- 1School of Design and Arts, Beijing Institute of Technology, Beijing, China
- 2School of Chemistry and Chemical Engineering, Beijing Institute of Technology, Beijing, China
Bionic adaptive camouflage material is a new artificial functional material, whose surface color can change adaptively according to the optical environments. Therefore, how to reduce the visual detectability of this material becomes a research hotspot of digital camouflage stealth technology. In order to clarify the limitations and opportunities of the bionic adaptive camouflage materials in the field of visual stealth, we summarize the applications of current adaptive camouflage materials and carry out a prospect of next-generation photonic crystal infrared camouflage material in this review. It is expected to provide a solution for the demand of digital camouflage adaptive materials in the modern battle field.
Introduction
In modern high-tech wars, military operation usually encounters quick mobility with various theater of war, frequent transformation of battlefields and different environmental backgrounds. The current camouflage painting technology cannot show self-adaptive camouflage capacity because traditional camouflage technology performs poorly in complicated optical backgrounds and battle fields with drastic change of temperature and humidity. Thus, in recent years, there has been an emerging demand on how to make camouflaged targets better adapt to the complex battlefield background. Meanwhile, bionic camouflage technology is becoming one of the vital ways to solve the focus issue (Lee et al., 2020; Xu et al., 2020). Organisms from many different phyla have evolved an impressive arsenal of light manipulation strategies that rely on the ability to generate and dynamically reconfigure hierarchically structured, complex optical material designs, often involving soft or fluid components. Optical components made fully or partially from reconfigurable, stimuliresponsive, soft solids or fluids—collectively referred to as soft photonics—are poised to form the platform for tunable optical devices with unprecedented functionality and performance characteristics (Kolle and Lee, 2018). Kolle and Lee (2018) presented the demonstration of the soft and skin-like imperceptible device that provides the instantaneous cloaking ability in the visible and IR region with the pixelized thermal operation inspired by the intriguing cloaking properties of cephalopods. They integrated the individual spectrum bands into one full-spectrum system that operates just by adjusting device temperature, thus exhibiting the active multi-spectral cloaking capability that is highly comparable to that of cephalopods. The skin-like cloaking platform not only translates fundamental camouflage features of cephalopods, but it also exhibits high practicality for the direct usage on the human skin unlike previous literature, which only examined the potential of their works in camouflage application (Figure 1).
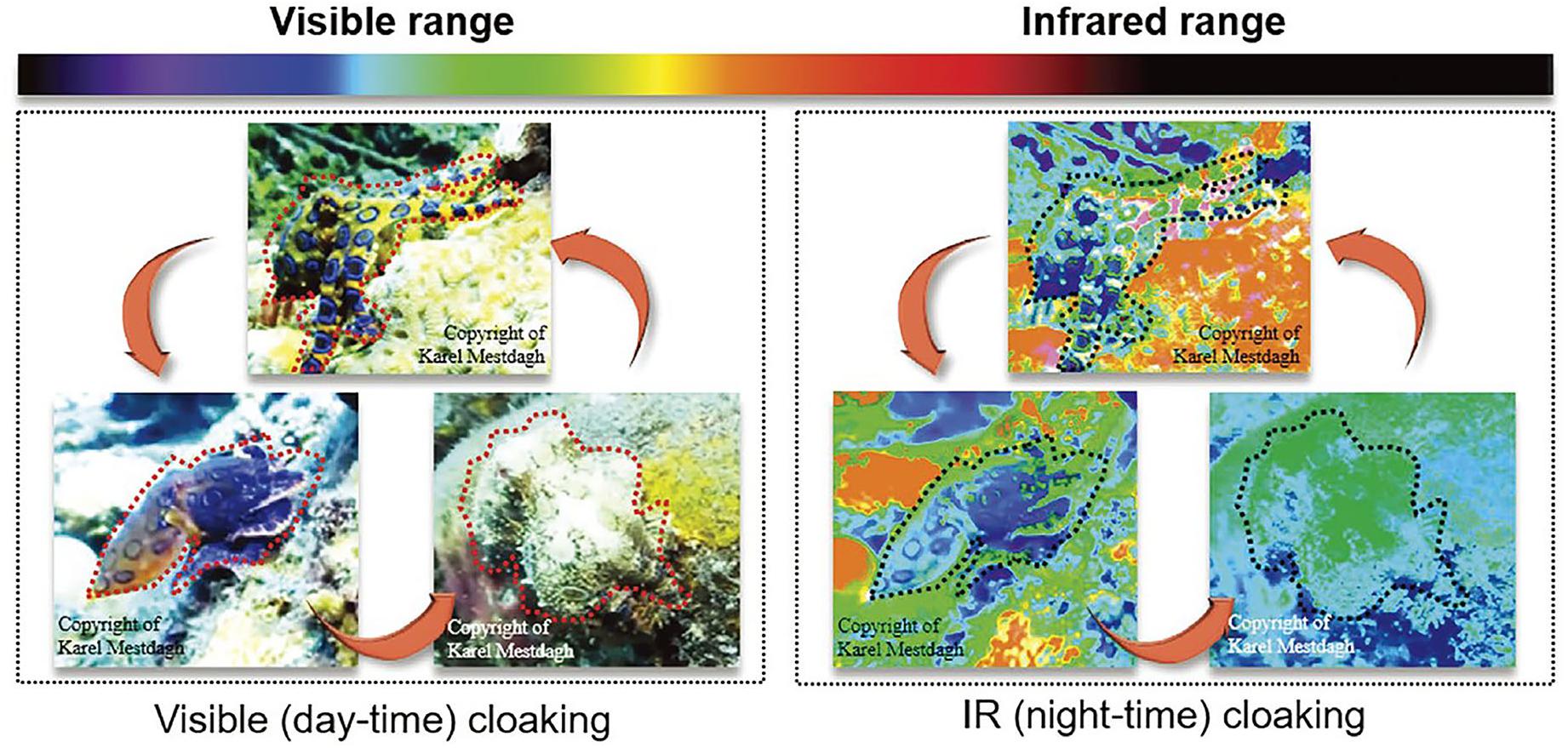
Figure 1. Dynamic camouflage ability of cephalopods both in the visible and infrared spectrum (Lee et al., 2020).
In nature, millions of living beings have undergone hundreds of millions of years of evolution, with reasonable and optimized structural features and adaptability to specific environments. They They have become the knowledge treasure and inspiration source for modern technology (Xu et al., 2018). Teyssier et al. (2015) show that chameleons shift color through active tuning of a lattice of guanine nanocrystals within a superficial thick layer of dermal iridophores combining microscopy, photometric videography and photonic band-gap modeling (Figure 2). In addition, they show that a deeper population of iridophores with larger crystals reflects a substantial proportion of sunlight especially in the near-infrared range (Teyssier et al., 2015). In the natural world that follows the law of the jungle, the weak are the prey of the strong. In order to survive and reproduce, many creatures are born with camouflage colors suitable for their environment. Their body color can be successfully merged into the natural environment to conceal their tracks.
