- 1School of Physics and Mechanical & Electrical Engineering, Hubei Engineering Technology Research Center of Environmental Purification Materials, Hubei University of Education, Wuhan, China
- 2State Key Laboratory for Modification of Chemical Fibers and Polymer Materials, College of Materials Science and Engineering, Donghua University, Shanghai, China
- 3Faculty of Science, Engineering and Technology, Swinburne University of Technology, Hawthorn, Victoria, Australia
- 4School of Chemical and Biomedical Engineering, Nanyang Technological University, Singapore, Singapore
Copper chalcogenides have been the subject of extensive research as promising candidates in thermoelectric conversion applications due to their advantageous properties, environmental compatibility, and abundance. The low intrinsic thermal conductivity and high electric conductivity of these materials are born out of the “phonon-liquid electron-crystal” structure between the copper and chalcogens. In this review, strategies for thermoelectric property improvement of copper chalcogenides are discussed. The challenges and the future development of binary copper chalcogenides for further thermoelectric conversion applications are outlined.
Introduction
Solid-state thermoelectric (TE) technology, using the Seebeck effect and Peltier effect, can convert directly and simply between heat and electricity (Figure 1A) (Wei et al., 2018). The dimensionless TE figure of merit (zT) is the key metric used to evaluate the performance of TE materials: zT = S2σT/κ. Where S, σ, κ, and T are Seebeck coefficient, electrical conductivity, thermal conductivity, and absolute temperature, respectively (Tan et al., 2016). The earliest studies in TE technology are derived from the 19th century (Shi et al., 2016). Copper sulfides was reported as a promising TE material in 1827. However, there were two main problems unsolved in practical application, which are the stable composition of Cu2S in reheating and contact between the Cu2S and the electrode in the TE generator. When the “phonon-liquid electron-crystal” (PLEC) concept was proposed in 2012, the low thermal conductivity and high TE performance of copper sulfides and copper selenides have been explained. In this review, we discuss the strategies for TE property improvement of copper chalcogenides based on reducing lattice thermal conductivity of single-component material and tuning compositions for optimizing TE parameters. Challenges for binary copper chalcogenides and perspectives on the flexible copper chalcogenides are outlined.
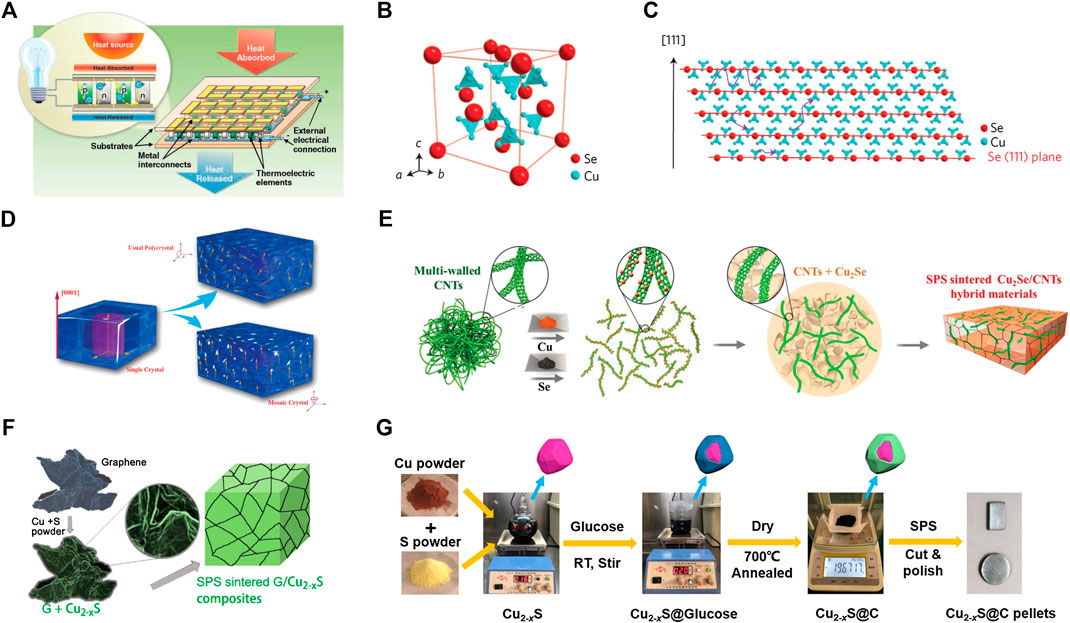
Figure 1. (A) A thermoelectric generator module and heat drives (inset). Reproduced with permission (Service, 2004). Copyright 2004, American Association for the Advancement of Science. (B) Unit cell of cubic Cu2Se. (C) Projected plane representation of the crystal structure along the cubic [1 −1 0] direction. The arrows indicate that the Cu ions can freely travel among the interstitial sites. Reproduced with permission (Liu et al., 2012). Copyright 2012, Nature Publication Group. (D) Structural characteristics of a single crystal, a usual polycrystal, and a mosaic crystal. Arrows refer to alignment of crystal grains. Reproduced with permission (He et al., 2015a). Copyright 2015, Wiley-VCH. (E) Fabrication process and formation mechanism of the Cu2Se/CNT hybrid materials. Reproduced with permission (Nunna et al., 2017). Copyright 2017, The Royal Society of Chemistry. (F) Process for fabricating G/Cu2-xS composite. Reproduced with permission (Tang et al., 2018). Copyright 2018, Elsevier. (G) Process for fabricating carbon-encapsulated Cu2−xS composite. Reproduced with permission (Chen et al., 2018). Copyright 2018, The Royal Society of Chemistry.
