- 1Transportation Engineering College, Dalian Maritime University, Dalian, China
- 2Liaoning Provincial Transportation Science Research Institute Co. Ltd., Shenyang, China
- 3Department of Civil and Environmental Engineering, Michigan Technological University, Houghton, MI, United States
- 4School of Transportation Science and Engineering, Harbin Institute of Technology, Harbin, China
The objective of this study aims to investigate the performance of thermoplastic polyurethane elastomers (TPU)-modified asphalt, with special focus on the influence of polyurethane synthesis parameters upon the performance of base asphalt. In this study, the TPU modifier was incorporated into base asphalt to prepare TPU-modified asphalt by using a self-determined laboratory process. The properties of polyester-based TPU and polyether-based TPU modified asphalt with different contents was analyzed by the base performance tests. The Fourier transform infrared spectroscopy (FTIR), fluorescent microscope, scanning electron microscopy (SEM), differential scanning calorimetry (DSC), thermal gravity (TG), dynamic shear rheology (DSR), and bending beam rheology (BBR) tests were conducted to expose chemical, microstructure, and rheological properties of the asphalt binders, respectively. The results indicated that the modified asphalt with 5% TPU modifier exhibited a favorable performance in terms of the penetration, ductility, softening point, and rotational viscosity, respectively. The isocyanate and polyol reacted to form carbamate in the TPU-modified asphalt, and moreover the isocyanate and aromatic compounds reacted to form halohydrins in the asphalt. Large particles contained in the polyester-based TPU-modified asphalt increased obviously with the increase of Ch (Hard segment content) and r (Isocyanate root index, molar ratio between NCO and OH) value, the fracture mode of it subjected to external load indicated a typical brittle fracture. The content of MDI in the synthesized polyurethane modifier was an important factor affecting the high-temperature stability of the modified asphalt. The polyester-based TPU modifier had a better high temperature performance than the polyether-based TPU modifier. The polyether polyol chain segment showed a greater flexibility as compared to polyester polyol; the addition of polyether polyols can be effectively offset of MDI chain rigidity as r ≤ 1 and Ch < 40% leading to the improvement of the viscoelastic property of asphalt. When Ch = 40% and r = 0.95, the polyether-based TPU-modified asphalt exhibited the same high-temperature grade as the polyester-based TPU-modified asphalt, whereas the polyether-based TPU-modified asphalt showed obviously higher low temperature grades. The polyester-based TPU with Ch = 40% and r = 1 can be used as a modifier to increase the durability of asphalt binders.
Introduction
Over the past 10 years, pavement distress, including rutting, raveling, peeling, and cracking, has been caused due to traffic channelization, heavy load, overload, and so on. Ordinary asphalt and its mixture can no longer meet the requirements of modern transportation and road surface structure, due to the occurrence of such problems. At present, the asphalt binder is usually modified by a polymer to improve the performance of the asphalt binder (Zhang et al., 2018; Xu et al., 2019; Jin et al., 2020; Xue et al., 2020). However, as a result of polymers in storage and use process factors such as heat, light, oxygen, the effect of aging degradation (Cong et al., 2019), and the density of polymer and asphalt, relative molecular mass, solubility, and other large differences, which leads to poor storage stability of polymer-modified asphalt and easy separation, the use of polymer-modified asphalt performance is reduced (Shirzad et al., 2020).
In general, chemically-modified asphalt is different from polymer-modified asphalt, which is modified by adding chemical modifiers under a specific preparation process. The modifier reacts with some active functional groups in asphalt to form new ones. Functional groups change the structure and properties of base asphalt, thereby making up for the deficiency of polymer-modified asphalt (Xing et al., 2020). The chemically-modified asphalt has received more focus than reactive-elastomer-modified asphalt, polyphosphoric-acid-modified asphalt, and epoxy asphalt (Liu et al., 2016; Apostolidis et al., 2018; Athira et al., 2020). Polyphosphoric-acid-modified asphalt has good rutting resistance, anti-aging performance, and storage stability, but after adding polyphosphoric acid the anti-cracking property at low temperatures will be weakened (Liu et al., 2020). Epoxy asphalt has high strength, good toughness, good water resistance, temperature resistance, good fatigue resistance, and so on (Kang et al., 2015; Gong et al., 2019), but due to the high cost, it is mainly used in steel bridge deck pavement engineering (Lu and Bors, 2015). Reactive elastomer can be transformed from chemically reactive by dissolving into hot asphalt. Its preparation technology is simple and can improve the temperature sensitivity, high-temperature stability, and fatigue resistance of asphalt (Cao et al., 2020).
Thermoplastic polyurethanes (TPU) is as an emerging organic polymer material. In terms of molecular structure, TPU is also a kind of block polymer, which is generally composed of a flexible long chain of oligo-polyols to form a soft segment, and a hard segment with diisocyanate and chain extender. The hard and soft segments may alternate arrangement to form the structure of repeating units (Mao et al., 2017). TPU can be used to replace rubber, plastic, and metal because of its light weight, low noise, corrosion resistance, and high economic value. In general, the main types of TPU can be divided into polyester, polyether, polyolefin, etc. In addition, one classification of TPU can be subdivided into 2, 4-tolylene diisocyanate (TDI) and 4,4′-diphenylmethane diisocyanate (MDI) according to the different diisocyanates (Yang et al., 2017). TDI and MDI are very different from each other in the subdivision use due to the differences between the structure and performance, although these are the main fundamental raw materials for synthesizing TPU (Shen, 2020). The molecular structure of MDI exhibits a better symmetry type compared with TDI, on account of the NCO group in the opposite position of two benzene rings of MDI. Moreover, the methylene between both the benzene rings can effectively reduce the abrasion resistance of the benzene rings; therefore MDI displays excellent flexibility and the molecular chain is relatively neat and easy to be crystallized, which makes the synthetic TPU use MDI as the hard segment to show higher modulus and lower mechanical properties. By contrast, the few application of TDI to some extent is due to its extreme toxicity, which is far less extensive than MDI (Zhu et al., 2005). In 1959, MDI was introduced to China (Wu et al., 2019), and its consumption exceeded TDI in 1984 (Parnell and Min, 2005). In recent years, as the global demand for TPU rose, the output of MDI increased rapidly. The products produced based on MDI have penetrated into almost all products in the TPU field, which have met environmental protection requirements. Owing to the TPU synthesized by MDI with excellent comprehensive properties.
