- 1School of Materials Science and Engineering, Chang’an University, Xi’an, China
- 2Engineering Research Center of Transportation Materials, Ministry of Education, Chang’an University, Xi’an, China
- 3School of Construction Machinery, Chang’an University, Xi’an, China
- 4School of Highway, Chang’an University, Xi’an, China
Cement asphalt emulsion mixture (CAEM) presents low early-age strength and poor flowability, which limits its application range at a certain degree. In this paper, CAEM with high early-age strength and flowability was proposed. The flowability, compressive strength, flexural strength of CAEM at different periods and 28 days elastic modulus of CAEM were investigated. In addition, evolution of composition and microstructure of CAEM was analyzed by X-ray diffraction (XRD), scanning electronic microscope (SEM), and mercury intrusion porosimetry (MIP). The results indicated that the compressive strength of CAEM can be enhanced quickly in several hours. It could achieve 17–24 MPa at 6 h and almost reached 79–90% of the 28 days compressive strength. SEM and XRD analysis found that the hydration products in CAEM at different periods were obviously different. The hydration products of 2 h filled the micro pores of the mixture. After 28 days, the hydration products were connected to each other, resulting in a denser structure. The results of MIP analysis showed that the pore cumulative volume of CAEM was similar for 2 h and 28 days samples. These results testified that CAEM had high early-age strength.
Introduction
Cement asphalt emulsion mixture (CAEM) is an organic-inorganic composite material formed by the combination of hydration of cement and demulsification of emulsified asphalt. CAEM has been widely used in road maintenance and rehabilitation due to the combined merits of the high mechanical properties of cement materials and the flexibility of asphalt materials, low environmental impact, and cost effectiveness (Zhong and Chen, 2002; Lu et al., 2009; Qiang et al., 2011; Doyle et al., 2013; Tian et al., 2013; Tyler et al., 2014; Ling et al., 2016; Ouyang et al., 2020). Despite the aforementioned benefits of CAEM, there is a defect that the corners cannot be fully compacted and result in a poor adhesion between new and old materials due to the poor rheological property of CAEM (Hu et al., 2009; Dołżycki et al., 2017; Liu and Liang, 2017). There will be secondary damage occurred on the repair site when it is exposed to the adverse weather such as rainfall or freezing and thawing. In addition, repair materials should meet the strength requirements to early open to traffic at different conditions. Studies have shown that it took a long time for repair materials to reach their full strength (Qiang et al., 2011; Fang et al., 2016; Liu and Liang, 2017; Du, 2018). Therefore, in order to improve the strength of the road and early open to traffic, the properties of the new CAEM must satisfy the requirements of good flowability and high early-age strength.
It is a common belief that increasing the dosage of cement could effectively improve the strength of CAEM (Lu et al., 2009; Yan et al., 2017; Ouyang et al., 2018). Yan et al. (2017) has studied the early strength of the asphalt emulsion mixture with various cement contents, and the results showed that the addition of cement to asphalt emulsion mixture improved its early-age strength. With the increase of cement content, the strength of mixture increased. However, excessive contents of cement may cause cracks in the pavement. Liu and Liang (2017) has reported that the flow time increased with the increase of asphalt to cement ratio (A/C), while the compressive strength, and 28 days elastic modulus showed the opposite trend. Lin et al. (2015) has studied the mechanism of early strength of CAEM and concluded that cement played a major role in the first 3-day of early strength, while emulsified asphalt played the predominant role in both early and final strength. Therefore, the effect of A/C on the early strength of CAEM is important. Studies have reported that the type of asphalt would also affect the strength of CAEM. Normally, both cationic and anionic asphalt emulsion are utilized in different types of CAEM. Research works conducted by Plank and Hirsch (2007), Tan et al. (2013), Ouyang et al. (2016), Li et al. (2018), etc. have shown that the anionic asphalt emulsion was more suitable than cationic asphalt emulsion for formulating CAEM when a higher strength was required. Therefore, an anionic asphalt emulsion was selected in the selection of materials in order to improve the strength of the CAEM.