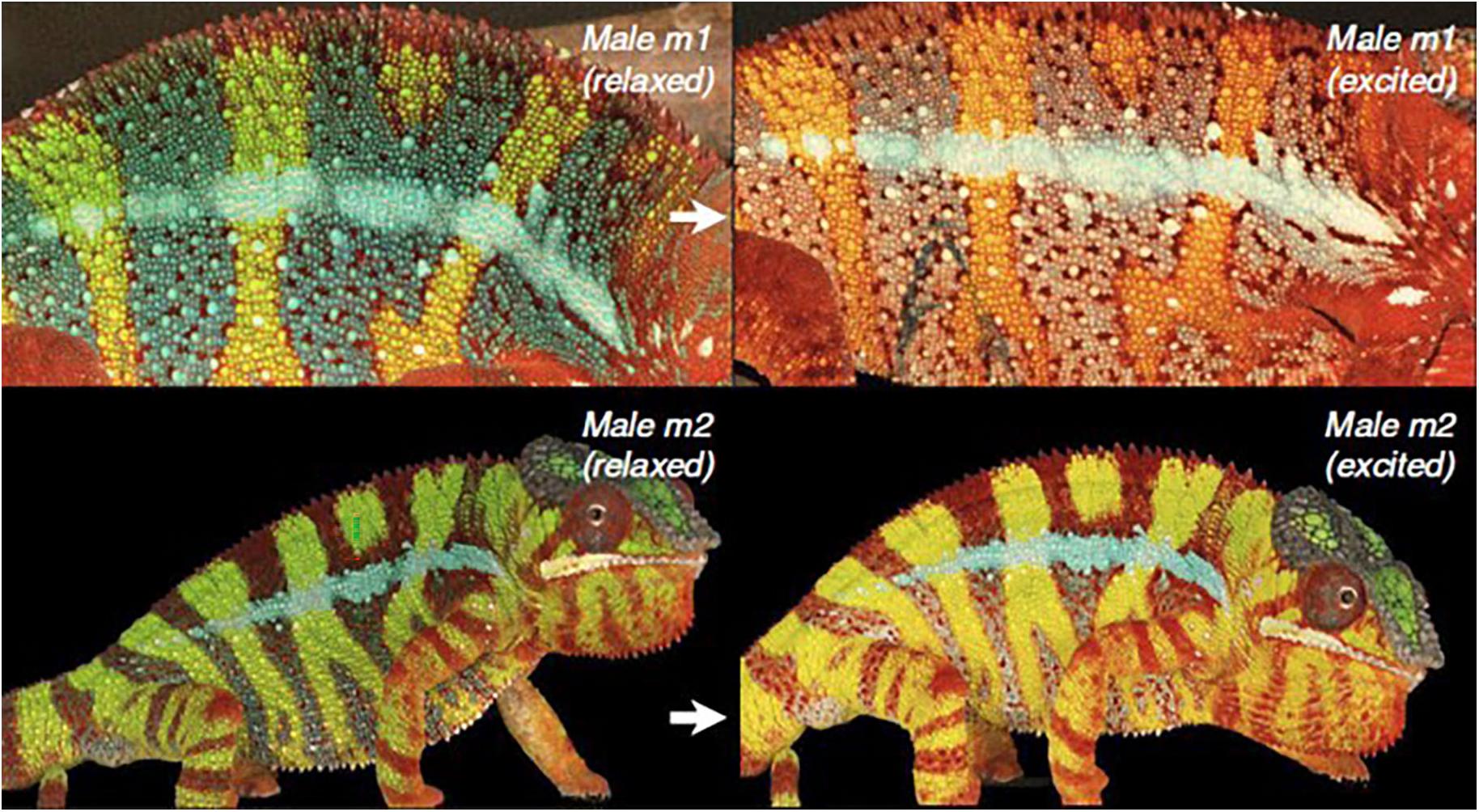
Figure 2. Color change and iridophore types in panther chameleons. Reversible color change is shown for two males (m1 and m2): during excitation (white arrows), background skin shifts from the baseline state (green) to yellow/orange and both vertical bars and horizontal mid-body stripe shift from blue to whitish (m1). Some animals have their blue vertical bars covered by red pigment cells.
In general, the camouflage colors of animals can be divided into three categories: one is the same color as the background color; the other is the camouflage color that matches the background color; the third is the flower pattern and spot pattern that contrast with the background color. The first and second categories are generally suitable for animals with restricted activities in certain environments. This is the root of traditional military camouflage. Indeed, digital camouflage painting is a kind of camouflage technology in which such information as natural background color with texture can be extracted by computer and digital image processing technique and then the minor unit of color lumps can be displayed. Actually, this digital camouflage painting is a common method to deal with visual reconnaissance, and reduce the optical differences between targets and surroundings to diminish conspicuousness of targets. The four-color camouflage of US Army is sensitive to the changes in seasons and environmental conditions (Figure 3). It is a four-color pattern made up of tawny, brown, dark green, black, which is a group of 12 standard camouflage colors made of alkyd resin paint. The four-color camouflage technique can take effect against ultraviolet, visible light, near-infrared reconnaissance as the visibility of the target can be reduced by 30% on average. Since 1977, the four-color camouflage has been applied to all technological equipment of the US army (land force) (Wen and Feng, 2017).
However, the current digital camouflage, which belongs to “Passive Mode,” can achieve anti-reconnaissance effect only in certain environment (Ye et al., 2010). When the environment changes, the existing camouflage targets are easily exposed. The visual stealth mode of military camouflage is mainly applied to the complicated battlefront environment. As the scope of military activities continues to expand, the environmental background has also undergone frequent changes. Therefore, it is of great importance to develop the visual stealth materials with the “self-adaption” function to the updated environmental background.
In fact, it is essential to learn from nature. The surface color of some living creatures can change with changes in the environment (Agez et al., 2017; Caro et al., 2017; Feller et al., 2017). For examples, oceanic cephalopods, such as sepia, their body surface colors can change in response to the surroundings’ alteration, such as light stimulation or temperature changes. They can achieve camouflage through integration with various background colors (Banisadr and Chen, 2017). Therefore, the combination of military camouflage technology and bionic materials can achieve the same “adaptive” camouflage function as living creatures (Yoshida and Lahann, 2008; Singh et al., 2012; Yu et al., 2014; Ordinario et al., 2017; Li et al., 2018). In the process of evolution, the special structure of organisms is gradually optimized, which brings endless inspiration to the research of bionic materials (Phan et al., 2013; Tadepalli et al., 2013; Brzicova et al., 2014).
Research on Bionic Camouflage Materials in Nature
Self-Adaption Regulation of “Camouflage Color” in Nature
Michel Milinkovic from University of Geneva in Switzerland found that the saurian animals, such as chameleons, can change light refraction to achieve color tuning by regulating the nanocrystals inside the skin surface. This transparent nanocrystal is located inside the iridophore layer and beneath the chromatophore of the chameleon. When the chameleon stays calmness, the nanocrystals are distributed in network and refract blue light. When the chameleon becomes excited, the nanocrystal structure becomes loose and refracts yellow or red light. In the deeper layer of the iridophore, the nanocrystal is more irregular, bigger and refracts stronger light. The two-layer structure of the iridophore is a new feature of evolution, which can make the chameleon achieve transformation between effective protective coloration and fresh bright colors, and it can also provide passive thermal protection.
As for oceanic cephalopods, there are two types of color changes: pigment color and structural color. The cells that can produce pigment molecules are pigment cells, which are located at the top of the corium layer, near the cuticle. Under the pigment cell layer, there are two kinds of cells that produce structural colors, the iridescent somatic cell and the white somatic cell (The white somatic cell usually exists in the octopus or sepia body, and the sleeve-fish does not have this cell) (Figure 4). In fact, the color demonstration of the cephalopod cuticle can be attributed to coefficient efforts of these three kinds of cells: the pigment cell, the iridescent somatic cell, and the white somatic cell.