The TPU synthesized using MDI (hereinafter referred to as TPU) is divided into two groups: polyester-based TPU and polyether-based TPU. The chemical reaction mechanism of those intermolecular is due to hydrogen bonding or light cross-linking between macromolecular chains, and these cross-linked structures can react reversibly as the temperature increases or decreases. The intermolecular forces will gradually weaken in the molten state, and the strong intermolecular forces will be restored after cooling, which can make TPU recover the properties of the original solid. At the same time, TPU has attracted attention due to its unique properties, such as abrasion resistance, ozone resistance, low temperature resistance, chemical resistance, etc. In view of these characteristics, TPU has been widely used in the fields of national defense technology, electronic technology, engineering construction, medical equipment, etc. (Król, 2007; Pang et al., 2007; Rosu et al., 2010; Li et al., 2012; Barrioni et al., 2015). The composition of TPU includes MDI and small molecule diol (chain extender) to form the hard segment, and polyol to form the soft segment (Liu et al., 2017). In order to develop and utilize TPU fully, the monomer structure, the hard segment content, and the molecular weight can be changed to meet the specification requirements in practical use. On the other hand, the performance of TPU can be modified within a wide range by adjusting the types and ratios of the soft segment, hard segment, and chain extender to make the products multiplicate properties (Zhang et al., 2016). It is very important to determine the optimal hard segment content and the appropriate soft segment structure in the synthesis of TPU, which has great significance for guiding the development of two-component TPU resins with low foaming, high strength, and high modulus, and then those are used for composite materials.
Until now, TPU has been applied in a lot of different fields; a small number of studies had investigated TPU as a polymer modifier to prepare the maintenance materials of asphalt pavement. Moreover, the studies on TPU-modified asphalt are rarely reported in literature, and researchers have not yet made a comprehensive and systematic evaluation, especially in regard to the microstructure and rheological properties of the TPU-composite-modified asphalt that needs to be investigated. Herein, in this study, the preparation process of TPU-composite-modified asphalt was given, and then the base performance tests of prepared modified asphalt with different TPU contents were tested to confirm whether they can meet the technical requirements. The rheological properties of the composite-modified asphalt with different hard segment contents and NCO indexes were analyzed through dynamic shear rheometer (DSR) and bending beam rheometer (BBR) tests, the multiple stress creep recovery (MSCR) test was performed to evaluate the resistance to permanent deformation of the TPU modified asphalt, and then the performance grade (PG) of the TPU-modified asphalt was determined. After that, the effects of polyester-based TPU and polyether-based TPU with different hard segment contents and NCO indexes on the phase microstructures and network structure of modified asphalt was investigated by using Fourier transform infrared spectroscopy (FTIR) to characterize the effects of TPU on chemical compositions and functional groups of asphalt, combined with discussing the compatibility and the reaction mechanism between TPU and base asphalt. Additionally, influences of TPU-modified asphalt on morphology were explored by using scanning electron microscope (SEM) and Fluorescence microscope. Finally, the thermal stability and endothermic and exothermic reactions of the TPU-modified asphalt were analyzed by using differential scanning calorimeter (DSC) and thermal gravity (TG) tests.
Materials and Methods
Materials
Required Materials for Synthesis of Polyurethane
As Shown in Figure 1, the 1,4-butylene adipate (PBA, Mn = 2,000) used in the study was acquired from Yantai Huaxin Polyurethane Co. LTD, China. Polytetramethylene ether glycol (PTMEG, Mn = 2,000), methylene diphenyl diisocyanate (MDI, Mn = 250.25), and 1,4-butylene glycol (BDO, Mn = 90.12) were supplied by Shanghai Aladdin Bio-Chem Technology Co., LTD.
Base Asphalt
The grade of base asphalt labeled as 90# produced at Panjin in China was used in this study, and its technical properties are presented in Table 1.
Explore the Preparation Process
Developed Preparation Method of TPU Modified Asphalt
In this study, the reaction temperature, shear time, and shear revolution were taken as control variables, and the appropriate preparation parameters of TPU-modified asphalt were determined based on the conventional performance test results and the continuous grading temperature. TPU-modified asphalt was prepared by using BME 100L laboratory special shearing equipment as shown in Figure 2. Firstly, the base asphalt was heated to C, and then it was fully mixed with TPU for t1 h through a high-speed shear instrument at S1 r/min. After that, the speed of shear instrument was set as S1 r/min, and the temperature of the composite system was heated to C, and the shear mixing was continued for another t2 h to obtain TPU-modified asphalt. Then the prepared TPU-modified asphalt samples were placed on the sample rack for testing.
Design Scheme and Result Analysis of Orthogonal Experiment
The L9 (34) orthogonal experiment was adopted, the effects of the reaction temperature, shear time, and shear revolution of TPU-modified asphalt were investigated. The specific orthogonal experimental design scheme is shown in Table 2.
