- 1State Key Laboratory of Advanced Technology for Materials Synthesis and Processing, School of Materials Science and Engineering, Wuhan University of Technology, Wuhan, China
- 2Hoffmann Institute of Advanced Materials, Shenzhen Polytechnic, Shenzhen, China
- 3State Key Laboratory of Luminescent Materials and Devices, Institute of Polymer Optoelectronic Materials and Devices, South China University of Technology, Guangzhou, China
- 4Department of Sports Medicine and Rehabilitation, Peking University Shenzhen Hospital, Shenzhen, China
Flexible perovskite solar cells (FPSCs) have shown great potential in the field of wearable power supply and integration with architectures in the future due to their advantages of high flexibility, light weight, portability, and compatibility with irregular electronic products. As a promising photovoltaic technology compatible with roll-to-roll manufacturing process, FPSCs have made significant progress in the past several years through composition engineering, interface modification, optimization of fabrication process, and exploitation of new charge transport materials. As a result, the light-to-electricity power conversion efficiency of FPSCs has exceeded 20% recently. In this mini review, the latest developments of FPSCs are systematically summarized and discussed, including the flexible substrates and electrodes, as well as the fabrication of high-quality perovskite films. Finally, a prospect on the massive manufacturing of FPSCs as well as the challenge is also discussed.
Introduction
With the overconsumption of fossil fuels and environmental pollution becoming increasingly serious, solar energy has now become one of the main energy supply approaches due to its clean, safe, and widely distributed characteristics (Jung et al., 2020; Zhang et al., 2020; Qi et al., 2020a). So far, various solar energy technologies have been developed and utilized, such as solar heating (Wang et al., 2019a), photovoltaics (Hu et al., 2020a), photocatalysis (Li U. et al., 2020), and solar architecture (Wang et al., 2019b). Among all of them, photovoltaic technologies, directly converting solar energy into electricity, have attracted more widespread attention and research interest. Besides the widely used silicon-based solar cells, diverse other types of solar cells have also been developed, including CdTe-based solar cells (Chen et al., 2020), GaAs-based solar cells (Zhao et al., 2019), dye-sensitized solar cells (Kim Y. J. et al., 2020), and solution-processable solar cells such as organic solar cells and perovskite solar cells (PSCs) (Hu et al., 2020a; Ge et al., 2020; Hu et al., 2020b). Over the past several years, numerous efforts have been devoted toward the research and development of PSCs due to their high light-to-electricity efficiency and low-cost fabrication, which emerges as a promising candidate for large-area commercialization (Hu et al., 2021; Ng et al., 2018; Hu H. et al., 2019).
In 2009, Miyasaka and coworkers first reported the application of perovskite materials in solar cells with a PCE of 3.81% (Kojima et al., 2009). Recently, the highest certified power conversion efficiency (PCE) of PSCs has reached 25.5%, which successfully demonstrated the photovoltaic performance of PSCs is comparable with the monosilicon solar cells in the commercialized market. This achievement is also beneficial for the development of FPSCs, which can be designed to be fabricated with different shapes and applied to wearable devices, portable devices, and building integrated photovoltaics. In the past years, significant progress has been obtained for FPSCs with a maximum reported PCE up to 20.21%. However, there are some technical challenges for FPSCs, including the low temperature for processing, instability caused by repeated mechanical bending, and so on. In this mini review, we systematically evaluate the latest research progress on flexible perovskite solar cells, including the flexible substrates, preparation of electrodes, and fabrication of high-quality perovskite films. Meanwhile, we also discuss the stretchability of FPSCs and the challenges of large-scale manufacturing. At the end, we provide the prospects for the development of FPSCs in the future.
