- School of Materials Science and Engineering, Zhejiang University, Hangzhou, China
Light-matter interactions are greatly enhanced and spatially well-confined in plasmonic nanostructures in the spectral resonance range. In colloidal plasmonic nanoparticle (NP), this effect is manifested by the strong absorption caused by the collective oscillation of conduction electrons in NPs, known as the localized surface plasmon resonance (LSPR). The strong spectral resonance due to LSPR also contributes to the large enhancement in optical nonlinearity, such as nonlinear absorption and optical Kerr effect, and the ultrafast transient photo-bleaching in those materials making them ideal platforms for exploring optical switching behavior that is needed in all optical devices. Here, we review the recent advances in the use of colloidal processed noble metal, metalloid, chalcogenide and oxide plasmonic NPs for ultrafast optical switches to drive pulse laser generation in both solid state and fiber lasers. The broadband and ultrafast response of these plasmonic NPs offers a significant advantage in devices performance and allows the pulse generation from near infrared (NIR) to middle infrared (MIR) spectral range with pulse duration down to 100-femtosecond level. Compared with commercial products and other optical switches based on 2D materials, these plasmonic NPs are expected to provide a competitive and cost-efficient material solution to compact ultrafast pulse lasers and relevant devices.
Introduction
Ultrafast pulse lasers that generate optical pulses with durations down to few femtoseconds (fs) have been applied in diverse fields, from fundamental sciences, such as precision spectroscopy, to large scale manufacturing like laser welding in industry (Keller, 2003). Pulse lasers employ different type of optical switches that compress constant laser wave to pulses by two mechanisms: Q-switching and mode-locking. For most compact fiber and solid state lasers, saturable absorber (SA) based optical switches are regarded as the compact and cost-efficient choice as compared to other switching devices including electric or acoustic switches as well as Kerr lens. Due to the nonlinear absorption of SAs, the switch blocks the low energy pulse which leads to accumulation of energy in the cavity, but becomes transparent and thus allows the output of high energy pulses by the laser.
Saturable absorption has been demonstrated in a range of materials, from transition metal (TM) doped glasses, organic dye solutions to different type of low dimensional materials (Liu et al., 2017), such as carbon nanotubes (Wang et al., 2008), graphenes (Bao et al., 2009), 2D semiconductors (Wang S. X. et al., 2014), and topological insulators and semimetals (Yu et al., 2013; Zhu et al., 2017). The process of saturable absorption is a typical nonlinear optical process and it can be simply understood by using the two-level model, in which the transient depletion of the ground state by the high energy pulse results in photo-bleaching and thus saturation of absorption. While many of the above materials show only limited saturable absorption bandwidth, such as TM doped glasses organic dye solutions and semiconductor based commercial product SESAM (semiconductor saturable absorption mirror) (Keller et al., 1996), gapless materials like graphene and semimetals show intrinsic broadband non-linear optical response, and therefore can be applied as optical switches operating from visible to mid-infrared (MIR) spectral range for the generation of mode-locking and Q-switching pulses (Sobon, 2015).
These gapless materials including Dirac materials like graphene generally derive their optical nonlinearity from the ultrafast thermalization and cooling of conduction electrons following optical excitation (Breusing et al., 2011; Lim et al., 2011; Baudisch et al., 2018), which is mechanistically similar to the ultrafast carrier relaxation process in metallic plasmonic nanostructures (Link and El-Sayed, 1999; Voisin et al., 2001). The saturable absorption associated with transient photo-bleaching in the sub-picosecond range makes them ideal candidate materials to realize ultrafast optical switching. In the present review, we discuss the recent advances in the application of noble metal, chalcogenide, and oxide colloidal plasmonic NPs in ultrafast optical switching for pulse laser generation from near infrared (NIR) to MIR spectral range.
General Properties of Colloidal Plasmonic Nanoparticles
Colloidal plasmonic NPs of noble metals, such as gold, are characterized by the reddish color due to LSPR that is located at an energy dependent on the carrier density, geometric factors including size and shape, and the dielectric environment. According to the Drude model, the frequency of LSPR can be expressed as:
where is the plasma frequency (N, e, ε0, and me are the carrier density, electron charge, free space permittivity and electron mass, respectively). εm is the permittivity of the surrounding environment and γ is the damping factor that contains the contribution from size and shape. A more detailed mathematical description of the shape functions of LSPR can be found in several comprehensive reviews (Kelly et al., 2003; Link and Ei-Sayed, 2003). Spherical NPs of gold and silver are best known for their visible region LSPR, while the LSPR can be extended to the NIR region for rod and triangle shaped nanostructures. To move the LSPR to the infrared region that is technically important for applications like optical communication, an alternative strategy is to explore those materials with a lower carrier density and therefore smaller plasma frequency. Such materials are apparently “bad metals,” which include intermetallic compounds and metalloid compounds (Blaber et al., 2010; Naik et al., 2013), as well as degenerate semiconductors upon heavy doping, such as copper chalcogenide (Luther et al., 2011), and transparent conducting oxides (TCO) (Agrawal et al., 2017; Coughlan et al., 2017) (Figure 1).