The penetration, softening point, ductility, and the continuous grading temperature of the TPU-modified asphalt samples were obtained to evaluate the physical properties of TPU-modified asphalt under different preparation conditions. The test results are shown in Figures 2, 3.
In order to ensure good fluidity and suitability for processing, the initial reaction temperature was set as 145°C. As can be seen from Figure 3, with the increase of reaction temperature, the ductility of the TPU-modified asphalt samples showed a declining trend. The softening point first increased, then decreased, and finally tended to be flat, while the penetration showed no obvious variation.
With the increase of shear time, the penetration of TPU-modified asphalt samples first increased and then decreased, the softening point increased, and the ductility first increased and then decreased, indicating that the shear time was quite short and the modifier had no obvious effect. If the shear time was too long, the hot asphalt would accelerate the aging of asphalt due to its long contact time with oxygen; on the other hand, the refining degree of the modifier would also increase accordingly, which would have an impact on the low temperature resistance ability of modified asphalt.
It was found that when S1 was 3,000 or 5,000 r/min, the performance of the TPU-modified asphalt samples had no significant effect, however when S2 was 5,000 r/min, the reaction temperature increased significantly, and the penetration and ductility of modified asphalt slightly decreased. This indicates that the temperature of asphalt increases rapidly during high-speed shear, and too fast shear speed will lead to a sharp rise in temperature and accelerate the aging of asphalt (Sheng et al., 2018).
As can be seen from Figure 4, the high temperature grading of TPU-modified asphalt raised with the increase of reaction temperature, and then tended to be stable.
Analysis of Gray Incidence
In order to obtain the influence relationship among the three investigation factors, reaction temperature, shear time, and shear revolution, non-dimensional treatment for the experimental data are produced in analysis process.
a. First the reference sequence and the comparison sequence were determined. Corresponding experimental data were extracted from the three factors of reaction temperature: shear time, shear revolution, and their horizontal changes. The analysis indexes included penetration, softening point, ductility, and high temperature continuous grading temperature, which were used as the comparison sequence Xi:
Where, i = 1,2,……,m; k = 1, 2,……, n.
Where, i = 1, 2,……,m; k = 1, 2,……, n.
Where i is the type of influencing factor and k is the level of influencing factors. The mean value method was used as shown in (2.2) to make variables dimensionless, as can be seen in Table 3.
Where, k = 1, 2,……, n.
Take {x0} = {59 62 54 79} for reference date.
b. According to Formula (2.4), the absolute difference between the data column of each evaluated object and the reference data column is calculated one by one, as shown in Table 4.
c. According to the difference sequences the maximum and the minimum difference between the poles was written as:
Where, i = 1, 2,……, m; k = 1, 2, ……, n.
d. The correlation coefficient and correlation degree were calculated, as shown in Table 7.
The correlation coefficient:
Where, ρ = 0.5, i = 1, 2,……,m; k = 1, 2,……, n.
The correlation degree:
Where, i = 1, 2,……, m.
e. As Shown in Table 5, order of correlation degree: γ2>γ3>γ9>γ6>γ4>γ1>γ7> γ8>γ5.
To sum up, when T1 and T2 were 145 and 150°C, t1 and t2 were 1 h and 0.5 h, S1 and S2 were 3,000 and 3,000 r/min, respectively, and the performance of the TPU-modified asphalt prepared was optimum.
The Equation Design of Polyurethane
The TPU can be plasticized by heating and the solvent can be dissolved. In the TPU molecular structure, the soft segment with a large proportion accounts for 50 to 90%, and the hard segment accounts for 10 to 50%. There are many kinds of raw materials for synthesizing TPU, and the chemical reactions involved are complicated. The quality guarantee of TPU comes from strict raw material ratio control (see Figure 5).
Usually, the hard segment of TPU chooses diisocyanates with cyclic, tight, symmetrical cores to make its particles dense with each other, and then the physical properties can be enhanced. Therefore, the methylene diphenyl diisocyanate (MDI) was chosen as a hard segment in this study. The molecular weight of the oligomeric diol used in the soft segment is generally 500–4,000 (1,000–2,000 is usually selected), hence the polytetramethylene ether glycol (PTMEG) and the poly (1,4-butylene adipate) (PBA) were used as the soft segment of TPU, respectively. Chain extenders with small molecular weights and no substituents (generally lower glycols are preferred) facilitate the aggregation of hard segments and urethane groups. Consequently, 1,4-butanediol was selected as the chain extender (BDO).
The hard segment content (Ch) is the mass fraction of hard segment in TPU, and the Ch is the mass sum of diisocyanate and small molecule diol. The isocyanate index (r) refers to the equivalent number ratio of diisocyanate to the sum of the oligomeric diol and small molecule diol, or the ratio of NCO/OH. The four raw materials used in this study were difunctional groups, then NCO/OH is also a molar ratio, written by:
Combining with the Equations (2.9, 2.10) to obtain Equation (2.11):
Where, Wi, Wd—the mass of MDI, BDO, and PBA/PTMEG, Mi, Md, and Mg—are the relative molecular mass of MDI, BDO, and PBA/PTMEG, Ch—is the hard segment content in TPU, and r—is the isocyanate root index.
The reaction mechanism of the TPU can be seen in Figure 6. PBA or PTMEG with a molecular weight of 2,000 were selected as the soft segment of the TPU, the hard segment content was designed to be 20, 30, and 40%, and the r was controlled 0.95, 1, and 1.05, respectively. The amount of PBA/PTMEG was 100g and the mass of BDO and MDI was calculated according to Equations (2.10, 2.11), respectively. The 18 kinds of synthetic TPUs are shown in Tables 6, 7.