Substrate and Electrode of Flexible Perovskite Solar Cells
The substrate, which plays a critical role in the flexible solar cells, not only affects the final photovoltaic performance of the device but also influences the mechanical stability (Jung et al., 2019). Herein, we summarize several requirements for the flexible substrates of FPSCs, as follows. 1) Good optical properties: the substrate should be optically transparent to absorb light especially visible-light region as much as possible. 2) High conductivity: the photovoltaic performance, including the fill factor and photocurrent density, is directly related to this charge-collecting layers. 3) Good barrier properties: significant degradation of performance always happens in most electronic devices because the substrate is vulnerable to oxygen or moisture. The substrate should be a barrier layer to avoid the penetration of oxygen and moisture to maintain a long-term stable performance. 4) Good chemical properties: the substrates should be chemically stable because they are exposed to many chemicals such as gases or solvents during the fabrication process. 5) Excellent mechanical properties: flexible substrates should comply with deformable transformation under severe stress and strain and effectively release stress without losing their original functions (Jung et al., 2019; Yang et al., 2018). The substrate for FPSCs can be divided into three categories: ultrathin flexible glass substrates, metal substrates (Heo et al., 2018; Troughton et al., 2015) (Ti, copper foil, and stainless-steel foil), and plastic substrates (Li M. et al., 2020; Ahmad et al., 2020) (polyethylene naphthalate (PEN) or polyethylene terephthalate (PET)). Flexible glass substrates with a thickness of several hundred micrometers can fulfill most of the requirements of the flexible substrate, as described above. The high temperature tolerance of up to over 600 °C, good chemical stability and conductivity, and perfect gas barrier properties are very attractive. However, fragility, high weight, and cost could be big concerns, compared with other candidates. The metal substrate has excellent thermal stability, charge conductivity, and corrosion resistance, but it is intrinsically opaque, which causes the loss of optical absorption, leading to a decrease of PCE (Nejand et al., 2017). On the contrary, the plastic substrate has the advantages of high optical transparency, excellent flexibility, low cost, and chemical stability. However, the main problem of plastic substrate is the limited processing tolerance temperature (below 150 °C) (You et al., 2020) and the poor gas barrier properties for oxygen and moisture. Therefore, all processes should be performed at a relatively low temperature. FPSCs prepared with plastic substrates have shown great development potential in flexible electronic devices. Selection of electrodes is also very important, and it should have the characteristics of high mechanical flexibility, good durability, high conductivity, transparency, and low sheet resistance (Li et al., 2018). Figure 1 shows the development of FPSCs in recent years. So far, indium tin oxide (ITO) (Kim et al., 2018), graphene (Luo et al., 2018), polymer (Hu et al., 2019a), and silver nanowires (Ag-NWs) (Lee et al., 2018) have been used as flexible electrodes of PSCs (summarized in Table 1). Najafi et al. fabricated highly durable FPSCs on flexible substrate PEN/ITO, where NiOx and ZnO were employed as the hole transport layer (HTL) and electron transport layer (ETL), respectively. Three cations-based perovskite films were prepared by one-step method, yielding the PCE of 16.6% (Najafi et al., 2018). Li et al. proposed a novel low-temperature approach to achieve high performance flexible planar PSCs for implementation on ITO/PEN substrate and a triple cation perovskite (Cs0.05(MA0.17FA0.83)0.95Pb(I0.85Br0.15)3), which boosts the PCE of FPSCs up to 17% (Li et al., 2019). Nevertheless, the mechanical robustness of ITO is poor, making it difficult to meet the requirements for ideal FPSCs. To overcome this problem, some organic molecules or polymer scaffolds are successfully adopted as structure to improve the interfacial contact. Wang et al. fabricated highly efficient and robust FPSCs on ITO/PEN flexible substrate. Firstly, thiourea is introduced into the perovskite precursor solution to modulate crystal growth and form a dense and uniform perovskite thin film. The bilayer interface is comprised of poly(3,4-ethylenedioxythiophene):poly(styrenesulfonate) (PEDOT:PSS)/poly (triaryl amine) (PTAA), which has a distinct offset in the highest occupied molecular orbital (HOMO) levels, enabling markedly enhanced charge extraction and spectral response. The PCE and a record fill factor of the FPSCs is 19.41% and 81%, respectively (Wang et al., 2020). In the work of Meng et al., PEDOT:poly(ethylene-co-vinyl acetate) (EVA) ink was prepared by a miniemulsion method; the obtained film yields good cohesion with the substrate because of the adhesive EVA counterpart and acts as HTL between perovskite films and ITO substrate, which simultaneously facilitates the perpendicular crystallization of perovskite on the flexible substrate and makes brittle ITO and perovskite adhere compactly and improves flexibility. The PCE of the FPSCs was 19.87% and 17.55% on the effective area of 1.01 cm2 and 31.2 cm2, respectively, and the PCE retention efficiency was more than 85% after 7,000 bending cycles (Meng et al., 2020a). Unfortunately, the shortage of indium could retard the rapid energy payback from perovskites. Therefore, more and more progresses have been made to use low-cost and high flexible electrode materials (such as carbon nanotubes, graphene, and Ag-NWs) to replace ITO layers. Zhou et al. fabricated an ultra-low-cost HTM-free C-PSCs with the structure of Ag-NWs-graphene (Ag-NWs-G)/SnO2/perovskite/carbon by using 1D-2D hybrid Ag-NWs-G transparent electrode instead of conventional ITO electrode. The device exhibited a decent PCE of 15.31% and long-term stability of 87.5% for 60 days in air conditions (Zhou et al., 2020). Tran and coworkers synthesized large-scale monolayer graphene directly on polyethersulfone (PES) substrate by chemical vapor deposition (CVD) at 150°C as the bottom electrode, a highly transparent aluminum-doped zinc oxide (AZO)/Ag/AZO multilayer was utilized as the top electrode, and a smooth and dense perovskite (MAPbI3) film was prepared by the one-step method. The semitransparent FPSCs show the highest PCE of ∼14.2%, maintaining over 90% of the initial PCE value after 1,000 bending cycles with a tensile strain of 1.5% (equivalent radii is 4 mm) (Tran et al., 2019).