Strategies for Thermoelectric Property Improvement
The fundamental challenge of designing high-performance TE materials stems from the strong correlation of S, σ, and κ, which requires large S and σ values, as well as a low κ value simultaneously (Zhou et al., 2018). However, these three parameters are strongly interdependent through carrier concentration (n). Among three parameters, κ comprises the electronic thermal conductivity (κe) and the lattice thermal conductivity (κL) (κ = κe+κL). κL, calculated by κL = 1/3CVvl, in which CV is heat capacity, v is speed of sound, and l is mean free path of phonons, is the only one with little dependence on n. Therefore, κL can be minimized through the independent crystal structure and/or microstructure design. This is one strategy for TE property improvement. The other strategy, tuning the composition, such as foreign elements introducing, metal chalcogenides mixing, hybrid compounds building, strongly relates to the n and carrier mobility (μ). Below we will briefly elaborate on these two strategies.
Reducing Lattice Thermal Conductivity of Single Component Material
Reducing κL can rely on decreasing the mean free path of lattice phonons and eliminating some of the vibrational modes. For example, face-centered cubic (fcc) copper selenide, as a superionic conductor, has two different sub-lattices inside its crystal structure. The sub-lattice frame of Se atoms provides a pathway for the movement of semiconducting electrons, and the highly disordered Cu ions move through the Se lattice frame with “liquid-like mobility” and high diffusivities, which explained as the “PLEC” concept (Figures 1B and 1C) (Qiu et al., 2016; Chen et al., 2018). In addition, the liquid-like mobile Cu ions also introduce extra scattering to lattice phonons to further disrupt heat propagation or alter phonon transfer, and thereby shortening phonon mean free path and suppressing κL.
Now, research on the PLEC Cu-based superionic conductors has become a topic that is very popular in the TE community. A low κL around 0.4–0.6 W m−1 K−1 was reported at a high-temperature of 1000 K for Cu2-xSe, which leads to an enhanced TE performance (zT = 1.5) (Liu et al., 2012). This high zT value results from the coexistence of Se and Cu sub-lattices inside the crystal structure of the Cu2-xSe phase. Introducing nanostructure for defects creation, an effective method to enhance the low-to-high wavelength phonon scattering, results in a very small value of κ. Cu2Se with this low κ (0.34 Wm−1K−1) reached a high zT of 2.1 at 973 K (Gahtori et al., 2015).
Copper sulfide and copper telluride are superionic conductors at high-temperatures as well. Thus, they correlate well with the PLEC character that has been observed in Cu2Se. It is reported that extremely low values of κL below 0.35 W m−1 K−1 and high values of zT of 1.7 at 1000 K for Cu2−xS with a slight copper deficiency (He et al., 2014). Copper telluride has an extremely high κe, therefore the κ is also quite high compared with that in copper selenide and copper sulfide. For example, the value of κ = 2 W m−1 K−1 at room temperature and reduced to 1 W m−1 K−1 by increasing the annealing temperatures of samples, and the best zT value is 1.1 at 1000 K (He et al., 2015b).
