- CNRS, Univ. Bx, Bx INP, ICMCB, UMR 5026, Pessac, France
Electrochromism is known as a modulation of the optical properties under an applied voltage. Used in various applications, aside to the commercialized smart windows based on transmissive electrochromic devices (ECDs), the opaque systems have received significant interest for displays purposes. Herein, in a novel approach to adjust color, electrochromic oxides with high EC performance were chosen as additives to commercialized conductive and electrochromic polymer ink. More precisely opaque films were deposited (using Bar Coater) from mixtures of V2O5, synthesized by polyol process, with poly(3,4-ethylenedioxythiophene, polystyrene sulfonate) PEDOT:PSS commercial ink in different weight percentages (wt%) ranging from 0 to 100 (V2O5 wt%). Films thicknesses varied from hundred of nms to few μms. As-deposited films colors range from blue for PEDOT:PSS to orange for V2O5. PEDOT:PSS-V2O5 films exhibit significant electrochromic properties associated with a good electrochemical stability. In the series, the hybrid film with 90 wt%–10 wt% PEDOT:PSS-V2O5 shows a reversible behavior with significant reflectance modulation (ΔR≈20.5% at 550 nm) in lithium based electrolyte and a good contrast (ΔE∗ > 30). The maximum contrast was reached for 3.7 μm thick layer. Our results indicate that the hybrid film, combining the benefit of mixing inorganic and organic materials, are promising materials for enhancing electrochromic devices color and hybrid electrochromic materials processability on large scale.
Introduction
Electrochromic materials that undergo reversible color change under an applied voltage (Lang et al., 2019) have received great interest due to their potential applications, such as smart windows (Runnerstrom et al., 2014; Nunes et al., 2019), information storage and displays (Mjejri et al., 2018b; Qingyi et al., 2019). Electrochromism occurs in a number of organic (Hu et al., 2018; Han et al., 2019), inorganic (Danine et al., 2014; Mjejri et al., 2016), and hybrid materials (Rodrigues et al., 2009) both in the visible and IR regions. Smart windows mature technology is typically based on combination of WO3 and IrOx or NiO (Granqvist et al., 2019). Optimizing both materials and devices architectures is interesting to develop the technology and target more application (Jung et al., 2004; Varghese et al., 2018; Danine et al., 2019). In particular, focusing on display applications, our interest in reflective devices increases with the need of enhancing the range of colors developed in ECDs. For inorganic materials, multi-electrochromism (e.g., materials displaying different colors depending on the voltage applied) is observed when vanadium oxide (V2O5) is deposited as a micrometric thick film. This material switch colors from blue, to green and orange depending on the voltage applied. Vanadium oxides offer several advantages: (i) they show both anodic and cathodic colorations (ii) their abundance and layered structure facilitate the exchange of a large amount of Li cations, and (iii) the existence of several oxidation states leading to the possibility of obtaining multicolor displays (Chu et al., 2016; Mjejri et al., 2019). The modulation of color may also be achieved by introducing new concepts of devices changing color independently on each side. In 2017, our group (Mjejri et al., 2017) reported for the first time a double-sided electrochromic device based on Metal-Organic Frameworks (MOFs) switching from light to dark blue on one side and from yellow to brownish on the other side. Integrating organic and inorganic materials into a hybrid material provides an opportunity to combine their advantages and explore new design. While transition metal oxides (TMOs) present generally a good redox activity they suffer, among other things from a too low electrical conductivity and a poor switching frequency. On the contrary, polymer-based electrochromic films are characterized by rapid kinetics, multi-color electrochromism, easy-processability using conventional printing technique (doctor blade, screen-printing, inkjet…), sufficient electrically conductivity while they typically suffer from shorter lifetime range and are highly sensitive to the working environment (Byker, 2001). To counter balance the pros and cons of both polymer and inorganic materials, hybrid systems are investigated. Recently, several groups have synthesized hybrid films by combining oxide and conductive polymer showing a better charge injection for a higher contrast for electrochromic displays (Lee et al., 2011) and better efficiency for solar cells (Lee et al., 2014). Following this path, we recently reported that the addition of low content of Fe2O3 in a PEDOT:PSS ink allowed to achieve a color modulation from bluish to reddish, while in first approximation Fe2O3 alone appears to be electrochromically inactive (Levasseur et al., 2019). Pursuing this hybrid approach herein, the electrochromic properties of bar coated opaque films, from mixture of electrochromically active V2O5 to commercial PEDOT:PSS ink, are investigated. V2O5 was preferentially used as it can be bring a larger color range to the PEDOT:PSS film. Indeed, other cathodic oxides such a Nb2O5 (Mjejri et al., 2018b) or WO3 (Danine et al., 2014) will only intensify the blue color of the PEDOT:PSS reduced state.
The preparation of oxide inks remains a big challenge for an easy film processability. A poor control of thickness and morphology is generally achieved with traditional coating techniques. Mixing a commercial PEDOT:PSS ink with oxides allows to promote higher quality film. Indeed, with the mixture of PEDOT:PSS-V2O5 presented in this paper, industrial technique such as screen-printing can be used for the layer deposition at room temperature and baked at the temperature applied for PEDOT:PSS (e.g., 120°C for 5 min). Another advantage consists in reducing the amount of V2O5 used to obtain a film as the oxide will only be added in small quantity in the PEDOT:PSS ink which can be beneficial for cost reason as this oxide requires expensive vanadium precursors. Vanadium oxide is generally deposited using vacuum techniques for good film quality, which are not suitable for large-scale display production. Other deposition techniques have been developed lately such as inkjet printing (Costa et al., 2012) or atomic layer deposition (Blanquart et al., 2013) and can be interesting for further study on V2O5 electrochromic properties. With our approach, a conductive layer is obtained thanks to the presence of PEDOT:PSS conductive polymer which can possibly reduce the number of layers needed for building the electrochromic display (Tashtoush and Kasasbeh, 2013). In summary, the goal of this study was to investigate a win-win strategy based on the polymer good processability and conductivity and the large color palette brought by the oxide.
Materials and Methods
Hybrid Inks Formulation
The hybrid electrochromic inks were formulated from the mixture of the commercial PEDOT:PSS ink Agfa Orgacon EL-P5015 and V2O5 powders synthesized by polyol process (Mjejri et al., 2018a). The commercial PEDOT:PSS ink characterized by high-viscosity commercial paste (>100,000 mPa⋅s) developed for screen printing was first homogenized with a three-roll mill and then diluted with ethanol to lower the viscosity. PEDOT:PSS was chosen thanks to its printing properties, its conductivity and it shows a very different reversible change of color from oxidized to reduced states making it suitable for electrochromism display fabrication (Andersson, 2006). The V2O5 weight percentages were calculated from the PEDOT:PSS paste mass. Seven premixes were prepared: weight ratio of (PEDOT:PSS paste)/(V2O5) = (100-x)/x, with x = 0.01%, 0.1%, 1%, 5%, 8%, 10%, 15%. These preparations were then diluted with ethanol with a mixing ratio of 40 wt% of PEDOT:PSS-V2O5 paste and 60 wt% of ethanol. The various hybrid inks with different weight ratio between PEDOT:PSS, V2O5 and ethanol were stirred for 15 min at room temperature, then dispersed using an ultrasonic bath for 30 min at room temperature and stirred again for 24 h.
To simplify the readability of the study, the ink formulation will be mentioned as the weight percentage of the vanadium oxide in the PEDOT:PSS as followed: 100 wt% V2O5 as 100%, 85 wt%–15 wt% PEDOT:PSS-V2O5 as 15%, 90 wt%–10 wt% PEDOT:PSS-V2O5 as 10%, 95 wt%–5 wt% PEDOT:PSS-V2O5 as 5%, 99 wt%–1 wt% PEDOT:PSS-V2O5 as 1% and PEDOT:PSS as 0%.
On the figures, 100% have been drawn in ochre, 15% in green, 10% in orange, 5% in purple, 1% in red, and 0% in blue. All percentages correspond to the wt%.
Hybrid Film Deposition and Characterization
The films were deposited with a bar coater (K control from RK Print Coat Instruments, Erichsen) with jointed whorl bars, noted from 1 to 8 with the following whorl diameters (mm): 0.08, 0.15, 0.31, 0.51, 0.64, 0.76, 1, 1.27. The coatings were done on ITO-coated (In2O3:Sn) glass substrates (commercialized by SOLEMS with a resistance of 30 Ω/sq) and then dried at 120°C/5 min on a hot plate. Depositions were made on 5∗2.5 cm2 ITO-coated glass substrates. The thickness of the PEDOT:PSS-V2O5 films were measured using a Veeco Dektak 6M Stylus Profilometer (Veeco Instruments Inc., New York, NY, United States). For the identification of the crystal phases of the hybrid films, X-ray diffraction (XRD, Philips PW 1820, PANalyticalX’Pert instrument, 2θ range from 10° to 50° and Cu Kα1 λ = 0.154056 nm) was used. The layer morphology was investigated with a JEOL JSM-840 (operating at 15 kV) scanning electron microscope (JEOL JSM-840, JEOL SAV-Europe, Croissy sur Seine, France).