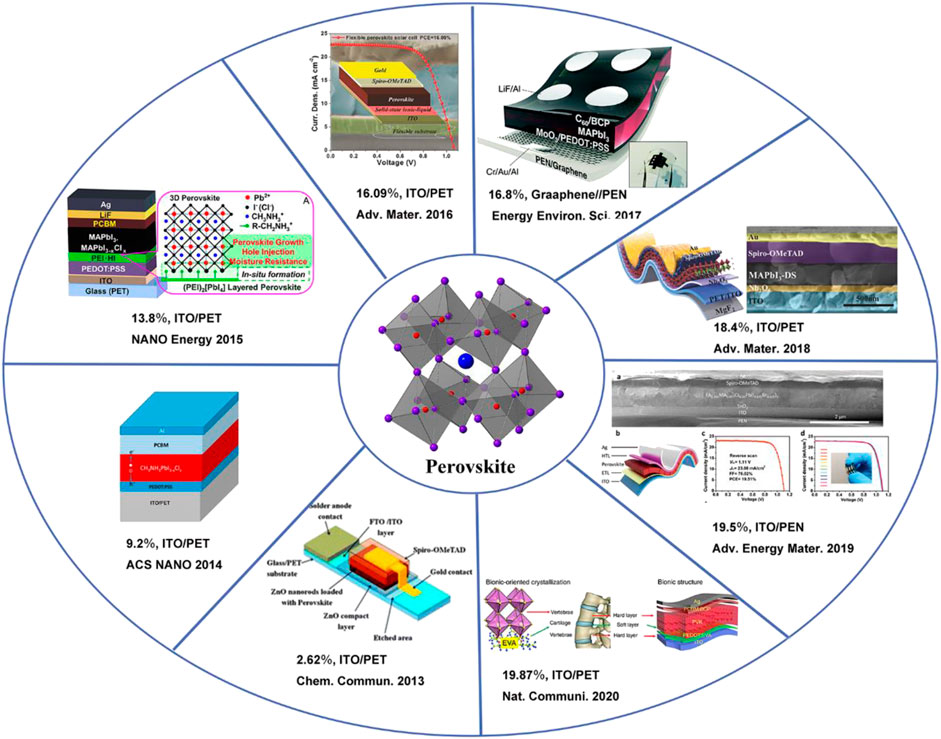
FIGURE 1. Illustration of the development for FPSCs from 2013 to 2020. This image gives the efficiency and electrode for flexible perovskite devices.
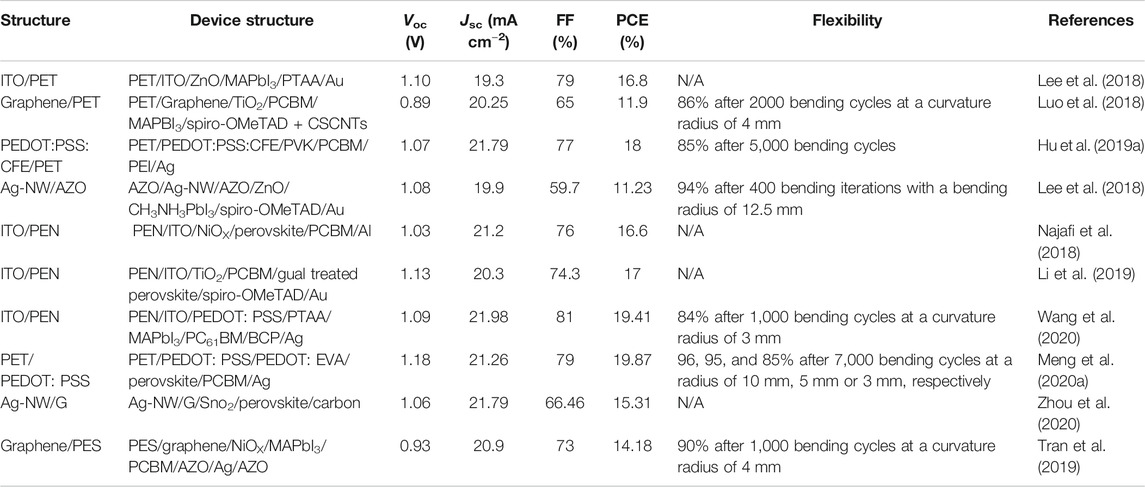
TABLE 1. Summary of the photovoltaic and flexibility performance of FPSCs fabricated on plastic/electrode substrates.