Tuning Compositions for Optimizing Thermoelectric Parameters
Tuning the composition, without the intrinsic restriction from monomers, more depends on the n and μ to optimizing three TE parameters S, σ, and κ. Firstly, foreign elements introducing by the fabrication process can generate vacancies in material structures which increase the hole concentration at cation positions or electron concentration at cation positions. Furthermore, foreign elements serve as point-defect scattering sources for phonons. These impurity atoms create both force differences (strain field fluctuations) and mass contrast (mass fluctuations) between the host atoms and the impurity atoms and are very effective for scattering high-frequency (short wavelength) phonons. Thus foreign elements, like Na, Ag, I, and Al, can affect TE properties by changing the degree of Cu deficiency. For example, Cu2Se1-xIx presents a zT value of 2.3 near room temperature as a result of critical scattering, which simultaneously leads to a high S and high κe (Liu et al., 2013).
Another attractive way is the mixture of homogeneous metal chalcogenides, which not only maintains the PLEC cubic structure of copper chalcogenides but also creates a unique composite structure. He et al. reported a mosaic crystal of mixed Cu2S-Cu2Te bulk nanomaterials, which achieves a zT value of 2.1 at 1000 K (He et al., 2015a). In this unique microstructure, electrons are freely transferred along with the frames of quasi-single crystals while phonons are strongly scattered by lattice strains or interfaces of mosaic nanograins (Figure 1D). In the Ag2Te-Cu2Te mixed structure, the high n in pristine Cu2Te is substantially reduced to an optimal range, resulting in much-reduced κ and enhanced power factors over the entire temperature range. Furthermore, the temperature of the last phase transition is significantly lowered when increasing Ag content, which leads to a zT of 1.8 is achieved at 1000 K in Cu2Te + 50% Ag2Te (Zhao et al., 2019).
Recently, the introduction of different forms of carbon (such as carbon nanotubes, graphene, and carbon encapsulation) into the copper chalcogenide bulks has achieved significant enhancement of TE properties. Carbon brings to high charge μ into a composite structure (Zhu et al., 2019), which benefits the κe but increases the κL as well. The introduction of a certain concentration of carbon in composite materials is the key to improve the TE properties. Cu2Se and multi-walled carbon nanotube (CNTs) hybrid materials, with a high degree of homogeneously dispersed molecular CNTs inside the Cu2Se matrix, possess greatly improved zT of 2.4 at 1000 K for Cu2Se/0.75 wt% CNTs, which is about 30% higher than that of the pristine Cu2Se (Figure 1E) (Nunna et al., 2017). Cu2−xS matrix and three-dimensional graphene heterointerface composites have created more interfaces between Cu2−xS and graphene leading to low energy carrier scattering, which can increase the S and cut down the κ. The zT value of 1.56 at 873 K is in the Cu2−xS with 0.75 wt% graphene (Figure 1F) (Tang et al., 2018). Carbon-encapsulated Cu2−xS composites have a 50% improvement of the κe and power factor than that of the pristine Cu2−xS. The phase transition from orthorhombic to tetragonal of these composites demonstrated the lower κL. A zT value of 1.04 has been achieved at 773 K in the 0.25 wt% carbon-encapsulated Cu2−xS composites (Figure 1G) (Chen et al., 2019).
Challenges for Binary Copper Chalcogenides
Cu ions may migrate from their initial locations to places where electrical potential or chemical potential is low, thus the stabilities of the copper chalcogenides system still worth discussion (Ge et al., 2016). In Dennler’s experiments, it showed that Cu2S is not electrically stable because both cracks and copper whiskers were observed in the sample after extended current stress testing (Figure 2A) (Dennler et al., 2014). Qiu et al. revealed the mechanism for atom migration and deposition in Cu-S/Se materials based on a critical chemical potential difference (Qiu et al., 2018). Constructing a series of electronically conducting but ion-blocking barriers to reset the chemical potential of such conductors to keep it below the threshold for decomposition can remain good stability under high electric currents and/or large temperature differences (Mao et al., 2019a). For example, high zT and large critical voltage can be decoupled through doping immobile ions into the liquid-like sublattice (Mao et al., 2019b). In recent reports, the Cu2Se/Yb0.3Co4Sb12 TE module shows good stability and high energy conversion efficiency of 9.1% (Figure 2B) (Qiu et al., 2019).
