- The Institute of Technological Sciences, Wuhan University, Wuhan, China
Thermoelectric (TE) materials have been of great importance for the capability of direct energy conversion between heat and electricity. In the past decades, people have developed various methods to enhance the performance of TE materials, which is characterized in terms of the dimensionless figure of merit of ZT. Combined with the advanced techniques of printing, the printable and flexible TE materials have been obtained, which can greatly broaden the application of the TE materials, thus improve the overall energy conversion efficiency. Most importantly, the flexible high performance TE materials-based device can reduce the consume of unrecoverable resources. In this review, we would like to discuss the strategies of enhancing the performance of TE materials. Then, the advanced techniques of printing of TE materials-based device will be involved. Finally, we would suggest some promising materials to enhance the thermoelectric performance and the potential applications in future.
Introduction
The world has been demanding more and more electricity by burning fossil fuels, which put both human beings and natural systems at stake. It is in urgent condition to find more efficient and environmentally friendly techniques to convert heat to electricity. Thermoelectric (TE) devices directly convert heat to electricity, thus have great potential to enhance the energy conversion efficiency (He and Tritt, 2017). The thermoelectric effect arises from the movement of charge carriers in metals and semiconductors. While a temperature gradient is applied to TE materials, the charge carriers diffuse from hot end to cold end, producing an electrostatic potential (Snyder and Toberer, 2008). When the electrostatic repulsion from the build-up of charge equals to the chemical potential for diffusion, steady current flows. The performances of TE materials are characterized in the form of dimensionless figure of merit ZT, ZT= , where α is the Seebeck coefficient, ρ is the electrical resistivity, κ is the thermal conductivity (Tiejun et al., 2017). The high zT value corresponds to high sensitivity of working variations (Goupil et al., 2013). After decades of tremendous efforts, researchers have found many TE materials categorized in different families, which is summarized in Table 1. TE devices are free of moving parts or noises, making them ready to integrate with other technologies. In addition, for the simple process of energy conversion, TE devices have been widely used in many fields, such as the keeping laser diodes at constant temperature. Compared to the efficiency of Carnot cycle, there is still long way to go to further improve the ZT value.
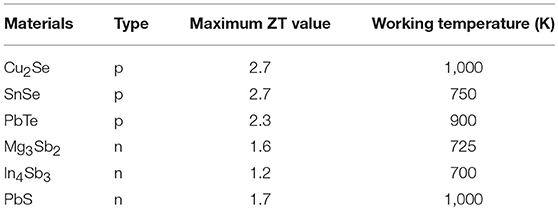
Table 1. Some typical TE materials with maximum ZT values and working temperatures (Tiejun et al., 2017).
The increased demanding of system-integration requires devices to be flexible (Rogers et al., 2010; Goupil et al., 2013; He and Tritt, 2017; Tiejun et al., 2017). These kinds of devices act as artificial skin, thus percept the signal from the inner skin sensitively, which can help people track the lesion of skin before the patients are aware of it. John A. Rogers et al. has reported that the compliant and stretchable TE materials in the form of coils that can be integrated into the compliant three-dimensional forms (Nan et al., 2018). Some other works have also demonstrated that by the technique of thermoelectric painting, people can achieve the shape-engineering of high-performance TE materials (Sun et al., 2014; Park et al., 2016).
