- 1Department of Mechanical Engineering, Jabalpur Engineering College, Jabalpur, India
- 2Energy Centre, Maulana Azad National Institute of Technology, Bhopal, India
- 3Energy Technology Research Center, Department of Mechanical Engineering, Prince of Songkla University, Hat Yai, Thailand
The experimental investigation has been done in the month of April 2015 for climate condition of Jabalpur, Madhya Pradesh, India (latitude 23° 18′ N; longitude 79° 95′ E) during full day, 0600 a.m. to 0600 p.m. The performance of the solar still with modification of water flow over the glass cover (sprinkler attachment) and nanoparticles (cuprous oxide) in basin water has been observed, recorded, and compared with conventional still. It has been found that the collection of pure water in modified solar sill was 4,000 ml/(m2-day) as compared to 2,900 ml/(m2-day) in conventional solar still. The efficiency of 34 and 22% has been obtained for modified solar still and conventional still, respectively. With design amendments, increase in overall effectiveness was found to be 54.54%. The computed cost of pure water produced in modified still is expected to (INR) Rs. 0.98/l, in view of 12-year life of the solar still.
Introduction
The available fresh water on earth is limited. More than two-third of the earth’s surface is covered with water but more than 97% of the available water is either salty or polluted. Rest nearly around 2.6% is fresh water. Less than 1% of fresh water is within reach of human and other organism. Even this small fraction is believed to be adequate to support life on earth but fresh water demand is increasing day by day due to increasing population (Tiwari et al., 2003; Kumar et al., 2015). Polluted water cannot be used directly for drinking purpose as it has harmful microbes and dissolved substance. Many developed and developing countries of the world are facing the problem of supply of drinking water and fresh water. The methods for production of fresh water used now a day are as follows: reverses osmosis, multi effect distillation, and mechanical vapor compression, etc. These methods involve major drawback of energy consumption in purification. Solar thermal desalination method is best suited for the fresh drinking water production at low cost. Interest in solar distillation systems is more because of their easy to operate and very less maintenance cost.
Identification of the Problem
Malik et al. (1982) have represented the conventional design of single-slope distillation system having a few disadvantage such as low efficiency and low amount of water distilled per unit area because of low temperature basin water. Sartori (1996) presented theoretical comparison between the thermal behaviors of basin type solar still and that from a solar evaporator. According to this, the evaporation in solar still in much less than that in open evaporation despite the higher water temperature in former system. It also observed that the distillation and evaporation rates increases with increase in water temperature and water difference for relatively higher water temperature of each system. The evaporative fraction is equivalent to more than 50% of the corresponding total heat transfer rate. He and Yan (2009) concluded the main reason of solar still having low productivity and efficiency over the conventional distillation process. The major challenges are rising the evaporation temperature and decreasing condensation temperature and latent heat of condensation should be released to atmosphere. Tiwari et al. (1985) analyzed the latent heat of vaporization of water lost to the surrounding by radiation and convection. This effect increases temperature difference between the glass cover and hot water basin by flowing of water on the glass cover. They show an improvement in performance of distilled output. Tripathi and Tiwari (2006) represented the thermal analysis of passive and active solar distillation system by using the concept of solar fraction inside the solar still. They observed that the solar fraction plays a very important role at lower values of solar altitude angle. The internal convective heat transfer coefficient decreases with the increase of water depth in the basin due to decrease in basin water temperature. Prasad and Tiwari (1996) analyzed double effect of active solar distillation unit and compared it with single effect of active solar distillation unit. Also, it has been observed that improving in the performance for a minimum flow rate of water on upper basin of the glass surface. Abu-Hijleh (1996) have been reported the effectiveness of film cooling under different–different conditions. The use of water film cooling on glass to improve the