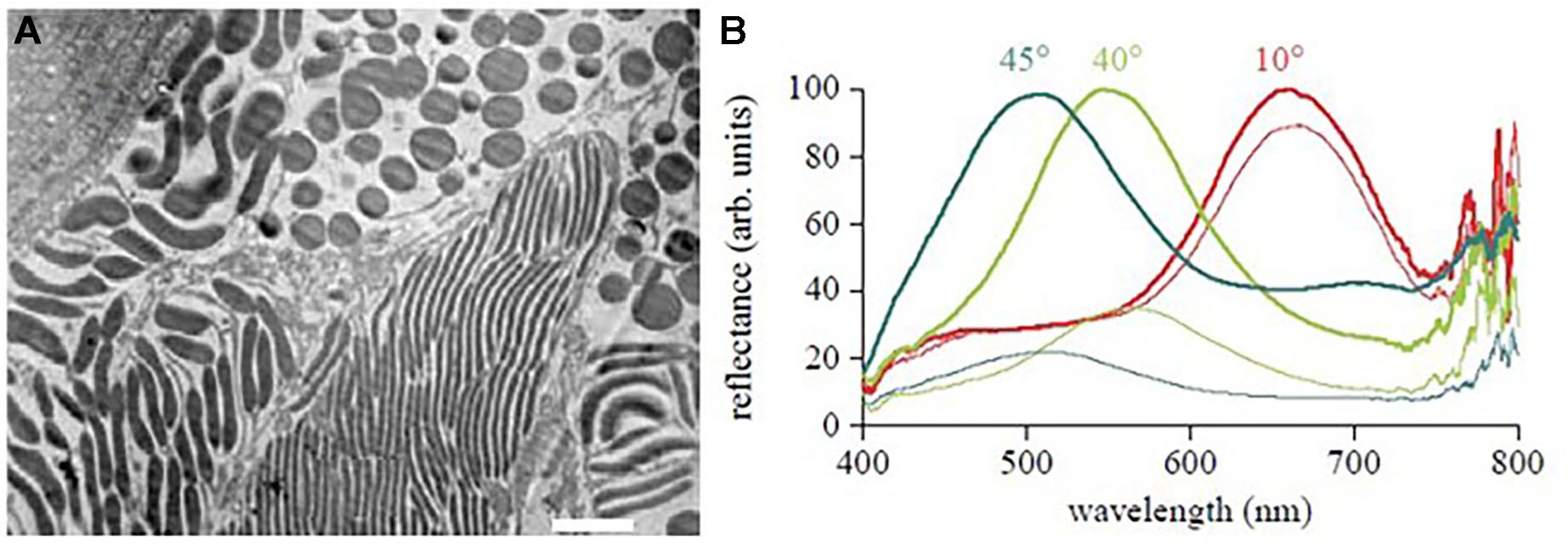
Figure 4. (A) Iridophore structure of sleeve-fish and (B) reflection spectrum under various incident light with different angles. Adapted from Mäthger et al. (2009).
The regularly arranged transparent structure in iridescent somatic cell forms the Bragg Reflector, which produces film interference to the transmitted light; meanwhile, the spherical white granule in the white somatic cell can cause broad-spectrum diffusive reflection and scattering of white light. Inside each pigment cell, there is a whippy pigment sac, whose surface has radicalized muscle fibers. Under the control of nerves, these muscle fibers can contract and relax, control the size of the pigment sac, thereby changing the density of pigment molecules in different pigment sacs.
The mode of color change of oceanic cephalopods is mainly structural color change. In the spherical white granule of the white somatic cell in the octopus and sepia, there is a protein that causes light interference. This type of protein is named “reflectin” (reflective protein) due to its high refractive index (Izumi et al., 2010; DeMartini et al., 2013; Ghoshal et al., 2013, 2014). Reflectin can accomplish self-assembly of the “Hexagon” or “Pentagon” basic units, which can be called “reflectin bricks.” These basic units are just like bricks. They are fully extended on the same plane and can produce branch structures and realize various patterns. Moreover, changes in seawater temperature and pH may cause the structural change of these reflective protein, thereby changing the structural color and achieving multiple colors (Li, 2011).
The wings of coleopteran insects (such as diamond weevils) are covered with iridescent glistening scales, like a coat decorated with gems. It is found that the chitin crystal on the wings can form a diamond-type array, which reflects green, yellow, orange sunlight. Bodo Wilts from University of Groningen analyzed the diamond-type scales of the diamond weevils using Scanning Electron Microscope (SEM) and scatterometer. The crystal structure inside the weevil can be analyzed through the tiny beams reflected by the scales of the weevil. The repeating crystal structures are demonstrated that they are mostly striation patterns or the single-track wrinkle patterns. It is found that the scale is a photonic crystal similar to opal. Each type of photonic crystal can reflect a specific wavelength of light in a specific direction.
Similarly, Lepidoptera insects (such as butterflies) can tune their colors by the structural colors on their scales. When the light irradiates on their surface, some microstructures such as ridges, lines, facets, and granules generate interference, scattering, and diffraction, so that the colors change accordingly. Sometimes the structural color is called metallochrome or iridescence.
Structural color show following characteristics: one is the diversity of color levels; the second is the diversity of color types; the third is the diversity of color changes. Meanwhile, color change can be divided into three types. Firstly, the filling medium can cause color change. When the alcoholic solution with different refractive index is filled into the gaps between the scales, the optical coloring conditions of the scale structure will change, leading to the change of color. Secondly, angle alteration can make color change. For instance, the scale colors of some insects will change with the alteration of incident angle and viewing angle of light. Thirdly, under some invariant coloring circumstances, neither change of the medium’s refractive index nor alteration of incident angle and viewing angle can make colors of insect scales change. Only the pigment coloring is the reason for color change.
In nature, there are many other living creatures that can change their body colors. They have attained amazing abilities of color change during continuous evolution over hundreds of millions of years. Therefore, it is of vital importance to learn from nature, make use of living creature’s color-change mechanism, investigate the principles of bionic stealth and then achieve the key technology of self-adaption camouflage with color change. Based on the bionic color-change materials, it is possible to address the self-adaption issue of traditional camouflage materials and give pivotal technological support to camouflage surfaces under the military situation.
The Application of Self-Adaption Bionic Camouflage Mechanism
Researchers have simulated the bionic color-change structure for the application of the camouflage materials (Rossiter et al., 2012; Yan et al., 2016; Wang et al., 2017).
Chu Sheng and Wang Guo-ping designed a mechanized artificial chameleon, which can use the metal nanoparticles on its skin to accomplish color transformation (Figure 5). The color range of variation can almost cover all tones in the visible spectrum (Wang et al., 2016). Li et al. (2020) prepared adaptive thermal camouflage devices by bridging the optical and radiative properties of nanoscopic platinum (Pt) films and silver (Ag) electrodeposited Pt films (Figure 6). These metal-based devices have large, uniform, and consistent IR tunabilities in mid-wave IR and long-wave IR atmospheric transmission windows. Furthermore, these devices can be easily multiplexed, enlarged, applied to rough and flexible substrates, or colored, demonstrating their multiple adaptive camouflaging capabilities (Li et al., 2020). Peng et al. (2018) proposed a novel approach for infrared stealth that relies on the combination of emissivity (ε) reduction in the atmospheric windows (3–5 and 8–14 μm) and radiative cooling in a non-atmospheric window (5–8 μm). The fabricated selective emitter has low emissivity (ε3–5μm = 0.18; ε8–14μm = 0.31) in the atmospheric windows for infrared “invisibility” and high emissivity (ε5–8μm = 0.82) outside the atmospheric window for radiative cooling and functions from ambient temperature to 200°C. This work shows that selective emissive materials have promising application prospects and provide a more effective solution for infrared stealth technology (Peng et al., 2018).