As is well known, the water to cement ratio (W/C) exerts a major influence on the flowability of cement concrete and can be expected to have an important effect on the flowability of CAEM. Ouyang et al. (2019b) has studied the effect of water content on mechanical properties of cement bitumen emulsion mixture and recommended that the optimum water content of cement bitumen emulsion mixture should be determined by the maximum indirect tensile strength in the mix design. In addition, the emulsified asphalt contains moisture, Ouyang and Shah (2018) also reported that the dosage of asphalt emulsion was the main factor influencing the rheological property of the fresh cement asphalt emulsion mortar. It was verified that the pore structures of hydration products had remarkable influences on the mechanical properties and durability of cementitious materials (Zhou et al., 2018; Zhou et al., 2020a, b), and were affected by W/C ratio obviously. Therefore, it is necessary to systematically study the high flowability properties and microstructure of CAEM with various W/C ratios.
In this paper, a new kind of CAEM with high early-age strength and flowability was proposed, the flowability, compressive strength, and flexural strength of CAEM at different periods and 28 days elastic modulus of CAEM were investigated. Additionally, X-ray diffraction (XRD), Scanning Electron Microscope (SEM), and mercury intrusion porosimetry (MIP) were used to characterize the microstructure evolution of the CAEM. The research results would provide reference parameters for pavement construction.
Materials and Experimental Methods
Materials
The selection and basis of materials were list as following:
(1) Asphalt emulsion: asphalt emulsion with evaporated residue content of 60.0% was prepared. The properties of asphalt emulsion are shown in Table 1. The test methods of asphalt emulsion were in accordance to Chinese Standard JTJ E20-2011.
(2) Cement: in order to obtain high early-age strength of CAEM, Sulphoaluminate cement (SAC 42.5), and Portland cement (P.O 42.5) were mixed with a ratio of 2:8 (wt%). The properties of cement are given in Table 2. The test methods of cement were in accordance to Chinese Standard GB 175-2007.
(3) Aggregate: the coarse aggregates used in this paper were crushed natural aggregates with continuous grading and the fine aggregates used were natural sand. The max particle size of coarse aggregates was 20 mm, the crushing value, sediment percentage, needle and sheet percentage of the coarse aggregates were 12.6, 0.5, and 8.5%, respectively. The fineness modulus of natural sand was 2.8, plotted in Zone II according to Chinese Standard JTG E42-2005.
(4) Additives: Studies (Tan et al., 2013; Ouyang et al., 2019a; Ouyang et al., 2020) have shown that adding additives could effectively improve the performance of CAEM. United expansing agent (solid content 30.1% and density 2750 kg m–3) and polycarboxylate superplasticizer (water reducing rate 25%) were added to improve the volume stability and flowability of CAEM. Additionally, organic silicon defoamer (PH = 7) was used to reduce air void content of CAEM, the compound admixture was used to control the setting time of CAEM, which was a mixture of boracic acid (H3BO3) and lithium carbonate (Li2CO3) in a ratio of 4:1 (wt%).
Mix Proportions
The raw materials were mixed in the bowl in this order: Firstly, aggregate and half of water mixed together for about 2 min; Secondly, the blended cement and additives mixed together for about 2 min, and then the remaining water and asphalt emulsion mixed together for 5 min until a homogeneous CAEM was obtained.
Mix proportions of CAEM are listed in Table 3. The water mentioned in the mix proportion does not include the water in the asphalt emulsion.
Experimental Methods
Flowability
The flowability of fresh CAEM was measured by the slump of the mixture according to the practical experience and Chinese Standard JTJ E30-2005.
Early-Age Mechanical Properties
The early-age mechanical properties of CAEM were quantified by compressive strength and flexural strength at 2 h, 6 h, 3 days, and 28 days, respectively. Cubic specimens with the dimension 100 mm × 100 mm × 100 mm were utilized for compressive strength. Beam specimens of 100 mm × 100 mm × 400 mm were used for flexural strength. Beside this, 28 days elastic modulus of CAEM was carried out on the specimens of 150 mm × 150 mm × 300 mm (according to Chinese Standard JTJ E30-2005). Three specimens were prepared for each test result. The specimens were prepared with concrete vibrator, and all specimens were cured at a temperature of 20 ± 2°C and a humidity of 95% (RH) until the testing date.