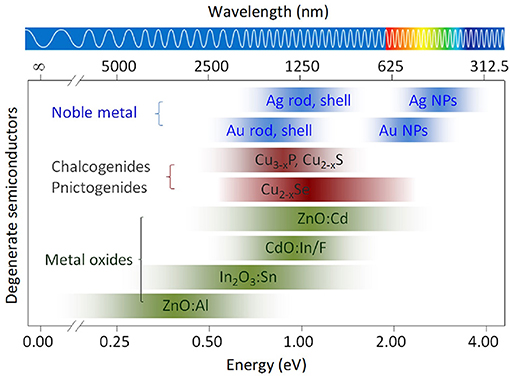
Figure 1. Spectral regions of LSPR for different type of nobel metal, chalcogenide/pnictogenide, and metal oxide based plasmonic materials. Except noble metal based nanostructures, all the plasmonic materials are assumed to have a spherical shape and the change of LSPR is mainly determined by the carrier density.
Short pulse laser excitation of the plasmonic NPs directly heats the conduction electrons, leading to the formation of non-equilibrium Fermi-Dirac distribution of thermalized electrons (Link and El-Sayed, 1999). The followed cooling process involves several stages including electron-electron scattering, electron-phonon scattering and phonon-phonon scattering, with the rate determined by both intrinsic materials properties like electron density and also the surrounding environment. Experimentally, this process can be examined by transient absorption spectroscopy based on a pump-probe setup with the use of ultrafast fs lasers. The information contained in the decay curves can be simply extracted by fitting with the double-exponential decay function, which gives two distinct time constant in time scale of sub-50 fs and 200-fs range. Therefore, it has been widely accepted that this process can be described by the two-temperature model (Sun et al., 1993; Rethfeld et al., 2002), i.e., a much higher electron temperature and the lower lattice temperature. Typically, the faster and the slower processes are interpreted as electron-electron and electron-phonon (or phonon-phonon) scattering. Due to the lower carrier density in non-noble metal based plasmonic materials, cooling rate of hot electrons is generally faster because of the lower electron heat capacity.
The ultrafast transient bleaching in the plasmonic nanostructures is manifested in the strong nonlinear optical absorption (saturable absorption), which can be described by the two-level model (Liu et al., 2017):
where, αs and αns are the saturable loss (modulation depth) and non-saturable-loss, respectively, and Isat is the saturable intensity. Experimentally, this process can be measured by the open-aperture Z-scan experiments, and combined with the close-aperture experiments the non-linear refractive index (n2) and the third order susceptibility (χ(3)) can be derived.
The resonant optical nonlinearity of these colloidal plasmonic NPs stems from the ultrafast response of the conduction electrons and in the off-resonance region the optical nonlinearity becomes pretty small. In the past decade, noble metal based plasmonic materials have been extensively studied and the reported third order susceptibility differs from sample to sample, roughly in the range of 10−5-10−6 esu (Stepanov, 2011; Obermeier et al., 2018). Concerning the nonlinear absorption, most experiments demonstrate that these plasmonic NPs are saturable absorbers, and the optical nonlinearity is also dependent on the sample geometry such as size and shape. Particularly, for anisotropic nanostructure like nanorods or aligned rod arrays, the optical nonlinearity as well as the ultrafast response becomes polarization dependent. In line with the linear absorption, both the third order susceptibility and the modulation depth are maximum when the laser polarization is parallel with axial direction of the rods (Wang et al., 2006; Zhang et al., 2016).
Noble Metal Based Colloidal Plasmonic NPs For Ultrafast Lasers
The ultrafast nonlinear response combined with the high optical modulation depth makes the colloidal plasmonic NPs highly attractive for use as the working materials in optical switches. When used as the switching materials in pulse lasers, it is believed that the fs-level short relaxation time component can facilitate pulse start, whereas the long picosecond (ps)-scale time component is needed for the stabilization of pulse train.
In the first demonstration of optical switching using metal NPs, a suspension of Pt NPs was used directly and inserted into the laser cavity in which an Nd-doped glass was used as the gain medium (Ganeev et al., 2009). The laser generated mode-locked pulses with a pulse duration of 5 ps and a repetition rate of 1 Hz at 1054 nm. Comparative experiments conducted by the author showed the Pt NP suspensions exhibited better stability than dye doped films when used as the SA in pulse lasers. Following this demonstration, the theoretical basis for the mode-locked pulse generation based on the plasmonic NP switches was established by Kim et al. who suggested a design criteria for SAs based on metal NPs and concluded that the minimum pulse duration can be much shorter than recovery time of the SA (Kim et al., 2012b). In their simulation, an Au-colloid (in the form of Au NP doped glass) was employed as SA to drive a Ho:YLF mode-locking laser. At the emission wavelength of 545 nm (by the Ho: 5S2 → 5I8 transition), the shortest pulse duration is around 100 fs, which is much smaller than the recovery time of the SA. In semiconductor disk lasers operating in blue spectral region, Kim et al. also predicted theoretically that the pulse duration can be down to 50 fs at 420 nm based on passive mode-locking by using SAs based on metal NPs (Kim et al., 2012a), while this prediction has not yet been confirmed experimentally.