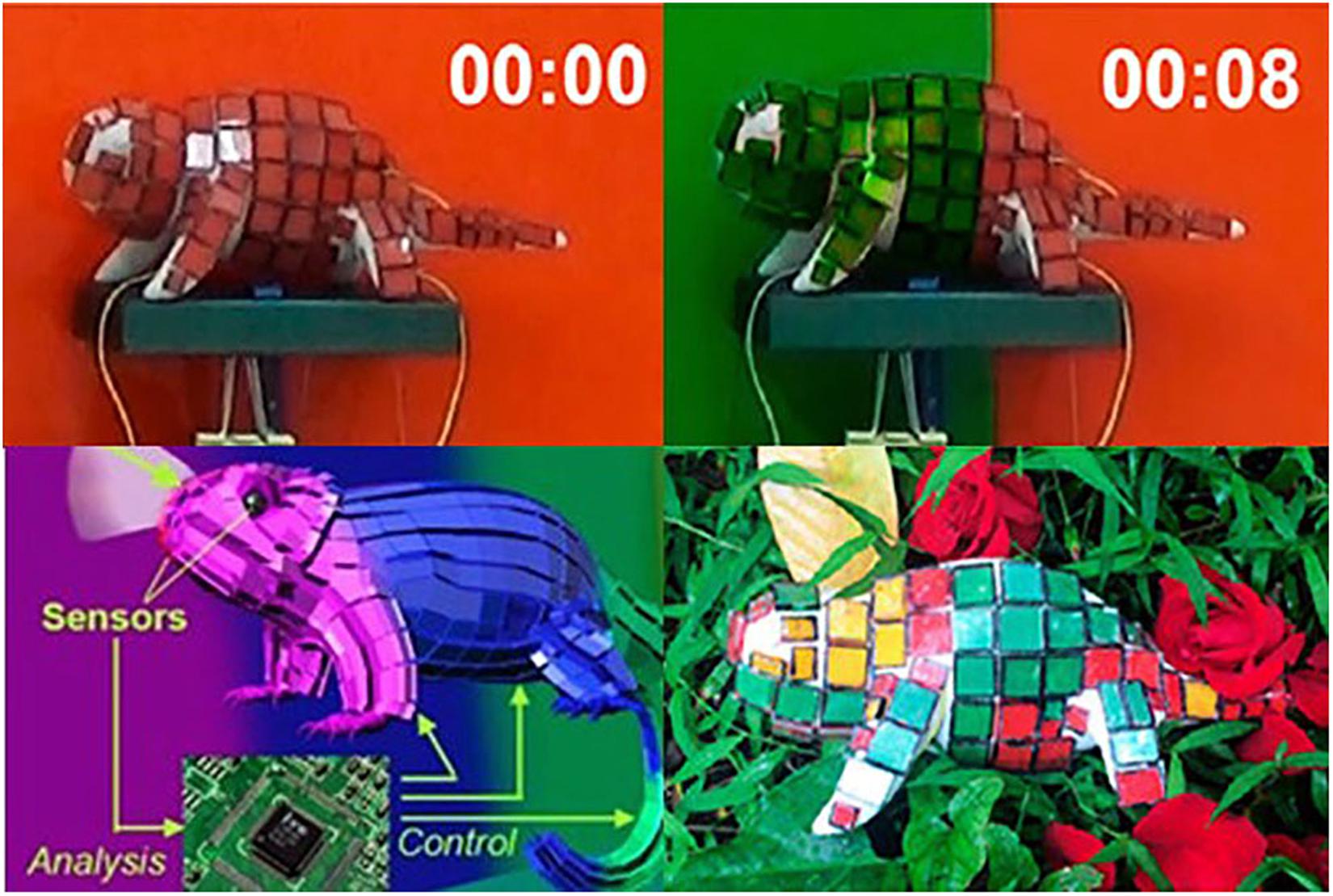
Figure 5. The pictures of artificial Chameleon. Adapted from Wang et al. (2016).
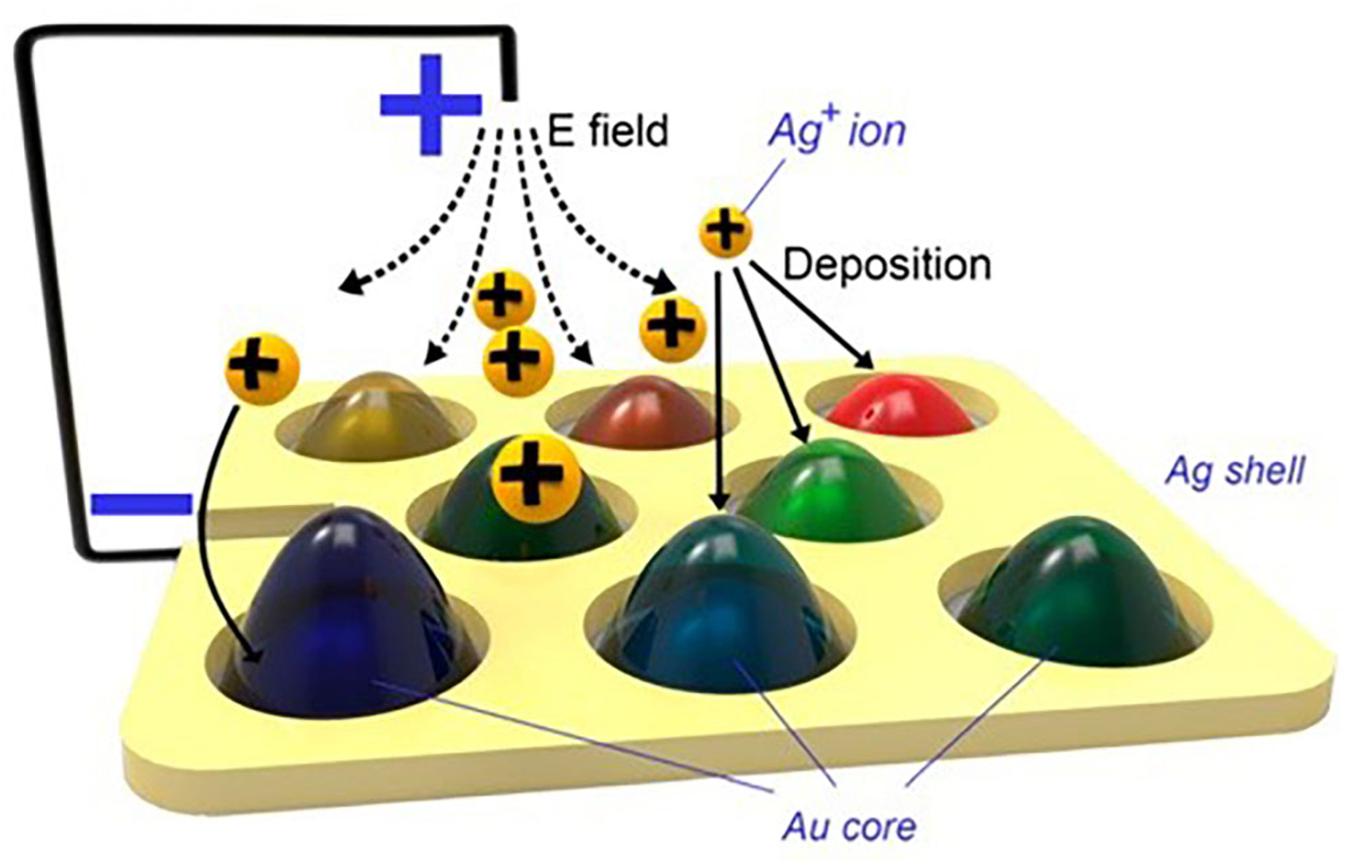
Figure 6. Schematic diagram of electrochemical silver plating and the colors of nanoparticles with different silver shell thickness. Adapted from Wang et al. (2016).
