Fabrication of VO2-Dispersed Glass in B2O3-P2O5-V2O5 System and Its Thermal Property
- 1Department of Applied Physics, Graduate School of Engineering, Tohoku University, Sendai, Japan
- 2Institute of Multidisciplinary Research for Advanced Materials, Tohoku University, Sendai, Japan
Phase change material (PCM), which enables heat storage based on phase transition, plays an important role for efficient energy utilization. This paper briefly describes our recent study about all-solid PCM by combination of vanadium dioxide (VO2) with latent heat storage capacity and multicomponent glass, i.e., VO2-dispersed glass. A B2O3-P2O5-V2O5 glass system is used as a matrix for the VO2-dispersed glass. An attempt to describe its latent heat storage function is given. Endothermic behavior due to structural phase transition of the VO2 crystals was observed in the VO2-dispersed sample, and the transition enthalpy corresponding to latent heat storage capacity was estimated to be ΔH~9.5 J/g. The value of the studied sample was larger than the value of dispersed glass in the BaO-TeO2-V2O5 system that was previously reported. Potential for PCM was considered in the studied sample.
Introduction
Heat storage technology is essential for efficient energy utilization and sustainable development because the technology can appropriately balance the supply and demand amounts of thermal energy for different times and/or spaces (Nomura et al., 2015a). For heat storage, materials able to release the absorbed/conserved energy, i.e., heat storage materials, play a crucial role. Particularly, the latent heat storage is based on the capture or release of energy when a material undergoes a phase change between solid and liquid state, and heat storage materials based on the phase transition are referred to as phase change materials (PCMs) (Hasnain, 1998; Farid et al., 2004; Jacob and Bruno, 2015). For example, ice, paraffin, fatty acids, and inorganic hydrates are frequently utilized as PCMs. However, since the heat storage mechanism of these PCMs involves a solid–liquid phase transition, it is imperative to ensure that the PCM inside the container is maintained in the liquid state. Moreover, damage to the container because of the large volume change occurring during the phase transition and leaking out of the liquid are inevitable. In order to prevent these issues coming from the presence of the liquid state, it is of particular interest to develop new PCMs (Nomura et al., 2015b; Tokoro et al., 2015).
A strongly correlated electron system, vanadium dioxide (VO2), has gained much interest as a PCM. A reversible solid–solid phase transition occurs at 68°C, accompanying an exothermic or endothermic reaction, and the latent heat of VO2 is comparable to that of the typical PCMs (Niitaka et al., 2010; Wu et al., 2011). Although VO2 is commercially available as a powder, if we use the VO2 powder as PCM, it is mandatory to use a container matrix. Thus, VO2 exhibits some disadvantages that impede large-scale PCM applications.
Glass exhibits immense advantages from the viewpoint of materials science, e.g., large-scale or mass production, flexibility, and formability (Sakamoto and Yamamoto, 2010). Recently, the authors' group has proposed a new PCM, i.e., VO2-dispersed glass, where the VO2 powders embedded in the glass matrix serve as a durable container-free PCM (Muramoto et al., 2018). 30BaO-10TeO2-60V2O5 (in mol%) glass was selected as the matrix on the basis of thermal and water-immersion tests. It was suggested that the Te–O polyhedral units contributed to a three-dimensional network structure of the glass (Ahamad et al., 2012), resulting in its high water resistivity. On the other hand, the deposit amount of Te element is relatively small, being comparable to that of noble metals, and additionally Te, and its related compounds are toxic. Another candidate in the vanadate system, B2O3-P2O5-V2O5 glass, was also considered, even though it possessed low water resistivity. Nevertheless, B2O3-P2O5-V2O5 glass consists of much more common and less-toxic substances, i.e., B2O3 and P2O5, and these oxides possess a considerably low melting temperature compared to that of a typical glass-former, SiO2. These lead us to consider that VO2 powder can be incorporated into a B2O3-P2O5-V2O5 glass without any degradation. Therefore, in this study, we have fabricated VO2-dispersed glasses by an incorporation technique and then examined the endothermic behavior based on the phase transition of VO2 crystals.