performance up to 6% was recommended. Abu-Hijleh and Mousa (1997) have been investigated numerically the proper use of film cooling may increase the solar still efficiency up to 20%. They show that small fraction of the cooling film evaporates over the glass surface. Gupta et al. (2015) have been reported that two experimental setups have been fabricated and their comparative performances have been evaluated under same ambient conditions. The conventional solar still gives 2.252 kg of distillate while modified solar still yields 2.645 kg of portable water. Overall efficiency of modified and conventional solar stills was calculated as 26.36 and 22.46%, respectively. It is observed that the increment in overall efficiency of modified still is 17.3%. Hence, the modified single-slope solar still is performed in better way. Abdalla (2013) observed the effect of the water flow over the glass cover in stepped solar still. The water productivity increases by 112% over conventional solar still. Tenthani et al. (2012) designed solar still with the inner surface of still were painted white. The distilled water outs were received 2.55 and 2.38 kg/m2 for experimental and conventional still, respectively. The thermal efficiency increased up to 6.5% over the conventional solar still. Madhlopa and Johnstone (2009) proposed a model that calculates the distribution of solar radiation inside a single-slope solar still. Solar fraction on a vertical surface is divided into beam and diffuse parts and the optical view factors of surfaces inside the solar still. The proposed model has been compared with the previous one. It has been found that the beam solar fraction is affected by both the geometry of the solar still and position of the sun in the sky. By contrast, the diffuse solar fraction is only dependent on the geometry of the solar distiller. Arunkumar et al. (2013) have concluded cold water and air flow on the glass cover to achieve highest productivity of water. Bhardwaj et al. (2013) reported glass is best material as a condensation surface in solar distillation system over any other material. It gives highest production of water as compared to other materials. Contact angle of covered glass is the most important factor to achieve higher productivity of water. Somwanshi and Tiwari (2014) evaluated the annual performance of conventional solar still with the water flow over the glass cover from the tank of air cooler and it has been found that improvement in annual yield between 41.3 and 56.5%. Increase in annual efficiency between 7.4 and 9.9% has been reported. Suneesh et al. (2014) investigated on V-type solar distillation system with and without cotton gauze top cover cooling (CGTCC). The pure water production was about 3,300 ml/m2-day for water flow over the glass cover without CGTCC and with CGTCC was 4,300 ml/m2-day. Kabeel et al. (2014a) used nano fluid (suspended nano-sized solid particle of aluminum oxide in water) to increase the heat transfer rate inside the solar basin. Nano fluid accelerates the water evaporation rate as compare with conventional solar still. An improvement of 166% in water productivity has been recorded in solar still integrated with external condenser. Kabeel et al. (2014b) have studied the effect of various nanoparticles on the performance of the solar still. The effect of nanoparticle concentration 0.008 and 0.12% by weight of water filled in the solar still basin on the performance of the solar still was significantly. The increase in the productivity of the modified solar still has been achieved up to 93.87%.
From above literature review, it is found that the yield of conventional/modified solar distillation system is low. This yield can be improved by controlling the operating parameters. Among various operation parameters, two critical parameters are most significant in performance improvement of single-slope solar, namely (i) solar radiation inside the water basin is not fully utilized because some solar radiation absorbed by the black painted vertical wall above the upper water surface and (ii) low temperature difference between glass cover and basin water temperature. In the present experimental study, three major modifications has been done: (i) walls of solar still have been coated in white paint to increase solar reflectivity of solar radiation inside basin, (ii) very thin water film is used on the glass cover to reduce the temperature of glass cover, and (iii) use of cuprous oxide nanoparticles (mixed with basin water) to increase the thermal conductivity of water. A critical experimental comparison of both solar stills has been done and discussed with respect to different operating parameters and economic viability.
