- 1Department of Nano Fusion Technology, Pusan National University, Busan, South Korea
- 2Department of Mechanical Engineering, Koje College, Geoje, South Korea
- 3School of Intelligent Automobile Engineering, Silla University, Busan, South Korea
- 4Department of Nanomechatronics Engineering, Pusan National University, Busan, South Korea
Diamond-like carbon (DLC) film doped with boron has unique properties and displays higher thermal resistance, lower internal stress, and better electrical conductivity than un-doped DLC film; this makes it is suitable for various applications, especially in outer space. Radio-frequency plasma-enhanced chemical vacuum deposition of boron-doped DLC film was performed to determine the optimal percentage of boron for improving thermal resistance. Additional heat treatment and 40 vol% B2H6/CH4 yielded the best electrical conductivity. X-ray photoelectron spectroscopy, thermal gravimetric analysis, Raman spectroscopy, and the four-point probe method were utilized to analyze the properties of boron-doped DLC film. The boron-doped DLC film displayed outstanding performance in terms of thermal resistance and electrical conductivity.
Introduction
Diamond-like carbon (DLC) has an amorphous crystalline structure and includes both sp3 (diamond-like) and sp2 (graphite-like) bonds. The ratio of these two types of bonds determine the crystalline properties and is sued to classify carbon materials (Lifshitz, 1999; Donnet and Erdemir, 2007). Conventional DLC has poor thermal resistance, leading to degradation of performance under high temperatures. At high temperatures, the sp3-bonds change to sp2-bonds, which influence the graphitization of DLC film (Robertson, 2002). DLC film is a metastable material under high stress. During heating and annealing, the structure of DLC film changes from diamond-like to graphite-like (Sánchez-López and Fernández, 2008). The thermal resistance of DLC films is usually poor, which limits their application in high temperature environments. The thermal resistance of DLC film is directly related to its physical characteristics and structure, chemical composition, and thickness. The addition of heterogeneous elements improves the overall properties of DLC films (Tallant et al., 1995). Research on boron-doped DLC films has mostly focused on their electrical properties. Usually, resistivity is measured in the range of 1 × 1012-1 × 1016 Ω/cm. Interestingly, the resistivity varies with the degree of deformation of the crystalline structure. It has been shown that doping with boron leads to graphitization (Kakiuchi et al., 2000), as well-improved electrical conductivity and reduced internal stress, in DLC films such as used Cu/Ti and OPT-DLC (Ahmad and Alsaad, 2007; Neeraj et al., 2012, 2013a). However, performance was not uniform among previous studies due to inconsistency in the deposition methods used. In space program, the utmost level of tribologic, thermal, and electrical characteristics have to require for long-time mission of solar cell's orientation, azimuth of antenna, gimbal adjustment of propulsions. The high performance of surface hardness but having conductivity to get rid of charging electrons in plasma circumstance on orbit is required, DLC treated electrical conductive, thermal resistive, and mechanical harness is expected the well-satisfied solution.
Experiment
The specimens in this study were (110) silicon wafers with dimensions of 20 mm (W) × 20 mm (L), as shown in Figure 1. First, ultrasonic cleaning was conducted for 30 min, using C3H6O (acetone), C2H5OH (ethanol), and deionized (DI) water, to remove impurities on the surface. Radio-frequency plasma-enhanced chemical vapor deposition RF-PECVD of the DLC film was performed. The inside wall was checked to scavenge negative remnant and the plasma sourced by argon (Ar) gas was sustained for 30 min, which induced a hot starting state, the operation of pre-cleaning gave the thermally stable circumstance in the chamber. The sample was pretreated to promote better adhesion on the surface of the DLC films, as shown in Table 1. Two gases, CH4 (methane) and B2H6 (diborane), were supplied under 0, 10, 20, 30, and 40 vol% conditions. The radio-frequency power supply was 300 W, the operation time was 60 min, and the pressure in the chamber was 2.0 × 10−2 Torr. The sample coatings conditions are shown in Table 2.