Later, a Q-Switching fiber laser was demonstrated by Jiang et al by using a composite film containing colloidal processed Au NPs with an average size of around 30 nm (Jiang et al., 2012). The optical switch was made by insertion of the Au-NP doped film in between two fiber connectors, which was incorporated within an Er-doped fiber laser cavity. Passive Q-switched pulse output at 1560 nm was demonstrated at a pumping power higher than 30 mW and a pulse duration of 3.2 μs with a repetition rate of 24.2 kHz was observed at the pumping power of 125 mW. However, mode-locking was not demonstrated in this laser possibly due to the small modulation depth at the lasing wavelength that is far from the LSPR peak of Au NPs. In the visible range where the LSPR of gold NPs are in resonance with the laser wavelength, Q-switching fiber laser has also been demonstrated by using similar SAs based on a Pr-doped fiber (Wu et al., 2015).
To extend the operating wavelength of the Au-based SA to the NIR, it is quite natural to use gold nanorods whose LSPR is located in IR range and dependent on the aspect ratio and rod diameter. By using a SA based on colloidal processed gold nanorods (with an aspect ratio of 5.5), Kang et al. demonstrated mode-locking laser pulse generation based on a similar Er-doped fiber laser cavity (Kang et al., 2013). A threshold pump power of 54 mW was needed to generate a stable pulse train with a repetition rate of 34.7 MHz and pulse duration of 12 ps. This is apparently not a transform-limited pulse, but typical for dissipative soliton pulse, as can be expected from the large time-bandwidth product. Soliton fiber lasers based on Er-doped gain fibers that generate shorter pulse duration down to the sub-ps level were reported later by the same group and other groups by further dispersion management and optimization of cavity design (Wang X. D. et al., 2014; Wang et al., 2016). Later, SAs based on other noble metal (such as silver) based plasmonic NPs were also employed for different fiber lasers in the NIR spectral region (Glubokov et al., 2014; Guo H. et al., 2016).
As the spectral response of gold nanorods covers a broad spectral region in the infrared region, it is easy to extend the operating wavelength of such noble metal NP based optical switches to drive mode-locking pulse output in fiber lasers based on, e. g., Yb-doped fiber at 1.0 μm (Kang et al., 2014), and Tm-doped fiber at 2.0 μm (Kang et al., 2015). On the other hand, the geometrical anisotropy of gold nanorods provides another dimension to modulate pulse generation in fiber lasers. In a recent report by Ma et al. it is demonstrated that the use of aligned gold nanorods stabilized in a polymer matrix as the SA enables the switching between Q-switching (of 46.9 kHz) and mode-locking (20.2 MHz) pulses output by simply changing the polarization direction of the laser (Ma et al., 2018). This is because the saturation modulation depth and optical nonlinearity of the aligned gold nanorods are both strongly dependent on the angle between the laser polarization and the axial direction of the nanorods (Ma et al., 2018).
Pulse Lasers Driven By Plasmonic NPs Beyond Noble Metals
To move the LSPR band to the IR spectral region, materials with a lower carrier density appears to be favorable choices, such as degenerate semiconductors and metalloid compounds. Monovalent copper chalcogenides as well as pnictogenides are typical degenerate semiconductors, which remain stabilized with high copper deficiency that leads to heavy p-type doping. Liu et al. reported in 2016 the NIR LSPR and the nonlinear optical properties of colloidal Cu3−xP NPs (Liu et al., 2016). The LSPR peak is tunable between 1390 and 1710 nm by controlling the synthesis conditions. Measurements showed that a thin film of Cu3−xP NPs exhibited a high modulation depth of 19% with a 100-fs response speed. The colloidal Cu3−xP NPs was coated onto the fiber end-facet of a Er-doped fiber laser and act as the optical switch, which enabled Q-switching pulse output at the 1.5 μm band. The Q-switched pulses were generated at the threshold pumping power of 35 mW and the repetition rate can be tuned between 5.817 and 30.35 kHz.