Electrochromic Measurements
Electrochemical measurements of hybrid films PEDOT:PSS-V2O5 deposited on ITO/glass were carried out in a three electrodes cell configuration using a BioLogic SP50 potentiostat/galvanostat apparatus. The counter electrode and reference electrode consisted of a platinum foil and saturated calomel electrode, SCE (ESCE = 0.234 V/ENH), respectively. The operating voltage was controlled between −1.0 V and +1.5 V at a scan rate of 20 mV s–1, in lithium-based electrolyte, namely, lithium bis-trifluoromethanesulfonimide (LiTFSI, Solvionic, purity N 99.99%) in 1-ethyl-3 methylimidazolium bis(trifluoromethanesulfonyl)-imide (EMIM-TFSI, purity N 99.99%) with a corresponding molar ratio of 1:9 (LiTFSI:EMIM-TFSI). All the electrochemical measurements were performed at room temperature. The ex-situ optical reflectance of PEDOT:PSS-V2O5 hybrid films were measured using a Varian Cary 5000 UV-vis-NIR spectrophotometer between 300 and 800 nm. Colorimetry analysis was carried out using a Konica Minolta CM-700D spectrophotometer with SCE 10°/D65 configuration (Konica Minolta Sensing Europe B.V), allowing the direct determination of colorimetric parameters of the CIE (L∗a∗b∗) color space.
Results and Discussion
Structural and Morphological Properties of PEDOT:PSS-V2O5 Hybrid Films
To optimize the electrochromic performance of the hybrid PEDOT:PSS-V2O5 films, the weight ratio between PEDOT:PSS and V2O5 was investigated. Figure 1 shows the photographs of the various films deposited by bar coater using the same bar (n = 3) in association with the L∗, a∗, b∗ chromaticity parameters, defined by the CIE LAB. A significant color gradient in relationship with the decrease of the V2O5 weight percentages is visible.
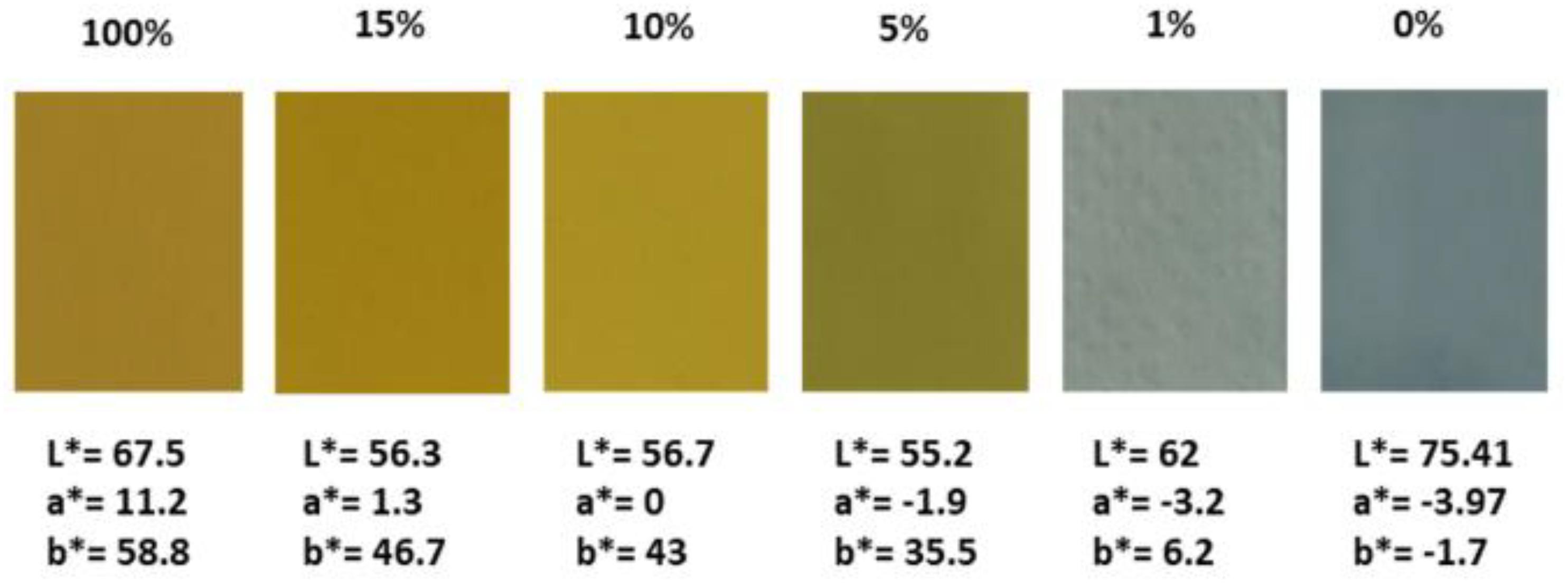
Figure 1. As-deposited hybrid films wt% PEDOT:PSS-V2O5 using the bar n = 3 with the corresponding L*, a*, b* chromaticity parameters.
From 100 wt% V2O5 yellow-orange film, the hybrid mixture remains close to the appearance of pure V2O5 till 10 wt%. The color turns greenish for 5 wt% V2O5, and for the 1 wt% V2O5, the hybrid film adopts a light blue-gray aspect while the PEDOT:PSS films appear blue.
As displayed in Figure 2, using any bars (from n = 1 to 8), the V2O5 wt% content has a strong influence on the film thickness. Overall, the thickness of hybrid films increases with increasing V2O5 wt%.
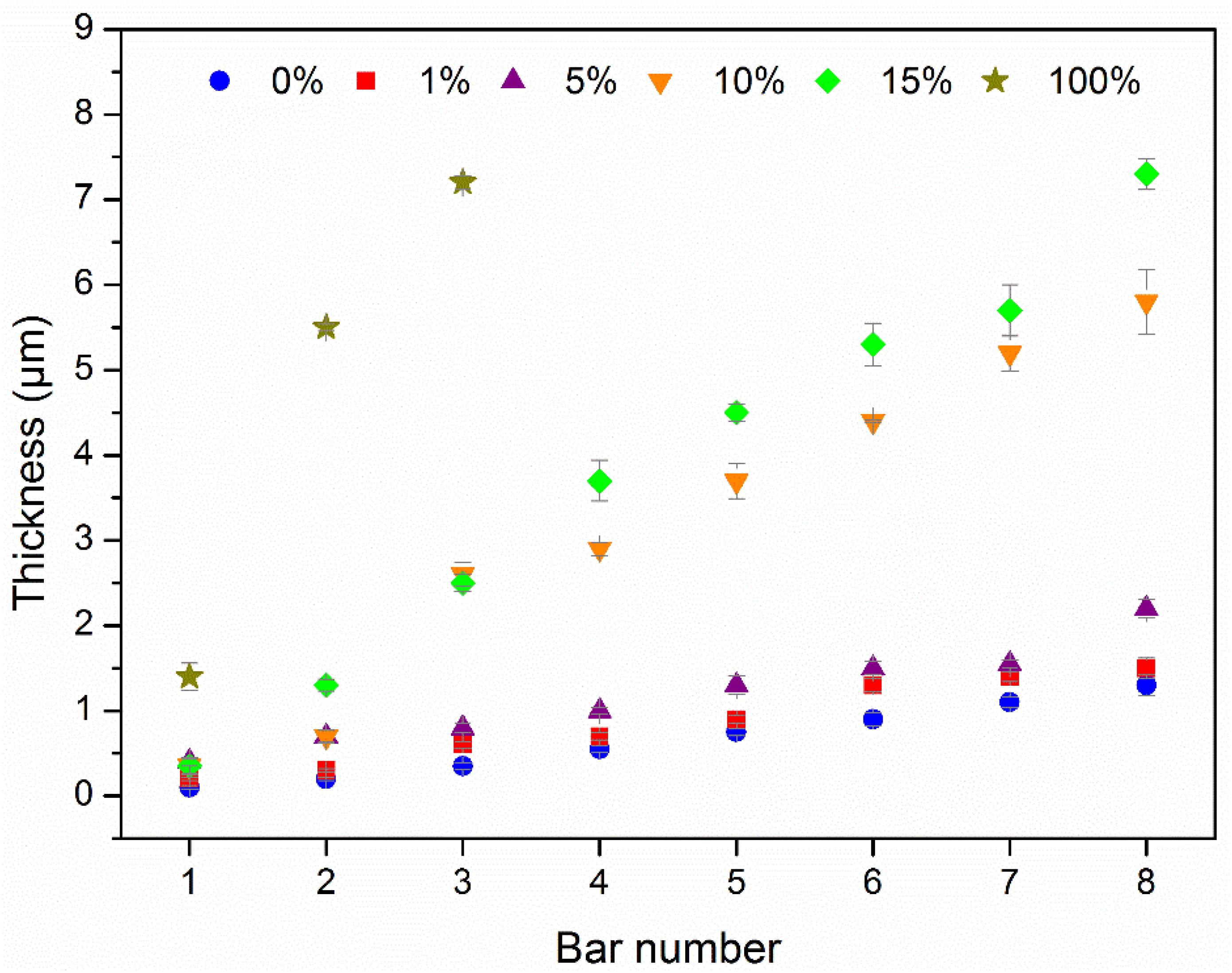
Figure 2. Evolution of layer thicknesses according to the bar number and the wt% of V2O5. Four measurements per sample were carried out showing a good film homogeneity.
If a low thickness increase is measured up to 5 wt% of V2O5, a first jump is observed for 10 wt% and 15 wt% while pure V2O5 reaches thickness of 7 μm already for bar n = 3. Using the bar (n = 3) corresponding to Figure 1, the thicknesses of the films vary from 0.6 μm for pure PEDOT:PSS and 1 wt% V2O5, 0.8 μm for 5 wt% V2O5, 2.5 μm for 10 wt% and 15 wt% V2O5 up to 7.2 μm for pure V2O5. The aspect and the chromaticity parameters of the films deposited with the bar n = 3 are relatively similar to the bar with a higher whorl diameter as the chromaticity parameters of the as-deposited state remain quite similar even when the film thicknesses increase.