Results and Discussion
Boron was doped into DLC film on Si substrate using B2H6. The state of the doped boron in the DLC film was analyzed using X-ray photoelectron spectroscopy (XPS), as shown in Figure 2. Under 40 vol% B2H6/CH4, a peak of B1s was detected in the range 196.38–199.68 eV. However, the peak B1s intensity was weaker than that of other crystalline carbon materials, such as diamond; it is thought that B-C or B-H settle during the unstable bonding progress. During the deposition reaction of DLC in the plasma, C-C and C-H chains are more likely to react than B-C and B-H. The binding force of boron is greater than that of carbon, but under low-deposition temperature process conditions, there is only enough energy for weak binding. To measure the thickness of the DLC film, and to determine the growth rate of the films deposited on the substrate, the Stylus Profiler instrument (Bruker) was used to measure the average growth rate at regular intervals. The growth rate of the boron-doped film was 897.6 nm/h under B2H6, while the growth rate of the un-doped film was 799.6 nm/h. Thus, boron doping increased film thickness. Hardness was tested with the nano-indenter. The maximum Vickers hardness was 1,572 HV for the un-doped DLC films, which was higher than that of the doped DLC films (1,176.3 HV; Figure 3). Structural stress is related with sp2 fraction of carbon, increase of sp3/sp2 ratio determined improved hardness but reduced the electrical conductivity, strong bonding with nearest neighbor atoms of sp3 and less sp2 fractions shows high H values (Neeraj et al., 2011, 2013b). This result could be due to the aggregation of nanoclusters splitting sp2 and sp3, in turn affecting the crystalline structure of the DLC film and creating a boundary composed of sp2. Thus, doped boron reduced the influence of the sp3 chains. The Raman spectra showed an asymmetric peak at 1,100–1,700 cm−1, D-peak, diamond-like carbon marked on 1,320 cm−1, and G-peak, graphite-like marked on 1,540 cm−1 are distinguished with vertical lines as shown in Figure 4. As the amount of doped boron increased, the intensity ratio of the D and G peaks (ID/IG ratio) gradually increased; the full width at half maximum (FWHM) of the G peak also increased, as shown in Table 3 (Ferrari et al., 2002). In DLC films with an amorphous carbon structure, e.g., when diamond- and graphite-like networks are mixed together, boron controls the properties of the carbon network. Boron doping and heat treatment in DLC promote the formation of sp2 bonding. The active boron in doping progress interfere the normal combination of sp3 with the faster and lighter property in atomic characteristics and cause causes a split in the amorphous carbon structure, and the aggregation of sp3 induces a chain of sp2 carbon atoms. The change from an amorphous state to a pseudo-crystalline state, transited region between the amorphous formation and full crystal one, leads to yield the formation of graphite-like particles and to reduce the surface hardness. The sp3 bonds are fundamental to the properties of DLC films. In this study, the number of sp3 bonds in the DLC film was proportional to the hardness. High internal stress (in particular, compressive stress) in DLC films is associated with irregular sp3 bonds. The hardness of the DLC film also increases with increased internal stress and the results indicated that boron doping reduces internal stress in DLC films. A muffle furnace was utilized for heat treatment to evaluate the delamination of thin films when heated. As shown in Figure 5, the heating temperatures were 300, 350, and 400°C at a rate of 10°C per min with 15 min holding time. Then the specimens were let cooled at room conditions before removed from the furnace. Morphologies of the heat-treated samples were examined by using scanning electron microscope (SEM) as shown in Figure 6. All the thin films showed no changes or remained intact when heat-treated at 300°C. However, the undoped-DLC thin films delaminated when the heating temperature was raised to 350°C. On the other hand, 30 vol% boron-doped DLC thin films remained intact at all heating