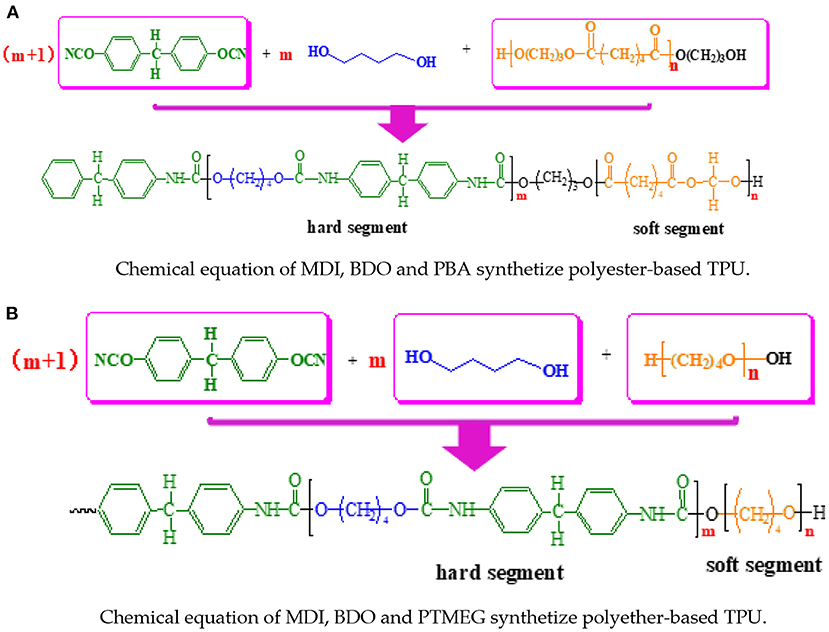
Figure 6. Reaction mechanism of the TPU. (A) Chemical equation of MDI, BDO, and PBA synthetize polyester-based TPU. (B) Chemical equation of MDI, BDO, and PTMEG synthetized polyether-based TPU.
Experimental Methods
Physical Performance Tests
In order to comprehensively evaluate the performance of TPU-modified asphalt, the conventional performance test results of asphalt were taken as the control index, and five repeated tests were conducted for each test. In this study, the conventional physical properties of the prepared TPU-modified asphalt were tested, including penetration (25°C), ductility (5°C), and softening point tests according to technical requirements of polymer-modified asphalt in Standard Test Methods of Bitumen and Bituminous Mixture in Highway Engineering (JTG E20-2011) in China
The penetration (25°C) is a method to determine the consistency of asphalt and also shows the rheological properties of the asphalt investigated. The formula of the penetration index of asphalt calculated from the value of penetration (25°C) and softening point is as follows:
Where lgP25 — Penetration index; TR&B — Softening point.
The rotary viscosity of asphalt was tested by Brookfield viscosity meter, the viscosity test for asphalt was performed at 135 and 175°C, respectively, and the experimental method also can be found in JTG E20-2011.
Microscopic Morphology Analysis
(1) FTIR test
The functional groups of reaction mixture characteristic groups were identified by FTIR characteristic adsorption peaks, which were tested by IRT-100 infrared spectrometer (SHIMADZU, Japan), ATR mode. The spectra were collected through 32 scans with a spectral resolution of 4 cm−1 for a spectral range from 400 to 4,000 cm−1.
(2) Fluorescence microscope
Fluorescent inverted microscope (BX41, Olympus Corporation, Japan) was used to explore the microstructure characteristics of modified asphalt. The polymer modifier was used to distinguish the polymer phase from the asphalt phase by the difference of the wavelength range of the light emitted by the polymer modifier under the irradiation of the fluorescent light source; in turn, the real phase structure of the polymer phase in asphalt was observed.
(3) SEM test
As shown in Figure 5, SEM (SUPRA 55 SAPPHIRE instrument, ZEISS Co., Germany) analysis equipment was used to visually characterize the compatibility between the TPU modifier and asphalt binder. The SEM test was employed to obtain a microscopic image of the cross sections of the TPU-modified asphalt magnified 500 and 5,000 times. As the asphalt material is not conductive, all samples were fixed on an aluminum sample stub and sputtered with gold under vacuum conditions (JFC-1100 model instrument, JEOL Co., Japan). Then the sample chamber was opened to place samples. Finally, the microstructure characteristics of the samples were observed by using SEM.
(4) TG test
TG (Q 50 instrument, TA Co., United States) analysis was used to characterize the impact of the thermal stability of TPU-modified asphalt. The mass of each sample was between 3mg and 5mg, and then the samples were heated from room temperature to 800°C at a rate of 20°C/min using nitrogen as the protection gas.
(5) DSC test
A DSC (Q 20 instrument, TA Co., United States) was used to analyze the thermal stability of TPU-modified asphalt. A scale (1/10,000 g) was adopted to measure the weight due to the high precision requirement of the sample that is not more than 5±1 mg. Using nitrogen as the protection gas, the sample was heated from room temperature to 130°C at a heating rate of 10°C/min, kept at a constant temperature for 2 min, then cooled to 0°C at a rate of 10°C/min. It was then kept at a constant temperature for 1 min after rising to 150°C at a heating rate of 10°C/min, and then the temperature DSC curves were obtained. The glass transition was analyzed by the DSC testing results; the glass transition temperature (Tg) can be obtained by the DSC testing curve of TPU-modified asphalt, and the DSC testing curve for glass transition is illustrated in Figure 7.