Mode-locked pulses generation was demonstrated later by Guo et al. based on copper-deficient chalcogenide NPs (Guo et al., 2016a,b; Guo H. et al., 2016). The copper chalcogenide systems offer even wider tenability of carrier density by both controlling the stoichiometry and the alloying effect. Their results indicated that the peak of LSPR of Cu2−xS NPs can be finely tuned between 1100 and 1500 nm with a bandwidth of over 400 THz. The spectral resonance determines the magnitude of optical nonlinearity and parameters such as modulation depth. For the Cu2−xS NPs with a LSPR peak at 1.3 μm, the modulation depth varies among 2 and 6.8 dB in the spectral range of 0.8–1.6 μm and exhibits a parabolic wavelength dependence with a peak value of 6.8 dB reached at 1.3 μm. Interestingly, at wavelengths far from the LSPR peak, the Cu2−xS NPs film still shows saturable absorption with high modulation depth. To drive these lasers the Cu2−xS NPs were processed into different type of switches. In the 1.5 μm Er-doped fiber laser, a micro-fiber based switch was employed in which the NPs was coated onto the fiber surface and this fiber-based switch was incorporated into the fiber laser cavity. Compared with thin-film based SAs, this micro-fiber switch based on evanescent field interaction could potentially suppress thermal damage of the working materials and thus increase the maximum operating power of the laser (Martinez and Sun, 2013). This method has been widely applied in optical switches based on different 2D materials, including graphene, metal chalcogenide, and black phosphorus (Sobon, 2015; Lee et al., 2016). This laser generates mode-locked pulses with a pulse duration of 295 fs and repetition rate of 7.28 MHz. In comparison, thin film SAs were fabricated and used to drive a solid state laser (using a Yb:LuAG as the gain medium) that produces mode-locking ps-level pulse at 1.0 μm, and a Er-doped ZBLAN fiber laser that generates Q-switching pulses at 2.8 μm.
In spite of the apparent performances of these noble metal and chalcogenide based optical switches, the use of them in high power lasers raises concerns on the stability as they are not stable against thermal shock and oxidation. In this regards, oxide based plasmonic NPs appears to be a better choice. Driven by the potential application of solution processed devices, colloidal synthesis of oxide plasmonic NPs based on, e.g., ITO (In2O3:Sn), has been well-developed in the past decades (Liang et al., 2017). On the other hand, TCOs like ITO have become of particular interest recently as their permittivity crossover wavelength is located in the NIR region. This wavelength is close to the bulk plasma wavelength and materials including ITO are regarded as the ENZ (epsilon-near-zero) media which have been intensively exploited for photonics applications (Liberal and Engheta, 2017; Niu et al., 2018). The ENZ character can also lead to the boost in optical nonlinearity (or Kerr nonlinearity) as the change in refractive index varies as , where ε is the permittivity (Alam et al., 2016; Caspani et al., 2016). At the ENZ wavelength, both the non-linear absorption and the non-linear refractive index are maximum, making this material highly suitable for applications in optical switches.
We have examined the non-linear optical properties of colloidal processed ITO NPs (see Figure 2), and confirmed the saturable absorption at the ENZ wavelength (1300–1600 nm) which gives a modulation depth of 160% (Guo et al., 2017). The enhanced optical nonlinearity can be interpreted by the synergy effect of ENZ conditions and the field enhancement by LSPR. The ITO NPs were processed into composite films by mixing with PVA (polyvinyl alcohol) and used as the optical switch to drive mode-locked pulse generation in an Er-doped fiber laser. We obtained the shortest pulse duration of 593 fs at the 1.5 μm band (Figure 2). Similar to ITO, other TCOs like AZO (ZnO:Al) with lower carrier density can be used for MIR optical switch, which is now under investigation. The significance of these results is two-fold: First, it provides a competitive materials solution for optical switch used in pulse lasers that is also viable for modern thin film fabrication processes. Second, this result opens up a new avenue for exploiting the applications of conventional oxides in nonlinear optics and ultrafast photonics.
