- 1Department of Chemistry and Industrial Chemistry, University of Genova, Genoa, Italy
- 2Coimbra Chemistry Centre-Institute of Molecular Sciences, Department of Chemistry, University of Coimbra, Coimbra, Portugal
- 3Department of Biotechnology, Chemistry and Pharmacy, University of Siena, Siena, Italy
Nitrosoalkenes react with 8-methyl-1,6-dihydropyrrolo[3,2-c]carbazole to give both 2- and 3-alkylated products via hetero-Diels-Alder reaction followed by the cycloadduct ring-opening. Quantum chemical calculations, at DFT level of theory, were carried out to investigate the regioselectivity of the cycloaddition of ethyl nitrosoacrylate with 1,6-dihydropyrrolo[3,2-c]carbazoles as well as with pyrrole and indole, allowing a more comprehensive analysis of the reactivity pattern of nitrosoalkenes with five-membered heterocycles. Furthermore, theoretical calculations confirmed that ethyl nitrosoacrylate reacts with these heterocycles via a LUMOheterodiene-HOMOdienophile controlled cycloaddition. The reactivity of one of the oxime-functionalized 1,6-dihydropyrrolo[3,2-c]carbazole was explored and a new hexahydropyrido[4′,3':4,5]pyrrolo[3,2-c]carbazole system was obtained in high yield via a one-pot, two-step procedure.
Introduction
The chemistry of conjugated nitrosoalkenes has been extensively studied for the synthesis and functionalization of a plethora of heterocyclic systems. These reactive intermediates act mainly as electron-deficient heterodienes in inverse electron-demand hetero-Diels-Alder reactions or as Michael-type acceptors in conjugated 1,4-addition reactions (Lopes et al., 2018a; Naumovich et al., 2019; Weinreb, 2019; Soares et al., 2022). In recent years, our research group has explored the reactivity and versatility of conjugated nitrosoalkenes as a synthetic tool to achieve heterocyclic structural diversity (Lopes et al., 2018a). Functionalization of five-membered heterocycles, such as pyrroles, dipyrromethanes, indoles and furans, was achieved by reaction with nitrosoalkenes, including the synthesis of 3-tetrazolyl and 3-triazolyl derivatives (Lopes et al., 2010; Lopes et al., 2011; Nunes et al., 2014; Lopes et al., 2015; Lopes et al., 2016; Alves et al., 2017; Jorda et al., 2017; Lopes et al., 2018b). One-pot methods have also been developed for the synthesis of dipyrromethanes (Pereira et al., 2014; Cardoso et al., 2021), bis(indolyl)methanes (Grosso et al., 2015; Grosso et al., 2017; Grosso et al., 2019), bis(pyrazol-1-yl)methanes (Grosso et al., 2014) and tetrapyrrolic compounds (Lopes and Pinho e Melo, 2020) via two consecutive hetero-Diels-Alder reactions (or conjugated additions) of in situ generated nitrosoalkenes with pyrroles, indoles, pyrazoles and dipyrromethanes, respectively. Moreover, tryptophan analogues have been obtained by reducing the oxime moiety of indoles C3-functionalized via hetero-Diels-Alder reactions with nitrosoalkenes. These tryptamine derivatives were used in the synthesis of 3-triazolyl- and 3-tetrazolyl-β-carbolines via Pictet–Spengler condensation followed by an oxidative step. ß-Carboline derivatives obtained by this strategy have shown interesting anticancer properties (Panice et al., 2019; Ribeiro et al., 2021; Ribeiro et al., 2022).
The reactivity of nitrosoalkenes with electron-rich heterocycles is strongly influenced not only by the nitrosoalkene substituents but also by the type of heterocycle (Nunes et al., 2014; Lopes et al., 2016). The pioneer work of Gilchrist and co-workers showed that the reaction of ethyl nitrosoacrylate (2, R = CO2Et), generated from ethyl bromopyruvate oxime 1a by action of base, with pyrrole and indole afforded the open chain oximes 4 and 8, respectively, through hetero-Diels-Alder reactions (Scheme 1) (Gilchrist and Roberts, 1983; Gilchrist and Lemos, 1993). The outcome and mechanistic pathway of the reaction of nitrosoalkenes with pyrrole and pyrrole derivatives depends on the nitrosoalkene substituent, as shown by experimental and theoretical studies (Nunes et al., 2014). In fact, ethyl nitrosoacrylate (2, R = CO2Et) reacts via hetero-Diels-Alder reaction, through the formation of the bicyclic 1,2-oxazine 3 followed by 1,2-oxazine ring-opening with concomitant rearomatization of the pyrrole unit, giving the open chain oxime 4, as a single isomer. However, aryl nitrosoalkenes 2 (R = Aryl) react with pyrrole by conjugated addition to give two isomeric oximes 5 and 6. On the other hand, the reaction of both nitrosoalkenes 2 with indole affords open chain oximes as single isomers via hetero-Diels-Alder reaction (Lopes et al., 2016). Furthermore, nitrosoalkenes 2 react with pyrrole to give 2-alkylated products, whereas indole undergoes alkylation at the 3-position, as would be expected from the opposite regioselectivity of the hetero-Diels-Alder reaction.
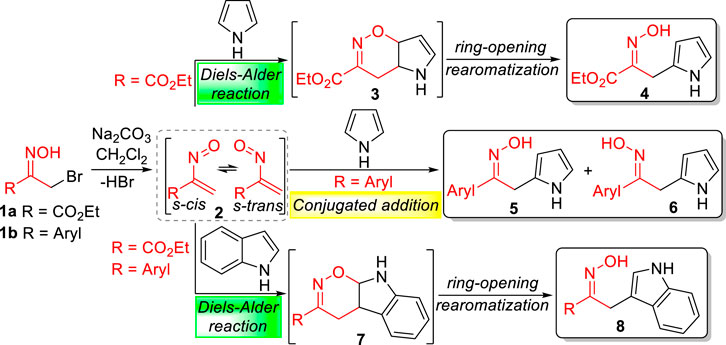
SCHEME 1. Conjugated 1,4-addition and hetero-Diels-Alder reactions of nitrosoalkenes with heterocycles.
Pyrrolocarbazoles are tetracyclic ring systems containing a pyrrole ring fused to a carbazole unit. Depending on the position of the pyrrole/carbazole ring junction and the relative position of the pyrrole nitrogen to the carbazole moiety, several structural isomers can be found (Giraud et al., 2019). The best known are the pyrrolo[2,3-c]carbazoles (Zhang and Ready, 2017), mainly because their core structure is present in the marine natural product dictyodendrin A, and pyrrolo[2,3-a]carbazoles for the recognized kinase inhibitory activity of some derivatives (Akué-Gédu et al., 2009; Giraud et al., 2012) (Figure 1). Reports on the synthesis and reactivity of pyrrolo[3,2-c]carbazoles are scarce. Recently, however, a few reports describing the synthesis (Benzi et al., 2019), photophysical and biological activity, namely, antioxidant (Bingul et al., 2019), anticancer and antibacterial activity, have been disclosed (Sengul et al., 2016; Saglam et al., 2021; Sinicropi et al., 2021). Compound 9 is an example of a pyrrolo[3,2-c]carbazole with high cytotoxicity against human colon cancer HT29 cells (Sengul et al., 2016).
Compounds containing an oxime moiety have found a wide range of biological applications, displaying anti-inflammatory, antimicrobial, antioxidant and anticancer activity (Surowiak et al., 2020). In this context, and following our interest in the chemistry of nitrosoalkenes, we decided to explore the reactivity of nitrosoalkenes towards 8-methyl-1,6-dihydropyrrolo[3,2-c]carbazole (10) aiming at the synthesis of oxime-functionalized pyrrolo[3,2-c]carbazoles. The combination of these two structural elements in a single molecule would lead to new chemical entities with increased interest in terms of potential biological activity and with the possibility of further structural modulation.