XRD, SEM, and MIP
X-ray diffraction was used in this study to observe the hydration of blended cementitious materials of CAEM. CAEM specimens were ground to a powder and passed through a 63 μm sieve for XRD analysis (Bołtryk and Małaszkiewicz, 2013).
Scanning Electron Microscope was used to observe the microstructure characterization of CAEM. The cured specimens were shaped to cube with 1.5 cm by side length. Then, the surface of the samples was sputter covered with a thin layer of gold before the SEM observation (Likitlersuang and Chompoorat, 2016; Li et al., 2018).
Pore cumulative volume and pore size distribution of CAEM were measured by an Auto Pore IV 9510 MIP. Crushed pieces of the samples were soaked in an absolute ethyl alcohol solution to stop the hydration before MIP test (Wang et al., 2017).
Results and Discussion
Flowability
Figure 1 shows the flowability results of fresh CAEM with various A/C ratios and various W/C ratios. It can be seen that the flowability of fresh CAEM was strongly affected by both A/C ratio and W/C ratio. As shown in Figure 1, the flowability of CAEM decreased with the increase of A/C ratio. A possible explanation was that the increase of A/C ratio caused the cement and asphalt emulsion to aggregate together, then entrapping water, and resulting in the decrease of flowability of CAEM (Wang et al., 2015). On the contrary, the flowability of CAEM increased with the increase of W/C ratio, and the slump reached almost 220 mm when the W/C ratio was 0.32.
It can also be concluded from Figure 1 that in order to obtain greater flowability of CAEM (the slump more than 200 mm), the A/C ratio, and W/C ratio can be adjusted based on the experimental experience.
Early-Age Mechanical Performance
Compressive Strength
Figure 2 shows the compressive strength results of CAEM with various W/C ratios and A/C ratios after 2 h, 6 h, 3 days, and 28 days of curing. From Figure 2, it can be concluded that the compressive strength of CAEM could reach more than 10 MPa at 2 h age, due to the use of composite cement in the mixture. It also can be seen that a significant reduction in the compressive strength of CAEM was obtained as the W/C ratio increased. As the W/C ratio increased from 0.30 to 0.32, the compressive strength decreased by up to 32.1% at 2 h, and 13.3% at 28 days, respectively. This indicated that increasing W/C ratio provides a negative effect on the compressive strength of CAEM.
It should be noted that the change trend of A/C was consistent with that of W/C. As the A/C ratio increased from 0.18 to 0.30, the compressive strength decreased by up to 38.6% at 2 h and 23.3% at 28 days. With the increase of A/C, the compressive strength decreased due to the droplets of asphalt emulsion dispersed in the cement matrix. In addition, the compressive strength at 6 h was approximately 79–90% of the 28 days compressive strength of CAEM, which almost reached 17–24 MPa. As is well known, the hydration rate of calcium sulfoaluminate cement is very fast compared to Portland cement, so that a good supply of hydration products are generated during the initial hydration period of CAEM, and the porosity is filled by the main hydration products, which contributes to the rapid increase of the early strength of CAEM.
Flexural Strength
Figure 3 shows the flexural strength results of CAEM with various W/C ratios and A/C ratios. From Figure 3, it can be seen that the flexural strength of CAEM decreased with the increase of W/C ratio or A/C ratio. The increase in A/C resulted in a more significant reduction in flexural strength of CAEM, indicating that the structure formed by cement hydrates could be weakened by the addition of asphalt emulsion. The flexural strength of W/C 0.30, W/C 0.31, and W/C 0.32 at 6 h were approximately 92.8, 94.5, and 95.3% of the 28 days strength of CEAM, respectively. The flexural strength curve tended to be stable after 6 h, indicating that the flexural strength mainly depended on the structure formed by cement hydrates before 6 h. However, the flexural strength of CAEM was still slightly increasing after 6 h due to the water present in the asphalt emulsion was still slowly released as the A/C ratio increased and the cement continued to hydrate.
