms inside High-Silica Chabazite Zeolite. J. Am. Chem. Soc. 138, 15743–15750. doi:10.1021/jacs.6b10169
Pan, Y., Chen, Y., Wu, K., Chen, Z., Liu, S., Cao, X., et al. (2019). Regulating the Coordination Structure of Single-Atom Fe-NxCy Catalytic Sites for Benzene Oxidation. Nat. Commun. 10, 4290. doi:10.1038/s41467-019-12362-8
Pan, Y., Liu, S., Sun, K., Chen, X., Wang, B., Wu, K., et al. (2018). A Bimetallic Zn/Fe Polyphthalocyanine‐Derived Single‐Atom Fe‐N 4 Catalytic Site:A Superior Trifunctional Catalyst for Overall Water Splitting and Zn-Air Batteries. Angew. Chem. Int. Ed. 57, 8614–8618. doi:10.1002/anie.201804349
Qiao, S., He, Q., Zhang, P., Zhou, Y., Chen, S., Song, L., et al. (2022). Synchrotron-radiation Spectroscopic Identification towards Diverse Local Environments of Single-Atom Catalysts. J. Mater. Chem. A. 10, 5771–5791. doi:10.1039/d1ta08254j
Qiu, H.-J., Ito, Y., Cong, W., Tan, Y., Liu, P., Hirata, A., et al. (2015). Nanoporous Graphene with Single-Atom Nickel Dopants: An Efficient and Stable Catalyst for Electrochemical Hydrogen Production. Angew. Chem. Int. Ed. 54, 14031–14035. doi:10.1002/anie.201507381
Qu, Y., Li, Z., Chen, W., Lin, Y., Yuan, T., Yang, Z., et al. (2018). Direct Transformation of Bulk Copper into Copper Single Sites via Emitting and Trapping of Atoms. Nat. Catal. 1, 781–786. doi:10.1038/s41929-018-0146-x
Rackemann, D. W., and Doherty, W. O. (2011). The Conversion of Lignocellulosics to Levulinic Acid. Biofuels, Bioprod. Bioref. 5, 198–214. doi:10.1002/bbb.267
Ruppert, A. M., Weinberg, K., and Palkovits, R. (2012). Hydrogenolysis Goes Bio: from Carbohydrates and Sugar Alcohols to Platform Chemicals. Angew. Chem. Int. Ed. 51, 2564–2601. doi:10.1002/anie.201105125
Shao, S., Yang, Y., Sun, K., Yang, S., Li, A., Yang, F., et al. (2021). Electron-Rich Ruthenium Single-Atom Alloy for Aqueous Levulinic Acid Hydrogenation. ACS Catal. 11, 12146–12158. doi:10.1021/acscatal.1c03004
Sun, S., Zhang, G., Gauquelin, N., Chen, N., Zhou, J., Yang, S., et al. (2013). Single-atom Catalysis Using Pt/Graphene Achieved through Atomic Layer Deposition. Sci. Rep. 3, 175–183. doi:10.1038/srep01775
Sun, T., Zhao, S., Chen, W., Zhai, D., Dong, J., Wang, Y., et al. (2018). Single-atomic Cobalt Sites Embedded in Hierarchically Ordered Porous Nitrogen-Doped Carbon as a superior Bifunctional Electrocatalyst. Proc. Natl. Acad. Sci. U.S.A. 115, 12692–12697. doi:10.1073/pnas.1813605115
Sun, X., Chen, C., Liu, S., Hong, S., Zhu, Q., Qian, Q., et al. (2019). Aqueous CO 2 Reduction with High Efficiency Using α‐Co(OH) 2 ‐Supported Atomic Ir Electrocatalysts. Angew. Chem. Int. Ed. 58, 4669–4673. doi:10.1002/anie.201900981
Tan, C., Cao, X., Wu, X.-J., He, Q., Yang, J., Zhang, X., et al. (2017). Recent Advances in Ultrathin Two-Dimensional Nanomaterials. Chem. Rev. 117, 6225–6331. doi:10.1021/acs.chemrev.6b00558
Wei, H., Huang, K., Wang, D., Zhang, R., Ge, B., Ma, J., et al. (2017). Iced Photochemical Reduction to Synthesize Atomically Dispersed Metals by Suppressing Nanocrystal Growth. Nat. Commun. 8, 1490. doi:10.1038/s41467-017-01521-4
Wei, H., Liu, X., Wang, A., Zhang, L., Qiao, B., Yang, X., et al. (2014). FeOx-supported Platinum Single-Atom and Pseudo-single-atom Catalysts for Chemoselective Hydrogenation of Functionalized Nitroarenes. Nat. Commun. 5, 5634. doi:10.1038/ncomms6634
Wright, W. R. H., and Palkovits, R. (2012). Development of Heterogeneous Catalysts for the Conversion of Levulinic Acid to γ-Valerolactone. ChemSusChem 5, 1657–1667. doi:10.1002/cssc.201200111
Xi, B., and Sun, X. (2019). Single-Atom Catalysts Electrostatically Stabilized by Ionic Liquids. Chem 5, 3012–3014. doi:10.1016/j.chempr.2019.11.013
Xue, Z.-H., Luan, D., Zhang, H., and Lou, X. W. (2022). Single-atom Catalysts for Photocatalytic Energy Conversion. Joule 6, 92–133. doi:10.1016/j.joule.2021.12.011
Yan, K., Lafleur, T., Wu, G., Liao, J., Ceng, C., and Xie, X. (2013). Highly Selective Production of Value-Added γ-valerolactone from Biomass-Derived Levulinic Acid Using the Robust Pd Nanoparticles. Appl. Catal. A: Gen. 468, 52–58. doi:10.1016/j.apcata.2013.08.037