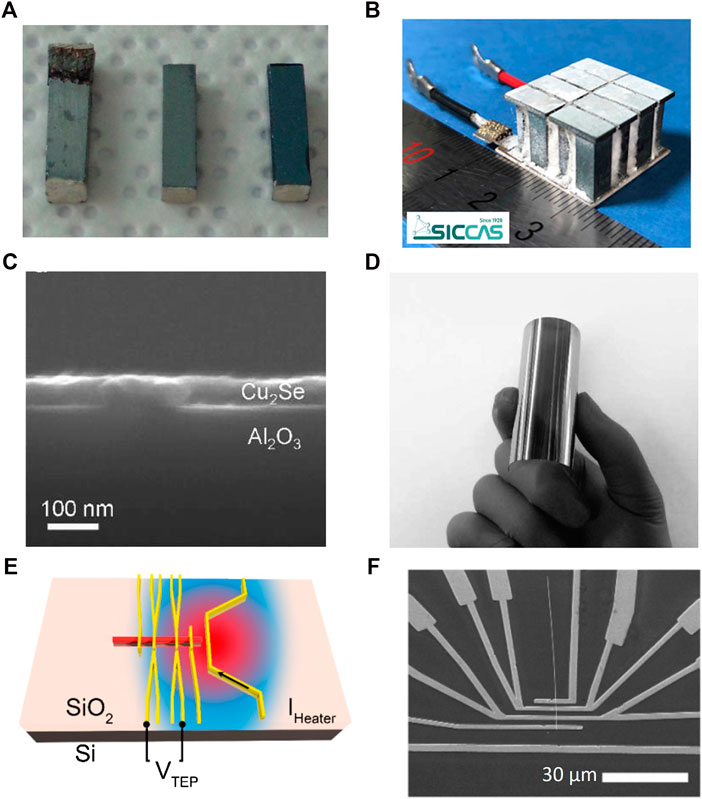
Figure 2. (A) Cu2S leg at a current density of 24 A cm−2 for 24 h and Cu1.8S and CuS legs both after a stress of 48 A cm−2 for 72 h (from the left to right). Reproduced with permission (Dennler et al., 2014) Copyright 2014, Wiley-VCH. (B) Optical image of the eight-couple Cu2Se/Yb0.3Co4Sb12 TE module. Reproduced with permission (Qiu et al., 2019) Copyright 2019, Elsevier Inc. (C) Cross-sectional scanning electron microscope (SEM) image of the Cu2Se film on Al2O3 substrate. (D) Photograph of the Cu2Se thin film on the polyimide substrate. Reproduced with permission (Lin et al., 2017). Copyright 2017, Wiley-VCH. (E) The illustration for the TE properties measurement for CdS/Cu2S superlattice nanowire. (F) SEM image of the fabricated device for thermopower measurement. Reproduced with permission (Xiong et al., 2017). Copyright 2017, American Chemical Society.
Flexible or thin-film TE materials can make up for application limitations from bulk materials, especially for light and portable devices. Several attempts have been used in the fabrication of flexible or thin-film copper chalcogenide structures. Typically, Cu2Se powder and the organic solution mixed making an ink solution, which was covered on flexible substrates evenly (Figures 2C and D). The Cu2Se thin film fabricated by this route exhibits a power factor of 0.62 mW cm−1 K−2 at 684 K (Lin et al., 2017). Besides, CdS/Cu2S superlattice nanowires shown the enhanced TE performance. For measurement, CdS/Cu2S superlattice nanowires electrically connected to two electrodes that also serve as the temperature sensors. With a temperature gradient (ΔT) created by a DC current over a nearby heating electrode, a thermal voltage can be generated along the nanowire and the thermopower can be calculated (Figures 2E and F) (Xiong et al., 2017).
Conclusions and Perspectives
Significant developments and progresses have been achieved in TE performance of binary copper chalcogenides. PLEC concept opens up a new situation to explain the low κ in copper chalcogenide superlattice structure. Furthermore, tuning compositions optimizes n leading to improved TE parameters. The main challenges for copper chalcogenide TE materials include the electrical and thermal stabilities during high-temperature measurement and the application limitations of the bulk modulus. It is hoped that binary copper chalcogenides will be widely applied in TE generators and device applications.
Author Contributions
All authors listed have made a substantial, direct, and intellectual contribution to the work, and approved it for publication.