Compressive Strength -Flexural Strength Ratio
The compressive strength -flexural strength ratio (C/F ratio) can reflect the toughness of the material. In general, the smaller C/F ratio means the better material toughness and better impact resistance. Conversely, the greater of the C/F ratio, the more brittle the material is, and the worse the impact resistance against the external.
Figure 4 shows the C/F ratio of CAEM with various W/C ratios and A/C ratios. As can be seen from Figure 4, the C/F value increased as the curing time increased, and it increased slightly after 6 h. The higher W/C ratio of CAEM, the lower C/F ratio, indicating that the higher toughness of CAEM. Similarly, the higher A/C ratio of CAEM, the lower C/F ratio, which means the higher the toughness of CAEM.
28 Days Elastic Modulus
From Figure 5, it can be clearly seen that when the W/C ratio was 0.30, the 28 days elastic modulus of CAEM was consistent with that of W/C ratio was 0.31. However, the 28 days elastic modulus of CAEM suddenly decreased when the W/C ratio was 0.32, indicating that the optimum W/C ratio should be less than 0.32. In addition. As Figure 5 depicts, a reduction in the 28 days elastic modulus of CAEM was observed as the A/C ratio increased. As the A/C ratio increased from 0.18 to 0.30, the 28 days elastic modulus decreased by 11.6 and 32.5%, respectively, which indicated that the higher content of asphalt emulsion, the lower 28 days elastic modulus of CAEM.
Microstructure Characterization
Microstructure Analysis
Figures 6A,B show the microstructure of CAEM at different curing time. From Figure 6A, it can be clearly observed that there was a large amount of needle shape crystals in the early hydration process, which filled the micro-pores of mixture and improved the strength of CAEM. In addition, the cement hydration consumed a portion of the water that occupied the micro air void spaces between asphalt emulsion and sand, which had a stiffening effect on CAEM (Fu et al., 2014). As CAEM continued to hydrate, C-S-H gels became the main binding phase in CAEM, the strength of hardened CAEM depended on the ability of the C–S–H to bind effectively other products of hydration and aggregates (Fu et al., 2014). It can be observed from Figure 6B that hydration products were interconnected in CAEM, resulting in the structure formed was much denser.
In order to observe the detailed information about the structure of crystalline substances, XRD was used in this study to obtain qualitatively phase composition of materials and identify crystalline phases of materials.
Figures 7A,B present X-ray diffractograms of the hydration products of CAEM after 2 h and 28 days curing time. From Figures 7A,B, it can be clearly observed that the hydration products of CAEM including calcium silicate hydrates (C-S-H gel), ettringite (AFt), 3CaO,⋅Al2O3,⋅CaSO4,⋅nH2O, (AFm), Ca(OH)2, (CH), and a small amount of unhydrated cement particles. The early hydration of CAEM was very fast, producing a large amount of ettringite (AFt). With the hydration continued, the amount of AFt in CAEM began to decrease. In contrast, the amount of C-S-H gels kept increasing.
Pore Structure Analysis
In order to determine the effect of W/C ratio and A/C ratio on the pore structure of the CAEM, MIP was used in this study to characterize the pore structure of the CAEM for 2 h samples and 28 days samples. The test results as shown in Figures 8, 9.