Experiment
In this study, the matrix glass with a composition of 15B2O3-10P2O5-75V2O5 (in mol%) was prepared by a conventional melt-quenching technique using an alumina crucible with a lid. Commercial reagent-grade powders of B2O3, (NH4)2HPO4, and V2O5 were used as raw materials. The mixture of the raw materials was melted at 800°C for 60 min under air. The melt was poured onto a steel plate heated at ~200°C, followed by pressing using another steel plate to obtain the as-quenched sample. The sample was confirmed to be in amorphous state as evidenced by X-ray diffraction (XRD) analysis (Cu-Kα radiation). Subsequently, to fabricate the VO2-dispersed glass, the incorporation method reported by Komatsu et al. (1995) was employed in the study: A powdered matrix glass and VO2 powder (purity: 99.9%; Kojundo Chemical Laboratory Co., Ltd.) were mixed using an alumina mortar. Second, the mixture was added into an alumina crucible and melted at 1,200°C for 15 min under air. The quenching process was similar to that utilized during the preparation of the matrix glass, finally furnishing VO2-dispersed glass.
The state of crystal phase in the dispersed sample was confirmed by XRD analysis and scanning electron microscope (SEM). Prior to XRD measurement, the dispersed samples were powdered by the use of an alumina mortar. The thermal properties of the matrix glass and VO2-dispersed samples and glass-transition (Tg), crystallization-onset (Tx), and crystallization-peak temperatures (Tp), were examined by differential thermal analysis (DTA; heating rate of 10 K/min, Rigaku Corporation, Thermoplus TG8120). The transition enthalpy (ΔH), which corresponds to the amount of the stored latent heat, of the VO2-dispersed sample was evaluated by differential scanning calorimetry (DSC; heating rate of 1 K/min, Seiko Instruments Inc., DSC6220). The specific heat, Cp [], was measured as a function of temperature (T), and ΔH was estimated on the basis of the thermodynamic relation, i.e., H = ∫ CpdT. The sample state used in the measurement was the bulk form with a weight of ca. 10 mg.
Results
Characterizations of the VO2-Dispersed Glass
Prior to fabrication of the VO2-dispersed sample, the 15B2O3-10P2O5-75V2O5 glass (matrix) was examined. The XRD measurement of the as-quenched sample indicated a halo pattern without any sharp diffraction peaks, which is a characteristic of the amorphous phase (cf. Figure 1). We have already obtained the DTA curve of 15B2O3-10P2O5-75V2O5 glass (Muramoto et al., 2018), and the parameters could be estimated on the basis of the previous DTA results as Tg = 225°C, Tx = 266°C, and Tp = 279°C. The values of Tg and Tp were almost identical to the values by Choi et al. (Choi and Ryu, 2015).
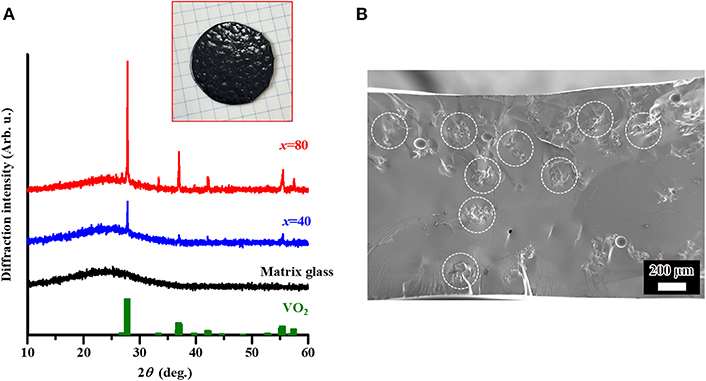
Figure 1. (A) Powder X-ray diffraction (XRD) results of the matrix glass with composition of 15B2O3-10P2O5-75V2O5, and the glasses dispersed with VO2 powder on the basis of the incorporation method. The values of x represent the additive amount of dispersed VO2 (in mol%). In addition, the ICDD patterns of VO2 (43–1051) are included. The inset corresponds to a photo of the dispersed sample with x = 80. (B) Scanning electron microscope (SEM) image of the cross-section of the dispersed sample with x = 40. The encircled region corresponds to the incorporated VO2 phase.
The VO2-dispered glasses with different additive amounts (x; mol%) of VO2 phase, i.e., 15B2O3-10P2O5-75V2O5:xVO2 composites with x = 40 and 80, were studied by XRD analysis. As a result, the crystalline diffraction peaks corresponding to VO2 phase could be observed in combination with the amorphous halo patterns due to matrix glass (Figure 1A), and the intensity of diffraction peaks increased with x. In addition, SEM observation was conducted in the sample with x = 40 as a representative in order to check the dispersion state in the matrix glass. As a result, we can see the inclusions with a size of ca. 100 μm, i.e., the presence of aggregation of VO2 particles (Figure 1B). The XRD and SEM results suggest that the VO2 phase is stably incorporated in the matrix glass. A possible explanation of the stable incorporation could be given by an abundance of vanadium around the incorporated VO2 phase, i.e., saturation of vanadium in the matrix glass, resulting in the suppression of vanadium migration/diffusion from the VO2 (Muramoto et al., 2018). Furthermore, the melting point of VO2 (~1,970°C) is much higher than the processing temperature for incorporation (1,200°C). This probably contributes to the stable incorporation as well.