Thermoelectric Materials
People have developed many strategies to fabricate different kinds of TE materials with the intention to enhance TE performance of materials (Tiejun et al., 2017). The evolution history of the TE materials has witnessed two major leaps. One of such advances is inspired by doping of semiconductors and other alloying methods, while the other one is related to the application of thermoelectric field (Goldsmid and Douglas, 1954; Joffe et al., 1956; Hicks and Dresselhaus, 1993). These historical advances have triggered great enhancement of TE performances. The ZT values of typical TE materials have surpassed the unity, higher than 2, even approaching 2.7 as shown in Table 1 which illustrates the temperature dependence of the ZT value (Tiejun et al., 2017). The basic foundations are from three combinatorial transport parameters (ρ, α, and κ) that relate to the intrinsic thermoelectric property of materials, the electrical resistivity and the thermal conductivity. From comparison, the middle temperature TE materials occupy more species than that of the low temperature TE materials (Zhou et al., 2008; Jong-Soo et al., 2009; Pei et al., 2011; Shi et al., 2011; Yanzhong et al., 2011; Biswas et al., 2012; Wang et al., 2012; Wei et al., 2012; Lipeng et al., 2015; Liu et al., 2015). One of such middle temperature TE materials is lead salt known as PbS, which has been fabricated on bird feathers (Xiaowei et al., 2013). The method of fabricating such advanced functional material is inspired by an ancient Egypt hair-dying method and can be completed in very short time, thus has very high fabrication efficiency (Walter et al., 2006). The TE materials combined with the biological materials can also work as the artificial materials to be applied in the field of medical engineering. The family of Bi2Te3 stands for the semiconductor TE materials decades ago and CoSb3 represents the historical advance of the TE materials development under the progress of the thermoelectric field. Till now, the thermoelectric field which was led by the development of quantum confinement is still the major strategy to obtain high performance TE materials (Joffe et al., 1956). The main theme is to inhibit the phonon transport but promote the electron transport. It is challenging to have the middle temperature TE materials work at room temperature but keep the same TE performance, thus people can apply such functional materials on more broad fields. The possible strategy is to combine and functionalize the TE materials with biological materials.
The foundation of the TE materials performance is the innovative transport mechanism that is enabled by the defects which cause the breaking of the translational symmetry of crystal lattice (He and Tritt, 2017). Both intrinsic and extrinsic defects in materials determine the carrier concentration, thereby the TE performance (He and Tritt, 2017). The electrical conductivity of a specific materials is linearly related to the carrier concentration and carrier mobility (Goupil et al., 2013). So, optimizing the carrier concentration is the most effective method to improve the TE performance. Extrinsic doping of some other kinds of elements that are different from the matrix will induce the intrinsic defects and then the intrinsic carrier concentration, leading to the electricity improvement of the TE materials. The different kinds of defects relating to the TE materials and performances are detailed in the reference (He and Tritt, 2017) for readers' further comprehension. Breakthrough in multiscale defects will benefit the overall improvement of the TE materials performance, which sheds light on the way to fabricate TE materials of even higher ZT values. Moreover, doping of various elements during selection from nature can be aided by the present hot research field of big data and accelerate the developing of new generation of TE materials.
The ZT value of TE materials is proportional to the Seebeck coefficient at given carrier concentration, which is effective in achieving high thermoelectric performance. The achievement of high Seebeck coefficient can be realized by the structural transition of the specific material, which results from the impurity of the material via the techniques of doping (Hicks and Dresselhaus, 1993). The working principle of TE materials is to increase the electric conductivity while suppress the thermal conductivity. Besides the strategies of enhancing the electricity of materials, it also requires to impede the thermal conductivity of materials, including the electric thermo conductivity, the lattice thermo conductivity and the bipolar thermal conductivity (Goupil et al., 2013; He and Tritt, 2017; Tiejun et al., 2017). Selecting the material with intrinsic low thermal conductivity will enable people more options of TE materials. However, reducing the thermal conductivity of the TE materials of high ZT value is complementary to the present TE materials. The carrier of the thermo conductivity is phonon. Scattering phonon is the most effective strategy to reduce the thermo conductivity, which can be achieved by the defects. As mentioned above, the defects relating to the carrier concentrations suppress the electricity of materials, but they can also, on the other hand, reduce the thermo conductivity. People have tried to manipulate the defects in materials to get the overall high TE performance from the increase of the electricity but the decrease of thermo conductivity. Among these different kinds of defects, grain boundaries of disordered atoms and twin boundaries of ordered atoms, especially the latter case, are the most promising methods people can work with to keep excellent electricity but suppress the thermo conductivity of TE materials (Liu et al., 2018b). We have already systematically studied the grain size effect to the TE performance of Sb2Te3 (Walter et al., 2006; Pei et al., 2011; Xiaowei et al., 2013). It shows that the grain size of the Sb2Te3 is significant to the decreasing of the thermal conductivity but the thermo conductivity keeps stable between room temperature and middle temperature (Pei et al., 2011). The small grain size possesses high ratio of grain boundaries, which benefit the carrier transport but block of the phonons, leading to the decrease in thermal conductivity but increase in electrical conductivity, thus the optimized figure of merit ZT. The comprehensive enhancement of thermo electric property is attributed to the defects (Walter et al., 2006). Furthermore, if the Sb2Te3 thin plate was sandwiched into package, the synchronous increase of electrical conductivity and decrease of thermal conductivity will be achieved by tailoring the electric flow along the more-electrically conductive parallel path and thermal flow blocked across the less-thermally conductive perpendicular direction (Xiaowei et al., 2013).