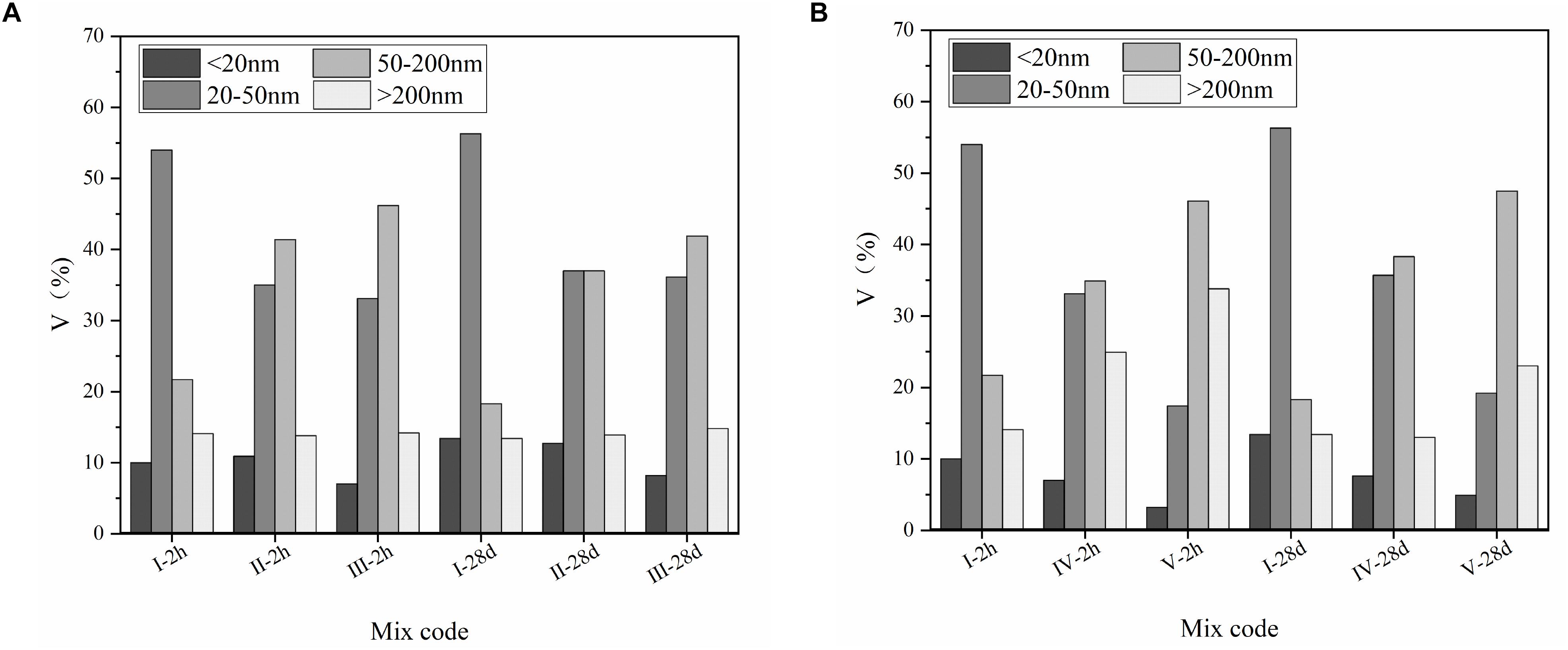
Figure 9. (A) Pore size distribution of the CAEM with various W/C ratios. (B) Pore size distribution of the CAEM with various A/C ratios.
Figure 8 presents the cumulative pore volume of CAEM with various W/C ratios and A/C ratios. From Figure 8, it can be seen that the cumulative volume of CAEM of 2 h samples and 28 days samples were similar, which indicated that the early hydration of CAEM was very fast at early-age and the hydration products were sufficient. It can also be seen from Figure 8 that the cumulative pore volume of CAEM increased with the increase of A/C ratio, possibly due to the asphalt emulsion resulted in the increase of the pore volume. With the increase of W/C, the cumulative pore volume of CAEM showed a similar law.
Figures 9A,B present the pore size distribution of the CAEM with various W/C ratios and A/C ratios. Wu (1988) proposed a classification of the pores into harmless pores (<20 nm), less harmful pores (20 ∼ 50 nm), harmful pores (50 ∼ 200 nm), and multiple harmful pores (>200 nm) according to the pore size, and pointed out that only the reduction of harmful pores and the addition of harmless pores could improve the mechanical properties and durability of concrete.
From Figure 9A, it can be concluded that the proportion of multiple harmful pores (>200 nm) of CAEM increased slightly with the increase of W/C ratio, indicating that excessive moisture provided a negative effect on the pore size distribution of CAEM. As can be seen from Figure 9B, the proportion of multiple harmful pores (>200 nm) of CAEM increased with the increase of A/C ratio, while the proportion of harmless pores (<20 nm) of CAEM decreased with the increase of A/C ratio. It can be explained that as the viscosity of CAEM increased with the increase of A/C, resulting in that it difficult for air bubbles to be discharged, which were introduced by CAEM during the process of mixing.