Results and discussion
Initially, the reaction of ethyl nitrosoacrylate (2a), generated in situ from ethyl bromopyruvate oxime (1a) by action of sodium carbonate, with pyrrolo[3,2-c]carbazole 10 was explored. The reaction, carried out in dichloromethane at room temperature, gave the 3- and 2-alkylated pyrrolo[3,2-c]carbazoles 11a and 12a, respectively, in 63% overall yield (Scheme 2). The same reactivity pattern was observed in the reaction of the less activated nitrosoalkene 2b with 10 affording the open chain oximes 11b and 12b in 37% and 8%, respectively (Scheme 2). In both cases, the regioisomeric open chain oximes were isolated as single stereoisomers, indicating that these were formed via hetero-Diels-Alder reaction followed by 1,2-oxazine ring-opening and concomitant rearomatization of the pyrrole unit.
The structural assignment of the two regioisomers was established by two-dimensional NMR spectroscopy. From the coupling observed in the COSY spectrum of compound 11a, it was possible to assign the signals corresponding to the protons H-2 (7.14 ppm), H-1 (10.49 ppm), H-4 (4.15 ppm) and the proton of the hydroxyimino moiety (11.35 ppm). The stereochemistry of the C,N-bond was established by the NOESY spectrum data, in which cross peaks were observed between the NOH proton and the H-4 protons, confirming the trans orientation of the OH and ester groups. The NOESY spectra of compounds 11b and 12b also showed a correlation between the proton of the oxime moiety and H-4 protons (see Supplementary Material), suggesting the trans orientation of the OH and ester groups.
Preliminary studies on the reactivity of the new 3-alkylated pyrrolo[3,2-c]carbazoles focused on the interconversion of the oxime-amine functional groups. However, the reduction reaction of pyrrolo[3,2-c]carbazole 11a, using zinc in acetic acid at room temperature, led to an unexpected but interesting result. One product was isolated whose 1H NMR spectrum features, recorded using acetone-d6 as solvent, were not those expected for the desired amine 13. From the analysis of the 1H NMR spectrum it was possible to confirm the presence of the pyrrolo[3,2-c]carbazole scaffold, signals corresponding to the ester group as well as to an ABX system, as it would be for amine 13. It was observed that when the product of the reduction reaction was treated with acetone for 1 h, a compound was isolated in 17% yield with a similar 1H NMR spectrum but in which the presence of two methyl groups could be observed (Scheme 3).
The structure of this new compound was unambiguously determined by X-ray crystallography as being hexahydropyrido [4′,3':4,5]pyrrolo[3,2-c]carbazole 14 (Scheme 3). This heterocyclic compound crystallized with an ethanol and water molecule as colourless plates in the triclinic crystal system within the P-1 space group, showing one molecule of 14⋅H2O⋅C2H5OH per asymmetric unit. Its molecular structure consists of a five-fused ring system, in which a piperidine ring is fused to the 1,6-dihydropyrrolo[3,2-c]carbazole moiety. This six-membered heterocycle contains two methyl groups at C (19) and an ester substituent at C (18). Bond lengths and bond angles are normal. The 1,6-dihydropyrrolo[3,2-c]carbazole moiety is almost planar with the largest deviations from the least-squares plane shown by C (9) (0.091 (4)Å), C (7) (0.086 (3)Å) and C (5) (0.081 (3)Å). In the tetrahydropyridine moiety the atoms C (18) and N (3) show a statistical disorder with two different positions with site occupation factors refined to 0.671) (conformer A) and 0.331) (conformer B). Ring puckering analysis (Cremer and Pople, 1975) of the tetrahydropyridine ring shows the following parameters: conformer A: θ = 51.27°, φ = 32.08°, total puckering amplitudes (QT) 0.45 Å; conformer B: θ = 48.51°, φ = 32.65°, QT 0.57 Å, suggesting a half chair conformation for both the conformations. A network of intra- and intermolecular hydrogen bonding interactions involves the water and ethanol molecules. In particular, an intramolecular H-bond interaction involves O (1 Et)-H … O (1w) d H … O (1w) = 1.97 (1)Å and O (1w)-H … O (2) d H … O (2) = 2.76 (4)Å, and intermolecular H-bond interactions are N (1)-H … O (1w) (x-1, y+1, z) d H … O (1w) = 2.021) Å; N (2)-H … O (1et) (x-1,y,z) d H … O (1w) = 2.051) Å.
The formation of the pentaheterocyclic system 14 can be explained considering the initial reduction of the oxime moiety to give amine 13 followed by its Pictet-Spengler condensation with acetone to give the final product.
The condensation of tryptamines with aldehydes and ketones, known as the Pictet-Spengler reaction, has been extensively explored for the synthesis of ring-fused indole derivatives, including tetrahydro-β-carbolines a core structure of various indole alkaloids (Stöckigt et al., 2011; Maity et al., 2019; Panice et al., 2019; Ribeiro et al., 2022). The mechanism of this transformation has been a research topic of some controversy as two pathways can be considered: the formation of spiroindolenines via the attack of indole’s C3 to the initially formed imine followed by a 1,2-migration/elimination sequence to restore aromaticity, or the direct C2 attack. However, there are several experimental studies where the spiroindolenines, intermediates of Pictet−Spengler-type reactions, were captured (Williams and Unger, 1970; Stöckigt et al., 2011; Chambers et al., 2016; Zheng and You, 2020). Furthermore, in recent years the interrupted Pictet–Spengler reaction is being explored as a strategy for the dearomatisation of indoles (James et al., 2016). Several successful syntheses of spirocyclic indolenine are known resulting from methodologies designed to allow the initial spirocyclisation step but preventing further reaction. The reported synthesis of hexahydropyrido[4′,3':4,5]pyrrolo[3,2-c]carbazole 14 is a new entry to Pictet−Spengler-type reactions.
This interesting result justified the optimization of the synthetic procedure, as a one-pot two-step procedure. Thus, a solution of compound 11a in acetic acid and acetone was treated with zinc powder at room temperature for 48 h. After the neutralization of the reaction medium and purification, the target compound 14 was isolated in 85% yield (Scheme 3).
Rationalization of the hetero-Diels-Alder reactions outcome
In order to investigate the observed and diverse regioselectivity, calculations at the DFT level of theory using the B3LYP hybrid functional (Becke, 1988; Lee et al., 1988; Becke, 1993) and the standard 6-31G (d,p) basis set were carried out for the hetero-Diels-Alder reaction of ethyl nitrosoacrylate (2a) with pyrrole, indole and 8-methyl-1,6-dihydropyrrolo[3,2-c]carbazole (10). For each heterocycle, relative stabilities of the different transition states (TS) involved in the hetero-Diels-Alder reactions were calculated, considering the two possible regioisomers and both endo and exo approaches (Figures 2–4). The individual contributions to the energy barriers associated with all the transition states studied for the reactions of ethyl nitrosoacrylate (2a) with pyrrole, indole and 8-methyl-1,6-dihydropyrrolo[3,2-c]carbazole (10) are reported in Tables 1–3, considering both zero-point-energy (ZPE) correction, and basis set superposition error (BSSE) correction.