Rheological Properties
(1) DSR test
The PU-composite-modified asphalt was measured using a DSR Gemini II ADS machine according to AASHTO T 315. The high temperature performance of modified asphalt was evaluated by calculating the complex shear modulus (G*), phase angle (δ), and rut factor (G*/sinδ). In this study, the DSR test was mainly carried out in the temperature sweep mode. In the temperature sweep analysis, the test temperature was set at 46, 52, 58, 64, 70, and 76°C, respectively. Using strain control mode, the strain was 12% and the loading frequency was set at 10 rad/s.
(2) BBR test
To evaluate the low-temperature performance of the PU-composite-modified asphalt, the BBR manufactured by Cannon Instrument Company was used to measure the creep stiffness (S) and creep rate (m-value) at low temperatures based on the ASTM D 6,648 and AASHTO T 313. The testing temperature were chosen respectively at −10, −16, −22, −28, and −34°C.
Moreover, the rheological tests were employed on the TPU-modified asphalt to determine the high and low temperature performance grades (PG).
(3) MSCR test
In this study, the multiple stress creep recovery (MSCR) test was used to evaluate the anti-permanent deformation ability of the TPU-modified asphalt. According to AASTO TP70-09, the percent recovery (R) reflecting the elasticity of the asphalt binder and non-recoverable creep compliance (Jnr) were calculated as a measure of the binder's contribution to permanent deformation behavior. Firstly, the composite modified asphalt samples were aged through RTFOT and then the stress-recovery control mode of DSR was conducted for 1s loading time, 9sunloading time, and a testing temperature of 64°C. During the test, the stress was applied at 1.0 kPa and repeated 10 times, then the stress was increased to 3.2 kPa and repeated 10 times.
As shown in Figure 8, in the MSCR test, two key parameters can be obtained: R and Jnr were calculated by using Equations (2.13, 2.14), respectively (Wang et al., 2017).
In addition to the irrecoverable creep compliance and strain recovery ratio, stress sensitivity is also an important parameter to describe the results of the MSCR test, which can be described by the relative differences of strain recovery ratio and unrecoverable creep compliance, namely Rdiff and Jnr−diff [see Equations (2.15, 2.16)], respectively. Especially for elastomer-modified asphalt, this parameter is of great research significance.
Results and Discussion
Conventional Physical Properties of Polyurethane Modified Asphalt
Influence of Ch and Isocyanate Root Index (r)
The influence of polyester-based TPU and polyether-based TPU-modified asphalt are shown from Figures 9–11; as can be seem the test results of all modified asphalt samples prepared met the technical requirements of polymer-modified asphalt in China based on the Standard in China (JTG E20-2011) (Sun et al., 2018). It should be emphasized that the base asphalt was simplified as base asphalt (BA) in the above figures.
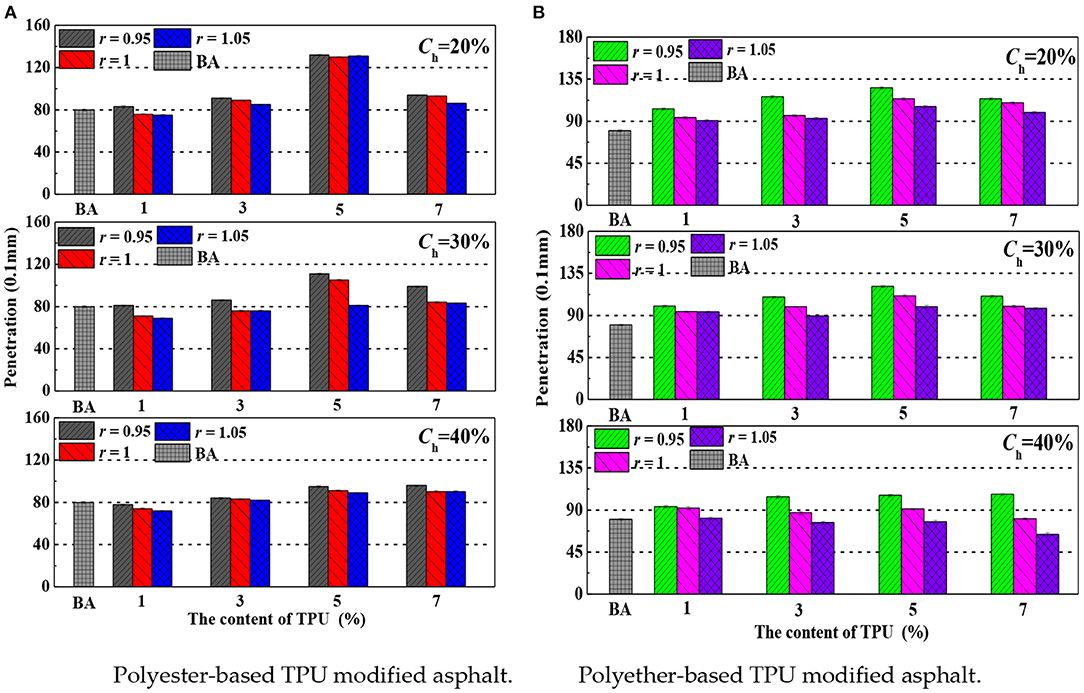
Figure 9. The penetration of modified asphalt. (A) Polyester-based TPU-modified asphalt. (B) Polyether-based TPU-modified asphalt.