Flexible Materials
In recent years, development of flexible and stretchable integrated sensors and devices for the applications has been hot ranging from surgical and diagnostic implements to cameras of superior performances (Jiang et al., 2007; Sang-Il et al., 2008; Wenwen et al., 2014, 2015; Yan et al., 2016; Yang et al., 2017). The reliable diagnosis of patients requires sensors to output signal of high sensitivity but without invasiveness. The scope of the flexible and stretchable materials should be extended to large-area, more complex but more efficient devices to approach the human-like sensing capability (Sekitani et al., 2009; Lipomi et al., 2011). There are some main routes to obtain such advanced functional devices. One of the routes is about the structural layouts in the conventional materials. The rigid material of very weak flexibility, take the silicon wafer as a typical example, can be imbedded into the polymeric substrate of considerable flexibility, such as poly (dimethylsiloxane) (PDMS). The polymeric substrate undergoes dozens of times of strain, but the rigid functional material of only a few percent strain (Rogers et al., 2010). The highly elastic fluorinated copolymer rubber can be used as the substrate with other functional materials. There is some work about the single-walled carbon nanotubes that are dispersed on stretchable rubber, the composite of which is viscous. The single-walled carbon nanotubes-rubber composite can be finely patterned with the direct printing technologies (Sekitani et al., 2009). Such printable composite can be integrated with organic transistors and light-emitting diodes (LED) to work as the flexible LED displays. The composite of SWNT and rubber was smooth under scanning electron microscope (SEM) characterization. Even under high stretch, it keeps very high conductivity. An extraordinarily high conductivity of 102 S cm−1 was achieved at the high stretch of 29%. Under the condition of such profound ability of both flexibility and electricity, the processes of bending or crumpling cause small mechanical or electrical damage of the materials (Sekitani et al., 2009). More importantly, the thermoelectric materials can be fabricated on the glass fabric by the screening printing technique and other polymerization-based techniques (Sun et al., 2014; Liu et al., 2018a). With these universal methods, people can easily obtain the flexible functional materials, consisting of the TE materials and the bio-compatible substrate. We figure out the fabrication paradigm of the printable TE materials by the advanced printing techniques together with the using of PDMS as shown in Figure 1.
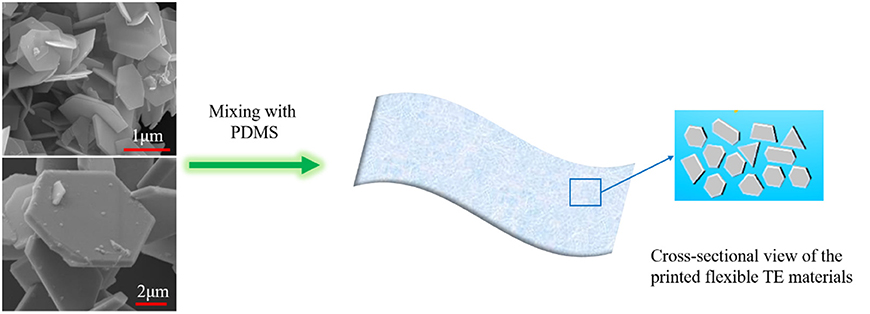
Figure 1. Schematic of the fabrication of printed flexible TE materials. SEM images were from our previous work (Fangfang et al., 2019).
Since the TE materials of such great superiority of direct energy conversion naturally occupy attention of researchers, the flexible and stretchable TE materials-based devices should be of great potential for future integrated applications. There is one exciting work about high temperature TE material which can work at the temperature of 3000 K (Tian et al., 2018). The reported material is about the high temperature reduced graphene oxide nanosheet. Such novel material can work at the temperature approaching 3300 K. Other researches about graphene show the excellent mechanical, optical, electrical, thermoelectrical properties. With these overall great properties of one specific material, it can be easily extended to the printable thermoelectric field. In recent years, some groups have developed some novel methods to print the flexible TE materials, which dramatically enhances the fabrication efficiency for wider applications of such materials (Fredrick et al., 2018; Hu et al., 2018; Liming et al., 2018). The reported technique (detailed in Ref. Yang et al., 2017) about the printed flexible TE materials is to use the TE materials-based inks, which is the C60/TE solution. In the hybrid solution, the soluble C60 serves as the flexible substrate during assembly, while the TE materials behave the same way as the pure TE materials. Most polymetric materials obsess the property of flexibility and the liquid phase of the corresponding material has very good solubility, which accomplished the shortage of the TE materials of solid states. Thus, this technique of the substrate/TE material hybrid is general for printing the flexible high-performance TE materials. The printed TE materials in the reference (Tian et al., 2018) work in the middle temperature without loss of the thermoelectricity.