Conflict of Interest
The authors declare that the research was conducted in the absence of any commercial or financial relationships that could be construed as a potential conflict of interest.
Acknowledgments
The authors thank the National Natural Science Foundation of China (51702091, 51702046); the College Outstanding Young Scientific and Technological Innovation Team of Hubei province (T201922); and the Special Funding of Preventing the spread of COVID-19, Hubei University of Education (20XGZX20).
References
Chen, X., Yang, J., Wu, T., Li, L., Luo, W., Jiang, W., et al. (2018). Nanostructured binary copper chalcogenides: synthesis strategies and common applications. Nanoscale 10(32), 15130–15163. doi:10.1039/c8nr05558k
Chen, X., Zhang, H., Zhao, Y., Liu, W.-D., Dai, W., Wu, T., et al. (2019). Carbon-encapsulated copper sulfide leading to enhanced thermoelectric properties. ACS Appl. Mater. Interfaces. 11, 22457–22463. doi:10.1021/acsami.9b06212
Dennler, G., Chmielowski, R., Jacob, S., Capet, F., Roussel, P., Zastrow, S., et al. (2014). Are binary copper sulfides/selenides really new and promising thermoelectric materials? Adv. Energy Mater. 4(9), 1301581. doi:10.1002/aenm.201301581
Gahtori, B., Bathula, S., Tyagi, K., Jayasimhadri, M., Srivastava, A. K., Singh, S., et al. (2015). Giant enhancement in thermoelectric performance of copper selenide by incorporation of different nanoscale dimensional defect features. Nano Energy. 13, 36–46. doi:10.1016/j.nanoen.2015.02.008
Ge, Z.-H., Zhao, L.-D., Wu, D., Liu, X., Zhang, B.-P., Li, J.-F., et al. (2016). Low-cost, abundant binary sulfides as promising thermoelectric materials. Mater. Today. 19(4), 227–239. doi:10.1016/j.mattod.2015.10.004
He, Y., Day, T., Zhang, T., Liu, H., Shi, X., Chen, L., et al. (2014). High thermoelectric performance in non-toxic earth-abundant copper sulfide. Adv. Mater. 26(23), 3974–3978. doi:10.1002/adma.201400515
He, Y., Lu, P., Shi, X., Xu, F., Zhang, T., Snyder, G. J., et al. (2015a). Ultrahigh thermoelectric performance in mosaic crystals. Adv. Mater. 27(24), 3639–3644. doi:10.1002/adma.201501030
He, Y., Zhang, T., Shi, X., Wei, S.-H., and Chen, L. (2015b). High thermoelectric performance in copper telluride. NPG Asia Mater. 7(8), e210. doi:10.1038/am.2015.91
Lin, Z., Hollar, C., Kang, J. S., Yin, A., Wang, Y., Shiu, H.-Y., et al. (2017). A solution processable high-performance thermoelectric copper selenide thin film. Adv. Mater. 29(21), 1606662. doi:10.1002/adma.201606662
Liu, H., Shi, X., Xu, F., Zhang, L., Zhang, W., Chen, L., et al. (2012). Copper ion liquid-like thermoelectrics. Nature Mater. 11(5), 422–425. doi:10.1038/nmat3273
Liu, H., Yuan, X., Lu, P., Shi, X., Xu, F., He, Y., et al. (2013). Ultrahigh thermoelectric performance by electron and phonon critical scattering in Cu2Se1-xIx. Adv. Mater. 25(45), 6607–6612. doi:10.1002/adma.201302660
Mao, T., Qiu, P., Du, X., Hu, P., Zhao, K., Xiao, J., et al. (2019a). Enhanced thermoelectric performance and service stability of Cu 2 Se via tailoring chemical compositions at multiple atomic positions. Adv. Funct. Mater. 30(6), 1908315. doi:10.1002/adfm.201908315
Mao, T., Qiu, P., Hu, P., Du, X., Zhao, K., Wei, T. R., et al. (2019b). Decoupling thermoelectric performance and stability in liquid‐like thermoelectric materials. Adv. Sci. 7(1), 1901598. doi:10.1002/advs.201901598
Nunna, R., Qiu, P., Yin, M., Chen, H., Hanus, R., Song, Q., et al. (2017). Ultrahigh thermoelectric performance in Cu2Se-based hybrid materials with highly dispersed molecular CNTs. Energy Environ. Sci. 10, 1928–1935. doi:10.1039/c7ee01737e