High-Quality Flexible Perovskite Film
High-performance FPSCs demand high-quality perovskite thin films with uniform morphology, full surface coverage, and high crystallinity. The perovskite thin films have been demonstrated with excellent absorption: a film about 300 nm thick is sufficient to absorb essentially all visible light above its band-gap (Wang et al., 2016). Moreover, all fabrication procedures can be conducted below 150°C, which is favorable for the preparation of high-efficiency FPSCs (summarized in Table 2). Deng and coworkers simply replaced the widely-used antisolvents with MABr with the dynamic spinning of perovskite precursor. It significantly reduced the formation energy of α-phase Formamidinium (FA) perovskites at 40°C. The optimized annealing temperature of 100°C provides FA-based perovskite films with high-quality morphology and crystallization. The resultant FPSCs exhibited the PCE of 18.5% and remained over 80% of the initial PCE after 1,200 bending cycles (Deng et al., 2020). Kim et al. fabricated all-printed PSCs on flexible substrates by gravure-printing, where an MAPbI3 film prepared by one-step method showed excellent surface morphology and the optimized devices displayed a PCE up to 17.2%. In addition, based on the two-step manufacturing of the perovskite photo-active layer, the efficiency of partial roll-to-roll processing of PSCs reached 9.7% (Kim et al., 2019). Lee et al. (2019) fabricated crack-free perovskite (MAPbI3) films on ultra-thin PET substrates (∼2.5 μm) with the aid of poly(dimethylsiloxane) (PDMS) supports. The FPSCs showed high efficiency of 17.03% and maintained as much as 88% of its initial efficiency after 100 cycles of crumpling (Lee et al., 2019).
The grain boundary of the perovskite is an important factor for the loss of PSCs efficiency and environmental stability, since charge carrier recombination and ionic migration of perovskite primarily occur at the grain boundaries. Furthermore, when the mechanical displacement is applied to the perovskite film, cracking occurs at the grain boundary, which makes the crack itself difficult to recover, resulting in low mechanical stability (Meng et al., 2020b). To overcome these issues, additives and interface engineering techniques have been applied to improve the crystallization quality of flexible perovskite films. Feng et al. reported an effective dimethyl sulfide (DS) additive technique developed to control the perovskite (MAPbI3) morphology on flexible substrates for FPSCs, yielding the PCE up to 18.40% (Feng et al., 2018). Duan et al. constructed a novel autonomously longitudinal scaffold by the interspersion in situ self-polymerization of methyl methacrylate (sMMA) in PbI2, in which perovskite solution can be confined within the scaffold network for crystallization with more effective nucleation sites. Moreover, sMMA oligomers can further polymerize and fill in the grain boundaries of an sMMA-interspersed MAPbI3 (sMMA-MAPbI3) film to form a cross-linking network capable of passivating defects, releasing mechanical stress, and impeding ions migration. The planar FPSCs based on sMMA-MAPbI3 exhibited superior photovoltaic performance (PCE of 20.12%) with remarkable output stability (72% of initial PCE after 5,000 bending cycles) (Duan et al., 2020). Dai et al. fabricated a large-area, high-efficiency flexible perovskite solar module by blade-coating on ITO-coated Willow glass. Ammonium chloride (NH4Cl) was added to the perovskite precursor solution to retard the nucleation of perovskite, leading to an improved film morphology. Taking the advantages of NH4Cl, the assembled single-junction FPSCs demonstrated an efficiency of 19.72% and 15.86% for small-area cells and large-area modules (42.9 cm2), respectively (Dai et al., 2020).
The stretchability of flexible perovskite films is also important as wearable electronic devices to fulfill the requirements of diverse applications. Currently, only fewer studies have reported the stretchability. Meng et al. incorporated a self-healing polyurethane (s-PU) with dynamic oxime-carbamate bonds as a scaffold into the perovskite films, which simultaneously improved crystallinity and passivated the grain boundary of the perovskite films. The stretchable PSCs with s-PU deliver a stabilized efficiency of 19.15% and recover 88% of their original efficiency after 1,000 cycles at 20% stretch (Meng et al., 2020). Hu et al. prepared a high-quality perovskite film, showing an elastic “brick-and-mortar” structure via a biomimetic-crystallization approach. Due to the existence of the lower nucleation free energy barrier, the insoluble poly(styrene-co-butadiene) (SBS) scaffold provided fewer nucleation sites but enhanced heterogeneous nucleation. Moreover, the interaction between PbX2 (X = I and Br) and the soluble polyurethane (PU) can slow down the crystallization rate of a high-quality perovskite film. The prepared FPSCs delivered a PCE of 15.01%. Importantly, a 56.02 cm2 area wearable PSCs device (7.91% PCE) has been successfully demonstrated with essential reproducibility and mechanical flexibility (Hu et al., 2020). Qi et al. designed a novel island-chain structure by laser engraving stretchable serpentine interconnect on PET/ITO flexible substrate. The stretchable PSCs exhibited a PEC of 17.68%. Furthermore, the PCE of the stretchable PSCs remains at 87% from the initial value even after stretching for 300 cycles at the largest ratio (80%) (Qi et al., 2020b). These latest reports show that flexible and stretchable PSCs have made a significant breakthrough in the field of wearable power supplies. Kim and coworkers demonstrated roll-to-roll-processed FPSCs via a three-step process including gravure-printing, antisolvent bathing, and annealing. They prepared highly crystalline, uniform-formamidinium- (FA-) based perovskite via tBuOH: EA bathing. FAPbI3-based perovskite layer was conducted by gravure printing and the best PCE of 19.1% was obtained for FPSCs. In addition, except for the top electrode, the full roll-to-roll gravure printing of FPSCs has been demonstrated on a pilot scale, and a 100-m-long roll can be produced through process optimization. The highest PCE of 16.7% was reported for roll-to-roll-processed PSCs, while 13.8% for fully roll-to-roll-produced PSCs (Kim Y. Y et al., 2020). This report provides a significant breakthrough for the large-scale production of FPSCs with high production and low cost.