The crystallinity of the hybrid films was studied by X-ray diffraction analysis (Figure 3). The PEDOT:PSS layer (Figure 3a) shows an amorphous behavior, whereas for the hybrid film with 1 wt% of V2O5 a first peak of V2O5 can be identified with a very weak signal associated with a low content (Figure 3b). For the 5 wt% V2O5 hybrid film, the peaks intensities increase with the appearance of a second peak (Figure 3c). With the film containing 10 wt% V2O5, all the peaks can be indexed as V2O5 (Space Group:Pmmm) (Figure 3d) revealing an agreement between color and cristallinity. Indeed, the d-spacing values of all diffraction peaks match the ones of the orthorhombic crystalline phase (JCPDS# 85-0601) and of the ITO substrate (JCPDS # 44-1087).
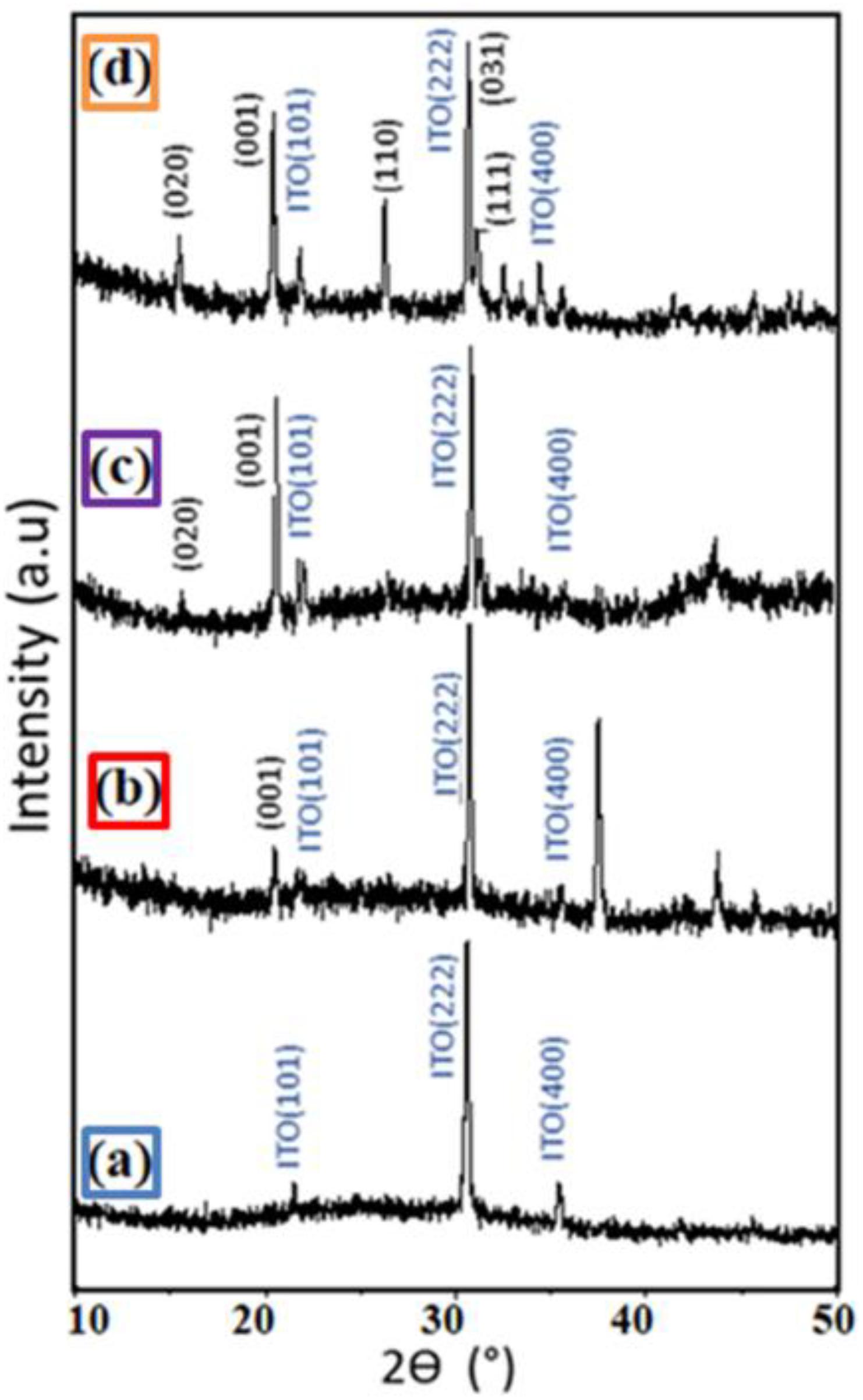
Figure 3. XRD patterns of the (a) film of PEDOT:PSS (b) film hybrid 99 wt%–1 wt% PEDOT:PSS-V2O5 (c) film hybrid 95 wt%–5 wt% PEDOT:PSS-V2O5 and (d) film hybrid 90 wt%–10 wt% PEDOT:PSS-V2O5. All the films were deposited by bar coater (bar n = 3) at room temperature.
The SEM analysis of the hybrid films (V2O5, PEDOT:PSS and hybrid films PEDOT:PSS-V2O5) (Figure 4) demonstrates that the films morphology changes with the amount of V2O5. The PEDOT:PSS film appears smooth and homogenous (Figure 4a). The SEM micrograph of the hybrid composite film 99 wt%–1 wt% PEDOT:PSS-V2O5 (Figure 4b), 5 wt% V2O5 (Figure 4c), 10 wt% V2O5 (Figure 4d) shows a homogenous dispersion of the V2O5 particles in the PEDOT:PSS ink. Not surprisingly, the higher is the amount of V2O5, the rougher is the surface and the more particles are visible. The V2O5 film appears homogenous and resembles to a collection of the sub-micronic particles (100 nm) of V2O5 (Figure 4e). The V2O5 particles seem to form bigger aggregate especially when the vanadium oxide amount increases. The 10 wt% V2O5 and the 100 wt% V2O5 films surfaces appear very similar. For the 10% hybrid film, the oxide particles seem to be embedded in the PEDOT:PSS which has an impact on the chromatic parameters as it will be mentioned later in the paper.
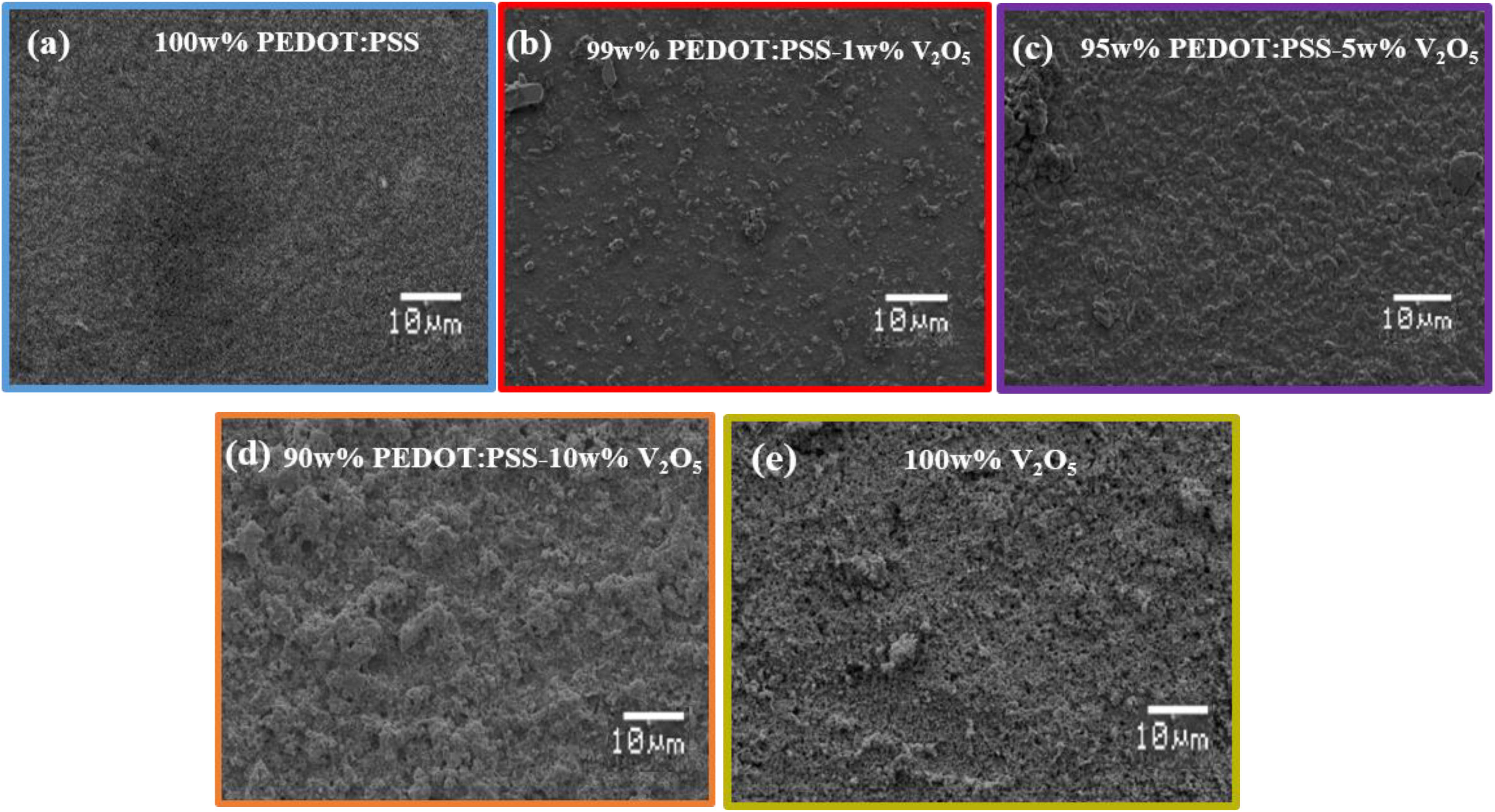
Figure 4. SEM of the (a) 100%PEDOT:PSS. (b) film hybrid 99 wt%–1 wt% PEDOT:PSS-V2O5. (c) film hybrid 95 wt%–5 wt% PEDOT:PSS-V2O5. (d) film hybrid 90 wt%–10 wt% PEDOT:PSS-V2O5. (e) 100 wt% V2O5 film.