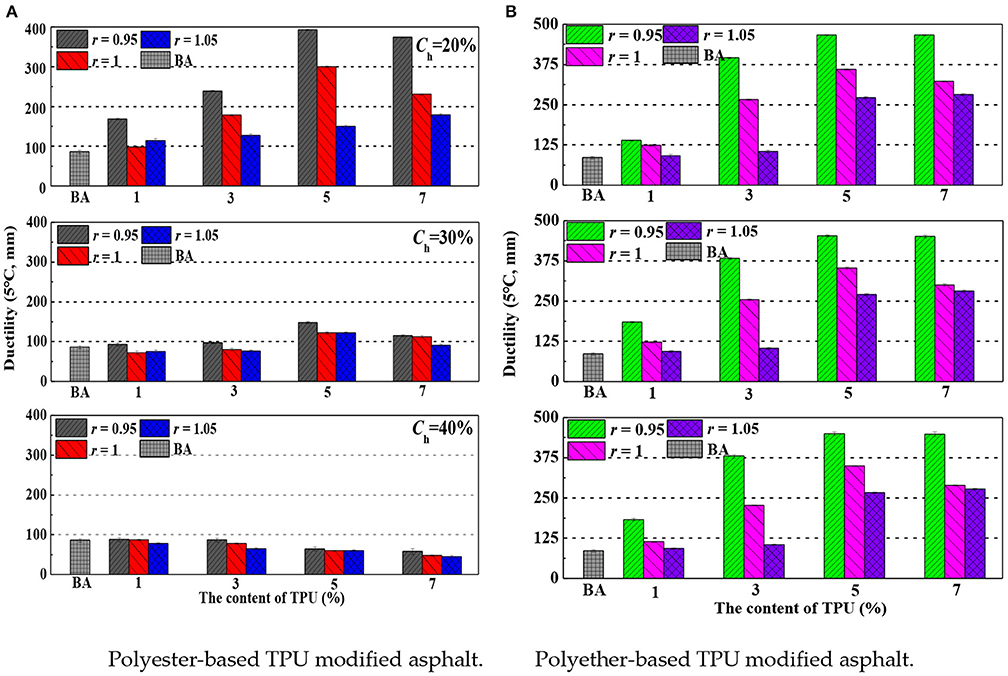
Figure 10. The ductility of modified asphalt. (A) Polyester-based TPU-modified asphalt. (B) Polyether-based TPU-modified asphalt.
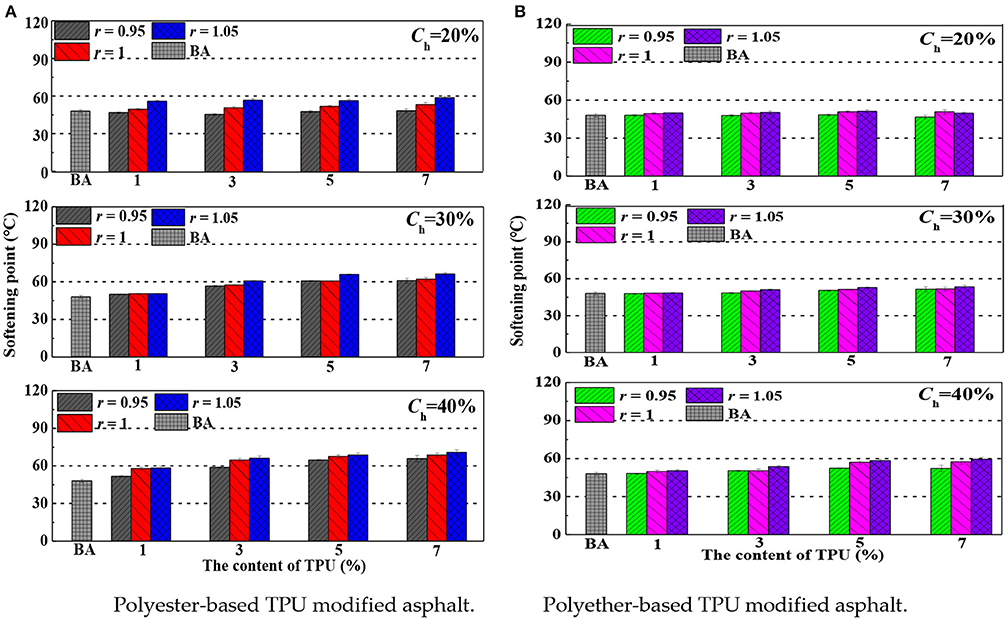
Figure 10A. The softening point of modified asphalt. (A) Polyester-based TPU-modified asphalt. (B) Polyether-based TPU-modified asphalt.
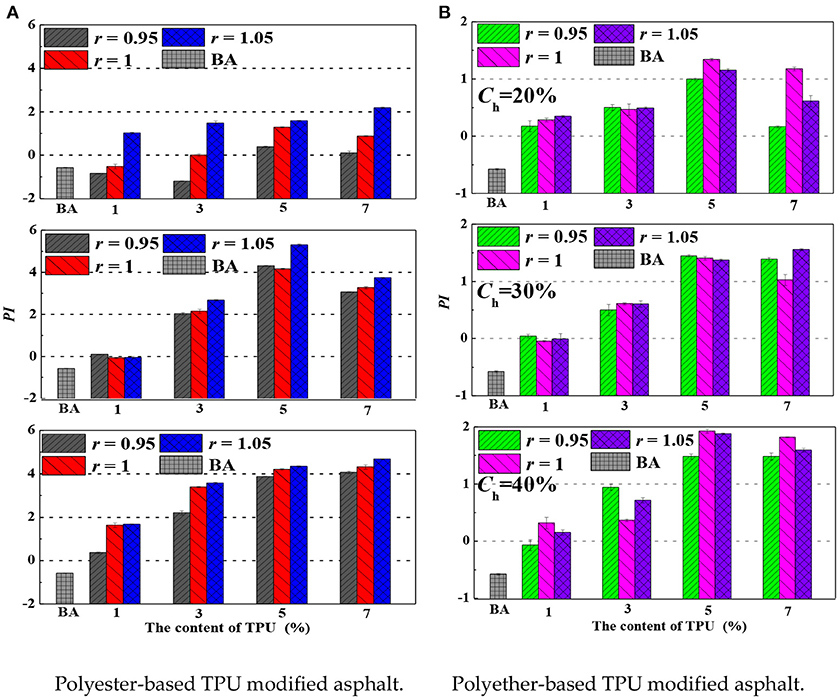
Figure 11. The penetration index (PI). (A) Polyester-based TPU-modified asphalt. (B) Polyether-based TPU-modified asphalt.