Challenges and Prospective
FPSCs have attracted extensive research with remarkable progress within the past years. The PCE of FPSCs has exceeded 20%, indicating a bright future of FPSCs. Despite the notable improvement in the efficiency and flexibility of FPSCs, manufacturing of FPSCs via a low-cost and massive-fabrication method is still challenging. For example, traditional flexible perovskite devices are still fabricated on ITO/PET or PEN substrates, but the rigidity of ITO can cause cracks in the thin film, leading to the failure of the device after repeated bending. Efforts have been made to apply polymer-based interfacial layers or low-cost carbon and organic composite materials to overcome these cracking problems. Meanwhile, perovskite layer also plays a critical role in determining the performance of FPSCs. A high-quality perovskite film with good morphology, full coverage, suitable band-gap, and highly orientated crystalline structure is important for the development of highly efficient FPSCs. At present, different methods have been reported to optimize the properties of perovskite films, such as compositional engineering of perovskite, additive engineering, and interface/grain-boundary passivation techniques.
So far, almost all the reported FPSCs with excellent PCE are limited within small areas; when the area of the device increases, the PCE decreases due to the inevitable loss of homogeneity in the films. Thus, it is of great importance to develop manufacturing technology for large-area PSCs without affecting the device efficiency. The roll-to-roll fabrication technique is one of the most promising approaches to realize its commercial applications, while the in-depth analysis should also be systematically conducted to speed up this process. Besides the PCE of FPSCs, it is necessary to pay more attention to the mechanical-photovoltaic loss of FPSCs. For instance, for wearable device applications, how to achieve the requirements of stretchability to adapt to complex body movements still needs more studies. Many efforts are still needed to facilitate the application of FPSCs including the low-cost massive manufacturing and long-term stability. Considering the development in the past years, it is reasonable to believe the application of FPSCs has a bright future.
Author Contributions
HH, QZ, and XZ conceived the idea and reviewed the manuscript. XL wrote the manuscript. CG, QF, SD, WD, YZ, and HL were involved in the manuscript discussion and correction.
Conflict of Interest
The authors declare that the research was conducted in the absence of any commercial or financial relationships that could be construed as a potential conflict of interest.
The reviewer XZ declared a shared affiliation, with no collaboration, with one of the authors HH to the handling editor at the time of the review.
Acknowledgments
The financial support from the National Natural Science Foundation of China (62004129, 22005202, 51472189, 52002301, and 62004069) is gratefully acknowledged, and this work was also supported by Shenzhen Polytechnic.