Electrochromic Properties of PEDOT:PSS-V2O5 Hybrid Films
The electrochromic activity of the hybrid PEDOT:PSS-V2O5 films was recorded in a lithium-based ionic liquid electrolyte. Figure 5 shows the cyclic voltammograms of single V2O5, single PEDOT:PSS and hybrid PEDOT:PSS-V2O5 films deposited onto ITO and cycled, at a scan rate of 20 mV s–1 between −1 V and +1.5 V, in a three electrodes cell using ionic liquid based LiTFSI:EMIM-TFSI (1:9) as a supporting electrolyte and SCE as reference electrode, for thickness of 1.5 μm. The shape of the cyclic voltammograms adopts significant changes as soon as the addition of 0.1 wt% V2O5 (Figure 5A). A small amount of V2O5 induces a high reactivity associated with strong increase in current and featured CV illustrated by the presence of redox peaks both in anodic and cathodic sweeps (Figure 5B). Higher peak resolution is achieved by cycling thinner films as reported in Figure 5B inset. The CV shape of a 1 wt% submicron film of 500 nm displays a specific signature with both anodic and cathodic distinguishable peaks. Such signature recalls the one observed for crystallized sputtered V2O5 thin films (Benmoussa et al., 2002). These changes in the electrochemical behavior do not have a strong impact for film with a V2O5 amount lower than 1 wt%. The comparison of the CV intensities indicates lower performances for hybrid films, PEDOT:PSS-V2O5 as compared to pure V2O5 film alone.
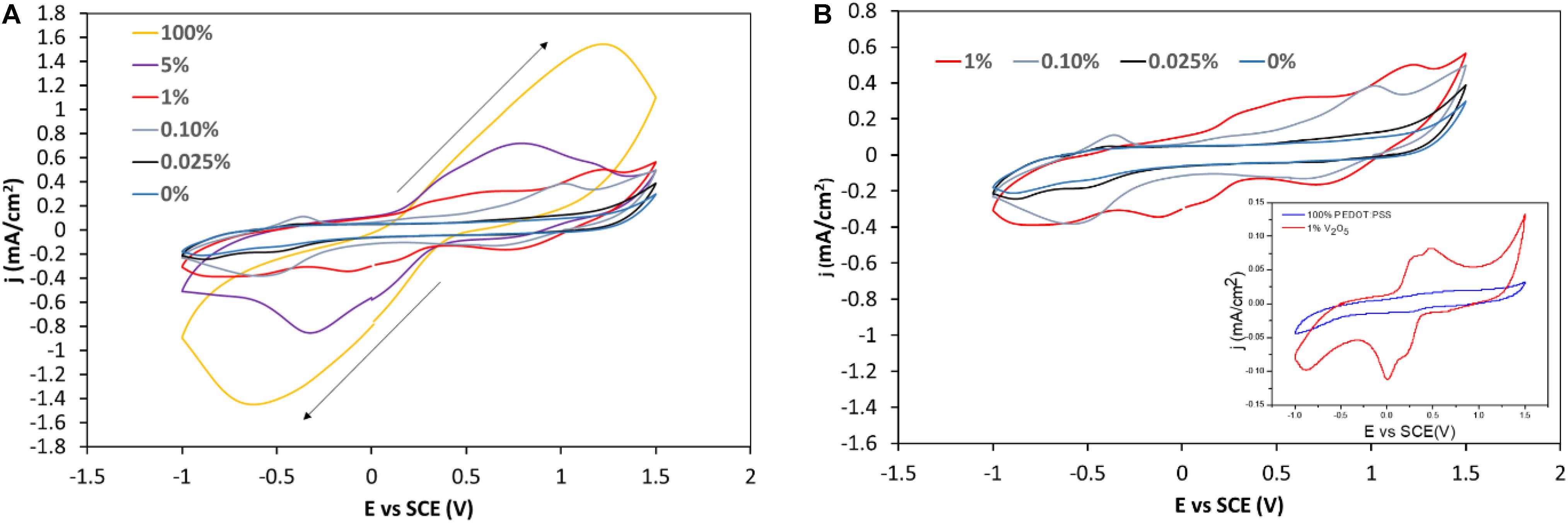
Figure 5. (A) Cyclic Voltammograms (CVs) of various wt% hybrid films PEDOT:PSS-V2O5 cycled in PEDOT:PSS-V2O5/Li-electrolyte/Pt vs. SCE using a 20 mV/s scan rate. (B) Zoom on the CVs of the film containing 1 wt% or less V2O5, inset CV of the 99%–1% PEDOT:PSS-V2O5 with a thickness of 0.5 μm.
Figure 6 shows the chronoamperograms recorded for 30 s at +1.5 V (Figure 6A) and for 30 s at −1 V (Figure 6B). The CA was applied for five cycles, the second cycle is drawn for a film thickness of 1.5 μm. Starting from 1 wt% V2O5, the switching time increases with a higher current density. This trend well agrees with the faster kinetic reported for electrochromic conductive polymers as compared to oxides (Thakur et al., 2012).
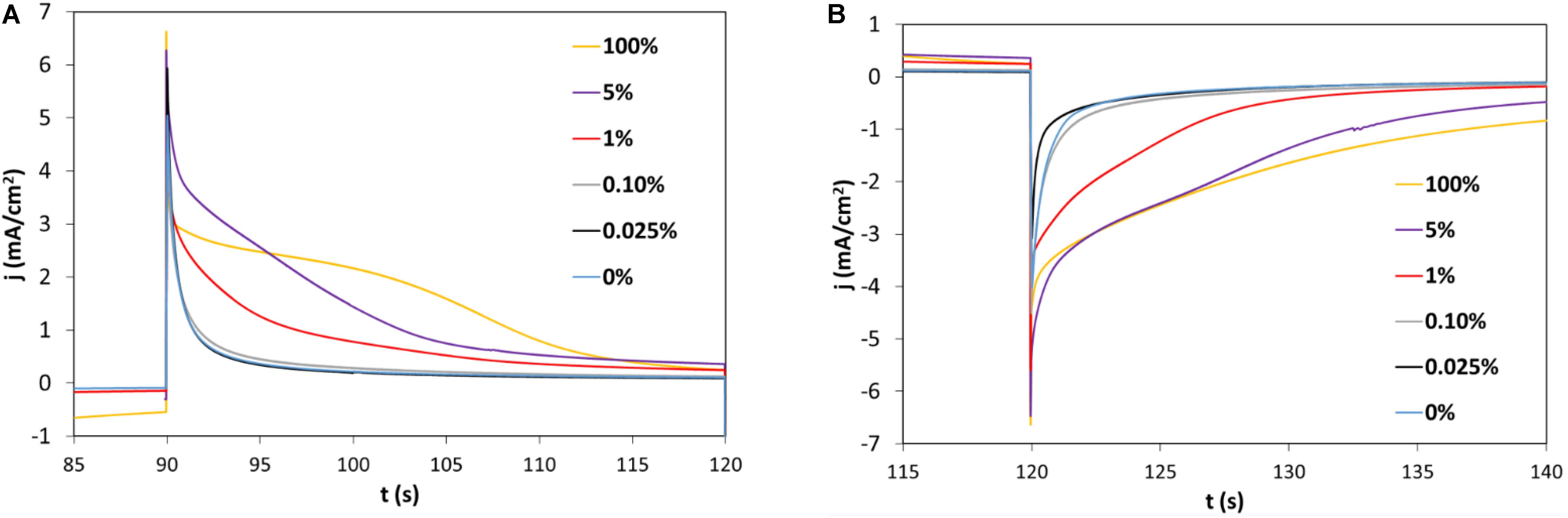
Figure 6. Chronoamperograms (CA) of wt% hybrid PEDOT:PSS-V2O5 cycled in LiTFSI:EMIM-TFSI (1:9) electrolyte/Pt vs. SCE with (A) the oxidation at E vs. SCE = +1.5 V and (B) the reduction at E vs. SCE = –1 V (second cycle is represented).
In order to evaluate the electrochromic properties, the chromaticity parameters L∗, a∗, b∗ were measured during chronoamperometry cycles. These three parameters defined by the CIE colorimetric space, are used to define and compare quantitatively the colors. The optical contrast (ΔE∗) can be deduced from these three chromaticity parameters thanks to equation (1)
Where , , represent the chromaticity parameters in reduced state and , , the ones in oxidized state, at a voltage of −1 V and +1.5 V for 30 s for each cycle, respectively. The optical contrast is determined for each film after two cycles of CA. Figure 7 displays the evolution of the optical contrast vs. the layer thickness for each wt%. A certain thickness is required to obtain a good change of color from the oxidized to the reduced state.