Conclusion
The high early-age strength and flowability of CAEM were studied in this paper, and evolution of microstructure of CAEM was analyzed by XRD, SEM, and MIP to reveal the hardening mechanism of CAEM. Based on the experimental results, the following conclusions can be drawn:
(1) The flowability of fresh CAEM is strongly affect by both A/C ratio and W/C ratio. The flowability of CAEM decreases with the increase of A/C ratio, while the flowability of CAEM increases with the increase of W/C ratio.
(2) The compressive strength of CAEM reaches 17–24 MPa after 6 h curing, which is almost 79–90% of the 28 days compressive strength of CAEM. The flexural strength of CAEM for 2 h samples can reach more than 4 MPa. The change trend of flexural strength is consistent to the compressive strength of CAEM, which indicate that increasing W/C ratio, or A/C ratio provide a negative effect on the mechanical properties.
(3) The smaller A/C value should be selected as much as possible in order to obtain high flowability of CAEM. As W/C increases, the flowability of CAEM increases, but the compressive strength of CAEM decreases, so the W/C value should be moderate in order to ensure the high early-age strength of CAEM.
(4) From SEM pictures of CAEM, it can be concluded that in the early period of CAEM hydration, the hydration products filled the micro pores of the mixture. And with the continued hydration, the hydration products were connected to each other, resulting in a denser structure. In addition, from MIP test results, it can be seen that the proportion of multiple harmful pores (>200 nm) of CAEM increases with the increase of W/C ratio or A/C ratio, indicating that increasing A/C ratio, or W/C ratio provide a negative effect on the pore size distribution of CAEM.
Data Availability Statement
The datasets generated for this study are available on request to the corresponding author.
Author Contributions
YT and WL conceived this project. XY, DL, ZW, JZ, and OX planned the experiments and carried out the experiments. YT and XY analyzed the data and wrote the manuscript. All the authors discussed the results and commented on the manuscript.
Funding
This work was supported by National Natural Science Foundation of China (51878065), the Fundamental Research Funds for the Central Universities, CHD (300102319201), Natural Science Foundation of Shaanxi Province of China (2017JM5030 and 2020JM-247), Western Transportation Construction Science and Technique Program (2013318J09230), and State Key Laboratory of High Performance Civil Engineering Materials (2018CEM010).
Conflict of Interest
The authors declare that the research was conducted in the absence of any commercial or financial relationships that could be construed as a potential conflict of interest.
References
Bołtryk, M., and Małaszkiewicz, D. (2013). Application of anionic asphalt emulsion as an admixture for concrete. Constr. Build. Mater. 40, 556–565. doi: 10.1016/j.conbuildmat.2012.11.110
Dołżycki, B., Jaczewski, M., and Szydłowski, C. (2017). The influence of binding agents on stiffness of mineral-cement-emulsion mixtures. Proc. Eng. 172, 239–246. doi: 10.1016/j.proeng.2017.02.103
Doyle, T. A., Mcnally, C., Gibney, A., and Tabakoviæ, A. (2013). Developing maturity methods for the assessment of cold-mix bituminous materials. Constr. Build. Mater. 38, 524–529. doi: 10.1016/j.conbuildmat.2012.09.008
Du, S. (2018). Effect of curing conditions on properties of cement asphalt emulsion mixture. Constr. Build. Mater. 164, 84–93. doi: 10.1016/j.conbuildmat.2017.12.179