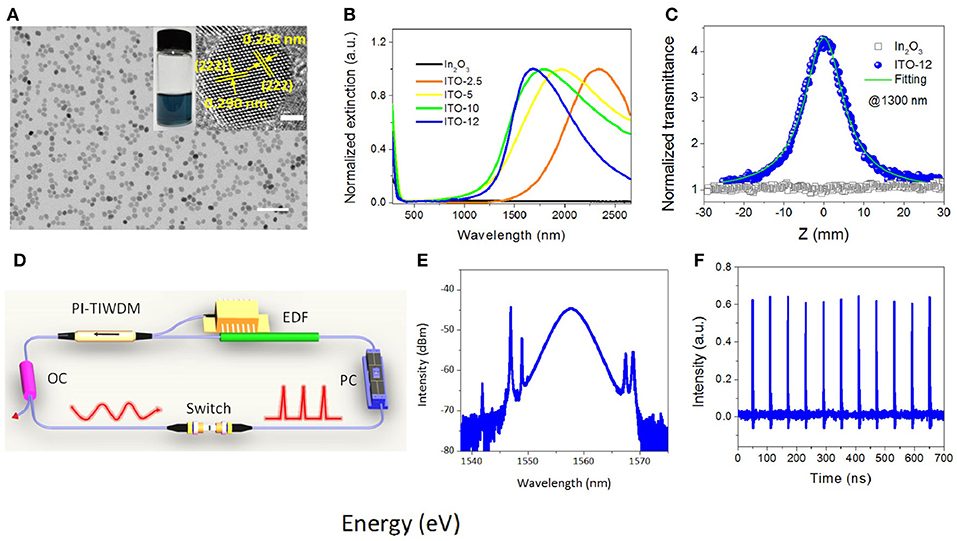
Figure 2. (A) Typical TEM images of colloidal ITO NPs, with an average diameter of ca. 9 nm. The Inset shows a photograph of the colloidal solution of ITO NPs and a high resolution TEM image of a single ITO NP. (B) Normalized absorption spectra of colloidal dispersions of ITO NPs doped with 0–12% SnO2 (denoted as In2O3, ITO-2.5, ITO-5, ITO-10, and ITO-12). (C) Z-scan trace of a PVA film containing ITO NPs recorded at 1.3 μm. ITO-12 shows notable saturable absorption, as compared to the undoped In2O3. (D) Setup of an EDF laser for mode-locking pulse generation (OC: output coupler; EDF: Er-doped fiber; PI-TIWDM: polarization-independent tap-isolator-wavelength-division multiplexer). (E) Mode-locked pulse laser spectrum. (F) Mode-locked pulse train, giving a repetition rate of 16.62 MHz.
Whereas the LSPR of NPs of ITO and other TCOs are located in wavelength mostly longer than 1.3 μm, oxides of W(VI) and Mo(VI) are known to stable with high concentration of oxygen deficiency and thus n-type doping. Plasmonic NPs of WO3−x and MoO3−x show LSPR in the visible to NIR spectral region (Manthiram and Alivisatos, 2012; de Castro et al., 2017). Nonlinear optical study performed recently confirmed the saturable absorption of MoO3−x films at 1.0 μm with 100-fs level fast response time (Wang et al., 2018). The MoO3−x based switch enabled the demonstration of a mode-locking pulse laser based on a Yb-doped fiber operating at 1.03 μm.
Concluding Remarks
I have discussed the recent advances in the development of optical switches for pulse laser pulse generation based on diverse colloidal plasmonic NPs. Compared with commercial products based on semiconductor thin films, the silent feature of the discussed materials and devices are obvious: low fabrication cost, wide operating bandwidth, and fast switching speed. However, commercialization of the relevant devices still faces significant technical challenges, including large scale fabrication, and chemical/thermal stability of materials. The use of micro-fiber based design could mitigate in part the laser thermal damage of the materials, while colloidal NPs still need to the protected against oxidation for practical use. In this regard, oxide plasmonic materials are far better and they can be fabricated in large quantity by commercial-viable techniques, which indeed could be a potential future direction. Nevertheless, device parameters, such as non-saturable loss and modulation depth, which are both wavelength-dependent, remain to be optimized for the reported materials as they are important for practical use in different pulse lasers.
Author Contributions
The author confirms being the sole contributor of this work and has approved it for publication.
Conflict of Interest Statement
The author declares that the research was conducted in the absence of any commercial or financial relationships that could be construed as a potential conflict of interest.
Acknowledgments
The author thanks the financial support of the National Natural Science Foundation of China (Grant No. 11504323 and 61775192) and the Open fund of the State Key lab of Fine ceramics, Shanghai Institute of Ceramics, Chinese Academy of Sciences (Grant No. SKL201706SIC).