Conclusion
The application-driven fundamental research has inspired people to develop multi-functional materials to be integrated into devices to obtain diverse information. TE materials integrated in devices directly convert energy from heat to electricity with high efficiency, while flexibility of such material-based devices makes it possible to work in the bio-related tissues/organs. The highest ZT value of about 2.7 achieved in Cu2Sn has attracted great interest theses years, but still far away from the power-generation-efficiency of Carnot cycle. Based on the theoretical strategies to improve the ZT value, we need to optimized the structure and distribution of defects in materials to obtain high ZT value. The small sized TE materials for printing and the combination of the materials to the polymeric substrate for flexible device integration also needs to be investigated for further stability. People need to find different approaches to optimize the materials and corresponding properties for the maximization of working efficiency. Furthermore, the printability of such TE materials would initiate the economic interest for wide application in the construction of national economics.
Author Contributions
XL have drafted the manuscript and ZW has checked it for final submission.
Funding
The authors acknowledge financial support from the Wuhan University Start-up funding under grant Nos. 413100010 and 413100015.
Conflict of Interest Statement
The authors declare that the research was conducted in the absence of any commercial or financial relationships that could be construed as a potential conflict of interest.
The handling editor declared a shared affiliation, though no other collaboration, with the authors at time of review.
References
Biswas, K., He, J., Blum, I. D., Wu, C. I., Hogan, T. P., Seidman, D. N., et al. (2012). High-performance bulk thermoelectrics with all-scale hierarchical architectures. Nature 489, 414–418. doi: 10.1038/nature11439
Fangfang, K., Bai, J., Bi, P., Liu, X., Wang, Z., and Xiong, R. (2019). Size effect enhanced thermoelectric properties of nanoscale Cu2-xSe. Ceramics Int. 45:8866. doi: 10.1016/j.ceramint.2019.01.215
Fredrick, K., Kwon, B., Eom, Y., Lee, J. E., Park, S., Jo, S., et al. (2018). 3D printing of shape-conformable thermoelectric materials using all-inorganic Bi2Te3-based inks. Nat. Energy 3:301. doi: 10.1038/s41560-017-0071-2
Goldsmid, H. J., and Douglas, R. W. (1954). The use of semiconductors in thermoelectric refrigeration. Br. J. Appl. Phys. 5:386.
Goupil, T. C., Seifert, W., Zabrocki, K., Muller, E., and Snyder, G. J. (2013). Hermodynamics of thermoelectric phenomenon and applications. Entropy 12, 1481–1517. doi: 10.3390/e13081481
He, J., and Tritt, T. M. (2017). Advances in thermoelectric materials research: Looking back and moving forward. Science 357:1369. doi: 10.1126/science.aak9997
Hicks, L. D., and Dresselhaus, M. S. (1993). Use of quantum-well superlattices to obtain a high figure of merit from nonconventional thermoelectric materials. MRS Proc. 326:413.
Hu, H., Zhu, X., Wang, C., Zhang, L., Li, X., Lee, S., et al. (2018). Stretchable ultrasonic transducer arrays for three-dimensional imaging on complex surfaces. Sci. Adv. 4:3979. doi: 10.1126/sciadv.aar3979
Jiang, H., Khang, D. Y., Song, J., Sun, Y., Huang, Y., and Rogers, J. A. (2007). Finite deformation mechanics in buckled thin films on compliant supports. Proc. Natl. Acad. Sci. U.S.A. 104, 15607–15612. doi: 10.1073/pnas.0702927104
Joffe, A. F., Airapetiants, S. V., Joffe, A. V., Kolomoetz, N. V., and Stilbans, L. S. (1956). Increasing the efficiency of semiconductor thermocouples. Doklady Akademii Nauk Sssr 106:981.