The neat rows of pits are etched on the silicon dioxide covering the conductive glass. Gold nanoparticles grow in these pitting layers through evaporating. And then these rows of pits were sealed by electrode plate of gelatin with silver-ion. Later the entire scale and shell were wrapped. At this moment, the “scales and shell” of the artificial chameleon can be dyed red by gold nanoparticles. In the circuit of “gelatin electrode—gold nanoparticles—conductive glass,” silver-ion can be reduced to silver through an electrochemical reduction reaction and deposited onto the surface of gold nanoparticles. After the gold core surface is plated with silver, as the thickness of the silver shell increases, the color of the nanoparticles changes from red to green, and finally to blue. By adjusting the conduction time of the electrochemical reaction, the thickness of the silver shell can be controlled well.
Actually, just by several seconds of current control, the color of these scales can be repeatedly changed from red to blue. These scales can be pasted onto 3D printed chameleon model. Furthermore, eyes of chameleon are equipped with a color sensor. Through controlling the time of electro-oxidation/electro-reduction reaction, the thickness of silver shell can be controlled in real time, so that the chameleon can change its body color as the background environment changes.
Researchers from UC Irvine got inspiration from calamari and then developed a sticker material that enables soldiers against infrared camera (Teyssier et al., 2015). There are iridescence cells in the calamari’s skin that have blood platelets containing reflective proteins. The calamari can regulate the thickness of these platelets and the gap between them, so that it can reflect various light. Researchers used colibacillus to cultivate the reflectin which can be packed on the hard surface layer. This film was exposed to acetic steam for heat inflation. The treated film can reflect the infrared light consistent with the infrared light reflected by the background to avoid infrared detection. The researchers integrated reflectin into a polymer sticker, which is flexible, light and thin, and available for back-stickup. By stretching the sticker, reflectin can be activated instead of acetic steam. When this sticker is pasted on the coat or equipment surfaces of the camouflage target, the camouflage target will be successfully “merged” into the background so as to avoid the “capture” of the infrared camera and achieve the purpose of camouflage.
A new “chameleon tank” are developed by the researchers in Germany and Canada. It can change its surface color at any time as the environment changes. There is a thin film on the surface of the chameleon tank, which can change the appearance characteristics of the tank according to the color of the surrounding environment, adjust the temperature of the tank in time, and make it difficult for the enemy to detect the trajectory of the tank by thermal radiation. Finally, the purpose of stealth is achieved.
It is reported that some color-changing and thermochromic coatings are currently being developed. The color-changing coatings are made of a special cloth with photosensitive substances. Therefore, their color can be automatically changed with the change of the environmental photochromism, so that the target wearing the color-changing coatings cannot be exposed in any environment. Meanwhile, the thermochromic coating is a “destined color-changing” to the alteration of environmental temperature, which can significantly reduce the detection probability of thermal imaging cameras and radar. Therefore, the successful development and application of these “Color-changing Magic” will have great potential on the battlefield in the future.
Research on Artificial Bio-Inspired Photonic Crystals Stealth Materials
In fact, there is a principle of survival of the fittest during the natural selection. The invisible master came into being after billions of years of evolution. For example, chameleons and marine cephalopods (such as octopus and pocket fish), their body color will change with the environmental temperature and humidity. The surface of the chameleon’s skin has opal-like nanostructures, which -respond to environmental variables as the color of the specific structure changes (Figure 7). When the environmental temperature changes, the nanostructure will change accordingly, causing the color of the structure to change, so as to achieve the invisibility. Cephalopod skin cells contain functional protein reflectin. This spindle-shaped protein assembles into a laminar Bragg reflector. Moreover, changes of temperature and pH in seawater can cause changes in the structure of proteins, leading to changes in the color of the structure. The above biological camouflage mechanism can be attributed to the change of the structure color of the photonic crystal (Holt et al., 2011; Wilts et al., 2012; Sun et al., 2013; Barrows and Bart, 2014; Deravi et al., 2014; Gur et al., 2016; Meng et al., 2019).
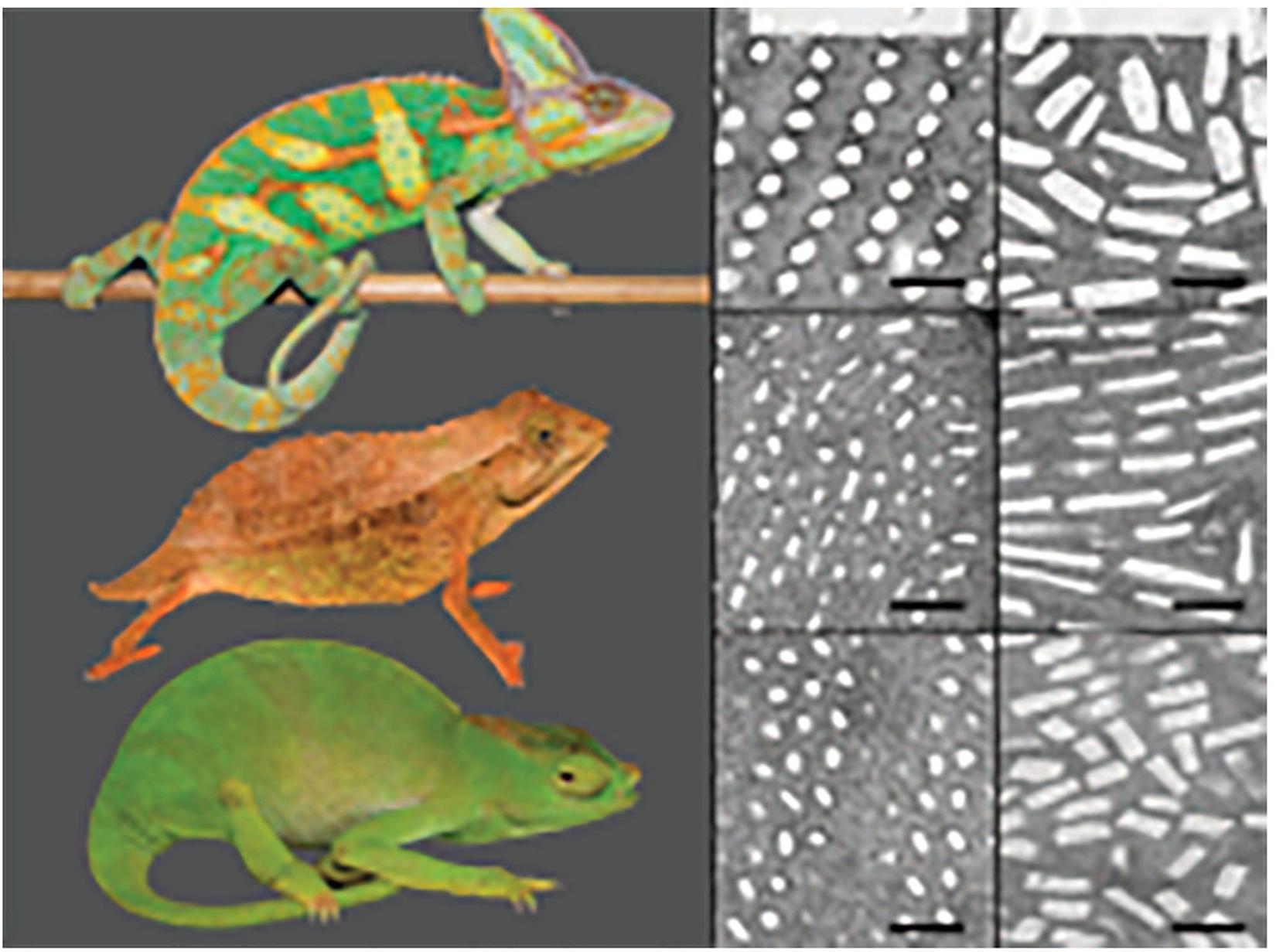
Figure 7. The invisibility and skin microstructure changes of chameleons and other camouflaged animals. Adapted from Teyssier et al. (2015).