References
Agrawal, A., Johns, R. W., and Milliron, D. J. (2017). Control of localized surface plasmon resonances in metal oxide nanocrystals. Annu. Rev. Mater. Res. 47, 1–31. doi: 10.1146/annurev-matsci-070616-124259
Alam, M. Z., De Leon, I., and Boyd, R. W. (2016). Large optical nonlinearity of indium tin oxide in its epsilon-near-zero region. Science 352, 795–797. doi: 10.1126/science.aae0330
Bao, Q. L., Zhang, H., Wang, Y., Ni, Z. H., Yan, Y. L., Shen, Z. X., et al. (2009). Atomic-layer graphene as a saturable absorber for ultrafast pulsed lasers. Adv. Funct. Mater. 19, 3077–3083. doi: 10.1002/adfm.200901007
Baudisch, M., Marini, A., Cox, J. D., Zhu, T., Silva, F., Teichmann, S., et al. (2018). Ultrafast nonlinear optical response of Dirac fermions in graphene. Nat. Commun. 9:1018. doi: 10.1038/s41467-018-03413-7
Blaber, M. G., Arnold, M. D., and Ford, M. J. (2010). A review of the optical properties of alloys and intermetallics for plasmonics. J. Phys. Condens. Mat. 22:143201. doi: 10.1088/0953-8984/22/14/143201
Breusing, M., Kuehn, S., Winzer, T., Malic, E., Milde, F., Severin, N., et al. (2011). Ultrafast nonequilibrium carrier dynamics in a single graphene layer. Phys. Rev. B 83:153410. doi: 10.1103/PhysRevB.83.153410
Caspani, L., Kaipurath, R. P. M., Clerici, M., Ferrera, M., Roger, T., Kim, J., et al. (2016). Enhanced nonlinear refractive index in epsilon-near-zero materials. Phys. Rev. Lett. 116:233901. doi: 10.1103/PhysRevLett.116.233901
Coughlan, C., Ibanez, M., Dobrozhan, O., Singh, A., Cabot, A., and Ryan, K. M. (2017). Compound copper chalcogenide nanocrystals. Chem. Rev. 117, 5865–6109. doi: 10.1021/acs.chemrev.6b00376
de Castro, I. A., Datta, R. S., Ou, J. Z., Castellanos-Gomez, A., Sriram, S., Daeneke, T., et al. (2017). Molybdenum oxides - from fundamentals to functionality. Adv. Mater. 29:1701619. doi: 10.1002/adma.201701619
Ganeev, R. A., Tugushev, R. I., and Usmanov, T. (2009). Application of the nonlinear optical properties of platinum nanoparticles for the mode locking of Nd:glass laser. Appl. Phys. B-Lasers O. 94, 647–651. doi: 10.1007/s00340-009-3371-9
Glubokov, D. A., Sychev, V. V., Mikhailov, A. S., Korolkov, A. E., Chubich, D. A., Shapiro, B. I., et al. (2014). Saturable absorber based on silver nanoparticles for passively mode-locked lasers. Quantum Electron 44, 314–316. doi: 10.1070/QE2014v044n04ABEH015353
Guo, H., Feng, M., Song, F., Li, H. Y., Ren, A. B., Wei, X. K., et al. (2016). Q-switched erbium-doped fiber laser based on silver nanoparticles as a saturable absorber. IEEE Photon. Tech. L. 28, 135–138. doi: 10.1109/LPT.2015.2487521
Guo, Q. B., Cui, Y. D., Yao, Y. H., Ye, Y. T., Yang, Y., Liu, X. M., et al. (2017). A solution-processed ultrafast optical switch based on a nanostructured epsilon-near-zero medium. Adv. Mater. 29:1700754. doi: 10.1002/adma.201700754
Guo, Q. B., Ji, M. X., Yao, Y. H., Liu, M., Luo, Z. C., Zhang, S., et al. (2016a). Cu-Sn-S plasmonic semiconductor nanocrystals for ultrafast photonics. Nanoscale 8, 18277–18281. doi: 10.1039/C6NR05954F
Guo, Q. B., Yao, Y. H., Luo, Z. C., Qin, Z. P., Xie, G. Q., Liu, M., et al. (2016b). Universal near-infrared and mid-infrared optical modulation for ultrafast pulse generation enabled by colloidal plasmonic semiconductor nanocrystals. ACS Nano 10, 9463-9469. doi: 10.1021/acsnano.6b04536
Jiang, T., Xu, Y., Tian, Q. J., Liu, L., Kang, Z., Yang, R. Y., et al. (2012). Passively Q-switching induced by gold nanocrystals. App. Phys. Lett. 101:151122. doi: 10.1063/1.4759120
Kang, Z., Li, Q., Gao, X. J., Zhang, L., Jia, Z. X., Feng, Y., et al. (2014). Gold nanorod saturable absorber for passive mode-locking at 1 mu m wavelength. Laser Phys. Lett. 11:035102. doi: 10.1088/1612-2011/11/3/035102
Kang, Z., Liu, M. Y., Gao, X. J., Li, N., Yin, S. Y., Qin, G. S., et al. (2015). Mode-locked thulium-doped fiber laser at 1982 nm by using a gold nanorods saturable absorber. Laser Phys. Lett. 12:045105. doi: 10.1088/1612-2011/12/4/045105
Kang, Z., Xu, Y., Zhang, L., Jia, Z. X., Liu, L., Zhao, D., et al. (2013). Passively mode-locking induced by gold nanorods in erbium-doped fiber lasers. App. Phys. Lett. 103:041105. doi: 10.1063/1.4816516
Keller, U. (2003). Recent developments in compact ultrafast lasers. Nature 424, 831–838. doi: 10.1038/nature01938