Material and Methods
Experimental Setup
Two solar stills are designed and fabricated to compare performance under various conditions. The schematic view of the experimental set up used is shown in Figure 1. These stills have single basin solar still having basin area (100 cm × 100 cm) with high side wall of 52.77 cm and low side wall of 10 cm. Stills were fabricated from galvanized sheet having a thickness of 1 mm. The basin was insulated from outside and bottom by insulating material (glass wool) of thickness 4 cm for reduction of heat loss from the basin to the outside environment. Envelop of basin over the insulating material is supported from outside by wooden sheet having a thickness of 3 cm. In the conventional solar still, inner surfaces of bottom and side wall of the basin were coated in black to increase the absorption of solar energy. The top of basin is covered with 5 mm thick glass sheet inclined at nearly 23° with the horizontal. The modified solar still has same dimension as of conventional still. In the modified solar still, bottom surface is painted in black color to increase absorptivity of bottom surface, while inner side of vertical walls are coated with white paint to increase the reflectivity of the wall. Water sprinkler was used to flow water over the glass surface. Both solar stills have been sealed by insulating and adhesive tape at the top to prevent vapor leakage from the basin to atmosphere. Condensate from inner surface of glass is accumulated in channel at lower side of the basin and further collected to a jar connected to channel by plastic tube.
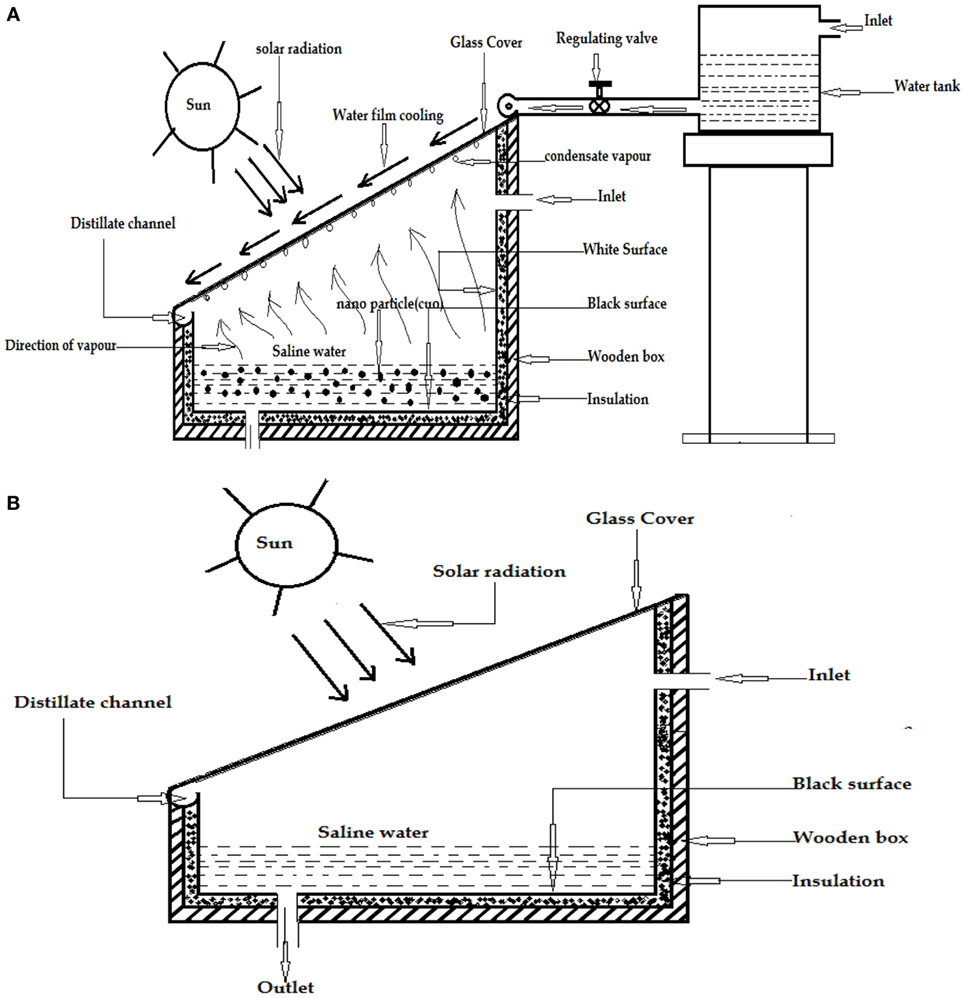
Figure 1. Schematic view of modified solar still and conventional solar still. (A) Modified solar still. (B) Conventional solar still.