For high-temperature targets, theoretical studies have confirmed that the photonic crystal has the obvious advantages in achieving infrared suppression and stealth of multi-band or broadband. And the theory was verified by experiments. Negative refractive metamaterials, including photonic crystals, are still in the initial stages of theoretical and experimental exploration, but the realization of “invisible cloaks” in the visible band has an attractive prospect. A lot of work has been done on the design and construction of photonic crystal structures.
The stealth function of photonic crystals has been studied in the United States for many years. The United States Military Academy at West Point has established a photonic crystal research center. In recent years, DAPA has also increased its investment and sponsorship in this field. In addition, Raytheon is developing bionic adaptive optoelectronic materials and equipment. At the same time, many European companies have also received AFRL/AFOSR/IOE (EOARD) investment and sponsorship in bionic stealth technology. Biomimetic stealth technology based on photonic crystals is the key technology for intelligent stealth in future weapons and equipment (Zhou et al., 2019). Advanced and reliable stealth technology must be possessed to improve the survivability of troops and equipment. Therefore, it is very necessary to carry out independent innovation research on bionic stealth technology.
The colloidal crystals used in stealth technology are usually composed of 2D or 3D photonic crystals with long-range periodicity. The surface color of the photonic crystal is mainly determined by the width and position of the photonic band gap, which is also closely related to the lattice parameters and the refractive index of the filler (Figure 8). Every parameter may cause the photonic band gap to change. On the surface of colloidal crystals, the discoloration mechanism can be divided into two categories. Firstly, some gaps of the colloidal crystal can be filled with the medium material, which can change its refractive index by external stimulation, aiming to change the refractive index between the colloidal particles and the medium surroundings. Secondly, the photonic band gap position of the photonic crystal can be adjusted by changing the lattice parameters.
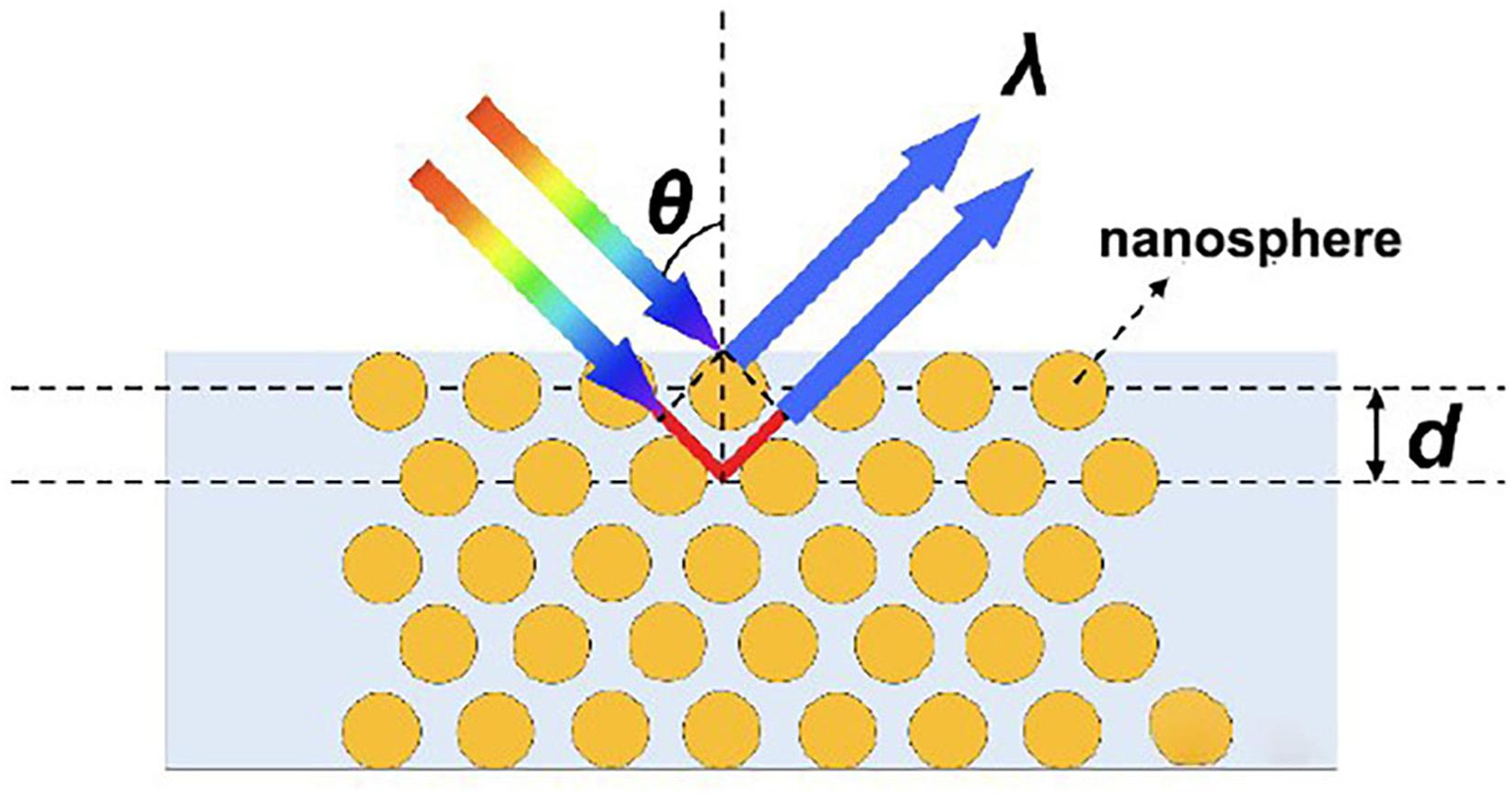
Figure 8. The diffraction mechanism diagram of photonic crystal. Adapted from Qi (2020).
Color-tunable colloidal crystals have great potential in many fields. In fact, many colloidal crystals can “sense” changes in external stimuli (such as electricity, light, heat, magnetism, and chemistry) and change the structure color. These studies are based on responsive polymers. In particular, responsive polymers can be filled into the gaps of opal photonic crystals composed of monodisperse polystyrene (or silica) microspheres through self-assembly. At the same time, the reactive polymer can be filled into the gaps of the inverse opal colloid. Under the stimulation of the external environment (such as temperature, electric field, magnetic field, pH), the volume of the polymer will change (swell or shrink), and its refractive index will also change, which leads to the change of the photonic band gap and the displacement of the diffraction peak. Furthermore, if the diffraction peak of the response photonic crystal is in the visible or near-infrared spectral range, the material will visually display color changes during the movement of the diffraction peak. Therefore, the performance of the responsive crystal can be evaluated by this discoloration ability. In order to obtain ideal diffraction peaks and the desired colors in the variable structure color design, it is of great significance to research the relationship between color change and colloidal crystal’s refractive index and lattice spacing.