conditions. The un-doped and boron-doped DLC films (under 30 vol% B2H6) were subjected to thermal gravimetric analysis (TGA). Figure 7 shows the TGA instrument. The TGA curve of the DLC films was typical of single-stage decomposition. The TGA curve of un-doped boron DLC is shown in Figure 8A. Weight loss occurred at around 300°C, and continued as the temperature increased. The TGA curve of the 30 vol% boron-doped DLC films is shown in Figure 8B. Weight loss occurred at around 460°C. These results show the improved thermal resistance of the boron-doped DLC film. DLC films doped with 10 and 40 vol% boron exhibited shedding phenomenon and cracks were found on their surfaces. The DLC films doped with 20 vol% B2H6 showed partial cracking on the surface. The DLC films doped with 30 vol% B2H6 exhibited an intact surface under all thermal treatments. Among the boron-doped DLC films, the 30 vol%-boron-doped films showed the highest thermal resistance. The four-point probe method was used to determine the electrical conductivity of the boron-doped DLC films. The films doped with 40 vol% B2H6 had the highest electrical conductivity, as shown in Figure 9. Increasing the boron concentration improved the electrical conductivity and reduced sheet resistance. The DLC films doped with 30 vol% B2H6 demonstrated the worst performance, irrespective of the heat treatment applied; this was attributed to saturation of sp3 and an accumulation of free electrons due to the action of boron inside the carbon structure. An electronic transport model was used to evaluate the relationship between DLC film conductivity and temperature, as show below:
Where k is the Boltzmann constant, lnσDC ~1/T is a straight line, Eact is the activation energy, and Eact/k is the slope of the curve, showing the relationship between conductivity and temperature. The heat activation mechanism depends on the carriers generated in the expanded state, which are near the Fermi level. When excited by heat, electrons jump through the Fermi gap. It has been reported that DLC films have a network that promotes confinement, and thus accumulation, of electrons. However, when thermal energy is supplied, excited electrons may demonstrate tunneling behavior, as seen in semiconductors. It has also been reported that a weak p-type dopant can form in DLC films under certain conditions; therefore, it is possible to obtain p-type semiconducting DLC films by doping them with boron (Al Mamun et al., 2018). For n-type semiconductors, Eact = EC - EF, while for p-type semiconductors, Eact = EF - EV, where EF is the energy level, EC is the leading band mobility edge, and EV is the valence band mobility edge (Ma and Liu, 2001; Godet, 2002). The conductivity of DLC films depends on its sp2 bonds and the extent of doping. In this study, improved electrical conductivity of DLC films was achieved through boron doping, which enabled formation of a p-type dopant and an increase in sp2 bonds (Wei and Hing, 2002). The surface of DLC films was visualized under SEM and no significant difference was seen between the amorphous and pseudo-crystalline structure. Finally it was applied the TEM, Cs-corrected TEM (Themis Z, FEI, USA), locared in Korea Institute of Ceramic Engineering and Technology (KICET) for finding the cross-sectional substantial view (Takanori et al., 2009) of crystal or atomic structure of B-DLC where also includes carbon crystal of sp2 and sp3. The amorphous crystal of B-DLC on upper layer and obvious crystal of 110-oriented Si substrate on lower layer as shown on Figure 10. A deposition method was proposed, as shown in Figure 11, and the results indicated that boron-doped DLC film included C-C, C-H, B-C, and B-H chains and an amorphous carbon network; there were signs of electron tunneling and formation of a boundary area led to clustering formation. Additionally, some of the B2H6 and CH4 gas escaped under the low-temperature conditions, which resulted in the rare phenomenon of linkage of boron with C-C and C-H.
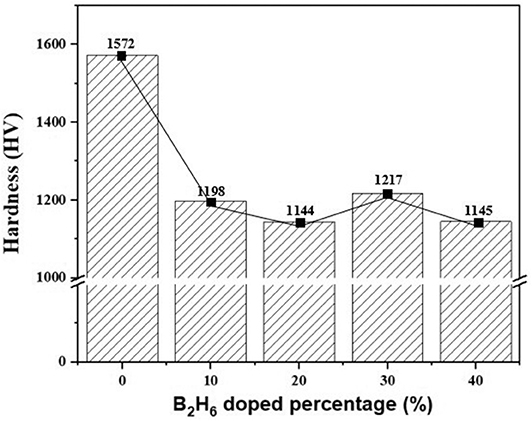
Figure 3. Vickers hardness of un-doped DLC films and boron doped DLC films with different B2H6 doping percentage.