Jong-Soo, R., Lee, K. H., Lee, S. M., Cho, E., Kim, S. I., Lee, E., et al. (2009). Peierls distortion as a route to high thermoelectric performance in In4Se3-δ crystals. Nature 459, 965–968. doi: 10.1038/nature08088
Liming, W., Zhang, Z., Geng, L., Yuan, T., Liu, Y., Guo, J., et al. (2018). Solution-printable fullerene/TiS2 organic/ inorganic hybrids for high-performance flexible n-type thermoelectrics. Energy Environ. Sci. 11:1307. doi: 10.1039/C7EE03617E
Lipeng, H., Wu, H., Zhu, T., Fu, C., He, J., Ying, P., et al. (2015). Tuning multiscale microstructures to enhance thermoelectric performance of n-type bismuth-telluride-based solid solutions. Adv. Energy Mater. 5:1500411. doi: 10.1002/aenm.201500411
Lipomi, D. J., Vosgueritchian, M., Tee, B. C., Hellstrom, S. L., Lee, J. A., Fox, C. H., et al. (2011). Skin-like pressure and strain sensors based on transparent elastic films of carbon nanotubes. Nat. Mater. 6:788. doi: 10.1038/nnano.2011.184
Liu, W., Kim, H. S., Chen, S., Jie, Q., Lv, B., Yao, M., et al. (2015). n-type thermoelectric material Mg2Sn0.75Ge0.25 for high power generation. Proc. Natl. Acad. Sci. U.S.A. 112, 3269–3274. doi: 10.1073/pnas.1424388112
Liu, X., Gu, H., Wang, M., Du, X., Gao, B., Elbaz, A., et al. (2018a). 3D Printing of bioinspired liquid superrepellent structures. Adv. Mater. 30:1800103. doi: 10.1002/adma.201800103
Liu, X., Sun, L., Zhu, L., Liu, J., Lu, K., and Lu, J. (2018b). High-order hierarchical nanotwins with superior strength and ductility. Acta Mater. 149, 397–406. doi: 10.1016/j.actamat.2018.01.047
Nan, K., Kang, S. D., Li, K., Yu, K. J., Zhu, F., Wang, J., et al. (2018). Compliant and stretchable thermoelectric coils for energy harvesting in miniature flexible devices. Sci. Adv. 4:5849. doi: 10.1126/sciadv.aau5849
Park, S. H., Jo, S., Kwon, B., Kim, F., Ban, H. W., Lee, J. E., et al. (2016). High-performance shape-engineerable thermoelectric painting. Nat. Commun. 7:13403. doi: 10.1038/ncomms13403
Pei, Y., Shi, X., LaLonde, A., Wang, H., Chen, L., and Snyder, G. J. (2011). Convergence of electronic bands for high performance bulk thermoelectrics. Nature 473, 66–69. doi: 10.1038/nature09996
Rogers, J. A., Someya, T., and Huang, Y. (2010). Materials and mechanics for stretchable electronics. Science 327:1603. doi: 10.1126/science.1182383
Sang-Il, P., Ahn, J.-H., Feng, X., Wang, S., Huang, Y., and Rogers, J. A. (2008). Theoretical and experimental studies of bending of inorganic electronic materials on plastic substrates. Adv. Funct. Mater. 18, 2673–2684. doi: 10.1002/adfm.200800306
Sekitani, T., Nakajima, H., Maeda, H., Fukushima, T., Aida, T., Hata, K., et al. (2009). Stretchable active-matrix organi clight-emitting diode display using printable elastic conductors. Nat. Mater. 8:494. doi: 10.1038/nmat2459
Shi, X., Yang, J., Salvador, J. R., Chi, M., Cho, J. Y., Wang, H., et al. (2011). Multiple-filled skutterudites: high thermoelectric figure of merit through separately optimizing electrical and thermal transports. J. Am. Chem. Soc. 133, 7837–7846. doi: 10.1021/ja111199y
Snyder, G. J., and Toberer, E. S. (2008). Complex thermoelectric materials. Nat. Mater. 7:105. doi: 10.1038/nmat2090
Sun, J. K., We, J. H., and Cho, B. J. (2014). A wearable thermoelectric generator fabricated on a glass fabric. Energy Environ. Sci. 7:1959. doi: 10.1039/c4ee00242c