The Application of Color-Changing Camouflage Coatings for Military Operation
Thermal and Photosensitive Fibers
New thermal and photosensitive fibers, which can automatically change the color, can be prepared by biomimetic materials. These fibers are sensitive to light, ambient temperature and humidity. In addition, the photosensitive chameleon fiber will change its color under ultraviolet light or visible light and display a reversible graphic pattern. The American “Chameleon” camouflage combat uniform is a successful individual equipment, which is made of photosensitive chameleon fibers. By sensing the changes in external visible light and the temperature of the heat source, it can change its color accordingly, thereby ensuring the uniform can change color with the external environment. Besides, Merck has successfully developed a thermal chameleon combat uniform based on a specific black fabric material. Microcapsules of Liquid crystal printed or dyed on black fabrics can change color in several temperature ranges, such as frigid regions, temperate regions, and tropical regions). When wearing a thermal liquid crystal chameleon combat uniform, soldiers can blend into the natural environment, even without a shield, and the enemy cannot identify them visually. In addition, this uniform also has the function of isolating the heat from the soldier, which will make infrared reconnaissance invalid.
Electrochemical Dye
Through electronic simulation technology, the camouflage coating can obtain signals from the surrounding colors and change its color accordingly, which is called electrochemical dye. In the best case, the information about the environment collected by low-light-level camera is processed by the computer and output as a signal to complete the conversion of the color system, and finally display the optical image of the soldier’s background on the soldier’s uniform.
Dynamic Optical Camouflage
Dynamic optical camouflage connects the visible light detector and the conductive molecule to realize the color and light conversion of the camouflage coating. When soldiers move from one background to another, dynamic optical camouflage technology can rapidly adapt to the new background, and constantly change the coat color. For example, when soldiers are in the forest, their camouflage uniforms can be the same color as the green and brown backgrounds. If soldiers move to a wheat field, the color of their coats will quickly change to orange, khaki, and brown.
Smart Camouflage Materials
The existing camouflage coating is passive. They can only achieve the effect of anti-reconnaissance in a static state. When there is wind, the enemy can easily find a “leaf” or a “bush” still in the swaying grass, causing to find the camouflage target through subtle differences. Therefore, active camouflage technology has received massive attention. The US Army has developed an electrochromic film that can be pasted or painted on camouflage uniforms. Meanwhile, this electrochromic film can be connected to micro smart processing and environmental sensor systems. During the wind, the system can change the graphic patterns on the film to achieve a swinging visual effect similar to the background and achieve the camouflage.
Conclusion and Prospects
The application of biomimetic adaptive color-changing materials in stealth technology is summarized in this article. For the demand of the new generation of discoloration camouflage coating, some solutions are proposed:
(A) Bionic adaptive color-changing materials suitable for battlefields not only need to select adaptive color-changing composite materials, but also need to collect environmental digital image data to establish a database of the main camouflage environment. Through image analysis software, the essential colors can be extracted from the digital environment image, thereby designing digital camouflage paintings based on different background environments. Furthermore, the color similarity and algorithm of digital camouflage painting database are analyzed. Through the structural color of the photonic crystal, traditional digital camouflage paintings can be obtained. In the optical environment, photonic crystals have the characteristics of structural discoloration, combined with the multiple matching of digital camouflage paintings, realizing the controllability of color changes, so that the bionic materials can meet the requirements of adaptive camouflage against complex environmental backgrounds.
(B) For the bionic adaptive stealth material, the priority is to set up the relationship between the color change and the optical background. In fact, the analysis of this relationship can be achieved by studying the color response of the photonic crystal to environmental variables such as temperature and humidity. Therefore, it is necessary to study the relationship between the microstructure and color of the biomimetic photonic crystal. How to adjust the microstructure of the bionic photonic crystal to meet specific color demands requires further research. In addition, how to optimize the chemical composition of the biomimetic photonic crystal to ensure the response to environmental variables is a crucial issue. Therefore, the use of suitable composite materials to construct photonic crystals is a hot spot of current research and one of the basic solutions.
(C) Although the current stealth material field is still based on traditional stealth methods, such as camouflage coating materials. Because of higher and further requirement, unceasingly updating diversity and precision of future detection methods for the stealth materials, the photonic crystals, which can be designed and dynamically adjusted due to their structure, will certainly play a greater role in the development of digital camouflage stealth in the future.
Author contributions
YQ organized and compiled this article. ZM, PW, and DY provided the ideas and references. All authors contributed to the article and approved the submitted version.
Funding
This work was financially supported by the 2018 Beijing Social Science Foundation project funding (Grant No. 18YTC025) and the Youth YUYOU Talent Training Program of North China University of Technology.
Conflict of Interest
The authors declare that the research was conducted in the absence of any commercial or financial relationships that could be construed as a potential conflict of interest.
References
Agez, G., Bayon, C., and Mitov, M. (2017). Multiwavelength micromirrors in the cuticle of scarab beetle Chrysina gloriosa. Acta Biomater. 48, 357–367. doi: 10.1016/j.actbio.2016.11.033
Banisadr, S., and Chen, J. (2017). Infrared actuation-induced simultaneous reconfiguration of surface color and morphology for soft robotics. Sci. Rep. 7, 17521–17530.
Barrows, F. P., and Bart, M. H. (2014). Photonic structures in biology: a possible blueprint for nanotechnology. Nanomater Nanotechnol. 4, 1–12. doi: 10.5772/58289
Brzicova, T., Feliu, N., and Fadeel, B. (2014). Biomimetic camouflage: nanoporous particles cloaked in cellular membranes. Nanomedicine 9, 19–20.
Caro, T., Stoddard, M. C., and Stuart-Fox, D. (2017). Animal coloration research: why it matters. Philos. Trans. R. Soc. Lond. B Biol. Sci. 372:20160333. doi: 10.1098/rstb.2016.0333
DeMartini, D. G., Ghoshal, A., Pandolfi, E., Weaver, A. T., Baum, M., and Morse, D. E. (2013). Dynamic biophotonics: female squid exhibit sexually dimorphic tunable leucophores and iridocytes. J. Exp. Biol. 216, 3733–3741. doi: 10.1242/jeb.090415
Deravi, L. F., Magyar, A. P., Sheehy, S. P., Bell, G. R. R., Mathger, L. M., Senft, S. L., et al. (2014). The structure-function relationships of a natural nanoscale photonic device in cuttlefish chromatophores. J. R. Soc. Interface 11:20130942. doi: 10.1098/rsif.2013.0942
Feller, K. D., Jordan, T. M., Wilby, D., and Roberts, N. W. (2017). Selection of the intrinsic polarization properties of animal optical materials creates enhanced structural reflectivity and camouflage. Philos. Trans. R. Soc. Lond. B Biol. Sci. 372:20160336. doi: 10.1098/rstb.2016.0336
Ghoshal, A., DeMartini, D. G., Eck, E., and Morse, D. E. (2014). Experimental determination of refractive index of condensed reflectin in squid iridocytes. J. R. Soc. Interface 11:20140106. doi: 10.1098/rsif.2014.0106
Ghoshal, A., Demartini, D. G., Eck, E., and Morse, D. E. (2013). Optical parameters of the tunable bragg reflectors in squid. J. R. Soc. Interface 10:20130386. doi: 10.1098/rsif.2013.0386
Gur, D., Leshem, B., Farstey, V., Oron, D., Addadi, L., and Weiner, S. (2016). Light-induced color change in the sapphirinid copepods: tunable photonic crystals. Adv. Funct. Mater. 26, 1393–1399. doi: 10.1002/adfm.201504339
Holt, A. L., Sweeney, A. M., Johnsen, S., and Morse, D. E. (2011). A highly distributed bragg stack with unique geometry provides effective camouflage for loliginid squid eyes. J. R. Soc. Interface 8, 1386–1399. doi: 10.1098/rsif.2010.0702
Izumi, M., Sweeney, A. M., Demartini, D., Weaver, J. C., Power, M. L., Tao, A., et al. (2010). Changes in reflectin protein phosphorylation are associated with dynamic iridescence in squid. J. R. Soc. Interface 7, 549–560. doi: 10.1098/rsif.2009.0299
Kolle, M., and Lee, S. (2018). Progress and opportunities in soft photonics and biologically inspired optics. Adv. Mater. 30:1702669. doi: 10.1002/adma.201702669
Lee, J., Sul, H., Jung, Y., Kim, H., Han, S., Choi, J. H., et al. (2020). Thermally controlled, active imperceptible artificial skin in visible-to-infrared range. Adv. Funct. Mater. 30:2003328. doi: 10.1002/adfm.202003328
Li, J. W. (2011). Mechanism of Structural Color in Creatures and Bionic Design of Structural Color. Ph.D. thesis, University of Science and Technology of China, Hefei, 31–35.