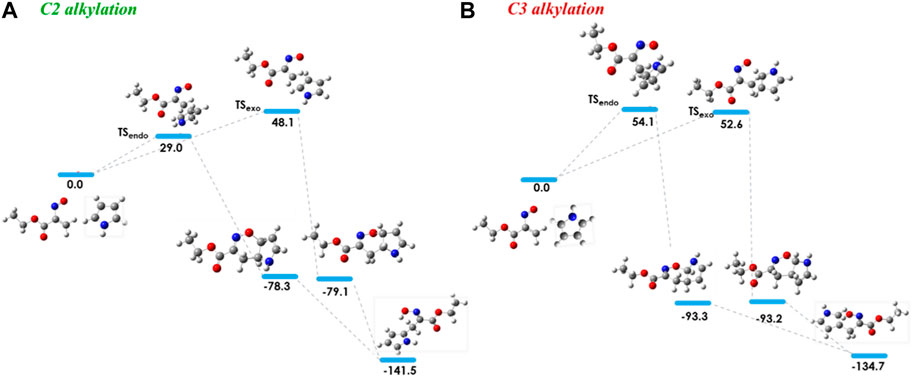
FIGURE 2. Relative stabilities (ΔE in kJ/mol) of the transition states involved in the hetero-Diels-Alder reaction of ethyl nitrosoacrylate (2a) with pyrrole considering the two regioisomers and both endo and exo approaches, both for C2 alkylation (A) and for C3 alkylation (B). All structures were optimized at the B3LYP/6-31G (d,p) level of theory. Color code: grey, carbon; red, oxygen; blue, nitrogen and white, hydrogen.
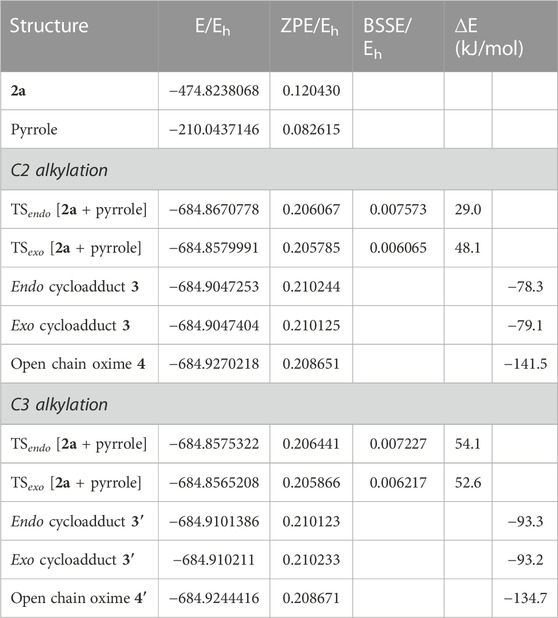
TABLE 1. Electronic energy, E, zero-point vibration energy, ZPE, basis set superposition error correction, BSSE, and energy relative to the reactants, ∆E, of the transition states and final products identified for the reaction between 2a and pyrrole for both C2 and C3 alkylation. All values are obtained at the B3LYP/6-31G (d,p) level. ∆E includes both ZPE and BSSE corrections.
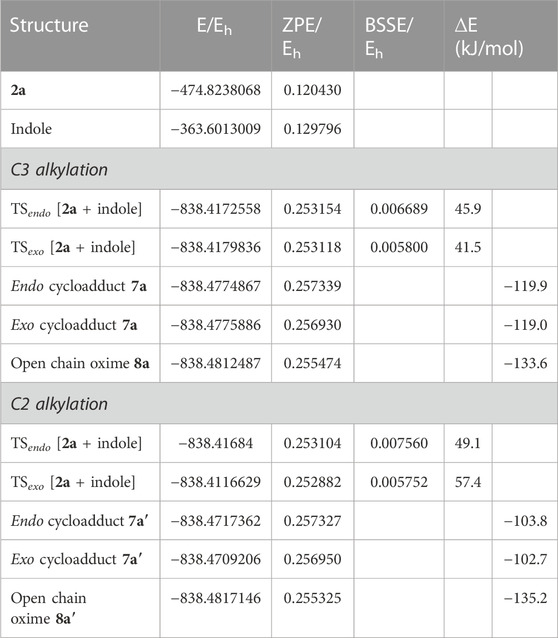
TABLE 2. Electronic energy, E, zero-point vibrational energy, ZPE, basis set superposition error correction, BSSE, and energy relative to the reactants, ∆E, of the transition states and final products identified for the reaction between 2a and indole for both C3 and C2 alkylation. All values are obtained at the B3LYP/6-31G (d,p) level. ∆E includes both ZPE and BSSE corrections.
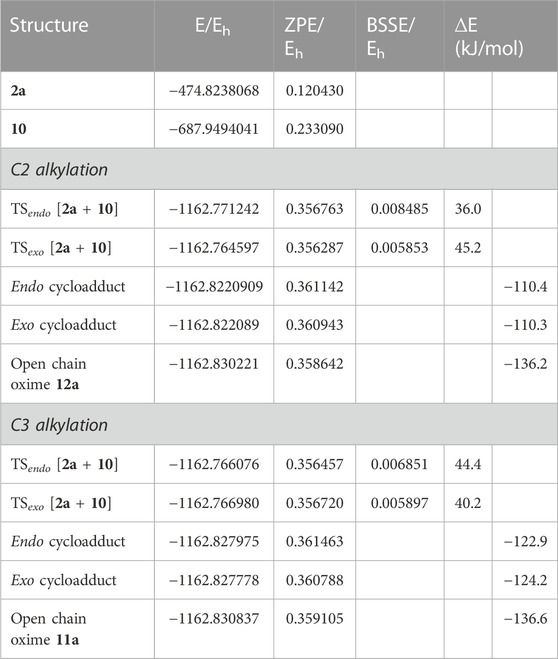
TABLE 3. Electronic energy, E, zero-point vibration energy, ZPE, basis set superposition error correction, BSSE, nand energy relative to the reactants, ∆E, of the transition states and final products identified for the reaction between 2a and pyrrolo [3,2-c]carbazole 10 for both C3 alkylation A) and C2 alkylation. All values are obtained at the B3LYP/6-31G (d,p) level. ∆E includes both ZPE and BSSE corrections.
In the reaction between nitrosoalkene 2a and pyrrole, the computational results showed that the energy barrier associated with the formation of cycloadduct 3 by an endo approach is lower (about 25 kJ/mol) than the energy required for the formation of cycloadduct 3’, which is in agreement with the regioselectivity observed experimentally (Figure 2). Furthermore, the open-chain oxime 4 was obtained as single product, which is more stable than the primarily formed bicyclic 1,2-oxazine 3, as confirmed by DFT calculations (about 63 kJ/mol) (Figure 2; Table 1).
The 3-alkylated indole 8a is obtained from the reaction of nitrosoalkene 2a with indole via the hetero-Diels-Alder reaction by an exo approach. Indeed, this mechanistic pathway involves a lower energy transition state (ΔE = 41.5 kJ/mol) than that which would lead to the C2 alkylation product (Figure 3). Once again, the theoretical predictions of the regioselectivity are in agreement with the experimental results. In addition, the cycloadduct 7a, involved in C3 alkylation pathway, is more stable (about 16 kJ/mol) than the homologue leading to C2 alkylation product (Figure 3; Table 2).
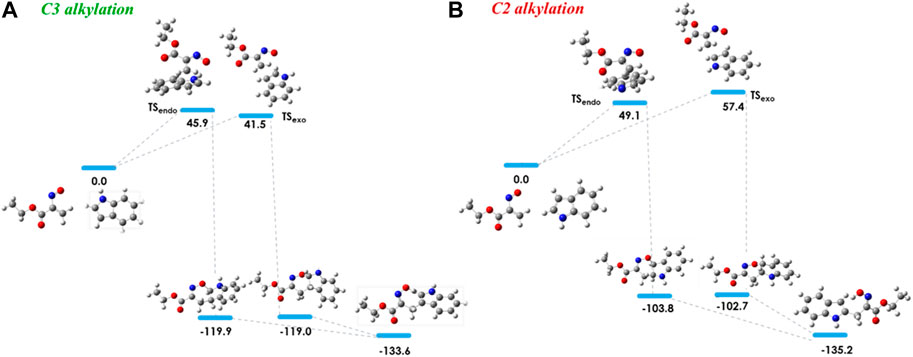
FIGURE 3. Relative stabilities (ΔE in kJ/mol) of transition states involved in the hetero-Diels-Alder reaction of ethyl nitrosoacrylate (2a) with indole considering the two regioisomers and both endo and exo approaches, both for C3 alkylation (A) and C2 alkylation (B). All structures were optimized at the B3LYP/6-31G (d,p) level of theory. Color code: grey, carbon; red, oxygen; blue, nitrogen and white, hydrogen.