As the result of DTA, the dispersed samples exhibited similar DTA curves to the matrix glass, i.e., endothermic region (224–229°C) and sharp exothermic peaks (280–290°C), which correspond to the Tg and Tp, respectively (Figure 2). We have found that the matrix glass crystalizes the V2O5 phase by means of thermal treatment at the Tp (Muramoto et al., 2018). In this study, crystallization of the V2O5 phase was also confirmed in the dispersed glasses heat-treated at the Tp for 1 h by means of the XRD measurement. Furthermore, we should mention that clear endothermic peaks are observed in the temperature range of ~60–80°C (onset temperature: 63–66°C), which is much lower than the Tg. Since their peaks tend to be large as the increase in x, it is reasonable to consider that the endothermic peaks originate from the phase transition of the dispersed VO2 phase because of the Tc~68°C. Thus, we have concluded that VO2-dispersed glass was successfully fabricated in the B2O3-P2O5-V2O5 system. This also leads us to expect that heat storage based on the solid-state phase transition of VO2 is possible in the dispersed glass sample. Meanwhile, the endothermic peak of the sample with x = 40 was relatively smaller than the peak expected from the sample with x = 80, in which the amount of the VO2 phase is double. As a possible explanation, the smallness of the endothermic peak is supposed to be due to low dispersion of incorporated VO2 phase in the matrix glass.
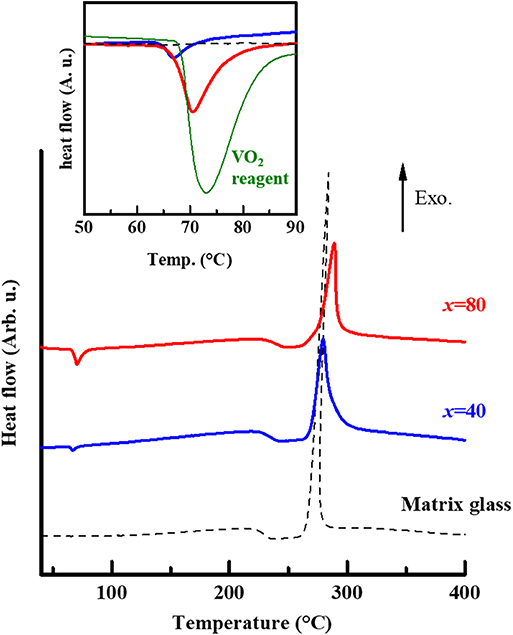
Figure 2. Differential thermal analysis (DTA) results of the dispersed samples, 15B2O3-10P2O5-75V2O5:x (x = 40 and 80; the studied samples are bulk form), together with the result of matrix glass referred from the paper (Muramoto et al., 2018). The inset shows the DTA curves in a low-temperature region, in which an endothermic reaction was observed in the heating process. The endothermic peaks with onset temperature are around the vicinity of Tc~68°C, indicating the structural phase transition of the dispersed VO2 phase. The DTA result in the VO2 reagent used in this study is also included (green curve; peak intensity was reduced to fit the graph).
Thermal Properties
We have measured the specific heat in the VO2-dispersed glass with x = 80 as a representative sample by means of DSC measurement in order to examine the latent heat storage function. A steep change in the specific heat (peak of the Cp-T curve), related to the phase transition of the dispersed VO2 powder, was observed around the phase-transition temperature of VO2 as well as the DTA result, and subsequently, the transition enthalpy (ΔH; the latent heat storage capacity) was estimated to be ΔH~9.5 J/g (Figure 3), corresponding to ca. 21% of that of the VO2 reagent, i.e., ~45 J/g (Muramoto et al., 2018). Thus, the latent heat storage function is also demonstrated in the dispersed sample in this study. Furthermore, the ΔH value obtained in this study is larger than the value of the VO2-dispersed sample with same amount of VO2, i.e., ΔH = 8.9 J/g for the 30BaO-10TeO2-60V2O5:80VO2 (Muramoto et al., 2018).