Li, J. X., Angsantikul, P., Liu, W. J., Ávila, B. E. F., Chang, X. C., Sandraz, E., et al. (2018). Biomimetic platelet-camouflaged nanorobots for binding and isolation of biological threats. Adv. Mater. 30:1704800. doi: 10.1002/adma.201704800
Li, M. Y., Liu, D. Q., Cheng, H. F., Peng, L., and Zu, M. (2020). Manipulating metals for adaptive thermal camouflage. Sci. Adv. 6:eaba3494. doi: 10.1126/sciadv.aba3494
Mäthger, L. M., Denton, E. J., Marshall, N. J., and Hanlon, R. T. (2009). Mechanisms and behavioural functions of structural coloration in cephalopods. J. R. Soc. Interface 6, 149–163. doi: 10.1098/rsif.2008.0366.focus
Meng, Z. P., Huang, B. T., Wu, S. L., Li, L., and Zhang, S. F. (2019). Bio-inspired transparent structural color film and its application in biomimetic camouflage. Nanoscale 11, 13377–13384. doi: 10.1039/c9nr04360h
Ordinario, D. D., Leung, E. M., Phan, L., Kautz, R., Lee, W. K., Naeim, M., et al. (2017). Protochromic devices from a cephalopod structural protein. Adv. Opt. Mater. 5:1600751. doi: 10.1002/adom.201600751
Peng, L., Liu, D. Q., Cheng, H. F., Zhou, S., and Zu, M. (2018). A multilayer film based selective thermal emitter for infrared stealth technology. Adv. Opt. Mater. 6:1801006. doi: 10.1002/adom.201801006
Phan, L., Walkup, W. G., and Gorodetsky, A. A. (2013). “Biomimetic camouflage inspired by cephalopods,” in Proceedings of the 245th ACS National Meeting & Exposition, Abstracts of Papers of the American Chemical Society, New Orleans, LA, 245.
Qi, F. L. (2020). “Preparation of photonic crystals and their sensing applications to organophosphates,” in Doctoral Dissertation. (Beijing University of technology).
Rossiter, J., Yap, B., and Conn, A. (2012). Biomimetic chromatophores for camouflage and soft active surfaces. Bioinspir. Biomim. 7:036009. doi: 10.1088/1748-3182/7/3/036009
Singh, A. V., Rahman, A., Sudhir Kumar, N. V. G., Aditi, A. S., Galluzzi, M., Bovio, S., et al. (2012). Bio-inspired approaches to design smart fabrics. Mater. Des. 36, 829–839. doi: 10.1016/j.matdes.2011.01.061
Sun, J. Y., Bhushan, B., and Tong, J. (2013). Structural coloration in nature. RCS Adv. 3, 14862–14889. doi: 10.1039/c3ra41096j
Tadepalli, S., Slocik, J. M., Gupta, M. K., Naik, R. R., and Singamaneni, S. (2013). Bio-optics and bio-inspired optical materials. Chem. Rev. 117, 12705–12763. doi: 10.1021/acs.chemrev.7b00153
Teyssier, J., Saenko, S. V., Marel, D. V., and Milinkovitch, M. C. (2015). Photonic crystals cause active colour change in chameleons. Nat. Commun. 6, 6368–6375.
Wang, G., Chen, X., Liu, S., Wong, C. P., and Chu, S. (2016). Mechanical chameleon through dynamic real time-plasmonic tuning. ACS Nano 10, 1788–1794. doi: 10.1021/acsnano.5b07472
Wang, X. Y., Deng, Y. Q., Yang, D., Xiao, Y., Zhao, H., Nian, Q. G., et al. (2017). Biomimetic inorganic camouflage circumvents antibody-dependent enhancement of infection. Chem. Sci. 8, 8240–8246. doi: 10.1039/c7sc03868b
Wen, Z. G., and Feng, F. P. (2017). Research progress in bionic mechanics. Guangdong Sci. Technol. 89–90.
Wilts, B. D., Michielsen, K., Kuipers, J., Raedt, H. D., and Stavenga, D. G. (2012). Brilliant camouflage: photonic crystals in the diamond weevil, Entimus imperialis. Proc. R. Soc. B Biol. Sci. 279, 2524–2530. doi: 10.1098/rspb.2011.2651
Xu, C. Y., Escobar, M. C., and Gorodetsky, A. A. (2020). Stretchable cephalopod-inspired multimodal camouflage systems. Adv. Mater. 32:1905717. doi: 10.1002/adma.201905717
Xu, C. Y., Stiubianu, G. T., and Gorodetsky, A. A. (2018). Adaptive infrared-reflecting systems inspired by cephalopods. Science 359, 1495–1500. doi: 10.1126/science.aar5191
Yan, Y., Liu, L., Cai, Z., Xu, J. W., Xu, Z., Zhang, D., et al. (2016). Plasmonic nanoparticles tuned thermal sensitive photonic polymer for biomimetic chameleon. Sci. Rep. 6, 31328–31337.
Ye, X. Q., Ji, L. H., Xie, Y. M., and Huang, L. N. (2010). Discussion on the combination of rehabilitation training robot and traditional Chinese medicine rehabilitation method. Chin. J. Rehabil. Med. 25, 781–784.
Yu, C. J., Li, Y. H., Zhang, X., Huang, X., Malyarchuk, V., Wang, S. D., et al. (2014). Adaptive optoelectronic camouflage systems with designs inspired by cephalopod skins. Proc. Natl. Acad. Sci. U.S.A. 111, 12998–13003. doi: 10.1073/pnas.1410494111
Keywords: photonic crystal, adaptive, stealth, digital camouflage, color-changing
Citation: Qiao Y, Meng Z, Wang P and Yan D (2021) Research Progress of Bionic Adaptive Camouflage Materials. Front. Mater. 8:637664. doi: 10.3389/fmats.2021.637664
Received: 13 December 2020; Accepted: 26 February 2021;
Published: 08 April 2021.
Edited by:
Brahim Aissa, MPB Technologies & Communications, CanadaReviewed by:
Dongqing Liu, National University of Defense Technology, ChinaXuan Shouhu, University of Science and Technology of China, China
Copyright © 2021 Qiao, Meng, Wang and Yan. This is an open-access article distributed under the terms of the Creative Commons Attribution License (CC BY). The use, distribution or reproduction in other forums is permitted, provided the original author(s) and the copyright owner(s) are credited and that the original publication in this journal is cited, in accordance with accepted academic practice. No use, distribution or reproduction is permitted which does not comply with these terms.
*Correspondence: Yu Qiao, NDk5OTA0Mjk1QHFxLmNvbQ==