Experimental Procedure
The experiment has been performed in month of April, 2015 at Jabalpur engineering college, Jabalpur (latitude 23° 10′ N; longitude 79° 55′ E) India. The experimental setup was kept facing south to receive maximum solar radiation. A photograph of the solar still experimental set up is shown in Figure 2. The experiments were performed during whole month of April 2015 but this paper analyses only data of a typical day with plenty of sunshine. The experiments were performed from 0700 to 1800 hours. Performance of modified solar still with cuprous oxide nanoparticle (0.12% weight concentration) mixed in basin water was compared with the conventional solar still. This concentration has been used by Kabeel et al. (2014b). The sprinkler was used to flow water at ambient temperature over the glass surface from period of 0800 to 1600 hours with the flow rate of 0.0001 kg/s. The flow rate was optimized by Somwanshi and Tiwari (2014). The depth of saline water is kept constant at 5 cm for the all sets of experiments.
Measuring Instruments
Several instruments were used to measure various performance depending parameters. The temperatures at different points of the still, such as water region, vapor region, inner glass surface, outer glass surface, and ambient temperature, have been measured by using k type thermocouple having least count 1°C. Thermocouples were connected to digital temperature indicator. The distillate output was measured by flask having capacity of 1,000 ml with least count of 10 ml. The total insolation was measured on glass cover with the help of data logging solar meter range 0–2,000 W/m2 with least count 0.1 W/m2.
Error Analysis
The experimental error has been found out in terms of percent uncertainty (internal + external) for the most sensitive parameter, i.e., distillate water. Experimental percentage uncertainty (U) is evaluate from Eqs 1 to 3 (Kumar and Tiwari, 2007):
where, σ is the SD expressed as:
where is the deviation from the mean and N and N0, number of set and number of observations in each set, respectively.
The external uncertainty is taken as the least count of the measuring instruments. The observed data for yield (distilled water) were found to be within the percent uncertainty of 11.04 and 12.02 for modified solar still and conventional solar still, respectively, which is quite acceptable.
Result and Discussions
Various parameters, such as ambient temperature, water temperature, vapor temperature, and glass temperature, solar radiation incident on the glass surface and distillate output, have been recorded hourly in this study at constant basin water depth. The experimental comparative study for performance evaluation has been done for modified solar still and the conventional solar still.
Climatological Conditions
The hourly profile of solar radiation and ambient temperature for a typical day are shown in Figure 3. The daily solar radiation received is in the range of 0–900 W/m2 and the ambient temperature is found during the project varying 28–37°C. The highest temperature and solar radiation occurs in between 1100 and 1300 hours as depicted from the Figure 3. The solar radiation profile increases in the morning time and obtain peak value nearly about mid-day. After the mid-day, solar radiation profile shows declination. The variation of ambient temperature also has similar nature to solar radiation profile.
Effect on Performance of Various Temperatures with Solar Radiation
Figure 4 shows the hourly variation of solar radiation, basin water temperature, and inner glass temperature and outer glass temperature with time for modified and conventional solar still. The temperature at various points, such as water temperature, vapor temperature, inner glass temperature and outer glass temperature, varies with the solar radiation. In the morning time, these temperatures increase with solar radiation and obtained higher values during period 1200–1400 hours. Solar rays get reflected from white coated wall in modified solar still and as a result availability of solar energy in still increases to a large extent. Vapor being a greenhouse gas traps more and more of it and makes evaporation faster. From Figure 4A, it is observed that the vapor temperature curve is above all till mid-day and after that the water temperature obtained higher value. Water flow over the glass cover from 0800 to 1600 hours reduces the temperature of glass cover and increases condensation simultaneously. Nanoparticles presented in water increases thermal conductivity and also work as storage medium. After mid-day, solar radiation as well as ambient temperature decreases. Nanoparticles maintain evaporation by releasing heat to water after mid-day.