The energy barriers calculated for the transition states of the hetero-Diels-Alder reaction of nitrosoalkene 2a with pyrrolo[3,2-c]carbazole 10 are very similar for the formation of both alkylated products (Figure 4). The DFT calculations showed that the formation of the 2-alkylated product proceeded via the endo transition state (TSendo), whereas the exo transition state (TSexo) was involved in the formation of the 3-alkylated product. The cycloadduct precursors of the 3-alkylated pyrrolo[3,2-c]carbazole 11a are more stable than the precursors of the 2-alkylated pyrrolo [3,2-c]carbazole 12a (about 13 kJ/mol), explaining the predominance of the 3-alkylated product. However, the stability of the open chain oximes 11a and 12a is very similar (Figure 4; Table 3). These computational results explain the isolation of both 2-alkylated and 3-alkylated products from the reaction between nitrosoalkene 2a and pyrrolo[3,2-c]carbazole 10.
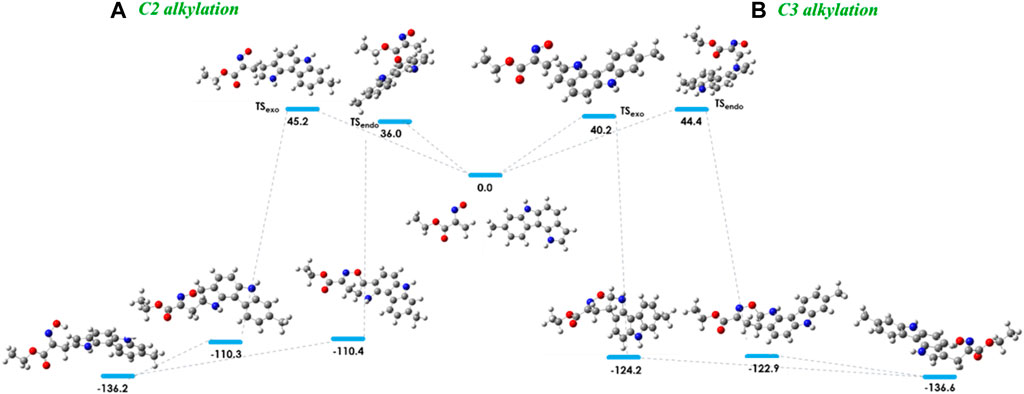
FIGURE 4. Relative stabilities (ΔE in kJ/mol) of transition states involved in the hetero-Diels-Alder reaction of ethyl nitrosoacrylate (2a) with 8-methyl-1,6-dihydropyrrolo [3,2-c]carbazole considering the C2 alkylation (A) and C3 alkylation (B) and both endo and exo approaches, obtained at the B3LYP/6-31G (d,p) level of theory. Color code: gray, carbon; red, oxygen; blue, nitrogen and white, hydrogen.
Frontier Molecular Orbital (FMO) analysis of the hetero-Diels–Alder reaction of ethyl nitrosoacrylate (2a) with pyrrole, indole and pyrrolo[3,2-c]carbazole 10 was carried out. The relative energy values of the HOMO and LUMO orbitals of the reactants were obtained at the HF/6-31G (d,p) level of theory (Figure 5). The results show that the energy difference between the LUMO of the nitrosoalkene and the HOMO of the heterocycles is smaller (between 7.99 and 9.08 eV) than that calculated for the HOMOnitrosoalkene-LUMOheterocycle pair (between 14.03 and 16.51 eV). Thus, the results show that these reactions are LUMOnitrosoalkene-HOMOheterocycle controlled and confirm that pyrrole, indole and 8-methyl-1,6-dihydropyrrolo [3,2-c]carbazole participate in inverse electron-demand hetero-Diels-Alder reaction with nitrosoalkene 2a acting as electron-rich 2π component. Furthermore, FMO analysis indicates that the molecular orbital energy profile of 8-methyl-1,6-dihydropyrrolo[3,2-c]carbazole is closer to the one of indole than to pyrrole.
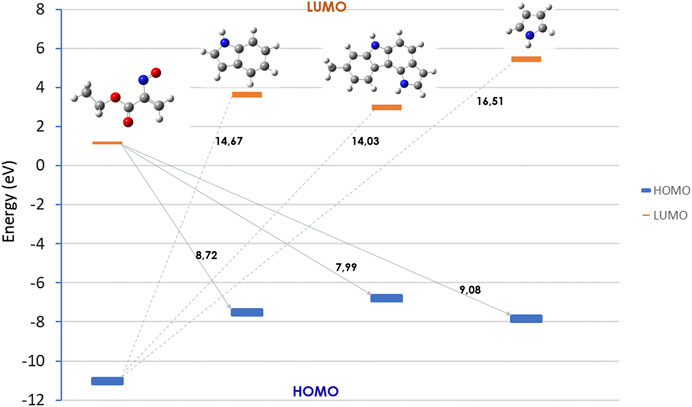
FIGURE 5. Relative energies (eV) of the HOMO and LUMO orbitals for nitrosoalkene 2a, pyrrole, indole and pyrrolo [3,2-c]carbazole 10, obtained at the HF/6-31G (d,p) level of theory.
The results of the Frontier Molecular Orbital interactions are in accordance with the observed regioselectivity, pointing generally to the formation of the product that stems from the interaction sites corresponding to the larger orbital coefficients. For indole the calculated orbital coefficients do not allow to distinguish between the two possible regiochemistries (Figure 6).
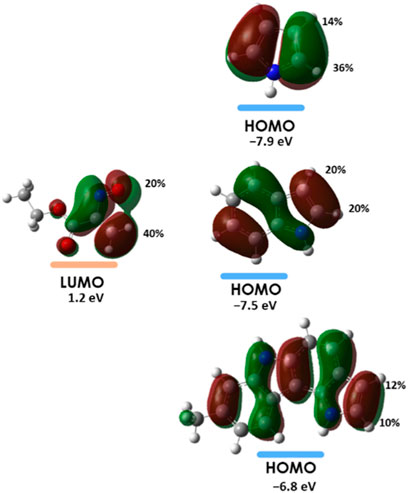
FIGURE 6. Orbital interaction diagrams for the hetero-Diels-Alder reactions with the indication of the orbital coefficients for the interacting orbitals obtained through NBO analysis at the HF/6-31G (d,p) level of theory.
Scheme 4 summarizes the mechanistic pathways leading to oxime-functionalized pyrrolo[3,2-c]carbazoles 11a and 12a. The 3-alkylated pyrrolo[3,2-c]carbazole is obtained via hetero-Diels-Alder reaction of nitrosoalkene 2a with an exo approach followed by 1,2-oxazine ring-opening reaction. The synthesis of the 2-alkylated derivative takes place with the initial endo cycloaddition reaction and subsequent conversion into the final oxime 12a. The selectivity towards the 3-alkylated pyrrolo[3,2-c]carbazole 11a is determined by the more exothermic formation of the hetero-Diels-Alder cycloadduct than that derived from the opposite regiochemistry. It should be noted that the chemical behaviour of 8-methyl-1,6-dihydropyrrolo[3,2-c]carbazole is closer to the reactivity observed for indole than to that of pyrrole.