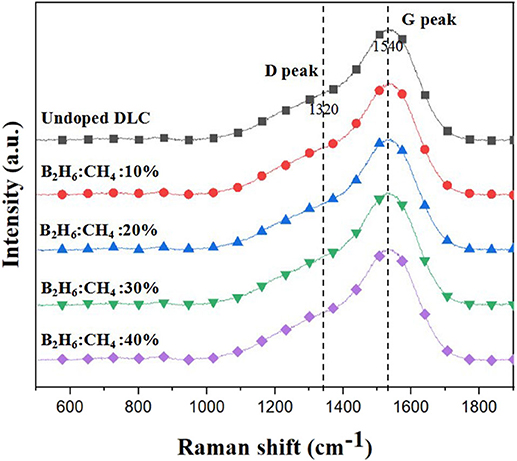
Figure 4. Raman spectra of un-doped DLC films and boron doped DLC films with different B2H6 doped percentage.
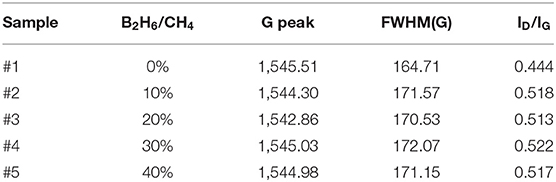
Table 3. Raman spectra results of the position of G peak, and FWHM of G peak and intensity ratio of the D peak and G peak.
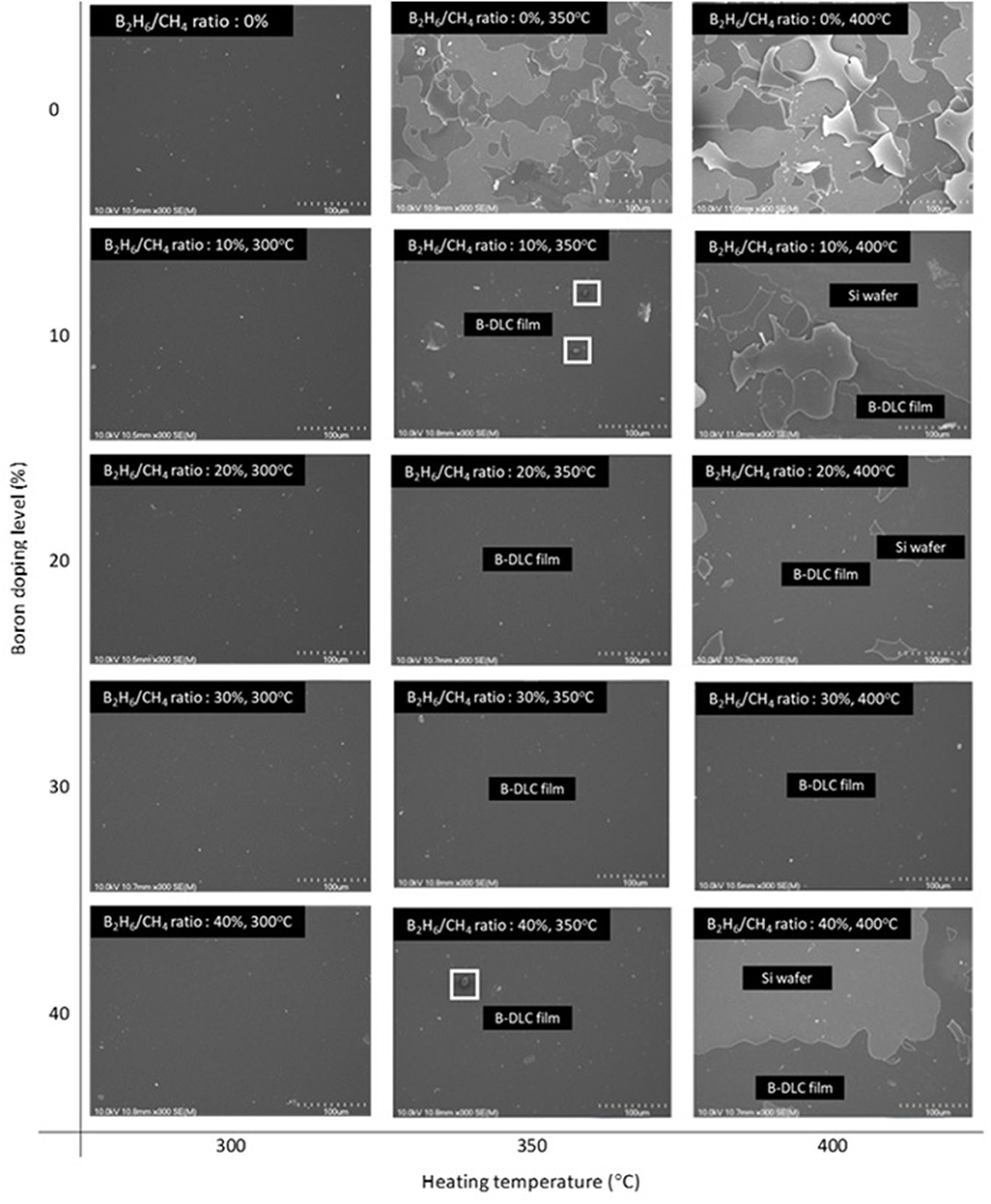
Figure 6. SEM images of thin films morphology and delamination after heat treated in muffle furnace.
Conclusion
A DLC film was deposited on Si substrate by RF-PECVD and born-doped using 10, 20, 30, or 40 vol% B2H6. The deposition rate of the boron-doped DLC film was higher than that of the un-doped DLC film. The hardness of, and amount of sp3 bonds in, the boron-doped DLC films were lower than those for the un-doped film. Boron doping reduced the internal stress due to a reduction in the sp3 network, which improved thermal resistance up to a temperature of 460°C, as shown by TGA; meanwhile, the 30 vol% boron doped DLC films exceled in all heating condition whereas the un-doped DLC film only showed thermal resistance up to 300°C. The un-doped DLC films had the worst electrical conductivity under all heat treatments whereby 40 vol% boron doped DLC films dramatically improved the electrical conductivity. It is possible to obtain p-type semiconducting DLC films by boron doping. As the temperature increased, more sp2 bonds developed, leading to an increase of clustering. This study shows that boron-doped DLC films may have applications in outer space-related projects.
Data Availability Statement
All datasets generated for this study are included in the article/supplementary material.
Author Contributions
WL, YP, and TK: contributed conception and design of the study. DS and DK: organized the database. WL, DS, and DK: performed the statistical analysis. WL and XT: wrote the first draft of the manuscript. WL and XT: wrote sections of the manuscript. All authors contributed to manuscript revision, read, and approved the submitted version.
Funding
This research was supported by Basic Science Research Program through the National Research Foundation of Korea (NRF) funded by the Ministry of Education (grant no. 2018R1D1A1B07046366).
Conflict of Interest
The authors declare that the research was conducted in the absence of any commercial or financial relationships that could be construed as a potential conflict of interest.