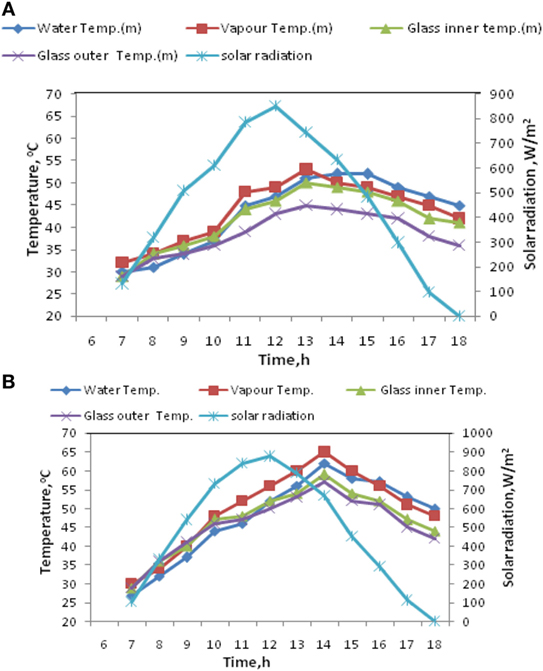
Figure 4. Hourly profile of solar radiation with various temperatures. (A) Modified solar still. (B) Conventional solar still.
In conventional solar still with black painted side walls, availability of energy to basin water enhances but absence of nanoparticles reduces rate of evaporation. Absence of sprinkler further reduces the condensation and as a cumulative result productivity of modified still has been better.
Distillate Productivity
Figure 5 shows the productivity curve for modified and conventional solar still with solar radiation and time of the day. The productivity of both still is zero till 1000 a.m. The curve shows increase in productivity after 1000 a.m. but productivity in modified still started earlier than conventional still. The productivity increases till after noon and then reduces with reduction in solar radiation. Nanoparticle liberate heat to water and increase in temperature of water takes place while water flow on the glass cover reduces temperature of glass cover. As a result, temperature difference takes place and condensation as well as productivity improved in modified still.
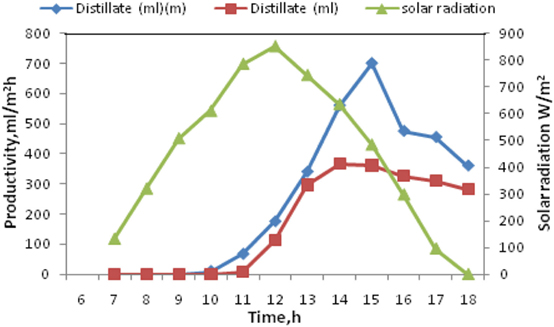
Figure 5. Hourly profile of productivity with solar radiation for modified and conventional solar still.
Efficiency of the Solar Stills
The efficiencies of modified solar still and conventional solar still are shown in Figure 6. The efficiency profile curve of modified solar still varies from zero value at the time nearly 1000 hours and achieve higher value after mid-day around 1400 hours. But, in conventional solar still, the efficiency profile was zero value around 1100 hours and achieved a higher value after 1400 hours. Significant value of efficiency is shown during 1000–1500 hours. Solar still takes times to reach steady-state condition from start to 1000 hours. After 1500 hours, instant efficiency gets affected from heat stored in water basin. Therefore, discussion on efficiency is focused during this period only.
The daily thermal efficiency (Eq. 4) of solar still is calculated by:
where
The above equation to calculate the efficiency (Tiwari et al., 2009) and the Eq. 5 is to calculate the latent heat of vaporization (Tiwari and Tiwari, 2006).