Conclusion
The reactivity of nitrosoalkenes towards 8-methyl-1,6-dihydropyrrolo[3,2-c]carbazole was studied leading to the synthesis of oxime-functionalized pyrrolo[3,2-c]carbazoles. The mechanistic pathway involves a hetero-Diels-Alder reaction leading to the construction of a 1,2-oxazine ring which undergoes a ring-opening reaction to give open-chain oximes. Calculations at the DFT level of theory were carried out to investigate the regioselectivity of the hetero-Diels-Alder reaction of ethyl nitrosoacrylate with 8-methyl-1,6-dihydropyrrolo[3,2-c]carbazole as well as with pyrrole and indole, allowing a comparison between these three types of heterocycles. The computational results allowed the rationalization of the regioselectivity observed in the cycloaddition reaction and the formation of the more stable open chain oximes as the final products. The relative energy values of the Frontier HOMO and LUMO molecular orbitals for the ethyl nitrosoacrylate and the studied heterocyclic dienophiles were also calculated, substantiating that the cycloadditions are LUMOheterodiene-HOMOdienophile controlled.
The construction of a new hexahydropyrido[4′,3':4,5]pyrrolo [3,2-c]carbazole system from one of the new 3-alkylated pyrrolo [3,2-c]carbazole was achieved in high yield via a one-pot two-step approach.
Experimental
General Information: NMR spectra were recorded on a Bruker Avance III instrument operating at 400 MHz (1H) or 100 MHz (13C). Chemical shifts are expressed in ppm relative to TMS and coupling constants (J) are in Hz. Infrared spectra (IR) were recorded in a Fourier Transform spectrometer. High-resolution mass spectra (HRMS) were obtained on a TOF VG Autospec M spectrometer with electrospray ionization (ESI). Melting points were recorded in open glass capillaries. Thin Layer Chromatography (TLC) was performed using precoated silica gel plates. Flash chromatography was performed using silica gel 60 as a stationary phase. Ethyl bromopyruvate oxime (1a) (Gilchrist and Roberts, 1983), 2-bromo-1-phenylethanone oxime (1b) (Masaki et al., 1967) and 8-methyl-1,6-dihydropyrrolo [3,2-c]carbazole (10) (Benzi et al., 2019) were prepared as described in the literature.
General procedure for the hetero-Diels-Alder reactions
Sodium carbonate (0.75 mmol) was added to a solution of a-bromooxime 1 (0.15 mmol) and 8-methylpyrrolo[3,2-c]carbazole 10 (0.22 mmol) in dry dichloromethane (10 mL). The reaction mixture was stirred at room temperature for the time indicated in each case, monitored by TLC. Upon completion, the mixture was filtered through a Celite pad, which was washed with ethyl acetate (2 × 10 mL). The solvent was evaporated, and the products were purified by flash chromatography.
(E)-3-(2-Ethoxycarbonyl-2-hydroxyiminoethyl)-8-methyl-1,6-dihydropyrrolo[3,2-c]carbazole (11a) and (E)-2-(2-Ethoxycarbonyl-2-hydroxyiminoethyl)-8-methyl-1,6-dihydropyrrolo[3,2-c]carbazole (12a). Obtained from oxime 1a (31.5 mg, 0.15 mmol) and 8-methylpyrrolo[3,2-c]carbazole 10 (48.5 mg, 0.22 mmol) as described in general procedure (reaction time: 18 h). Purification of the crude product by flash chromatography [ethyl acetate/hexane (1:1)], gave, in order of elution, 12a obtained as a beige solid (11.0 mg, 21%) and 11a obtained as a beige solid (22.0 mg, 42%).
Data for compound 11a: mp 199.7°C-201.6°C (from ethyl acetate/hexane). IR (KBr) ν 796, 1020, 1136, 1196, 1389, 1429, 1643, 1714, 3261, 3419 and 3446 cm-1. 1H NMR (Acetone-d6) δ: 1.21 (t, J = 7.2 Hz, 3H), 2.49 (s, 3H), 4.15 (d, J = 0.8 Hz, 2H), 4.17 (q, J = 7.2 Hz, 2H), 7.02 (dd, J = 8.0 and 0.8 Hz, 1H), 7.14 (dd, J = 2.0 and 0.8 Hz, 1H), 7.25 (d, J = 8.4 Hz, 1H), 7.33 (s, 1H), 7.74 (d, J = 8.8 Hz, 1H), 8.24 (d, J = 8.0 Hz, 1H), 10.22 (br s, 1H), 10.50 (br s, 1H), 11.35 (s, 1H). 13C NMR (Acetone-d6) δ: 14.4, 21.1, 22.0, 61.5, 105.0, 108.3, 111.4, 111.6, 118.0, 120.5, 121.0, 121.3, 121.5, 121.8, 130.9, 134.2, 138.1, 140.3, 152.5, 164.9. HRMS (ESI): calcd. for C20H18N3O3, 348.1354 [M-H+]; found, 348.1352.
Data for compound 12a: mp 154.8°C-156.0°C (from carbon tetrachloride). IR (KBr) ν 804, 1020, 1132, 1261, 1382, 1464, 1614, 1722, 2924, 2977 and 3381 cm-1. 1H NMR (Acetone-d6) δ: 1.26 (t, J = 7.2 Hz, 3H), 2.49 (s, 3H), 4.22 (d, J = 1.2 Hz, 2H), 4.25 (q, J = 7.2 Hz, 2H), 6.32 (dd, J = 2.4 and 1.2 Hz, 1H), 7.03 (dd, J = 7.6 and 1.2 Hz, 1H), 7.19 (d, J = 8.4 Hz, 1H), 7.33 (s, 1H), 7.46 (d, J = 8.8 Hz, 1H), 8.19 (d, J = 8.0 Hz, 1H), 10.18 (br s, 1H), 10.26 (br s, 1H), 11.58 (br s, 1H). 13C NMR (Acetone-d6) δ: 14.4, 22.0, 24.3, 61.9, 102.0, 105.2, 111.6, 118.8, 120.4, 120.9, 121.2, 122.8, 124.4, 130.6, 131.3, 134.3, 137.8, 140.3, 150.7, 164.9. HRMS (ESI): calcd. for C20H20N3O3, 350.1499 [M + H+]; found, 350.1496.
(E)-3-(2-Phenyl-2-hydroxyiminoethyl)-8-methyl-1,6-dihydropyrrolo[3,2-c]carbazole (11b) and (E)-2-(2-phenyl-2-hydroxyiminoethyl)-8-methyl-1,6-dihydropyrrolo[3,2-c]carbazole (12b). Obtained from oxime 1b (32.1 mg, 0.15 mmol) and 8-methylpyrrolo[3,2-c]carbazole 10 (48.5 mg, 0.22 mmol) as described in general procedure (reaction time: 48 h). Purification of the crude product by flash chromatography [ethyl acetate/hexane, (1:2)], gave, in order of elution, 12b obtained as a beige solid (4.2 mg, 8%) and 11b obtained as a beige solid (19.6 mg, 37%).
Data for compound 11b: mp 179.8°C-181.7°C (from ethyl acetate/hexane). IR (KBr) ν 687, 761, 943, 1124, 1298, 1331, 1389, 1429, 1462, 1639, 2918, 3059 and 3392 cm-1. 1H NMR (Acetone-d6) δ: 2.48 (s, 3H), 4.39 (d, J = 1.2 Hz, 2H), 7.00 (dd, J = 8.0 and 1.2 Hz, 1H), 7.05 (dd, J = 2.4 and 1.2 Hz, 1H), 7.24-7.29 (m, 4H), 7.32 (br s, 1H), 7.75-7.77 (m, 3H), 8.21 (d, J = 8.0 Hz, 1H), 10.22 (br s, 1H), 10.41 (br s, 1H), 10.51 (s, 1H). 13C NMR (Acetone-d6) δ: 22.0, 22.1, 105.0, 109.6, 111.5, 112.4, 117.8, 120.5, 120.9, 121.1, 121.3, 121.8, 127.3, 128.9, 129.2, 131.0, 134.2, 137.7, 138.2, 140.3, 157.2. HRMS (ESI): calcd. for C23H20NO3, 354.1601 [M + H+]; found, 354.1598.