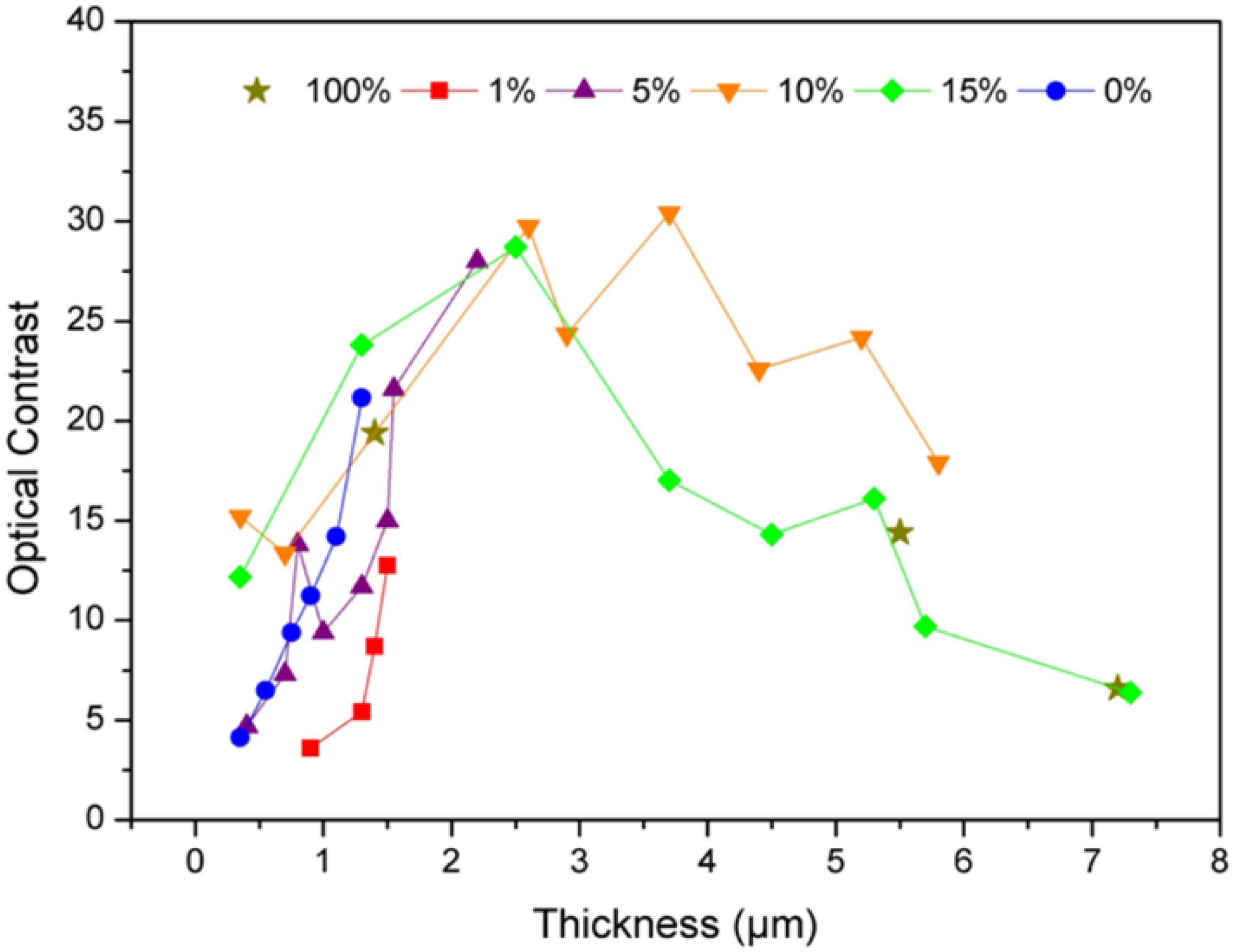
Figure 7. Optical contrast ΔE* in relation with the thickness of the electrochromic layer and the wt% V2O5 in the PEDOT:PSS ink.
However, over a thickness of 4 μm, contrast decrease for all the films containing at least 10 wt% of vanadium oxide. The decrease in contrast for a thick layer may illustrate a limitation in the redox process that does not occur only on the surface of the film but remain limited to a certain thickness. For a high oxide amount (above 10 wt%) layer for the mixture between PEDOT:PSS and V2O5 the film resistance increases also, which is in agreement with a longer color switching time.
In order to minimize the amount of V2O5 for cost reason and to obtain a similar contrast value as for pure vanadium oxide, the ink formulation with 90 wt%–10 wt% PEDOT:PSS-V2O5 was chosen as the best candidate for a satisfying color change and a good thickness control.
Electrochromic Properties of 90 wt%–10 wt% PEDOT:PSS-V2O5
A more detailed study focusing on the 90 wt%–10 wt% PEDOT:PSS-V2O5 was then carried out to evaluate the impact of the thickness on the electrochromic performance. Figure 8 shows the CVs for various thicknesses, the electrochemical behavior of a submicron film is very different from the behavior of a film with a thickness of 2.5 μm and more (Figure 8A). The current density value does not evolve much for films over this thickness. The same observation can be deduced from the CA curves (Figure 8B) with furthermore, a shift toward higher current density value for layers above 2.5 μm for both oxidation and reductions parts after 20 s. This change in slope may indicate a different redox reaction on the surface of the film that may change with the amount of vanadium oxide.
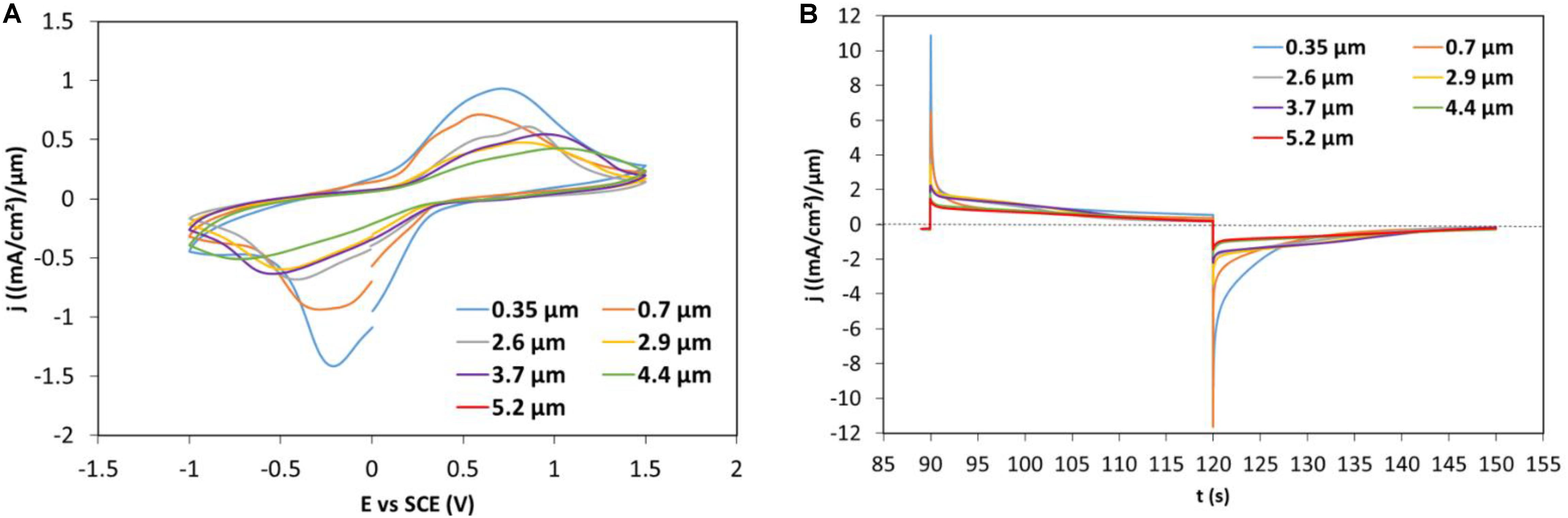
Figure 8. (A) CVs of 90 wt%–10 wt% PEDOT:PSS-V2O5 with various thicknesses cycled in PEDOT:PSS -V2O5/Li-electrolyte/Pt vs. SCE using a 20 mV/s scan rate varying between +1.5 V and –1 V (B) Corresponding CAs of the second cycle with E = +1.5 V for 30 s and E = –1 V for 30 s.
Figure 9 displays the evolution of the chromaticity parameters vs. thickness for the initial (i.e., as-deposited), oxidized (+1.5 V) and reduced (−1 V) states of 100 wt% PEDOT:PSS (in blue), 100 wt%V2O5 (in ochre) and a 90 wt%–10 wt% PEDOT:PSS-V2O5 (in orange). The hybrid PEDOT:PSS-V2O5 and pure V2O5 exhibit similar trend with only a small shift toward lower values of the L∗ parameter for the three states (Figure 9A), more than five points lost for the a∗ parameter (Figure 9B) and again a shift toward lower values for the b∗ parameter (Figure 9C).