The daily efficiencies for modification solar still and conventional solar still are approximately 34 and 22%, respectively.
Cost Analysis
The use full life of the still (“n”) was taken as approximately 12 year and rate of interest (i) taken as 10%. The capital recovery factor (CRF) (Eq. 6) given by Somwanshi and Tiwari (2014).
The cost water produced annually is computed for Jabalpur climate Rs. 0.98/l.
Conclusion
The following conclusions have been drawn after three major modifications in conventional solar stills: (i) white painted wall surfaces, (ii) sprinklers attachment with water flow rate of 0.0001 kg/s, and (iii) using cuprous oxide nanoparticle (by weight 0.12% concentration) in the basin water.
• The productivity of modified solar still has been found increased using combined effect of cuprous oxide nanoparticle, water sprinkler attachment on the glass surface, and white painted vertical walls of the modified solar still.
• Computed daily yield for modified solar sill is 4,000 ml/(m2-day) and conventional solar still is 2,900 ml/(m2-day). The water productivity increased by 37.9%. These results show better performance of modified solar still in this study.
• The daily efficiency of modified solar still is obtained 34% and conventional solar still is 22%. Therefore, the increase in efficiency with design modifications was 54.54%.
• The cost of distillate in modified solar still has been computed to INR 0.98/l considering Jabalpur (India) climatic conditions which is worthwhile.
Nomenclature
Author Contributions
BG designed the experimental setup. AK did analysis of experimental data and formulate manuscript. PB reviewed the manuscript.
Conflict of Interest Statement
The authors declare that the research was conducted in the absence of any commercial or financial relationships that could be construed as a potential conflict of interest.
The reviewer, AM, and handling editor declared their shared affiliation, and the handling editor states that the process nevertheless met the standards of a fair and objective review.
References
Abdalla, A. (2013). Improving the performance of stepped solar still. Desalination 319, 60–65. doi: 10.1016/j.desal.2013.04.003
Abu-Hijleh, B. (1996). Enhanced solar still performance using water film cooling of the glass cover. Desalination 107, 235–244. doi:10.1016/S0011-9164(96)00165-8
Abu-Hijleh, B., and Mousa, H. A. (1997). Water film cooling over the glass cover of a solar still including evaporation effects. Energy 22, 43–48. doi:10.1016/S0360-5442(96)00088-6
Arunkumar, T., Jayaprakash, R., Ahsanb, A., Denkenberger, D., and Okundamiya, M. (2013). Effect of water and air flow on concentric tubular solar water desalting system. Appl. Energy 103, 109–115. doi:10.1016/j.apenergy.2012.09.014
Bhardwaj, R., Kortenaar, M., and Mudde, R. (2013). Influence of condensation surface on solar distillation. Desalination 326, 37–45. doi:10.1016/j.desal.2013.07.006
Gupta, B., Baredar, P., Shanka, P., and Sharma, R. (2015). “Comparative experimental investigation on modified and conventional single slope solar still,” in Proceedings of the International Conference on Computational Heat and Mass Transfer (Warangal, India: National Institute of Technology), Paper No. 1440080209.