Data for compound 12b: mp 118.0°C-119.5°C (from carbon tetrachloride). IR (KBr) ν 694, 760, 800, 1184, 1288, 1385, 1620, 1697, 2850, 2920 and 3398 cm-1. 1H NMR (Acetone-d6) δ: 2.49 (s, 3H), 4.45 (d, J = 0.8 Hz, 2H), 6.33 (dd, J = 2.0 and 0.8 Hz, 1H), 7.03 (dd, J = 8.0 and 1.6 Hz, 1H), 7.17 (d, J = 8.4 Hz, 1H), 7.31-7.38 (m, 4H), 7.42 (d, J = 8.4 Hz, 1H), 7.57-7.61 (m, 1H), 7.82-7.85 (m, 1H), 8.15 (d, J = 8.0 Hz, 1H), 10.18 (br s, 1H), 10.33 (br s, 1H), 10.82 (s, 1H). 13C NMR (Acetone-d6) δ: 21.1, 24.6, 101.2, 104.2, 107.1, 110.7, 117.8, 120.0, 120.2, 124.9, 126.2, 127.3, 128.2, 128.5, 128.8, 129.8, 131.6, 133.4, 136.3, 136.9, 139.4, 154.9. HRMS (ESI): calcd. for C23H20NO3, 354.1601 [M + H+]; found, 354.1598.
Ethyl 1,1,9-trimethyl-1,2,3,4,7,12-hexahydropyrido[4′,3':4,5]pyrrolo[3,2-c]carbazole-3-carboxylate (14): Zinc powder (80 mg, 1.22 mmol) was added portion-wise to a solution of 3-alkylated pyrrolo [3,2-c]carbazole 11a (35.6 mg; 0.102 mmol) in acetic acid (1.5 mL) and acetone (0.1 mL). The resulting mixture was stirred at room temperature for 24 h. After this time, zinc powder (80 mg, 1.22 mmol) was added and the resulting mixture stirred at room temperature for more 24 h. Upon completion, the zinc salts were removed by filtration through a Celite pad, which was washed with ethyl acetate (3 × 10 mL). The filtrate was neutralized with aqueous NaOH 5% to pH 7 and then extracted with ethyl acetate (3 × 20 mL). The organic extracts were dried over Na2SO4 and the solvent evaporated off. Compound 14 was purified by flash chromatography [ethyl acetate/hexane, (1:1)] and obtained as a beige solid (32.5 mg, 85%). mp 114.0°C-115.8°C (from ethyl acetate/hexane). IR (KBr) ν 808, 1030, 1192, 1265, 1469, 1620, 1705, 2921, 2962 and 3392 cm-1. 1H NMR (CDCl3) δ: 1.37 (t, J = 7.2 Hz, 3H), 1.58 (s, 3H), 1.66 (s, 3H), 2.55 (s, 3H), 2.84 (dd, J = 15.2 and 11.2 Hz, 1H), 3.20 (dd, J = 15.2 and 4.2 Hz, 1H), 4.00 (dd, J = 11.2 and 4.2 Hz, 1H), 4.28-4.36 (m, 2H), 7.13 (dd, J = 8.0 and 1.6 Hz, 1H), 7.22 (d, J = 8.4 Hz, 1H), 7.26 (s, 1H), 7.49 (d, J = 8.8 Hz, 1H), 7.95 (d, J = 8.0 Hz, 1H), 8.10 (s, 1H), 8.11 (s, 1H). 13C NMR (CDCl3) δ: 14.4, 22.2, 27.0, 28.9, 30.1, 51.1, 53.1, 61.3, 104.4, 107.4, 107.8, 111.0, 116.5, 119.6, 120.3, 120.7, 120.9, 129.9, 134.6, 137.0, 137.3, 139.2, 174.0. HRMS (ESI): calcd. for C23H26N2O3, 376.2019 [M+]; found, 376.2015.
Crystallographic data for Ethyl 1,1,9-trimethyl-1,2,3,4,7,12-hexahydropyrido[4′,3':4,5]pyrrolo[3,2-c]carbazole-3-carboxylate (14): A single crystal of compound 14. H2O.C2H5OH was submitted to X-ray data collection on a Bruker APEX-II CCD diffractometer with a graphite monochromated Cu-Kα radiation (λ = 1.54178 Å) at 100 K. The structure was solved by direct methods implemented in SHELXS-97 program (Sheldrick, 2008). The refinement was carried out by full-matrix anisotropic least-squares on F2 for all reflections for non-H atoms by means of the SHELXL (version 2019/2) program (Sheldrick, 2015). The structure crystallizes with a water and an ethanol molecule in the Triclinic crystal system, space group P-1 with one molecule in the asymmetric unit. Crystallographic data for this structure has been deposited with the Cambridge Crystallographic Data Centre as supplementary publication no. CCDC 2222762. Copies of the data can be obtained, free of charge, on application to CCDC, 12 Union Road, Cambridge CB2 1EZ, UK; (fax: +44 0) 1223 336 033; or e-mail: ZGVwb3NpdEBjY2RjLmNhbS5hYy51aw==).
Computational methodology
Calculations were performed with Gaussian 09 (Frisch et al., 2009) and Gamess (Schmidt et al., 1993) program packages. All structures were fully optimized at the DFT level of theory, using the B3LYP hybrid functional (Becke, 1988; Lee et al., 1988; Becke, 1993) and the standard 6-31G (d,p) basis set.
Vibrational frequencies were calculated at the same level of theory to evaluate the zero-point vibrational energy, ZPE, and to confirm the nature of the stationary points, that in the case of the transition states were characterized by having only one imaginary frequency. Inspection of the corresponding imaginary frequency allowed to confirm that the transition states connect the reactants with the expected products. The geometrical counterpoise correction was added to all transition state structures. Frontier molecular orbitals were calculated on DFT-optimized structures at the HF level of theory with the 6-31G(d) basis set. The orbital coefficients were calculated using the NBO module of Gaussian. Graphical representations were obtained with Gaussview.
Data availability statement
The datasets presented in this study can be found in online repositories. The names of the repository/repositories and accession number(s) can be found below: https://www.ccdc.cam.ac.uk/, 2222762.
Author contributions
TP and GP contributed to conception and design of the study. SL wrote the first draft of the manuscript. SL and AB performed the synthetic work. SN performed the DFT calculations. GG performed the X-ray diffraction studies. TP, GP, AP, SL SN contributed to data analysis and interpretation. All authors contributed to the article and approved the submitted version.
Funding
The Coimbra Chemistry Centre–Institute of Molecular Sciences (CQC-IMS) is supported by Portuguese Foundation for Science and Technology (FCT) through projects UIDB/00313/2020 and UIDP/00313/2020 (National Funds) and the IMS special complementary funds provided by FCT. This work was also supported by Project PTDC/QUI-QOR/0103/2021, funded by national funds (PIDDAC) via FCT.
Acknowledgments
AB thanks to the University of Genova for financial support. We also acknowledge the UC-NMR facility for obtaining the NMR data (www.nmrccc.uc.pt).
Conflict of interest
The authors declare that the research was conducted in the absence of any commercial or financial relationships that could be construed as a potential conflict of interest.
Publisher’s note
All claims expressed in this article are solely those of the authors and do not necessarily represent those of their affiliated organizations, or those of the publisher, the editors and the reviewers. Any product that may be evaluated in this article, or claim that may be made by its manufacturer, is not guaranteed or endorsed by the publisher.