Qiu, P., Agne, M. T., Liu, Y., Zhu, Y., Chen, H., Mao, T., et al. (2018). Suppression of atom motion and metal deposition in mixed ionic electronic conductors. Nat. Commun. 9(1), 2910. doi:10.1038/s41467-018-05248-8
Qiu, P., Mao, T., Huang, Z., Xia, X., Liao, J., Agne, M. T., et al. (2019). High-efficiency and stable thermoelectric module based on liquid-like materials. Joule 3(6), 1538–1548. doi:10.1016/j.joule.2019.04.010
Qiu, P., Shi, X., and Chen, L. (2016). Cu-based thermoelectric materials. Energy Storage Mater. 3, 85–97. doi:10.1016/j.ensm.2016.01.009
Service, R. F. (2004). THERMOELECTRICS: temperature rises for devices that turn heat into electricity. Science 306, 806–807. doi:10.1126/science.306.5697.806
Shi, X., Chen, L., and Uher, C. (2016). Recent advances in high-performance bulk thermoelectric materials. Int. Mater. Rev. 61(6), 379–415. doi:10.1080/09506608.2016.1183075
Tan, G., Zhao, L.-D., and Kanatzidis, M. G. (2016). Rationally designing high-performance bulk thermoelectric materials. Chem. Rev. 116(19), 12123–12149. doi:10.1021/acs.chemrev.6b00255
Tang, H., Sun, F.-H., Dong, J.-F., Asfandiyar, Zhuang, H.-L., Pan, Y., et al. (2018). Graphene network in copper sulfide leading to enhanced thermoelectric properties and thermal stability. Nano Energy. 49, 267–273. doi:10.1016/j.nanoen.2018.04.058
Wei, T.-R., Qin, Y., Deng, T., Song, Q., Jiang, B., Liu, R., et al. (2018). Copper chalcogenide thermoelectric materials. Sci. China Mater. 62(1), 8–24. doi:10.1007/s40843-018-9314-5
Xiong, Z., Cai, Y., Ren, X., Cao, B., Liu, J., Huo, Z., et al. (2017). Solution-processed CdS/Cu2S superlattice nanowire with enhanced thermoelectric property. ACS Appl. Mater. Interfaces. 9(38), 32424–32429. doi:10.1021/acsami.7b09346
Zhao, K., Liu, K., Yue, Z., Wang, Y., Song, Q., Li, J., et al. (2019). Are Cu 2 Te‐based compounds excellent thermoelectric materials? Adv. Mater. 31(49), 1903480. doi:10.1002/adma.201903480
Zhou, X., Yan, Y., Lu, X., Zhu, H., Han, X., Chen, G., et al. (2018). Routes for high-performance thermoelectric materials. Mater. Today. 21(9), 974–988. doi:10.1016/j.mattod.2018.03.039
Keywords: thermoelectric property, nanostructure, thermal conductivity, carrier concentration, flexible materials
Citation: Chen X, Yang J, Katkus T, Wu T, Tao J, Li J, Wang C, Wang X and Dai W (2020) Exploring Thermoelectric Property Improvement for Binary Copper Chalcogenides. Front. Mater. 7:589568. doi: 10.3389/fmats.2020.589568
Received: 04 August 2020; Accepted: 06 October 2020;
Published: 28 October 2020.
Edited by:
Peter Reiss, Commissariat à l'Energie Atomique et aux Energies Alternatives (CEA), FranceReviewed by:
Pengfei Qiu, Shanghai Institute of Ceramics (CAS), ChinaAndreu Cabot, Institut de Recerca de l'Energia de Catalunya, Spain
Copyright © 2020 Chen, Yang, Katkus, Wu, Tao, Li, Wang, Wang and Dai. This is an open-access article distributed under the terms of the Creative Commons Attribution License (CC BY). The use, distribution or reproduction in other forums is permitted, provided the original author(s) and the copyright owner(s) are credited and that the original publication in this journal is cited, in accordance with accepted academic practice. No use, distribution or reproduction is permitted which does not comply with these terms.
*Correspondence: Jianping Yang, amlhbnBpbmd5YW5nQGRodS5lZHUuY24= Wei Dai, ZGFpd2VpQGh1ZS5lZHUuY24=