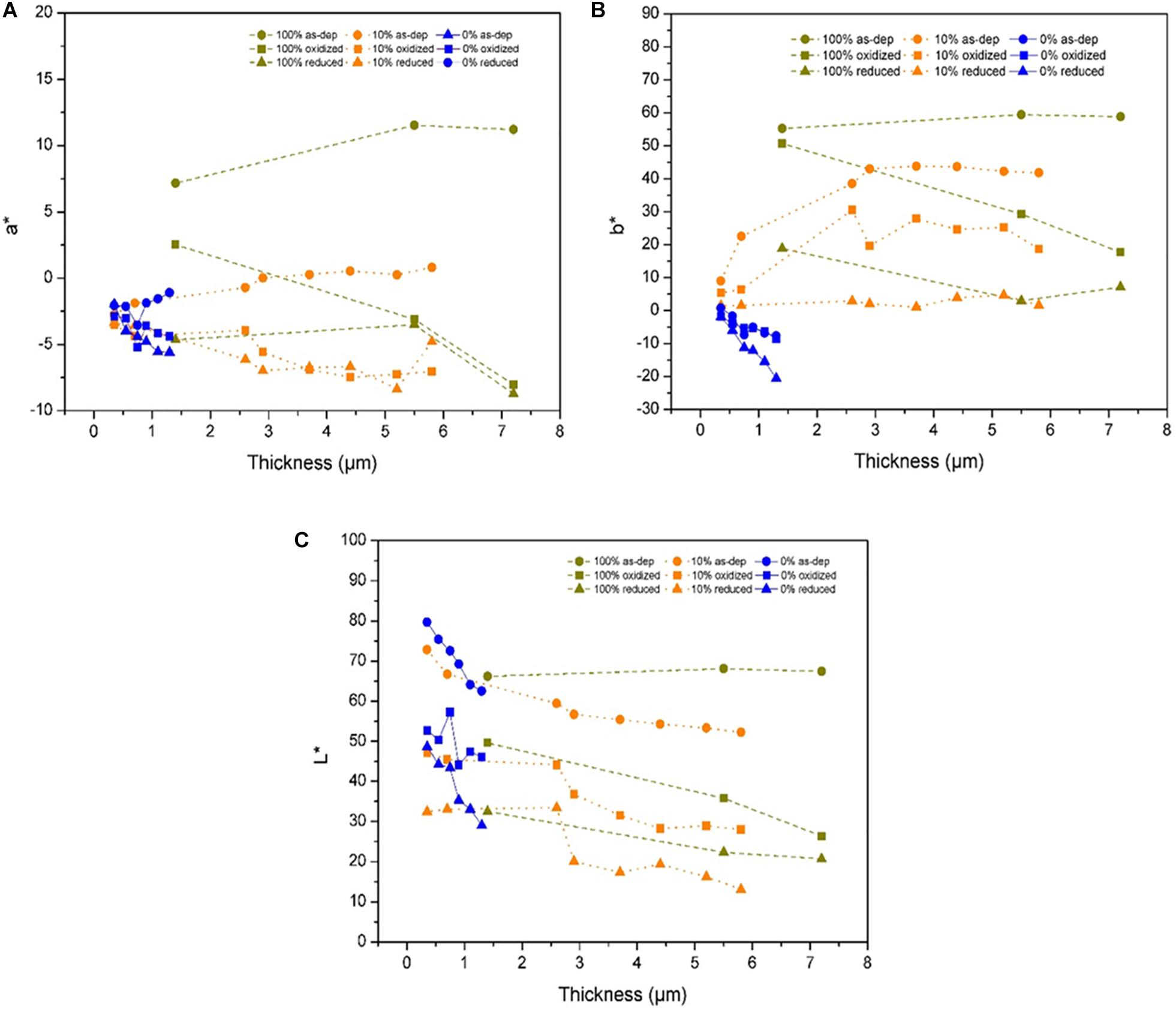
Figure 9. Comparison of the chromaticity parameters between initial, oxidized state at E = +1.5 V and reduced state at E = –1 V with (A) parameter a*. (B) parameter b*. (C) parameter L* for 100 wt% V2O5 10 wt% V2O5, and 0 wt% V2O5.
The presence of PEDOT:PSS, responsible of a slightly darker color, has a definitive impact on the b∗ parameter. The shift toward darker and more bluish films may be correlated to the SEM micrographs on the Figure 4, in which V2O5 appears embedded in PEDOT:PSS.
Initially, the corresponding curve follows the same trend and gives to the mixture a bluer color for low thickness especially in the reduced state. However, and as already observed on the electrochemical behavior, for film above 2.5 μm the V2O5 behavior takes the lead and has the strongest impact on the chromaticity parameters.
On the Figure 10 are gathered, the colors in oxidized and reduced states of the 0 wt% V2O5, 100 wt% V2O5 and 90 wt%–10 wt% PEDOT:PSS-V2O5 films for various thicknesses. For a given thickness, the left picture illustrated the oxidized state (E = +1.5 V) and the right one the reduced state (E = −1 V). The pictures together with the chromaticity parameters were recorded during CA measurements. In agreement with the Figure 8B, the thicker is the layer, the harder the current density is driven to a value close to 0. Therefore after few cycles, the greenish layer of the surface is correlated with an irreversible reaction from V5+ to V4+ with the Li+ insertion.
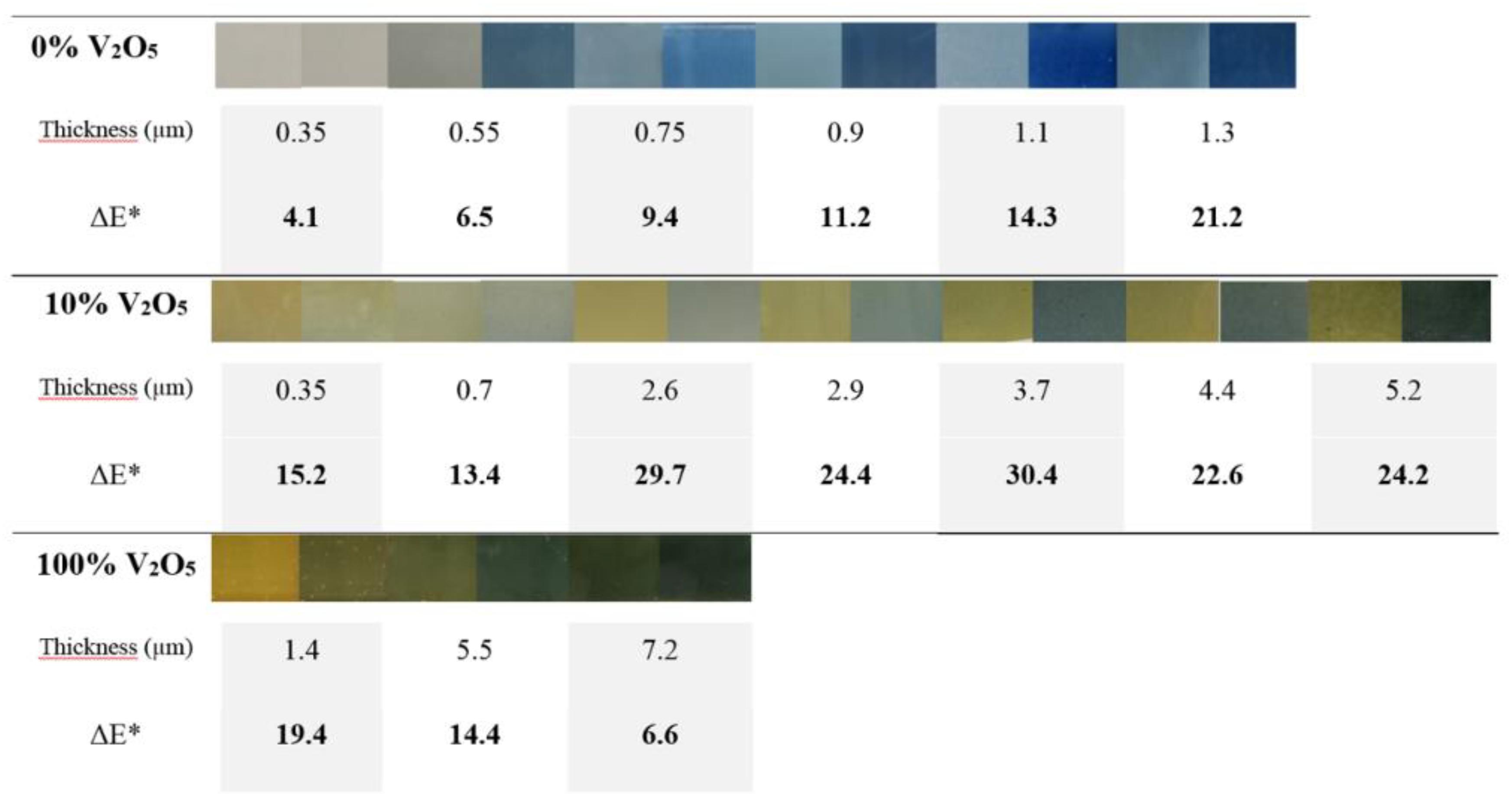
Figure 10. Visual comparison between the colors in the oxidized and reduced states for 0 wt% V2O5, 100 wt% V2O5, and 90 wt%–10 wt% PEDOT:PSS-V2O5 vs. thickness (μm) during CA after three cycles and contrast value ΔE*.
When comparing the CA curves (not shown here) between 0 wt% V2O5, 100 wt% V2O5, and 90 wt%–10 wt% PEDOT:PSS-V2O5, it could be noticed that the 0 wt% V2O5 corresponds to a very fast kinetic with less than 1 s for color change. 10 wt% V2O5 adopts a visual change in 5 s while it takes at least 15 s to observe a change of color from the oxidized to the reduced state for 100 wt% V2O5. As expected the changing color mechanism for PEDOT:PSS is much faster than the one of crystallized V2O5. Besides the presence of the polymer helps to reduce the time necessary to change the color from oxidized to reduced states (and vice-versa) without affecting the optical contrast when only 10%wt V2O5 is added to the polymer ink.
Aiming at a better investigation of the optical properties, the reflectance spectra was recorded on the film with the highest contrast between the oxidized and reduced states for the mixture 90 wt%–10 wt% PEDOT:PSS-V2O5 (e.g., a film thickness of 3.7 μm) (Figure 11). The reversible color changes of hybrid composite films between the two states are associated with reflectance values of about 8.3% for the reduced state and 28.9% for the oxidized state at 550 nm, respectively, leading to an optical reflectance modulation, ΔR, of 20.6%.