Supplementary material
The Supplementary Material for this article can be found online at: https://www.frontiersin.org/articles/10.3389/fchem.2023.1229669/full#supplementary-material
References
Akué-Gédu, R., Rossignol, E., Azzaro, S., Knapp, S., Filippakopoulos, P., Bullock, A. N., et al. (2009). Synthesis, kinase inhibitory potencies, and in vitro antiproliferative evaluation of new pim kinase inhibitors. J. Med. Chem. 52, 6369–6381. doi:10.1021/jm901018f
Alves, A. J. S., Lopes, S. M. M., Henriques, M. S. C., Paixão, J. A., and Pinho e Melo, T. M. V. D. (2017). Hetero-diels–alder and ring-opening reactions of furans applied to the synthesis of functionalized heterocycles. Eur. J. Org. Chem. 4011–4025. doi:10.1002/ejoc.201700453
Becke, A. D. (1988). Density-functional exchange-energy approximation with correct asymptotic behavior. Phys. Rev. A 38, 3098–3100. doi:10.1103/PhysRevA.38.3098
Becke, A. D. (1993). Density-functional thermochemistry. III. The role of exact exchange. J. Chem. Phys. 98, 5648–5652. doi:10.1063/1.464913
Benzi, A., Bianchi, L., Maccagno, M., Pagano, A., Petrillo, G., and Tavani, C. (2019). Sequential annulations to interesting novel pyrrolo[3,2-c]carbazoles. Molecules 24, 3802. doi:10.3390/molecules24203802
Bingul, M., Şenkuytu, E., Boğa, M., Uslu, T. N., Kandemir, H., and Sengul, I. F. (2019). Synthesis, photophysical and antioxidant properties of pyrrolo[3,2-c]carbazole and dipyrrolo[3,2-c:2′,3′-g]Carbazole compounds. Res. Chem. Intermed. 45, 997–1008. doi:10.1007/s11164-018-3661-0
Cardoso, A. L., Lopes, S. M. M., Grosso, C., Pineiro, M., Lemos, A., and Pinho e Melo, T. M. V. D. (2021). One-pot synthetic approach to dipyrromethanes and bis(indolyl)methanes via nitrosoalkene chemistry. J. Chem. Edu. 98, 2661–2666. doi:10.1021/acs.jchemed.1c00184
Chambers, S. J., Coulthard, G., Unsworth, W. P., O'Brien, P., and Taylor, R. J. K. (2016). From heteroaromatic acids and imines to azaspirocycles: Stereoselective synthesis and 3D shape analysis. Chem. Eur. J. 22, 6496–6500. doi:10.1002/chem.201600823
Cremer, D., and Pople, J. A. (1975). General definition of ring puckering coordinates. J. Am. Chem. Soc. 97, 1354–1358. doi:10.1021/ja00839a011
Frisch, M. J., Trucks, G. W., Schlegel, H. B., Scuseria, G., Robb, M. A., and Cheeseman, J. R., Gaussian 09, 2009, 2009, Wallingford, CT, USA, Gaussian Inc.
Gilchrist, T. L., and Lemos, A. (1993). Reaction of pyrroles with ethyl 2-nitroso- and 2-Azo-propionates, and with ethyl cyanoformate N-oxide: A comparison of the reaction pathways. J. Chem. Soc. Perkin Trans. 1, 1391–1395. doi:10.1039/P19930001391
Gilchrist, T. L., and Roberts, T. G. (1983). Addition and cycloaddition reactions of the electrophilic vinyl nitroso compounds 3-Nitrosobut-3-en-2-one, 2-nitrosopropenal, and ethyl 2-nitrosopropenoate. J. Chem. Soc. Perkin Trans. 1, 1283–1292. doi:10.1039/P19830001283
Giraud, F., Akué-Gédu, R., Nauton, L., Candelon, N., Debiton, E., Théry, V., et al. (2012). Synthesis and biological activities of 4-substituted pyrrolo[2,3-a]carbazole pim kinase inhibitors. Eur. J. Med. Chem. 56, 225–236. doi:10.1016/j.ejmech.2012.08.029
Giraud, F., Pereira, E., Anizon, F., and Moreau, P. (2019). Synthesis and applications of dihydropyrrolocarbazoles. Eur. J. Org. Chem. 2019, 5025–5042. doi:10.1002/ejoc.201900269
Grosso, C., Brigas, A., de los Santos, J. M., Palacios, F., Lemos, A., and Pinho e Melo, T. M. V. D. (2019). Natural deep eutectic solvents in the hetero-diels-alder approach to bis(indolyl)methanes. Monatsh. Chem. 150, 1275–1288. doi:10.1007/s00706-019-02421-7
Grosso, C., Cardoso, A. L., Lemos, A., Varela, J., Rodrigues, M. J., Custodio, L., et al. (2015). Novel approach to bis(indolyl)methanes: De novo synthesis of 1-hydroxyiminomethyl derivatives with anti-cancer properties. Eur. J. Med. Chem. 93, 9–15. doi:10.1016/j.ejmech.2015.01.050
Grosso, C., Cardoso, A. L., Rodrigues, M. J., Marques, C., Barreira, L., Lemos, A., et al. (2017). Hetero-diels-alder approach to bis(indolyl)methanes. Bioorg. Med. Chem. 25, 1122–1131. doi:10.1016/j.bmc.2016.12.028
Grosso, C., Lemos, A., and Pinho e Melo, T. M. V. D. (2014). Conjugate addition of pyrazoles to halogenated nitroso- and azoalkenes: A new entry to novel bis(pyrazol-1-yl)methanes. Synlett 25, 2868–2872. doi:10.1055/s-0034-1379306
James, M. J., O'Brien, P., Taylor, R. J. K., and Unsworth, W. P. (2016). Synthesis of spirocyclic indolenine. Chem. Eur. J. 22, 2856–2881. doi:10.1002/chem.201503835
Jorda, R., Lopes, S. M. M., Reznickova, E., Krystof, V., and Pinho e Melo, T. M. V. D. (2017). Biological evaluation of dipyrromethanes in cancer cell lines: Antiproliferative and pro-apoptotic properties. ChemMedChem 12, 701–711. doi:10.1002/cmdc.201700152
Lee, C., Yang, W., and Parr, R. G. (1988). Development of the colle-salvetti correlation-energy formula into a functional of the electron density. Phys. Rev. B 37, 785–789. doi:10.1103/PhysRevB.37.785
Lopes, S. M. M., Cardoso, A. L., Lemos, A., and Pinho e Melo, T. M. V. D. (2018a). Recent advances in the chemistry of conjugated nitrosoalkenes and azoalkenes. Chem. Rev. 118, 11324–11352. doi:10.1021/acs.chemrev.8b00375
Lopes, S. M. M., Henriques, M. S. C., Paixao, J. A., and Pinho e Melo, T. M. V. D. (2015). Exploring the chemistry of furans: Synthesis of functionalized bis(furan-2-yl)-methanes and 1,6-dihydropyridazines. Eur. J. Org. Chem. 2015, 6146–6151. doi:10.1002/ejoc.201500878
Lopes, S. M. M., Lemos, A., and Pinho e Melo, T. M. V. D. (2010). A hetero-diels-alder approach to functionalized 1H-tetrazoles: Synthesis of tetrazolyl-1,2-oxazine, -oximes and 5-(1-aminoalkyl)-1H-tetrazoles. Tetrahedron Lett. 51, 6756–6759. doi:10.1016/j.tetlet.2010.10.095
Lopes, S. M. M., Novais, J. S., Costa, D. C. S., Castro, H. C., Figueiredo, A. M. S., Ferreira, V. F., et al. (2018b). Hetero-diels-alder reactions of novel 3-Triazolyl-nitrosoalkenes as an approach to functionalized 1,2,3-triazoles with antibacterial profile. Eur. J. Med. Chem. 143, 1010–1020. doi:10.1016/j.ejmech.2017.11.052