References
Ahmad, T., Wilk, B., Radicchi, E., Fuentes Pineda, R., Spinelli, P., Herterich, J., et al. (2020). New fullerene derivative as an n‐type material for highly efficient, flexible perovskite solar cells of a p‐i‐n configuration. Adv. Funct. Mater. 30 (45), 2004357. doi:10.1002/adfm.202004357
Chen, X., Shen, X., Shen, S., Reese, M. O., and Hu, S. (2020). Stable CdTe photoanodes with energetics matching those of a coating intermediate band. ACS Energ. Lett. 5 (6), 1865. doi:10.1021/acsenergylett.0c00603
Dai, X., Deng, Y., Van Brackle, C. H., Chen, S., Rudd, P. N., Xiao, X., et al. (2020). Scalable fabrication of efficient perovskite solar modules on flexible glass substrates. Adv. Energ. Mater. 10 (1), 1903108. doi:10.1002/aenm.201903108
Deng, W., Li, F., Li, J., Wang, M., Hu, Y., and Liu, M. (2020). Anti-solvent free fabrication of FA-Based perovskite at low temperature towards to high performance flexible perovskite solar cells. Nano Energy 70, 104505. doi:10.1016/j.nanoen.2020.104505
Duan, X., Li, X., Tan, L., Huang, Z., Yang, J., Liu, G., et al. (2020). Controlling crystal growth via an autonomously longitudinal scaffold for planar perovskite solar cells. Adv. Mater. 32 (26), 2000617. doi:10.1002/adma.202000617
Feng, J., Zhu, X., Yang, Z., Zhang, X., Niu, J., Wang, Z., et al. (2018). Record efficiency stable flexible perovskite solar cell using effective additive assistant strategy. Adv. Mater. 30 (35), 1801418. doi:10.1002/adma.201801418
Ge, C., Xue, Y., Li, L., Tang, B., and Hu, H. (2020). Recent progress in 2D/3D multidimensional metal halide perovskites solar cells. Front. Mater. 7, 601179. doi:10.3389/fmats.2020.601179
Heo, J. H., Shin, D. H., Lee, M. L., Kang, M. G., and Im, S. H. (2018). Efficient organic–inorganic hybrid flexible perovskite solar cells prepared by lamination of polytriarylamine/CH3NH3PbI3/anodized Ti metal substrate and graphene/PDMS transparent electrode substrate. ACS Appl. Mater. Inter. 10 (37), 31413–31421. doi:10.1021/acsami.8b11411
Hu, H., Qin, M., Fong, P. W. K., Ren, Z., Wan, X., Singh, M., et al. (2020a). Perovskite quantum wells formation mechanism for stable efficient perovskite photovoltaics-A real‐time phase‐transition study. Adv. Mater. 33, 2006238. doi:10.1002/adma.202006238
Hu, H., Singh, M., Wan, X., Tang, J., Chu, C.-W., and Li, G. (2020b). Nucleation and crystal growth control for scalable solution-processed organic-inorganic hybrid perovskite solar cells. J. Mater. Chem. A. 8, 1578. doi:10.1039/c9ta11245f
Hu, R., Ge, C., Chu, L., Feng, Y., Xiao, S., Ma, Y., et al. (2021). Novel photoelectric material of perovskite-like (CH3)3SPbI3 nanorod arrays with high stability. J. Energ. Chem. 59, 581–588. doi:10.1016/j.jechem.2020.12.003
Hu, X., Huang, Z., Li, F., Su, M., Huang, Z., Zhao, Z., et al. (2019b). Nacre-inspired crystallization and elastic “brick-and-mortar” structure for a wearable perovskite solar module. Energy Environ. Sci. 12 (3), 979–987. doi:10.1039/c8ee01799a
Hu, X., Meng, X., Zhang, L., Zhang, Y., Cai, Z., Huang, Z., et al. (2019a). A mechanically robust conducting polymer network electrode for efficient flexible perovskite solar cells. Joule 3 (9), 2205. doi:10.1016/j.joule.2019.06.011
Hu, H., , Ren, Z., Fong, P. W. K., Qin, M., Liu, D., Lei, D., et al. (2019). Room-temperature meniscus coating of >20% perovskite solar cells: a film formation mechanism investigation. Adv. Funct. Mater. 29, 1900092. doi:10.1002/adfm.201900092
Hu, R., , Zhang, Y., Paek, S., Gao, X.-X., Li, X. A., and Nazeeruddin, M. K. (2020). Enhanced stability of α-phase FAPbI3 perovskite solar cells by insertion of 2D (PEA)2PbI4 nanosheets. J. Mater. Chem. A. 8, 8058–8064. doi:10.1039/c9ta14207j
Jung, H. S., Han, G. S., Park, N.-G., and Ko, M. J. (2019). Flexible perovskite solar cells. Joule 3, 1850–1880. doi:10.1016/j.joule.2019.07.023
Jung, S. K., Heo, J. H., Oh, B. M., Lee, J. B., Park, S. H., Yoon, W., et al. (2020). Chiral stereoisomer engineering of electron transporting materials for efficient and stable perovskite solar cells. Adv. Funct. Mater. 30 (13), 1905951. doi:10.1002/adfm.201905951