Fang, X., Garcia, A., Winnefeld, F., Partl, M. N., and Lura, P. (2016). Impact of rapid-hardening cements on mechanical properties of cement bitumen emulsion asphalt. Mater. Struc. 49, 487–498. doi: 10.1617/s11527-014-0512-3
Fu, Q., Xie, Y., Zheng, K., Song, H., and Zhou, X. (2014). Influence of asphalt on mechanical properties of cement and asphalt mortar. Kuei Suan Jen Hsueh Pao J. Chin. Ceramic Soc. 42, 642–647. doi: 10.7521/j.issn.0454-5648.2014.05.14
Hu, S. G., Wang, T., Wang, F. Z., and Liu, Z. C. (2009). Adsorption behaviour between cement and asphalt emulsion in cement–asphalt mortar. Adv. Cement Res. 21, 11–14. doi: 10.1680/adcr.2007.00034
Li, W., Hong, J., Zhu, X., Yang, D., and Miao, C. (2018). Retardation mechanism of anionic asphalt emulsion on the hydration of Portland cement. Constr. Build. Mater. 163, 714–723. doi: 10.1016/j.conbuildmat.2017.12.150
Likitlersuang, S., and Chompoorat, T. (2016). Laboratory investigation of the performances of cement and fly ash modified asphalt concrete mixtures. Int. J. Pavement Res. Technol. 9, 337–344. doi: 10.1016/j.ijprt.2016.08.002
Lin, J., Wei, T., Hong, J., Zhao, Y., and Liu, J. (2015). Research on development mechanism of early-stage strength for cold recycled asphalt mixture using emulsion asphalt. Constr. Build. Mater. 99, 137–142. doi: 10.1016/j.conbuildmat.2015.09.019
Ling, C., Hanz, A., and Bahia, H. (2016). Measuring moisture susceptibility of Cold Mix Asphalt with a modified boiling test based on digital imaging. Constr. Build. Mater. 105, 391–399. doi: 10.1016/j.conbuildmat.2015.12.093
Liu, B., and Liang, D. (2017). Effect of mass ratio of asphalt to cement on the properties of cement modified asphalt emulsion mortar. Constr. Build. Mater. 134, 39–43. doi: 10.1016/j.conbuildmat.2016.12.137
Lu, C. T., Kuo, M. F., and Shen, D. H. (2009). Composition and reaction mechanism of cement–asphalt mastic. Constr. Build. Mater. 23, 2580–2585. doi: 10.1016/j.conbuildmat.2009.02.014
Ouyang, J., Han, B., Cao, Y., Zhou, W., Li, W., and Shah, S. P. (2016). The role and interaction of superplasticizer and emulsifier in fresh cement asphalt emulsion paste through rheology study. Constr. Build. Mater. 125, 643–653. doi: 10.1016/j.conbuildmat.2016.08.085
Ouyang, J., Hu, L., Yang, W., and Han, B. (2019a). Strength improvement additives for cement bitumen emulsion mixture. Constr. Build. Mater. 198, 456–464. doi: 10.1016/j.conbuildmat.2018.11.280
Ouyang, J., Pan, B., Xu, W., and Hu, L. (2019b). Effect of water content on volumetric and mechanical properties of cement bitumen emulsion mixture. J. Mater. Civil Eng. 31:04019085. doi: 10.1061/(asce)mt.1943-5533.0002736
Ouyang, J., and Shah, S. P. (2018). Factors influencing the structure build-up of fresh cement asphalt emulsion paste. Road Mater. Pavement Des. 19, 87–103. doi: 10.1080/14680629.2016.1236744
Ouyang, J., Yang, W., Chen, J., and Han, B. (2020). Effect of superplasticizer and wetting agent on pavement properties of cold recycled mixture with bitumen emulsion and cement. J. Mater. Civil Eng. 32:04020136. doi: 10.1061/(ASCE)MT.1943-5533.0003194
Ouyang, J., Zhao, J., and Tan, Y. (2018). Modeling mechanical properties of cement asphalt emulsion mortar with different asphalt to cement ratios and temperatures. J. Mater. Civil Eng. 30:04018263. doi: 10.1061/(ASCE)MT.1943-5533.0002480
Plank, J., and Hirsch, C. (2007). Impact of zeta potential of early cement hydration phases on superplasticizer adsorption. Cement Concr. Res. 37, 537–542. doi: 10.1016/j.cemconres.2007.01.007