The penetration is not only a method to determine the consistency of asphalt, but also a reflection of the rheological properties of asphalt for road petroleum asphalt with medium and low traffic volume. When r value and Ch were constant, with the content of polyester-based TPU increasing, the penetration first increased and then started to flatten out, when the amount of modifier and Ch remained unchanged, the penetration gradually decreased with the increase of r value. When there was an identical amount of modifier and the same r value, the penetration decreased with the increase of Ch content. However, the penetration of polyether-based TPU-modified asphalt had the same changing trend as the polyester-based TPU-modified asphalt, nevertheless the penetration of polyether-based TPU-modified asphalt was slightly higher. The results showed that polyester-based TPU-modified asphalt had a better high-temperature performance than polyether-based TPU-modified asphalt. For the TPU, which was composed of MDI, both the Ch and r value increase can enhance the hardness of modified asphalt. Accordingly, this improves the high-temperature deformation resistance of base asphalt.
The low temperature elongation of modified asphalt can be characterized using the 5°C ductility, and then the low temperature crack resistance of modified asphalt can also be reflected. The greater the 5°C ductility, the better the low temperature crack resistance of modified asphalt will be. When r value was identical and the Ch was fixed, the 5°C ductility first increased and then decreased with the polyester-based TPU content increasing; when the amount of modifier and Ch remained unchanged, the 5°C ductility gradually decreased with the increase of r value. When the same addition of modifier and r value was certain, the ductility at 5°C also decreased with the increase of Ch.
By contrast, the 5°C ductility of polyether-based TPU had little change, which is different from polyester-based TPU-modified asphalt. The main reason is that the increase of polyester-based TPU content changed the viscoelastic and elastic ratio of matrix asphalt, accelerated the phase transition of asphalt components, and increased the glass transition components, which made the asphalt molecular chains more prone to fracture.
The softening point represents the malleable fluidity of asphalt and reflects the high-temperature stability of asphalt. When r value and Ch were constant, the softening point increased gradually with the increase of polyester-based TPU content; when the addition amount of modifier was constant and Ch was the same, the softening point increased slightly with r value increasing. When the amount of modifier was unchanged with a certain content of r value, the softening point tended to rise with the increase of Ch content. For polyether-based TPU, the content of modifier has a limited effect on softening point, but with the increase of r value and Ch, the softening point of base asphalt gradually increased which indicated that in addition to the amount of polyester-based TPU increasing, the original compatibility of based asphalt was changed. Namely, polyester polyol had greater cohesion energy and higher viscosity after heating and melting, which will further improve the viscosity of asphalt, limit the mobility of asphalt, and enhance the thermal stability of modified asphalt. Therefore, the softening point showed an increasing trend, which proved that polyester-based TPU can effectively improve the high-temperature performance of based asphalt. The softening point of polyether-based TPU had a limited affected on Ch and r value due to the polyether-based TPU with weak polarity; the molecular weight of the soft segment increasing, namely the ether group increasing, also offset the influence of soft segment increasing to the hard segment declining on temperature. Therefore, it can be seen that polyester-based TPU has better high-temperature stability.
It can be seen from Figure 11A that the PI index of polyether-based TPU-modified asphalt ranged from −2 to +2, and its colloidal type is Sol-Gel (Chen, 2003). This type of asphalt shows a certain resilience, and its type of modified asphalt has a certain thixotropy. However, its colloidal structure type is easily affected by temperature, as the high temperature area above the softening point may be gelatinous, which proved that the modified asphalt has non-Newtonian (composite) flow characteristics. This means that the colloidal structure type PI of polyether-based TPU-modified asphalt will change with the change of temperature. When the Ch of polyester-based TPU was 20 or 30%, as the blending amount was >3%, the modified asphalt of PI > +2. At this time, the asphalt type belonged to Gel type, and the elastic effect of this kind of asphalt was greater, which shows typical thixotropic characteristics.
Asphalt is a temperature-sensitive material with different properties at different temperatures. The less sensitive the asphalt on temperature, the larger its PI. As shown in Figure 11B, the PI of polyester-based TPU-modified asphalt increases with the increase of r value, modifier content, and Ch. When the Ch > 20% and the modifier content was higher than 3%, the type of modified asphalt changed from Sol-Gel type to Gel type. When the modifier was increased, the PI of polyether-based TPU-modified asphalt first increased and then decreased, and the effect of r value on PI was irregular. With the increase of Ch, the PI of polyester-based TPU-modified asphalt slightly increased, but the addition of polyether-based TPU could not change the type of modified asphalt. Although polyester-based TPU can better reduce the temperature sensitivity of asphalt, it was also found that polyether-based TPU is more suitable as a modifier of asphalt through comparison. Because it is different from gelatinous asphalt, polyester-based TPU-modified asphalt is an intermediate type between Sol asphalt and gelatinous asphalt. Asphaltene content is relatively moderate, and the content of colloid forms micelles were suitable, but dispersed in the oil medium which has elasticity, and has no obvious yield point.