He, T., and Yan, L. (2009). Application of alternative energy integration technology in sea water distillation. Desalination 249, 104–108. doi:10.1016/j.desal.2008.07.026
Kabeel, A., Omara, Z., and Essa, F. (2014a). Enhancement of modified solar still integrated with external condenser using nano fluids: an experimental approach. Energy Convers. Manag. 78, 493–498. doi:10.1016/j.enconman.2013.11.013
Kabeel, A., Omara, Z., and Essa, F. (2014b). Improving the performance of solar still by using nano fluids and providing vacuum. Energy Convers. Manag. 86, 268–274. doi:10.1016/j.enconman.2014.05.050
Kumar, A., and Tiwari, G. N. (2007). Effect of mass on convective mass transfer coefficient during open sun and greenhouse drying of onion flakes. J. Food Eng. 79, 1337–1350. doi:10.1016/j.jfoodeng.2006.04.026
Kumar, P., Kumar, A., Prakash, O., and Kaviti, A. (2015). Solar stills system design: a review. Renew. Sustain. Energ. Rev. 51, 153–181. doi:10.1016/j.rser.2015.04.103
Madhlopa, A., and Johnstone, C. (2009). Model for computation of solar fraction in a single-slope solar still. Solar Energy 83, 873–882. doi:10.1016/j.solener.2008.12.002
Malik, M., Tiwari, G., Sodha, M., and Kumar, A. (1982). Solar energy conversion and photo energy systems. Solar Distillation 2, 1–10.
Prasad, B., and Tiwari, G. (1996). Analysis of double effect active solar distillation. Energy Convers. Manag. 37, 1947–1956. doi:10.1016/0196-8904(95)00359-2
Sartori, E. (1996). Solar still versus solar evaporator: a comparative study between their thermal behaviors. Solar Energy 56, 199–206. doi:10.1016/0038-092X(95)00094-8
Somwanshi, A., and Tiwari, A. (2014). Performance enhancement of a single basin solar still with flow of water from air cooler on the cover. Desalination 352, 92–102. doi:10.1016/j.desal.2014.08.011
Suneesh, P., Jayaprakash, R., Arunkumar, T., and Denkenberger, D. (2014). Effect of air flow on “V” type solar still with cotton gauze cooling. Desalination 337, 1–5. doi:10.1016/j.desal.2013.12.035
Tenthani, C., Madhlopa, A., and Kimambo, C. (2012). Improved solar still for water purification. J. Sustainable Energy Environ. 3, 111–113.
Tiwari, A., and Tiwari, G. (2006). Effect of water depths on heat and mass transfer in a passive solar still: in summer climatic condition. Desalination 195, 78–94. doi:10.1016/j.desal.2005.11.014
Tiwari, G., Dimri, V., and Chel, A. (2009). Parametric study of an active and passive solar distillation system: energy and exergy analysis. Desalination 242, 1–18. doi:10.1016/j.desal.2008.03.027
Tiwari, G., Madhuri, and Garg, H. (1985). Effect of water flow on the glass cover of the single basin solar still with an intermittent flow of waste hot water in the basin. Energy Convers. Manag. 25, 315–322. doi:10.1016/0196-8904(85)90049-4
Tiwari, G., Singh, H., and Tripathi, R. (2003). Present status of solar distillation. Solar Energy 75, 367–373. doi:10.1016/j.solener.2003.07.005
Keywords: solar still, water distillation, sprinkler attachment, nanoparticles (cuprous oxide), cost analysis
Citation: Gupta B, Kumar A and Baredar PV (2017) Experimental Investigation on Modified Solar Still Using Nanoparticles and Water Sprinkler Attachment. Front. Mater. 4:23. doi: 10.3389/fmats.2017.00023
Received: 13 March 2017; Accepted: 06 July 2017;
Published: 02 August 2017
Edited by:
Sravendra Rana, University of Petroleum and Energy Studies, IndiaReviewed by:
Jamil Muhammad, Konkuk University, South KoreaAmit Kumar Mondal, University of Petroleum and Energy Studies, India (Surajit Mondal contributed to the review of Amit Kumar Mondal)
Copyright: © 2017 Gupta, Kumar and Baredar. This is an open-access article distributed under the terms of the Creative Commons Attribution License (CC BY). The use, distribution or reproduction in other forums is permitted, provided the original author(s) or licensor are credited and that the original publication in this journal is cited, in accordance with accepted academic practice. No use, distribution or reproduction is permitted which does not comply with these terms.
*Correspondence: Bhupendra Gupta, Ymh1cGVuZHJhMjQzQHlhaG9vLmNvbQ==