Lopes, S. M. M., Nunes, S. C. C., Caratão, C. C., Pais, A. A. C. C., and Pinho e Melo, T. M. V. D. (2016). Reactivity of 1-arylnitrosoethylenes towards indole derivatives. Monatsh. Chem. 147, 1565–1573. doi:10.1007/s00706-016-1763-1
Lopes, S. M. M., Palacios, F., Lemos, A., and Pinho e Melo, T. M. V. D. (2011). Diels-alder reactions of 3-(1H-Tetrazol-5-yl)-nitrosoalkenes: Synthesis of functionalized 5-(substituted)-1H-tetrazoles. Tetrahedron 67, 8902–8909. doi:10.1016/j.tet.2011.09.051
Lopes, S. M. M., and Pinho e Melo, T. M. V. D. (2020). Meso-Substituted corrodes from nitrosoalkenes and dipyrromethanes. J. Org. Chem. 85, 3328–3335. doi:10.1021/acs.joc.9b03151
Maity, P., Adhikari, D., and Jana, A. K. (2019). An overview on synthetic entries to tetrahydro-β-carbolines. Tetrahedron 75, 965–1028. doi:10.1016/j.tet.2019.01.004
Masaki, M., Fukui, K., and Ohta, M. (1967). Reaction of.alpha.-halo oximes with triphenylphosphine. Formation of imidoyl bromide of oximinophophonium salts by a novel catalytic effect of bases. J. Org. Chem. 32, 3564–3568. doi:10.1021/jo01286a061
Naumovich, Y. A., Ioffe, S. L., and Sukhorukov, A. Y. (2019). Michael addition of P-nucleophiles to conjugated nitrosoalkenes. J. Org. Chem. 84, 7244–7254. doi:10.1021/acs.joc.9b00924
Nunes, S. C. C., Lopes, S. M. M., Gomes, C. S. B., Lemos, A., Pais, A., and Pinho e Melo, T. M. V. D. (2014). Reactions of nitrosoalkenes with dipyrromethanes and pyrroles: Insight into the mechanistic pathway. J. Org. Chem. 79, 10456–10465. doi:10.1021/jo502095k
Panice, M. R., Lopes, S. M. M., Figueiredo, M. C., Goes Ruiz, A. L. T., Foglio, M. A., Nazari Formagio, A. S., et al. (2019). New 3-Tetrazolyl-β-carbolines and β-Carboline-3-carboxylates with anti-cancer activity. Eur. J. Med. Chem. 179, 123–132. doi:10.1016/j.ejmech.2019.05.085
Pereira, N. A. M., Lopes, S. M. M., Lemos, A., and Pinho e Melo, T. M. V. D. (2014). On-water synthesis of dipyrromethanes via bis-hetero-diels-alder reaction of azo- and nitrosoalkenes with pyrrole. Synlett 25, 423–427. doi:10.1055/s-0033-1340300
Ribeiro, J. L. P., Loureiro, J. B., Lopes, S. M. M., Saraiva, L., and Pinho e Melo, T. M. V. D. (2022). 3-(1,2,3-Triazol-4-yl)-β-Carbolines and 3-(1H-Tetrazol-5-yl)-β-Carbolines: Synthesis and evaluation as anticancer agents. Pharmaceuticals 15, 1510. doi:10.3390/ph15121510
Ribeiro, L. P. J., Alves, C., Cardoso, L. A., Lopes, M. M. S., and Pinho e Melo, M. V. D. T. (2021). Reduction of oximes and hydrazones: Asymmetric and diastereoselective approaches. Curr. Org. Chem. 25, 2175–2198. doi:10.2174/1385272825666210706151631
Saglam, M. F., Gündogdu, A., Hora, M., Kandemir, H., and Sengul, I. F. (2021). Synthesis of pyrrolo[3,2-c]carbazole-2-carbohydrazides and pyrrolo[3,2-c]carbazol-2-yl-1,3,4-oxadiazoles and their in vitro antibacterial evaluation. Synth. Commun. 51, 3164–3174. doi:10.1080/00397911.2021.1966040
Schmidt, M. W., Baldridge, K. K., Boatz, J. A., Elbert, S. T., Gordon, M. S., Jensen, J. H., et al. (1993). General atomic and molecular electronic structure system. J. Comput. Chem. 14, 1347–1363. doi:10.1002/jcc.540141112
Sengul, I. F., Astarci, E., and Kandemir, H. (2016). Synthesis of novel pyrrolo[3,2-c]carbazole and dipyrrolo[3,2-c:2′,3′-g]carbazole derivatives. Synlett 27, 1277–1281. doi:10.1055/s-0035-1560601
Sheldrick, G. (2008). A short history of SHELX. Acta Cryst. A 64, 112–122. doi:10.1107/S0108767307043930
Sheldrick, G. (2015). Crystal structure refinement with SHELXL. Acta Cryst. C 71, 3–8. doi:10.1107/S2053229614024218
Sinicropi, M. S., Tavani, C., Rosano, C., Ceramella, J., Iacopetta, D., Barbarossa, A., et al. (2021). A nitrocarbazole as a new microtubule-targeting agent in breast cancer treatment. Appl. Sci. 11, 9139. doi:10.3390/app11199139
Soares, M. I. L., Cardoso, A. L., and Pinho e Melo, T. M. V. D. (2022). Diels-alder cycloaddition reactions in sustainable media. Molecules 27, 1304. doi:10.3390/molecules27041304
Stöckigt, J., Antonchick, A. P., Wu, F., and Waldmann, H. (2011). The Pictet–spengler reaction in nature and in organic chemistry. Angew. Chem. Int. Ed. 50, 8538–8564. doi:10.1002/anie.201008071
Surowiak, A. K., Lochyński, S., and Strub, D. J. (2020). Unsubstituted oximes as potential therapeutic agents. Symmetry 12, 575. doi:10.3390/sym12040575
Weinreb, S. M. (2019). Nitrosoalkenes: Underappreciated reactive intermediates for formation of carbon–carbon bonds. Synlett 30, 1855–1866. doi:10.1055/s-0037-1611899
Williams, J. R., and Unger, L. R. (1970). Biogenetically patterned synthesis of the spiro[indoline-3,4′-proline] system. J. Chem. Soc. Chem. Commun. 0, 1605–1606. doi:10.1039/C29700001605
Zhang, W., and Ready, J. M. (2017). Total synthesis of the dictyodendrins as an arena to highlight emerging synthetic technologies. Nat. Prod. Rep. 34, 1010–1034. doi:10.1039/C7NP00018A
Keywords: nitrosoalkenes, pyrrolo[3,2-c]carbazole, hetero-Diels-Alder reactions, pictet-spengler reaction, DFT calculations
Citation: Benzi A, Lopes SMM, Nunes SCC, Giorgi G, Bianchi L, Tavani C, Pais AACC, Petrillo G and Pinho e Melo TMVD (2023) Reactivity of ethyl nitrosoacrylate toward pyrrole, indole and pyrrolo[3,2-c]carbazole: an experimental and theoretical study. Front. Chem. 11:1229669. doi: 10.3389/fchem.2023.1229669
Received: 26 May 2023; Accepted: 26 July 2023;
Published: 08 August 2023.
Edited by:
Alexey Sukhorukov, N. D. Zelinsky Institute of Organic Chemistry (RAS), RussiaReviewed by:
Burak Kuzu, Van Yüzüncü Yıl University, TürkiyeTao Shi, The Scripps Research Institute, United States
Copyright © 2023 Benzi, Lopes, Nunes, Giorgi, Bianchi, Tavani, Pais, Petrillo and Pinho e Melo. This is an open-access article distributed under the terms of the Creative Commons Attribution License (CC BY). The use, distribution or reproduction in other forums is permitted, provided the original author(s) and the copyright owner(s) are credited and that the original publication in this journal is cited, in accordance with accepted academic practice. No use, distribution or reproduction is permitted which does not comply with these terms.
*Correspondence: Teresa M. V. D. Pinho e Melo, dG1lbG9AY2kudWMucHQ=