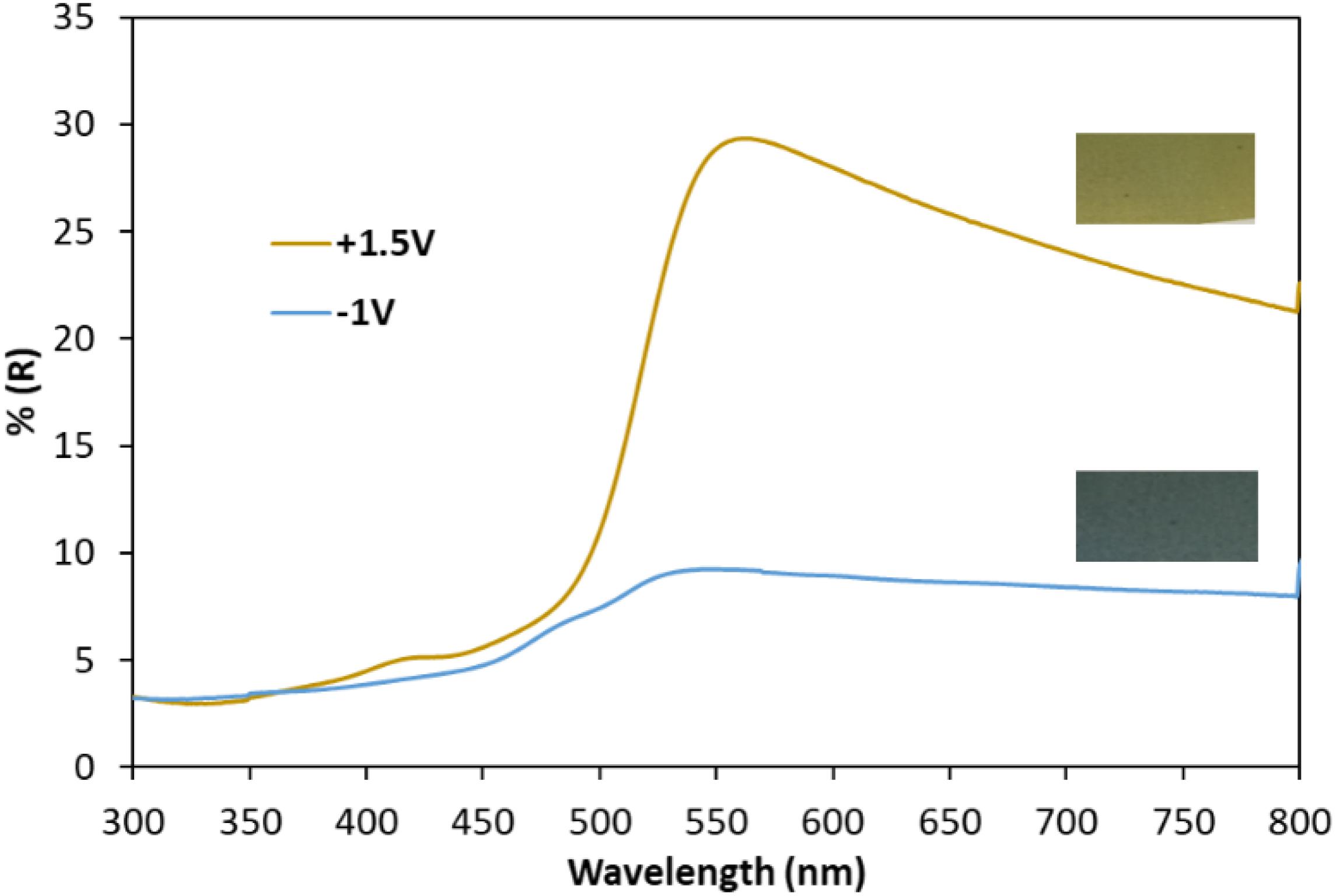
Figure 11. Ex-situ reflectance spectra of the oxidized film (yellow-green) at +1.5 V and the reduced film (blue) at –1 V of the 3.7 μm thick hybrid film of 90 wt%–10 wt% PEDOT:PSS-V2O5.
The electrochromic performance are characterized thanks to the optical density (OD) which can be calculated directly from the reflectance spectra and represents the logarithm of the ratio of the reflectance at the oxidized state (Rox) to the reflectance at the reduced state (Rred) as shown on the Equation 2.
A ΔOD of 0.54 is estimated at 550 nm. The color efficiency (CE) can be calculated with the charge transferred per unit area (Q) deduced from the CA curves.
In this case, Q = 1.85 C.cm–2 and CE obtained equals to 0.29 cm2.C–1. This rather low value may be explained by a measurement of the capacity in chronoamperometry mode rather than in dynamic mode using cyclicvoltammetry. Besides, there is a lack of comparison with other hybrid systems mixing two electrochromic materials as a single layer.
Memory Effect of 90 wt%–10 wt% PEDOT:PSS-V2O5 Film
Figure 12 gathers the chromaticity parameters of the 0 wt% V2O5 and the 90 wt%–10 wt% PEDOT:PSS-V2O5 films for different thicknesses recorded as initial state, in reduced state (−1 V), and after resting the film for 3 months. No voltage was applied meanwhile and films were stored at ambient atmosphere and room temperature. The PEDOT:PSS film goes back to its most stable state exhibiting no memory effect with a very low value difference between initial and 3 months values. On the contrary, as reported by our group in a prior work, V2O5 shows a strong memory effect (Mjejri et al., 2018a). After 3 months, if the chromaticity parameters exhibit different values as compared to the first applied voltage at E = −1 V they also display a big difference with the initial data. From a blue-green film at E = −1 V, a green film is visible after 3 months. The mixture of 10 wt% V2O5 in the PEDOT:PSS induced a memory-effect.
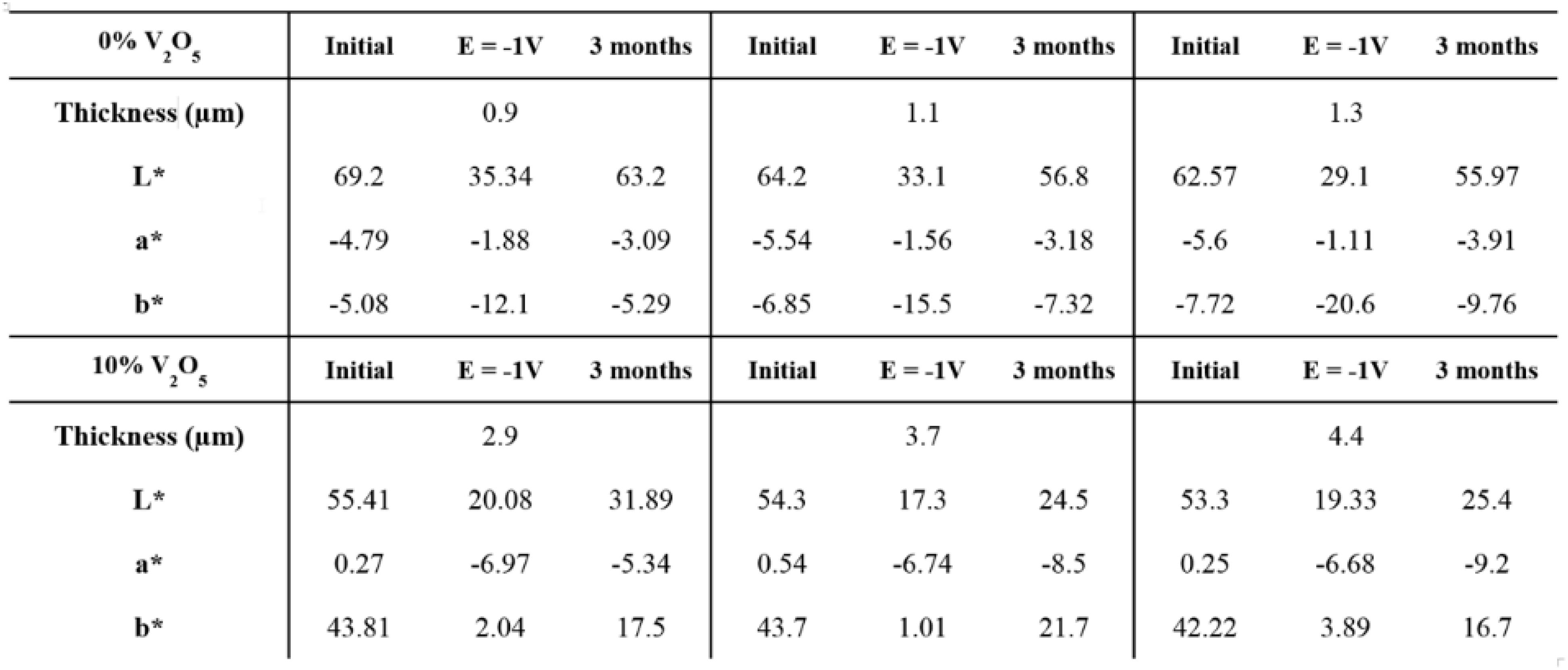
Figure 12. Illustration of the memory-effect by comparison between the standard PEDOT:PSS and 90 wt%–10 wt% PEDOT:PSS-V2O5 with chromaticity parameters taken at the initial stage, at E = –1 V and after 3 months after applying first E = –1 V, leaving the samples rest at room temperature and ambient atmosphere without any cycle in between.
Conclusion
In this paper, hybrid composite films with different compositions between a polymer ink of PEDOT:PSS and V2O5 synthesized by the polyol process were successfully deposited with a bar coater technique. The influence of the film thickness according to the amount of vanadium oxide in PEDOT:PSS-V2O5 hybrids was studied via electrochemical techniques and surface morphology. The introduction of 10 wt% V2O5 with a film thickness of 3.7 μm is sufficient to mimic the behavior of pure V2O5 change of color with a faster reversible color change from oxidized to reduced states. Indeed 10 wt% V2O5 are sufficient to gain color tuning, significant optical contrast and memory-effect suggesting that there is no need to mix further inorganic material in the polymer. Our approach is a promising route to improve the electrochromic properties including the tuning of color of displays based on PEDOT:PSS-based ink in a very simple way, combining advantages of V2O5 inorganic and PEDOT:PSS polymer materials. The memory-effect was also improved for hybrid films as compared to single PEDOT-PSS. Furthermore, dispersing the vanadium oxide in a polymer ink reduces the overall toxicity, the cost, improves the process control and allows the use of the hybrid in industrial technique such as screen-printing which is promising for developing large-scale electrochromic displays.