Yang, D., Yu, H., He, T., Zuo, S., Liu, X., Yang, H., et al. (2019). Visible-light-switched Electron Transfer over Single Porphyrin-Metal Atom center for Highly Selective Electroreduction of Carbon Dioxide. Nat. Commun. 10, 3844. doi:10.1038/s41467-019-11817-2
Yang, Y., Yang, F., Wang, H., Zhou, B., and Hao, S. (2021). Amine-promoted Ru1/Fe3O4 Encapsulated in Hollow Periodic Mesoporousorganosilica Sphere as a Highly Selective and Stable Catalyst for Aqueous Levulinic Acid Hydrogenation. J. Colloid Interf. Sci. 581, 167–176. doi:10.1016/j.jcis.2020.07.114
Ye, L., Duan, X., Wu, S., Wu, T.-S., Zhao, Y., Robertson, A. W., et al. (2019). Self- Regeneration of Au/CeO2 Based Catalysts with Enhanced Activity and Ultra-stability for Acetylene Hydrochlorination. Nat. Commun. 10, 914. doi:10.1038/s41467-019-08827-5
Ye, L., Han, Y., Feng, J., and Lu, X. (2020). A Review about GVL Production from Lignocellulose: Focusing on the Full Components Utilization. Ind. Crops Prod. 144, 112031. doi:10.1016/j.indcrop.2019.112031
Yi, J.-D., Xu, R., Wu, Q., Zhang, T., Zang, K.-T., Luo, J., et al. (2018). Atomically Dispersed Iron-Nitrogen Active Sites within Porphyrinic Triazine-Based Frameworks for Oxygen Reduction Reaction in Both Alkaline and Acidic Media. ACS Energ. Lett. 3, 883–889. doi:10.1021/acsenergylett.8b00245
Yuan, J., Li, S.-S., Yu, L., Liu, Y.-M., Cao, Y., He, H.-Y., et al. (2013). Copper-based Catalysts for the Efficient Conversion of Carbohydrate Biomass into γ-valerolactone in the Absence of Externally Added Hydrogen. Energy Environ. Sci. 6, 3308. doi:10.1039/c3ee40857d
Zhang, H., Li, H., Hu, Y., Venkateswara Rao, K. T., Xu, C., and Yang, S. (2019). Advances in Production of Bio-Based Ester Fuels with Heterogeneous Bifunctional Catalysts. Renew. Sustain. Energ. Rev. 114, 109296. doi:10.1016/j.rser.2019.109296
Zhang, H., Wei, J., Dong, J., Liu, G., Shi, L., An, P., et al. (2016). Efficient Visible-Light-Driven Carbon Dioxide Reduction by a Single-Atom Implanted Metal-Organic Framework. Angew. Chem. Int. Ed. 55, 14310–14314. doi:10.1002/anie.201608597
Zhang, K., Meng, Q., Wu, H., Yuan, T., Han, S., Zhai, J., et al. (2021). Levulinic Acid Hydrogenation to γ-valerolactone over Single Ru Atoms on a TiO2@nitrogen Doped Carbon Support. Green. Chem. 23, 1621–1627. doi:10.1039/d0gc04108d
Zhang, L., Banis, M. N., and Sun, X. (2018). Single-atom Catalysts by the Atomic Layer Deposition Technique. Natl. Sci. Rev. 5, 628–630. doi:10.1093/nsr/nwy054
Zhang, L., Han, L., Liu, H., Liu, X., and Luo, J. (2017). Potential-Cycling Synthesis of Single Platinum Atoms for Efficient Hydrogen Evolution in Neutral Media. Angew. Chem. Int. Ed. 56, 13694–13698. doi:10.1002/anie.201706921
Zhang, X., Sun, Z., Wang, B., Tang, Y., Nguyen, L., Li, Y., et al. (2018). C-C Coupling on Single-Atom-Based Heterogeneous Catalyst. J. Am. Chem. Soc. 140, 954–962. doi:10.1021/jacs.7b09314
Zhang, Z. (2016). Synthesis of γ-Valerolactone from Carbohydrates and its Applications. ChemSusChem 9, 156–171. doi:10.1002/cssc.201501089
Keywords: biomass, value-added chemicals, γ-Valerolactone, single-atom catalysts (SACs), levulinic acid (LA)
Citation: Meng Y, Jian Y, Chen D, Huang J, Zhang H and Li H (2022) Reductive Upgrading of Biomass-Based Levulinic Acid to γ-Valerolactone Over Ru-Based Single-Atom Catalysts. Front. Chem. 10:895198. doi: 10.3389/fchem.2022.895198
Received: 13 March 2022; Accepted: 18 March 2022;
Published: 01 April 2022.
Edited by:
Xianxiang Liu, Hunan Normal University, ChinaReviewed by:
Ping Zhu, Technical University of Denmark, DenmarkFumin Zhang, Zhejiang Normal University, China
Copyright © 2022 Meng, Jian, Chen, Huang, Zhang and Li. This is an open-access article distributed under the terms of the Creative Commons Attribution License (CC BY). The use, distribution or reproduction in other forums is permitted, provided the original author(s) and the copyright owner(s) are credited and that the original publication in this journal is cited, in accordance with accepted academic practice. No use, distribution or reproduction is permitted which does not comply with these terms.
*Correspondence: Heng Zhang, aHpoYW5nMjNAZ3p1LmVkdS5jbg==; Hu Li, aGxpMTNAZ3p1LmVkdS5jbg==