Qiang, W., Peiyu, Y., Ruhan, A., Jinbo, Y., and Xiangming, K. (2011). Strength mechanism of cement-asphalt mortar. J. Mater. Civil Eng. 23, 1353–1359. doi: 10.1061/(asce)mt.1943-5533.0000301
Tan, Y., Ouyang, J., Lv, J., and Li, Y. (2013). Effect of emulsifier on cement hydration in cement asphalt mortar. Constr. Build. Mater. 47, 159–164. doi: 10.1016/j.conbuildmat.2013.04.044
Tian, Y., Jin, X. Y., Jin, N. G., Zhao, R., Li, Z. J., and Ma, H. Y. (2013). Research on the microstructure formation of polyacrylate latex modified mortars. Constr. Build. Mater. 47, 1381–1394. doi: 10.1016/j.conbuildmat.2013.06.016
Tyler, R., Wang, S., Shu, X., Huang, B., and Clarke, D. B. (2014). Laboratory investigation into mechanical properties of cement emulsified asphalt mortar. Constr. Build. Mater. 65, 76–83. doi: 10.1016/j.conbuildmat.2014.04.113
Wang, Y., Yuan, Q., Deng, D., Ye, T., and Fang, L. (2017). Measuring the pore structure of cement asphalt mortar by nuclear magnetic resonance. Constr. Build. Mater. 137, 450–458. doi: 10.1016/j.conbuildmat.2017.01.109
Wang, Z., Shu, X., Rutherford, T., Huang, B., and Clarke, D. (2015). Effects of asphalt emulsion on properties of fresh cement emulsified asphalt mortar. Constr. Build. Mater. 75, 25–30. doi: 10.1016/j.conbuildmat.2014.11.013
Yan, J., Leng, Z., Li, F., Zhu, H., and Bao, S. (2017). Early-age strength and long-term performance of asphalt emulsion cold recycled mixes with various cement contents. Constr. Build. Mater. 137, 153–159. doi: 10.1016/j.conbuildmat.2017.01.114
Zhong, S., and Chen, Z. (2002). Properties of latex blends and its modified cement mortars. Cem. Concr. Res. 32, 1515–1524. doi: 10.1016/S0008-8846(02)00813-X
Zhou, Y., Cai, J., Chen, R., Hou, D., Xu, J., Lv, K., et al. (2020a). The design and evaluation of a smart polymer-based fluids transport inhibitor. J. Clean. Prod. 257:120528. doi: 10.1016/j.jclepro.2020.120528
Zhou, Y., Cai, J., Hou, D., Chang, H., and Yu, J. (2020b). The inhibiting effect and mechanisms of smart polymers on the transport of fluids throughout nano-channels. Appl. Surf. Sci. 500:144019. doi: 10.1016/j.apsusc.2019.144019
Zhou, Y., Hou, D., Jiang, J., Liu, L., She, W., and Yu, J. (2018). Experimental and molecular dynamics studies on the transport and adsorption of chloride ions in the nano-pores of calcium silicate phase: the influence of calcium to silicate ratios. Microporous Mesoporous Materi. 255, 23–35. doi: 10.1016/j.micromeso.2017.07.024
Keywords: cement asphalt emulsion mixture, high early-age strength, high flowability, mechanism, microstructure characteruizations
Citation: Tian Y, Yan X, Lu D, Wang Z, Zhang J, Xu O and Li W (2020) Characteristics of the Cement Asphalt Emulsion Mixture With Early-Age Strength and Flowability. Front. Mater. 7:122. doi: 10.3389/fmats.2020.00122
Received: 13 February 2020; Accepted: 16 April 2020;
Published: 14 May 2020.
Edited by:
Dae-Wook Park, Kunsan National University, South KoreaReviewed by:
Yang Zhou, Southeast University, ChinaIonut Ovidiu Toma, Gheorghe Asachi Technical University of Iaşi, Romania
Copyright © 2020 Tian, Yan, Lu, Wang, Zhang, Xu and Li. This is an open-access article distributed under the terms of the Creative Commons Attribution License (CC BY). The use, distribution or reproduction in other forums is permitted, provided the original author(s) and the copyright owner(s) are credited and that the original publication in this journal is cited, in accordance with accepted academic practice. No use, distribution or reproduction is permitted which does not comply with these terms.
*Correspondence: Yaogang Tian, dGlhbmd1YW5nNzhAMTI2LmNvbQ==; Weiguang Li, eXhoNDA2Nzc5MTM2QDEyNi5jb20=