Kim, J.-H., Seok, H.-J., Seo, H.-J., Seong, T.-Y., Heo, J. H., Lim, S.-H., et al. (2018). Flexible ITO films with atomically flat surfaces for high performance flexible perovskite solar cells. Nanoscale 10 (44), 20587. doi:10.1039/c8nr06586a
Kim, Y. Y., Yang, T. Y., Suhonen, R., Välimäki, M., Maaninen, T., Kemppainen, A., et al. (2019). Gravure‐printed flexible perovskite solar cells: toward roll‐to‐roll manufacturing. Adv. Sci. 6 (7), 1802094. doi:10.1002/advs.201802094
Kim, Y. J., , Choi, H., Kim, C. S., Lee, G., Park, J., Park, S. E., et al. (2020). Dye-sensitized solar cell-thermoelectric hybrid generator utilizing bipolar conduction in a unified element. ACS Appl. Energ. Mater. 3 (5), 4155. doi:10.1021/acsaem.0c00513
Kim, Y. Y., , Yang, T. Y., Suhonen, R., Kemppainen, A., Hwang, K., Jeon, N. J., et al. (2020). Roll-to-roll gravure-printed flexible perovskite solar cells using eco-friendly antisolvent bathing with wide processing window. Nat. Commun. 11 (1), 1. doi:10.1038/s41467-020-18940-5
Kojima, A., Teshima, K., Shirai, Y., and Miyasaka, T. (2009). Organometal halide perovskites as visible-light sensitizers for photovoltaic cells. J. Am. Chem. Soc. 131 (17), 6050. doi:10.1021/ja809598r
Lee, E., Ahn, J., Kwon, H.-C., Ma, S., Kim, K., Yun, S., et al. (2018). All-solution-processed silver nanowire window electrode-based flexible perovskite solar cells enabled with amorphous metal oxide protection. Adv. Energ. Mater. 8 (9), 1702182. doi:10.1002/aenm.201702182
Lee, G., Kim, M.-c., Choi, Y. W., Ahn, N., Jang, J., Yoon, J., et al. (2019). Ultra-flexible perovskite solar cells with crumpling durability: toward a wearable power source. Energ. Environ. Sci. 12 (10), 3182. doi:10.1039/c9ee01944h
Li, J., Han, G., Vergeer, K., Dewi, H. A., Wang, H., Mhaisalkar, S., et al. (2019). Interlayer engineering for flexible large-area planar perovskite solar cells. ACS Appl. Energ. Mater. 3 (1), 777. doi:10.1021/acsaem.9b01924
Li, L., Zhang, S., Yang, Z., Berthold, E. E. S., and Chen, W. (2018). Recent advances of flexible perovskite solar cells. J. Energ. Chem. 27 (3), 673. doi:10.1016/j.jechem.2018.01.003
Li, M., , Zuo, W. W., Ricciardulli, A. G., Yang, Y. G., Liu, Y. H., Wang, Q., et al. (2020). Embedded nickel‐mesh transparent electrodes for highly efficient and mechanically stable flexible perovskite photovoltaics: toward a portable mobile energy source. Adv. Mater. 32 (38), 2003422. doi:10.1002/adma.202003422
Li, Y., , Li, B., Zhang, D., Cheng, L., and Xiang, Q. (2020). Crystalline carbon nitride supported copper single atoms for photocatalytic CO2 reduction with nearly 100% CO selectivity. ACS nano 14 (8), 10552. doi:10.1021/acsnano.0c04544
Luo, Q., Ma, H., Hou, Q., Li, Y., Ren, J., Dai, X., et al. (2018). All‐carbon‐electrode‐based endurable flexible perovskite solar cells. Adv. Funct. Mater. 28 (11), 1706777. doi:10.1002/adfm.201706777
Meng, X., Cai, Z., Zhang, Y., Hu, X., Xing, Z., Huang, Z., et al. (2020a). Bio-inspired vertebral design for scalable and flexible perovskite solar cells. Nat. Commun. 11 (1), 1. doi:10.1038/s41467-020-16831-3
Meng, X., Xing, Z., Hu, X., Huang, Z., Hu, T., Tan, L., et al. (2020b). Stretchable perovskite solar cells with recoverable performance. Angew. Chem. Int. Ed. 59, 16602. doi:10.1002/anie.202003813
Najafi, M., Di Giacomo, F., Zhang, D., Shanmugam, S., Senes, A., Verhees, W., et al. (2018). Highly efficient and stable flexible perovskite solar cells with metal oxides nanoparticle charge extraction layers. Small 14 (12), 1702775. doi:10.1002/smll.201702775
Nejand, A. B., Nazari, P., Gharibzadeh, S., Ahmadi, V., and Moshaii, A. (2017). All-inorganic large-area low-cost and durable flexible perovskite solar cells using copper foil as a substrate. Chem. Commun. (Camb) 53 (4), 747. doi:10.1039/c6cc07573h
Ng, A., Ren, Z., Hu, H., Fong, P. W. K., Shen, Q., Cheung, S. H., et al. (2018). A cryogenic process for antisolvent‐free high‐performance perovskite solar cells. Adv. Mater. 30, 1804402. doi:10.1002/adma.201804402