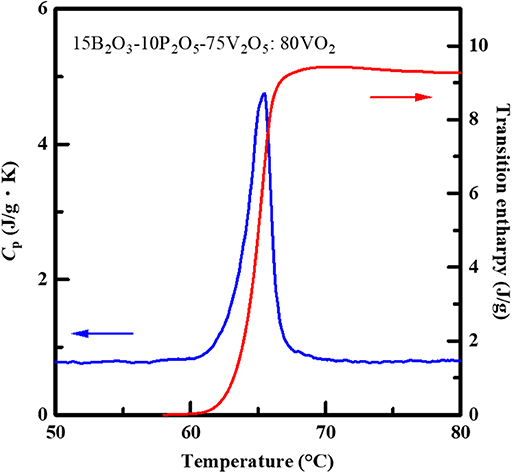
Figure 3. Heat capacity and transition enthalpy for the VO2-dispersed glass in the B2O3-P2O5-V2O5 system with x = 80. The transition enthalpy, ΔH, was obtained by thermodynamic treatment based on H = ∫ CpdT because the enthalpy corresponded to the latent heat storage capacity.
Discussion
Vanadate-system glasses are recognized to be synthesized at low melting-process temperature compared to the usual silicate glass. This is quite important for fabrication of the VO2-dispersed glass in order to prevent the degradation/decomposition of dispersed VO2 phase due to its transforming into other oxide phases, e.g., Magneli phase (Baldassarre et al., 2007), and to energy saving during the fabrication process. The thermal assessment in this study revealed that, apart from the problem of low water resistivity, the studied B2O3-P2O5-V2O5 system is able to involve the VO2 powder and to allow its phase transition, which provides a latent heat storage function. In addition, the Tg of the studied glass matrix is lower than that of matrix previously studied; Tg = 276°C for 30BaO-10TeO2-60V2O5 glass (Muramoto et al., 2018), suggesting the re-formability of the dispersed glass after its fabrication.
Barde et al. described a weakness against water in B2O3-P2O5-V2O5 system glass, which is evidenced by DTA (Barde et al., 2015). It is supposed that, under low moisture conditions, the VO2-dispersed glass in this study works as an all-solid PCM. Otherwise, the addition of other glass constituents, which contributes to the improvement of water resistivity, also seems to be effective for the PCM application because Kubiki et al. reported that the addition of WO3 provides the strengthening of the glass network in vanadate-system glass, resulting in its high chemical durability (Kubiki et al., 2013). In addition, another point that should be improved is the dispersibility of VO2 in a glass matrix. The SEM result (Figure 1B) suggests that the VO2 particles are inhomogeneously dispersed in the matrix glass because the size of inclusion is ca. 100 μm despite the size of the VO2 particle being estimated to be ~20 μm (Muramoto et al., 2018), i.e., aggregation of VO2 particles. Irradiation of the ultrasonic wave during the incorporation process may be effective to obtain a well-dispersed sample.
Summary
We already have proposed a new all-solid PCM, i.e., VO2-dispered glass, and demonstrated the latent heat storage in the BaO–TeO2-V2O5 system. In this study, to avoid the use of Te element, which is a rare metal and is toxic, another candidate vanadate system was considered, i.e., the B2O3-P2O5-V2O5 system, as a matrix glass. We have been able to fabricate the VO2-dispersed glasses in this system and demonstrated the heat storage function due to the structural phase transition of VO2. Furthermore, the latent heat storage capacity observed in the studied VO2-dispersed sample is larger than the capacity of the sample in the BaO-TeO2-V2O5 system, suggesting the potential of all-solid PCM application.
Data Availability Statement
The datasets generated for this study are available on request to the corresponding author.
Author Contributions
KM fabricated and characterized the materials. YT, NT, and TF designed the study. YY and SS contributed to the thermal analysis. and YT and TF wrote the paper.
Conflict of Interest
The authors declare that the research was conducted in the absence of any commercial or financial relationships that could be construed as a potential conflict of interest.
Acknowledgments
This work was supported by the Ministry of Education, Culture, Sports, Science and Technology of the Japanese government. The authors would like to thank Dr. Takamichi Miyazaki of the Department of Instrumental Analysis, Tohoku University, for significant contribution to this study.