References
Ahmad, A. A., and Alsaad, A. M. (2007). Adhesive B-doped DLC films on biomedical alloys used for bone fixation. Bull. Mater. Sci. 30, 301–308. doi: 10.1007/s12034-007-0050-5
Al Mamun, M. A., Furuta, H., and Hatta, A. (2018). Pulsed DC plasma CVD system for the deposition of DLC films. Mater. Today Commun. 14, 40–46. doi: 10.1016/j.mtcomm.2017.12.008
Donnet, C., and Erdemir, A., (eds.). (2007). Tribology of Diamond-Like Carbon Films: Fundamentals and Applications. Boston, MA: Springer Science & Business Media. doi: 10.1007/978-0-387-49891-1
Ferrari, A. C., Rodii, S. E., Robertson, J., and Milne, W. I. (2002). Is stress necessary to stabilise sp3 bonding in diamond-like carbon? Diamond Relat. Mater. 11, 994–999. doi: 10.1016/S0925-9635(01)00705-1
Godet, C. (2002). Variable range hopping revisited: the case of an exponential distribution of localized states. J. Non-Crystal. Solids 299, 333–338. doi: 10.1016/S0022-3093(01)01008-0
Kakiuchi, H., Kobayashi, T., and Terai, T. (2000): Property change of diamond-like carbon thin films due to ion implantation. Nuclear Instruments Methods Phys. Res. Section B: Beam Interact. Mater. Atoms 166, 415–419. doi: 10.1016/S0168-583X(99)01056-3
Lifshitz, Y. (1999). Diamond-like carbon—present status. Diamond Related Mater. 8, 1659–1676. doi: 10.1016/S0925-9635(99)00087-4
Ma, Z. Q., and Liu, B. X. (2001). Boron-doped diamond-like amorphous carbon as photovoltaic films in solar cell. Solar Energy Mater. Solar Cells 69, 339–344. doi: 10.1016/S0927-0248(00)00400-1
Neeraj, D., Kumar, S., and Malik, H. (2013b). Strange hardness characteristic of hydrogenated diamond-like carbon thin film by plasma enhanced chemical vapor deposition process Appl. Phys. Lett.102:011917. doi: 10.1063/1.4775372
Neeraj, D., Kumar, S., and Malik, H. K. (2013a). Role of base pressure on the structural and nano-mechanical properties of metal/diamond-like carbon bilayers. Appl. Surface Sci. 274, 282–287. doi: 10.1016/j.apsusc.2013.03.037
Neeraj, D., Kumar, S., Malik, H. K., Govind, Rauthan, C. M. S., and Panwar, O. S. (2011). Correlation of sp3 and sp2 fraction of carbon with electrical, optical and nano-mechanical properties of argon-diluted diamond-like carbon films Appl. Surface Sci. 257, 6804–6810. doi: 10.1016/j.apsusc.2011.02.134
Neeraj, D., Kumar, S., Singh, S., and Malik, H. K. (2012). Oxygen modified diamond-like carbon as window layer for amorphous silicon solar cells. Solar Energy 86, 220–230. doi: 10.1016/j.solener.2011.09.025
Robertson, J. (2002). Diamond-like amorphous carbon. Mater. Sci. Eng. R: Rep. 37, 129–281. doi: 10.1016/S0927-796X(02)00005-0
Sánchez-López, J. C., and Fernández, A. (2008). Doping and Alloying Effects on DLC Coatings. Tribology of Diamond-Like Carbon Films. (Boston, MA: Springer), 311–338. doi: 10.1007/978-0-387-49891-1_12
Takanori, T., Komiyama, T., Miki, H., Takagi, T., and Aoyama, T. (2009). XPS and TEM study of W-DLC/DLC double-layered film Thin Solid Films 517, 5010–5013. doi: 10.1016/j.tsf.2009.03.033
Tallant, D. R., Parmeter, J. E., Siegal, M. P., and Simpson, R. L. (1995). The thermal stability of diamond-like carbon. Diamond Rel. Mater. 4, 191–199. doi: 10.1016/0925-9635(94)00243-6
Keywords: DLC, boron-doped, RF-PECVD, thermal treatment, electrical resistance
Citation: Li W, Tan XY, Park YM, Shin DC, Kim DW and Kim TG (2020) Improved Thermal Resistance and Electrical Conductivity of a Boron-Doped DLC Film Using RF-PECVD. Front. Mater. 7:201. doi: 10.3389/fmats.2020.00201
Received: 17 February 2020; Accepted: 02 June 2020;
Published: 07 July 2020.
Edited by:
Ram Gupta, Pittsburg State University, United StatesReviewed by:
Hitendra Kumar Malik, Indian Institute of Technology Delhi, IndiaHo Won Jang, Seoul National University, South Korea
Copyright © 2020 Li, Tan, Park, Shin, Kim and Kim. This is an open-access article distributed under the terms of the Creative Commons Attribution License (CC BY). The use, distribution or reproduction in other forums is permitted, provided the original author(s) and the copyright owner(s) are credited and that the original publication in this journal is cited, in accordance with accepted academic practice. No use, distribution or reproduction is permitted which does not comply with these terms.
*Correspondence: Tae Gyu Kim, tgkim@pusan.ac.kr