Tian, L., Pickel, A. D., Yao, Y., Chen, Y., Zeng, Y., Lacey, S. D., et al. (2018). Thermoelectric properties and performance of flexible reduced graphene oxide films up to 3,000 K. Nat. Energy 3:148. doi: 10.1038/s41560-018-0086-3
Tiejun, Z., Liu, Y., Fu, C., Heremans, J. P., Snyder, J. G., and Zhao, X. (2017). Compromise and synergy in high-efficiency thermoelectric materials. Adv. Mater. 29:1605884. doi: 10.1002/adma.201605884
Walter, P., Welcomme, E., Hallégot, P., Zaluzec, N. J., Deeb, C., Castaing, J., et al. (2006). Early use of PbS nanotechnology for an ancient hair dyeing formula. Nano Lett. 6:2215. doi: 10.1021/nl061493u
Wang, H., Pei, Y., LaLonde, A. D., and Snyder, G. J. (2012). Weak electron–phonon coupling contributing to high thermoelectric performance in n-type PbSe. Proc. Natl. Acad. Sci. U.S.A. 109, 9705–9709. doi: 10.1073/pnas.1111419109
Wei, L., Tan, X., Yin, K., Liu, H., Tang, X., Shi, J., et al. (2012). Convergence of conduction bands as a means of enhancing thermoelectric performance of n-type Mg2Si1–xSnx solid solutions. Phys. Rev. Lett. 108:166601. doi: 10.1103/PhysRevLett.108.166601
Wenwen, Z., Bi, P., Kang, H., Wei, W., Liu, F., Shi, J., et al. (2014). Low thermal conductivity and high thermoelectric figure of merit in p-type Sb2Te3/poly(3,4-ethylenedioxythiophene) thermoelectric composites. Appl. Phys. Lett. 105:023901. doi: 10.1063/1.4887504
Wenwen, Z., Yang, D., Wei, W., Liu, F., Tang, X., Shi, J., et al. (2015). Decoupling electrical and thermal properties in antimony telluride based artificial multilayer for high thermoelectric performance. Appl. Phys. Lett. 107:203901. doi: 10.1063/1.4936123
Xiaowei, L., Tan, Y., Zhang, F., Ouyang, P., Gu, J., and Zhang, D. (2013). An ancient method-inspired route for fast fabrication of ‘PbS bird feathers'. MRS Commun. 3, 61–65. doi: 10.1557/mrc.2013.2
Yan, X., Zheng, W., Liu, F., Yang, S., and Wang, Z. (2016). Thickness effects for thermoelectric property of antimony telluride nanoplatelets via solvothermal method. Sci. Rep. 6:37722. doi: 10.1038/srep37722
Yang, C., Souchay, D., Knei, M., Bogner, M., Wei, H. M., Lorenz, M., et al. (2017). Transparent flexible thermoelectric material based on non-toxic earth-abundant p-type copper iodide thin film. Nat. Commun. 8:16076. doi: 10.1038/ncomms16076
Yanzhong, P., Lensch-Falk, J., Toberer, E. S., Medlin, D. L., and Jeffrey Snyder, G. (2011). High thermoelectric performance in PbTe due to large nanoscale Ag2Te precipitates and La doping. Adv. Funct. Mater. 21, 241–249. doi: 10.1002/adfm.201000878
Keywords: printability, thermoelectric materials, flexible materials, defects, sensor
Citation: Liu X and Wang Z (2019) Printable Thermoelectric Materials and Applications. Front. Mater. 6:88. doi: 10.3389/fmats.2019.00088
Received: 30 August 2018; Accepted: 09 April 2019;
Published: 26 April 2019.
Edited by:
Wu Wei, Wuhan University, ChinaReviewed by:
Yang Yang, University of Southern California, United StatesXiang Qi, Xiangtan University, China
Copyright © 2019 Liu and Wang. This is an open-access article distributed under the terms of the Creative Commons Attribution License (CC BY). The use, distribution or reproduction in other forums is permitted, provided the original author(s) and the copyright owner(s) are credited and that the original publication in this journal is cited, in accordance with accepted academic practice. No use, distribution or reproduction is permitted which does not comply with these terms.
*Correspondence: Ziyu Wang, enl3YW5nQHdodS5lZHUuY24=