References
Ahamad, M. N., Vaish, R., and Varma, K. B. R. (2012). Calorimetric studies on 2TeO2-V2O5 glasses. J. Therm. Anal. Calorim. 105, 239–243. doi: 10.1007/s10973-011-1305-3
Baldassarre, L., Perucchi, A., Arcangeletti, E., Nicoletti, D., Castro, D. D., Postorino, P., et al. (2007). Electrodynamics near the metal-to-insulator transition in V3O5. Phys. Rev. B 75:245108. doi: 10.1103/PhysRevB.75.245108
Barde, R. V., Nemade, K. R., and Waghuley, S. A. (2015). AC conductivity and dielectric relaxation in V2O5-P2O5-B2O3 glasses. J. Asian Ceram. Soc. 3, 116–122. doi: 10.1016/j.jascer.2014.11.006
Choi, S. Y., and Ryu, B. K. (2015). Nanocrystallization of vanadium borophosphate glass for improving the electrical and catalytic properties. J. Nanomater. 76:201597. doi: 10.1155/2015/201597
Farid, M. M., Khudhair, A. M., Razack, S. A. K., and Al-Hallaj, S. (2004). A review on phase change energy storage: materials and applications. Energy Convers. Manage. 45, 1597–1615. doi: 10.1016/j.enconman.2003.09.015
Hasnain, S. M. (1998). Review on sustainable thermal energy storage technologies, part I: heat storage materials and techniques. Energy Convers. Manage. 11, 1127–1138. doi: 10.1016/S0196-8904(98)00025-9
Jacob, R., and Bruno, F. (2015). Review on shell materials used in the encapsulation of phase change materials for high temperature thermal energy storage. Renew. Sust. Energy Rev. 48, 79–87. doi: 10.1016/j.rser.2015.03.038
Komatsu, T., Shioya, K., and Matusita, K. (1995). Fabrication of transparent tellurite glasses containing potassium niobate crystals by an incorporation method. J. Am. Ceram. Soc. 76, 2923–2926. doi: 10.1111/j.1151-2916.1993.tb04040.x
Kubiki, S., Matsuda, K., Akiyama, K., Homonnay, Z., Sinkó, K., Kuzmann, E., et al. (2013). Enhancement of electrical conductivity and chemical durability of 20R2O·10Fe2O3·xWO3·(70–x)V2O5 glass (R=Na, K) caused by structural relaxation. J. Non-Cryst. Solids 378, 227–233. doi: 10.1016/j.jnoncrysol.2013.07.012
Muramoto, K., Takahashi, Y., Terakado, N., Yamazaki, Y., Suzuki, S., and Fujiwara, T. (2018). VO2-dispersed glass: a new class of phase change material. Sci. Rep. 8:2275. doi: 10.1038/s41598-018-20519-6
Nomura, T., Tsubota, M., Okinaka, N., and Akiyama, T. (2015a). Improvement on heat release performance of direct-contact heat exchanger using phase change material for recovery of low temperature exhaust heat. ISIJ Int. 55, 441–447. doi: 10.2355/isijinternational.55.441
Nomura, T., Zhu, C., Sheng, N., Saito, G., and Akiyama, T. (2015b). Microencapsulation of metal-based phase change material for high-temperature thermal energy storage. Sci. Rep. 5:9117. doi: 10.1038/srep09117
Sakamoto, A., and Yamamoto, S. (2010). Glass-ceramics: engineering principles and applications. Int. J. Appl. Glass Sci. 1, 237–247. doi: 10.1111/j.2041-1294.2010.00027.x
Tokoro, H., Yoshikiyo, M., Imoto, K., Namai, A., Nasu, T., Nakagawa, K., et al. (2015). External stimulation-controllable heat-storage ceramics. Nat. Commun. 6:7037. doi: 10.1038/ncomms8037
Keywords: glass, vanadium dioxide, latent heat storage, vanadate, thermal property
Citation: Muramoto K, Takahashi Y, Terakado N, Yamazaki Y, Suzuki S and Fujiwara T (2020) Fabrication of VO2-Dispersed Glass in B2O3-P2O5-V2O5 System and Its Thermal Property. Front. Mater. 7:5. doi: 10.3389/fmats.2020.00005
Received: 15 October 2019; Accepted: 07 January 2020;
Published: 18 February 2020.
Edited by:
Ashutosh Goel, Rutgers, The State University of New Jersey, United StatesReviewed by:
Guglielmo Macrelli, Independent Researcher, Este, ItalyNadja Lönnroth, Huawei Technologies Oy (Finland) Co. Ltd, Finland
Copyright © 2020 Muramoto, Takahashi, Terakado, Yamazaki, Suzuki and Fujiwara. This is an open-access article distributed under the terms of the Creative Commons Attribution License (CC BY). The use, distribution or reproduction in other forums is permitted, provided the original author(s) and the copyright owner(s) are credited and that the original publication in this journal is cited, in accordance with accepted academic practice. No use, distribution or reproduction is permitted which does not comply with these terms.
*Correspondence: Yoshihiro Takahashi, takahashi@laser.apph.tohoku.ac.jp; Takumi Fujiwara, fujiwara@laser.apph.tohoku.ac.jp