Data Availability Statement
All datasets generated for this study are included in the article/supplementary material.
Author Contributions
RF, IM, and HR conducted the investigation and methodology. AR supervised the project. RF wrote the original draft.
Funding
This activity included in the SUPERSMART project has received funding from the European Institute of Innovation and Technology (EIT) (Grant Agreement: 17161). This body of the European Union receives support from the European Union’s Horizon 2020 Research and Innovation Program. RF wishes to thank the financial support of Luquet-Duranton.
Conflict of Interest
RF was employed by the company Luquet-Duranton.
The remaining authors declare that the research was conducted in the absence of any commercial or financial relationships that could be construed as a potential conflict of interest.
Acknowledgments
The authors wish to thank Eric Lebraud for the XRD measurements.
References
Andersson, P. (2006). Electrochromic Polymer Devices: Active-Matrix Displays and Switchable Polarizers. Licentiate Thesis, Linköping University, Sweden.
Benmoussa, M., Outzourhit, A., Bennouna, A., and Ameziane, E. L. (2002). Electrochromism in Sputtered V2O5 thin films: structural and optical studies. Thin Solid Films 405, 11–16. doi: 10.1016/S0040-6090(01)01734-5
Blanquart, T., Niinistö, J., Gavagnin, M., Longo, V., Heikkilä, M., Puukilainen, E., et al. (2013). Atomic layer deposition and characterization of vanadium oxide thin films. RSC Adv. 3, 1179–1185. doi: 10.1039/C2RA22820C
Byker, H. (2001). Electrochromics and polymers. Electrochim. Acta 46, 2015–2022. doi: 10.1016/S0013-4686(01)00418-2
Chu, J., Kong, Z., Lu, D., Zhang, W., Wang, X., Yu, Y., et al. (2016). Hydrothermal synthesis of vanadium oxide nanorods and their electrochromic performance. Mater. Lett. 166, 179–182. doi: 10.1016/j.matlet.2015.12.067
Costa, C., Pinheiro, C., Henriques, I., and Laia, C. A. T. (2012). Electrochromic properties of inkjet printed vanadium oxide gel on flexible Polyethylene Terephthalate/Indium Tin oxide electrodes. ACS Appl. Mater. Interf. 4, 5266–5275. doi: 10.1021/am301213b
Danine, A., Cojocaru, L., Faure, C., Olivier, C., Toupance, T., Campet, G., et al. (2014). Room temperature UV treated WO3 thin films for electrochromic devices on paper substrate. Electrochim. Acta 129, 113–119. doi: 10.1016/j.electacta.2014.02.028
Danine, A., Manceriu, L., Faure, C., Labrugère, C., Penin, N., Delattre, A., et al. (2019). Toward simplified electrochromic devices using silver as counter electrode material. ACS Appl. Mater. Interf. 11, 34030–34038. doi: 10.1021/acsami.9b12380
Granqvist, C. G., Arvizu, M. A., Qu, H., Wen, R., and Niklasson, G. A. (2019). Advances in electrochromic device technology: multiple roads towards superior durability. Surf. Coat. Technol. 357, 619–625. doi: 10.1016/j.surfcoat.2018.10.048
Han, Y., Lin, Y., Sun, D., Xing, Z., Jiang, Z., and Chen, Z. (2019). Poly(Aryl Amino Ketone)-based materials with excellent electrochromic and electrofluorochromic behaviors. Dyes Pigments 163, 40–47. doi: 10.1016/j.dyepig.2018.11.045
Hu, C. H., Yamada, Y., and Yoshimura, K. (2018). Poly(3,4-Alkylenedioxythiophenes): PXDOTs Electrochromic Polymers as Gasochromic materials. Solar Energ. Mater. Solar Cells 187, 30–38. doi: 10.1016/j.solmat.2018.07.022
Jung, Y., Lee, J., and Tak, Y. (2004). Electrochromic mechanism of IrO2 prepared by pulsed anodic electrodeposition. Electrochem. Solid State Lett. 7, H5–H8. doi: 10.1149/1.1634083
Lang, A. W., Osterhölm, A. M., and Reynolds, J. R. (2019). Paper-based electrochromic devices enabled by nanocellulose-coated substrates. Adv. Funct. Mater. 29:1903487. doi: 10.1002/adfm.201903487
Lee, J. S., Choi, Y. J., Park, H. H., and Pyun, J. C. (2011). Electrochromic properties of Poly(3,4-Ethylenedioxythiophene) Nanocomposite Film Containing SiO2 Nanoparticles. J. Appl. Polym. Sci. 122, 3080–3085. doi: 10.1002/app.34130
Lee, S., Kim, H. P., Yusoff, A. R. M., and Jang, J. (2014). Organic photovoltaic with PEDOT:PSS and V2O5 mixture as hole transport layer. Solar Energ. Mater. Solar Cells 120, 238–243. doi: 10.1016/j.solmat.2013.09.009
Levasseur, D., Mjejri, I., Rolland, T., and Rougier, A. (2019). Color tuning by oxide addition in PEDOT:PSS-based electrochromic devices. Polymers 11:179. doi: 10.3390/polym11010179
Mjejri, I., Doherty, C. M., Rubio-Martinez, M., Drisko, G. L., and Rougier, A. (2017). Double-sided electrochromic device based on metal–organic frameworks. ACS Appl. Mater. Interf. 9, 39930–39934. doi: 10.1021/acsami.7b13647
Mjejri, I., Gaudon, M., and Rougier, A. (2019). Mo addition for improved electrochromic properties of V2O5 thick films. Solar Energ. Mater. Solar Cells 198, 19–25. doi: 10.1016/j.solmat.2019.04.010
Mjejri, I., Gaudon, M., Song, G., Labrugère, C., and Rougier, A. (2018a). Crystallized V2O5 as oxidized phase for unexpected multicolor electrochromism in V2O3 thick film. ACS Appl. Energ. Mater. 1, 2721–2729. doi: 10.1021/acsaem.8b00386
Mjejri, I., Grocassan, R., and Rougier, A. (2018b). Enhanced coloration for hybrid niobium-based electrochromic devices. ACS Appl. Energ. Mater. 1, 4359–4366. doi: 10.1021/acsaem.8b00967
Mjejri, I., Manceriu, L. M., Gaudon, M., Rougier, A., and Sediri, F. (2016). Nano-vanadium pentoxide films for electrochromic displays. Solid State Ionics 292, 8–14. doi: 10.1016/j.ssi.2016.04.023
Nunes, S. C., Saraiva, S. M., Pereira, R. F. P., Pereira, S., Silva, M. M., Carlos, L. D., et al. (2019). Sustainable dual-mode smart windows for energy-efficient buildings. ACS Appl. Energ. Mater. 2, 1951–1960. doi: 10.1021/acsaem.8b02041
Qingyi, L., Xu, Z., Wanan, C., Yan, W., Caiyu, Y., Ye, C., et al. (2019). Donor-acceptor conjugated polymers containing isoindigo block for novel multifunctional materials for electrochromic, resistance memory, and detector devices. Solar Energ. Mater. Solar Cells 200:109979. doi: 10.1016/j.solmat.2019.109979
Rodrigues, L. C., Barbosa, P. C., Silva, M. M., Smith, M. J., Gonçalves, A., and Fortunato, E. (2009). Application of hybrid materials in solid-state electrochromic devices. Opt. Mater. 31, 1467–1471. doi: 10.1016/j.optmat.2009.02.004
Runnerstrom, E. L., Llordés, A., Lounis, S. D., and Milliron, D. J. (2014). Nanostructured electrochromic smart windows: traditional materials and NIR-selective plasmonic nanocrystals. Chem. Commun. 50, 10555–10572. doi: 10.1039/C4CC03109A
Tashtoush, N., and Kasasbeh, O. (2013). Optical properties of vanadium pentoxide thin films prepared by thermal evaporation method. Jordan J. Pharmaceut. Sci. 6:1. doi: 10.31257/2018/jkp/100101
Thakur, V. K., Ding, G., Ma, J., Lee, P. S., and Lu, X. (2012). Hybrid materials and polymer electrolytes for electrochromic device applications. Adv. Mater. 24, 4071–4096. doi: 10.1002/adma.201200213
Keywords: electrochromism, PEDOT:PSS, V2O5, color tuning, bar coating, hybrid materials
Citation: Futsch R, Mjejri I, Rakotozafy H and Rougier A (2020) PEDOT:PSS-V2O5 Hybrid for Color Adjustement in Electrochromic Systems. Front. Mater. 7:78. doi: 10.3389/fmats.2020.00078
Received: 22 December 2019; Accepted: 18 March 2020;
Published: 30 April 2020.
Edited by:
Verónica de Zea Bermudez, University of Trás-os-Montes and Alto Douro, PortugalReviewed by:
Aysegul Uygun Oksuz, Süleyman Demirel University, TurkeyVishnu Baba Sundaresan, The Ohio State University, United States
Copyright © 2020 Futsch, Mjejri, Rakotozafy and Rougier. This is an open-access article distributed under the terms of the Creative Commons Attribution License (CC BY). The use, distribution or reproduction in other forums is permitted, provided the original author(s) and the copyright owner(s) are credited and that the original publication in this journal is cited, in accordance with accepted academic practice. No use, distribution or reproduction is permitted which does not comply with these terms.
*Correspondence: Aline Rougier, YWxpbmUucm91Z2llckBpY21jYi5jbnJzLmZy