Qi, J., Chen, S., Lan, C., Wang, A. C., Cui, X., You, Z., et al. (2020a). Large‐grained perovskite films enabled by one‐step meniscus‐assisted solution printing of cross‐aligned conductive nanowires for biodegradable flexible solar cells. Adv. Energ. Mater. 10 (35), 2001185. doi:10.1002/aenm.202001185
Qi, J., Xiong, H., Hou, C., Zhang, Q., Li, Y., and Wang, H. (2020b). A kirigami-inspired island-chain design for wearable moistureproof perovskite solar cells with high stretchability and performance stability. Nanoscale 12 (6), 3646. doi:10.1039/c9nr10691j
Tran, V.-D., Pammi, S. V. N., Park, B.-J., Han, Y., Jeon, C., and Yoon, S.-G. (2019). Transfer-free graphene electrodes for super-flexible and semi-transparent perovskite solar cells fabricated under ambient air. Nano Energy 65, 104018. doi:10.1016/j.nanoen.2019.104018
Troughton, J., Bryant, D., Wojciechowski, K., Carnie, M. J., Snaith, H., Worsley, D. A., et al. (2015). Highly efficient, flexible, indium-free perovskite solar cells employing metallic substrates. J. Mater. Chem. A. 3, 9141–9145. doi:10.1039/c5ta01755f
Wang, X., Li, X., Liu, G., Li, J., Hu, X., Xu, N., et al. (2019a). An interfacial solar heating assisted liquid sorbent atmospheric water generator. Angew. Chem. Int. Ed. 58 (35), 12054. doi:10.1002/anie.201905229
Wang, X., Zhu, K., Jing, X., Wang, Q., Li, F., Yu, L., et al. (2019b). Ester-substituted pentathiophene copolymer-based sky-blue semitransparent solar cells for building windows. ACS Appl. Energ. Mater. 3 (1), 915. doi:10.1021/acsaem.9b02005
Wang, Y., Bai, S., Cheng, L., Wang, N., Wang, J., Gao, F., et al. (2016). High-efficiency flexible solar cells based on organometal halide perovskites. Adv. Mater. Weinheim 28 (22), 4532. doi:10.1002/adma.201504260
Wang, Z., Zeng, L., Zhang, C., Lu, Y., Qiu, S., Wang, C., et al. (2020). Rational interface design and morphology control for blade‐coating efficient flexible perovskite solar cells with a record fill factor of 81%. Adv. Funct. Mater. 30 (32), 2001240.
Yang, D., Yang, R., Priya, S., and Liu, S. (2018). Recent advanced in flexible perovskite solar cell: fabrication and application. Angew. Chem. Int. Edition 58, 4466.
You, S., Zeng, H., Ku, Z., Wang, X., Wang, Z., Rong, Y., et al. (2020). Multifunctional polymer‐regulated SnO2 nanocrystals enhance interface contact for efficient and stable planar perovskite solar cells. Adv. Mater. 32 (43), 2003990. doi:10.1002/adma.202003990
Zhang, C. C., Yuan, S., Lou, Y. H., Liu, Q. W., Li, M., Okada, H., et al. (2020). Perovskite films with reduced interfacial strains via a molecular‐level flexible interlayer for photovoltaic application. Adv. Mater. 32 (38), 2001479. doi:10.1002/adma.202001479
Zhao, P., Feng, L., Lin, Z., Wang, J., Su, J., Hu, Z., et al. (2019). Theoretical analysis of two‐terminal and four‐terminal perovskite/copper indium gallium selenide tandem solar cells. Sol. RRL 3 (11), 1900303. doi:10.1002/solr.201900303
Keywords: flexible perovskite solar cells, substrate and electrode, long-term stability, charge transport, photovoltaic performance
Citation: Liang X, Ge C, Fang Q, Deng W, Dey S, Lin H, Zhang Y, Zhang X, Zhu Q and Hu H (2021) Flexible Perovskite Solar Cells: Progress and Prospects. Front. Mater. 8:634353. doi: 10.3389/fmats.2021.634353
Received: 27 November 2020; Accepted: 11 January 2021;
Published: 16 March 2021.
Edited by:
Annie Ng, Nazarbayev University, KazakhstanReviewed by:
Ruiyuan Hu, Nanjing University of Posts and Telecommunications, ChinaAhmed Mourtada Elseman, Central Metallurgical Research and Development Institute (CMRDI), Egypt
Xiaolu Zheng, Shenzhen University, China
Copyright © 2021 Liang, Ge, Fang, Deng, Dey, Lin, Zhang, Zhang, Zhu and Hu. This is an open-access article distributed under the terms of the Creative Commons Attribution License (CC BY). The use, distribution or reproduction in other forums is permitted, provided the original author(s) and the copyright owner(s) are credited and that the original publication in this journal is cited, in accordance with accepted academic practice. No use, distribution or reproduction is permitted which does not comply with these terms.
*Correspondence: Xintao Zhang, zhangxintao@sina.com; Quanyao Zhu, cglamri@whut.edu.cn; Hanlin Hu, hanlin.hu@foxmail.com