Keller, U., Weingarten, K. J., Kartner, F. X., Kopf, D., Braun, B., Jung, I. D., et al. (1996). Semiconductor saturable absorber mirrors (SESAM's) for femtosecond to nanosecond pulse generation in solid-state lasers. IEEE J. Sel. Top. Quant. 2, 435–453. doi: 10.1109/2944.571743
Kelly, K. L., Coronado, E., Zhao, L. L., and Schatz, G. C. (2003). The optical properties of metal nanoparticles: The influence of size, shape, and dielectric environment. J. Phys. Chem. B 107, 668–677. doi: 10.1021/jp026731y
Kim, K. H., Griebner, U., and Herrmann, J. (2012a). Theory of passive mode-locking of semiconductor disk lasers in the blue spectral range by metal nanocomposites. Opt. Express 20, 16174–16179. doi: 10.1364/OE.20.016174
Kim, K. H., Griebner, U., and Herrmann, J. (2012b). Theory of passive mode locking of solid-state lasers using metal nanocomposites as slow saturable absorbers. Opt. Lett. 37, 1490–1492. doi: 10.1364/OL.37.001490
Lee, D., Park, K., Debnath, P. C., Kim, I., and Song, Y. W. (2016). Thermal damage suppression of a black phosphorus saturable absorber for high-power operation of pulsed fiber lasers. Nanotechnology 27:365203. doi: 10.1088/0957-4484/27/36/365203
Liang, X. Y., Bai, S., Wang, X., Dai, X. L., Gao, F., Sun, B. Q., et al. (2017). Colloidal metal oxide nanocrystals as charge transporting layers for solution-processed light-emitting diodes and solar cells. Chem. Soc. Rev. 46, 1730–1759. doi: 10.1039/C6CS00122J
Liberal, I., and Engheta, N. (2017). Near-zero refractive index photonics. Nat. Photonics 11, 149–158. doi: 10.1038/nphoton.2017.13
Lim, G. K., Chen, Z. L., Clark, J., Goh, R. G. S., Ng, W. H., Tan, H. W., et al. (2011). Giant broadband nonlinear optical absorption response in dispersed graphene single sheets. Nat. Photonics 5, 554–560. doi: 10.1038/nphoton.2011.177
Link, S., and Ei-Sayed, M. A. (2003). Optical properties and ultrafast dynamics of metallic nanocrystals. Ann. Rev. Phys. Chem. 54, 331–366. doi: 10.1146/annurev.physchem.54.011002.103759
Link, S., and El-Sayed, M. A. (1999). Spectral properties and relaxation dynamics of surface plasmon electronic oscillations in gold and silver nanodots and nanorods. J. Phys. Chem. B 103, 8410–8426. doi: 10.1021/jp9917648
Liu, X. F., Guo, Q. B., and Qiu, J. R. (2017). Emerging low-dimensional materials for nonlinear optics and ultrafast photonics. Adv. Mater. 29:1605886. doi: 10.1002/adma.201605886
Liu, Z. K., Mu, H. R., Xiao, S., Wang, R. B., Wang, Z. T., Wang, W. W., et al. (2016). Pulsed lasers employing solution-processed plasmonic Cu3-xP colloidal nanocrystals. Adv. Mater. 28, 3535–3542. doi: 10.1002/adma.201504927
Luther, J. M., Jain, P. K., Ewers, T., and Alivisatos, A. P. (2011). Localized surface plasmon resonances arising from free carriers in doped quantum dots. Nat. Mater. 10, 361–366. doi: 10.1038/nmat3004
Ma, Z. J., Zhang, H., Hu, Z. L., Gan, J. L., Yang, C. S., Luo, Z. C., et al. (2018). Composite film with anisotropically enhanced optical nonlinearity for a pulse-width tunable fiber laser. J. Mater. Chem. C 6, 1126–1135. doi: 10.1039/C7TC03711B
Manthiram, K., and Alivisatos, A. P. (2012). Tunable localized surface plasmon resonances in tungsten oxide nanocrystals. J. Am. Chem. Soc. 134, 3995–3998. doi: 10.1021/ja211363w
Martinez, A., and Sun, Z. P. (2013). Nanotube and graphene saturable absorbers for fibre lasers. Nat. Photonics 7, 842–845. doi: 10.1038/nphoton.2013.304
Naik, G. V., Shalaev, V. M., and Boltasseva, A. (2013). Alternative plasmonic materials: beyond gold and silver. Adv. Mater. 25, 3264–3294. doi: 10.1002/adma.201205076
Niu, X. X., Hu, X. Y., Chu, S. S., and Gong, Q. H. (2018). Epsilon-Near-Zero photonics: a new platform for integrated devices. Adv. Opt. Mater. 6:1701292. doi: 10.1002/adom.201701292
Obermeier, J., Schumacher, T., and Lippitz, M. (2018). Nonlinear spectroscopy of plasmonic nanoparticles. Adv. Phys. 3:1454341. doi: 10.1080/23746149.2018.1454341
Rethfeld, B., Kaiser, A., Vicanek, M., and Simon, G. (2002). Ultrafast dynamics of nonequilibrium electrons in metals under femtosecond laser irradiation. Phys. Rev. B 65:214303. doi: 10.1103/PhysRevB.65.214303
Sobon, G. (2015). Mode-locking of fiber lasers using novel two-dimensional nanomaterials: graphene and topological insulators [Invited]. Photon. Res. 3, A56–A63. doi: 10.1364/PRJ.3.000A56
Stepanov, A. L. (2011). Nonlinear optical properties of implanted metal nanoparticles in various transparent matrixes: a review. Rev. Adv. Mater. Sci. 27, 115–145.
Sun, C. K., Vallee, F., Acioli, L., Ippen, E. P., and Fujimoto, J. G. (1993). Femtosecond Investigation of Electron Thermalization in Gold. Phys. Rev. B 48, 12365–12368. doi: 10.1103/PhysRevB.48.12365
Voisin, C., Del Fatti, N., Christofilos, D., and Vallee, F. (2001). Ultrafast electron dynamics and optical nonlinearities in metal nanoparticles. J. Phys. Chem. B 105, 2264–2280. doi: 10.1021/jp0038153
Wang, F., Rozhin, A. G., Scardaci, V., Sun, Z., Hennrich, F., White, I. H., et al. (2008). Wideband-tuneable, nanotube mode-locked, fibre laser. Nat. Nanotechnol. 3, 738–742. doi: 10.1038/nnano.2008.312
Wang, Q. Q., Han, J. B., Gong, H. M., Chen, D. J., Zhao, X. J., Feng, J. Y., et al. (2006). Linear and nonlinear optical properties of Ag nanowire polarizing glass. Adv. Funct. Mater. 16, 2405–2408. doi: 10.1002/adfm.200600096
Wang, S. X., Yu, H. H, Zhang, H. J., Wang, A. Z., Zhao, M. W., Chen, Y. X., et al. (2014). Broadband few-layer MoS2 saturable absorbers. Adv. Mater. 26, 3538–3544. doi: 10.1002/adma.201306322
Wang, W. Q., Yue, W. J., Liu, Z. Z., Shi, T. C., Du, J., Leng, Y. X., et al. (2018). Ultrafast nonlinear optical response in plasmonic 2D molybdenum oxide nanosheets for mode-locked pulse generation. Adv. Opt. Mater. 6:1700948. doi: 10.1002/adom.201700948
Wang, X. D., Luo, Z. C., Liu, H., Liu, M., Luo, A. P., and Xu, W. C. (2014). Microfiber-based gold nanorods as saturable absorber for femtosecond pulse generation in a fiber laser. App. Phys. Lett. 105:161107. doi: 10.1063/1.4899133
Wang, X. D., Luo, Z. C., Liu, M., Tang, R., Luo, A. P., and Xu, W. C. (2016). Wavelength-switchable femtosecond pulse fiber laser mode-locked by silica-encased gold nanorods. Laser Phys. Lett. 13:045101. doi: 10.1088/1612-2011/13/4/045101
Wu, D. D., Peng, J., Cai, Z. P., Weng, J., Luo, Z. Q., Chen, N., et al. (2015). Gold nanoparticles as a saturable absorber for visible 635 nm Q-switched pulse generation. Opt. Express 23, 24071–24076. doi: 10.1364/OE.23.024071
Yu, H. H., Zhang, H., Wang, Y. C., Zhao, C. J., Wang, B. L., Wen, S. C., et al. (2013). Topological insulator as an optical modulator for pulsed solid-state lasers. Laser Photonics Rev. 7, L77–L83. doi: 10.1002/lpor.201300084
Zhang, H., Hu, Z. L., Ma, Z. J., Gecevicius, M, Dong, G. P., Zhou, S. F., et al. (2016). Anisotropically enhanced nonlinear optical properties of ensembles of gold nanorods electrospun in polymer nanofiber film. ACS Appl. Mater. Interface 8, 2048–2053. doi: 10.1021/acsami.5b10411
Keywords: colloidal plasmonic nanoparticles, nonlinear optical properties, saturable absorption, optical switch, pulse laser
Citation: Liu X (2018) Colloidal Plasmonic Nanoparticles for Ultrafast Optical Switching and Laser Pulse Generation. Front. Mater. 5:59. doi: 10.3389/fmats.2018.00059
Received: 28 June 2018; Accepted: 04 September 2018;
Published: 25 September 2018.
Edited by:
Xin-Hao Li, Shanghai Jiao Tong University, ChinaReviewed by:
Haolan Xu, University of South Australia, AustraliaHaohai Yu, Shandong University, China
Copyright © 2018 Liu. This is an open-access article distributed under the terms of the Creative Commons Attribution License (CC BY). The use, distribution or reproduction in other forums is permitted, provided the original author(s) and the copyright owner(s) are credited and that the original publication in this journal is cited, in accordance with accepted academic practice. No use, distribution or reproduction is permitted which does not comply with these terms.
*Correspondence: Xiaofeng Liu, eGZsaXVAemp1LmVkdS5jbg==