As illustrated in Figure 11, the viscosity of polyester-based TPU-modified asphalt is consistent with that of polyether TPU-modified asphalt. The viscosity of Ch and modifier content both increased with the increase of r value. Compared with polyester-based TPU-modified asphalt, the viscosity of polyether TPU-modified asphalt was not obviously increasing. It was also in line with the Technical Specifications for Construction of Highway Asphalt Pavements (JTG F40-2004) in China and SHRP standards. It was found that the test results of the rotary viscosity of the polymer modified asphalt generally did not exceed 3 Pa·s at 135°C (China Communications Press, 2011).
According to the data in Figure 12, both types of modified asphalt met the specification requirements. This was mainly that the modified asphalt with the above formula had strong resistance to flow deformation when subjected to external load. It is found that polyester-based TPU increased the internal intermolecular friction resistance of asphalt as it flowed. Although polyether-based TPU also increased the internal intermolecular friction resistance of asphalt when it flowed to a certain extent, it is not as effective as polyester-based TPU in improving the high temperature performance. In addition, it was also found that the viscosity of polyester-based TPU-modified asphalt and polyether-based TPU-modified asphalt decreased with the temperature increasing. At the same temperature, polyester-based TPU-modified asphalt had higher viscosity than polyether-based TPU-modified asphalt, and the viscosities had a great difference between each other at 135°C. Both of the modified asphalts mitigated the difference of viscosity at 175°C which indicated that polyester-based TPU-modified asphalt had a better temperature sensitivity. However, due to the viscosity temperature characteristics of the high temperature section having an important impact on the pumping performance of asphalt and the mixing, rolling, and other construction performance of the mixture, the asphalt must have an appropriate viscosity in the range of service temperature. Polyether-based TPU is more suitable to be used as the modifier of asphalt, considering the factors of mixing, transportation, pumping, and construction workability.
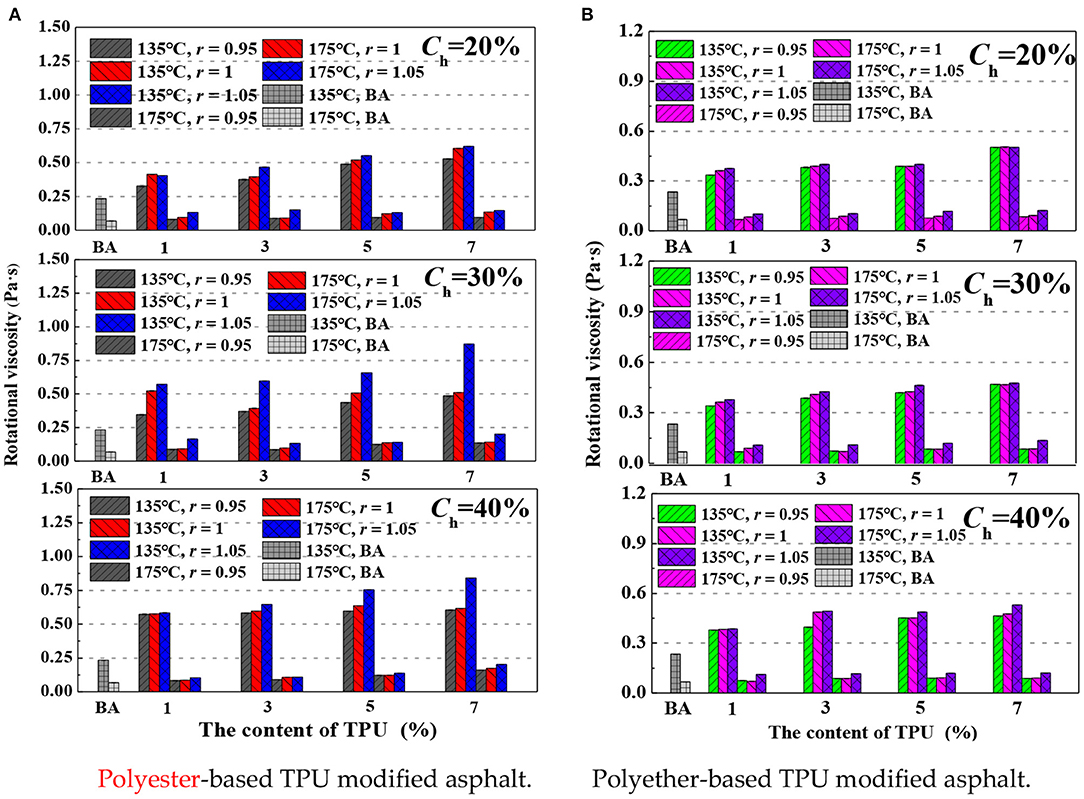
Figure 12. The rotational viscosity. (A) Polyester-based TPU-modified asphalt. (B) Polyether-based TPU-modified asphalt.
Influence of Molecular Weight
TPU is a linear molecule, and molecular weight is a key index of material performance. The performance indexes of polyester-based TPU-modified asphalt with different molecular weights are shown in Figure 13. With the molecular weight of TPU increasing, the penetration and ductility of polyester-based TPU-modified asphalt first increased and then decreased. However, the softening point rose first, then decreased, and then continued to rise. PI showed a trend of first rising and then falling.
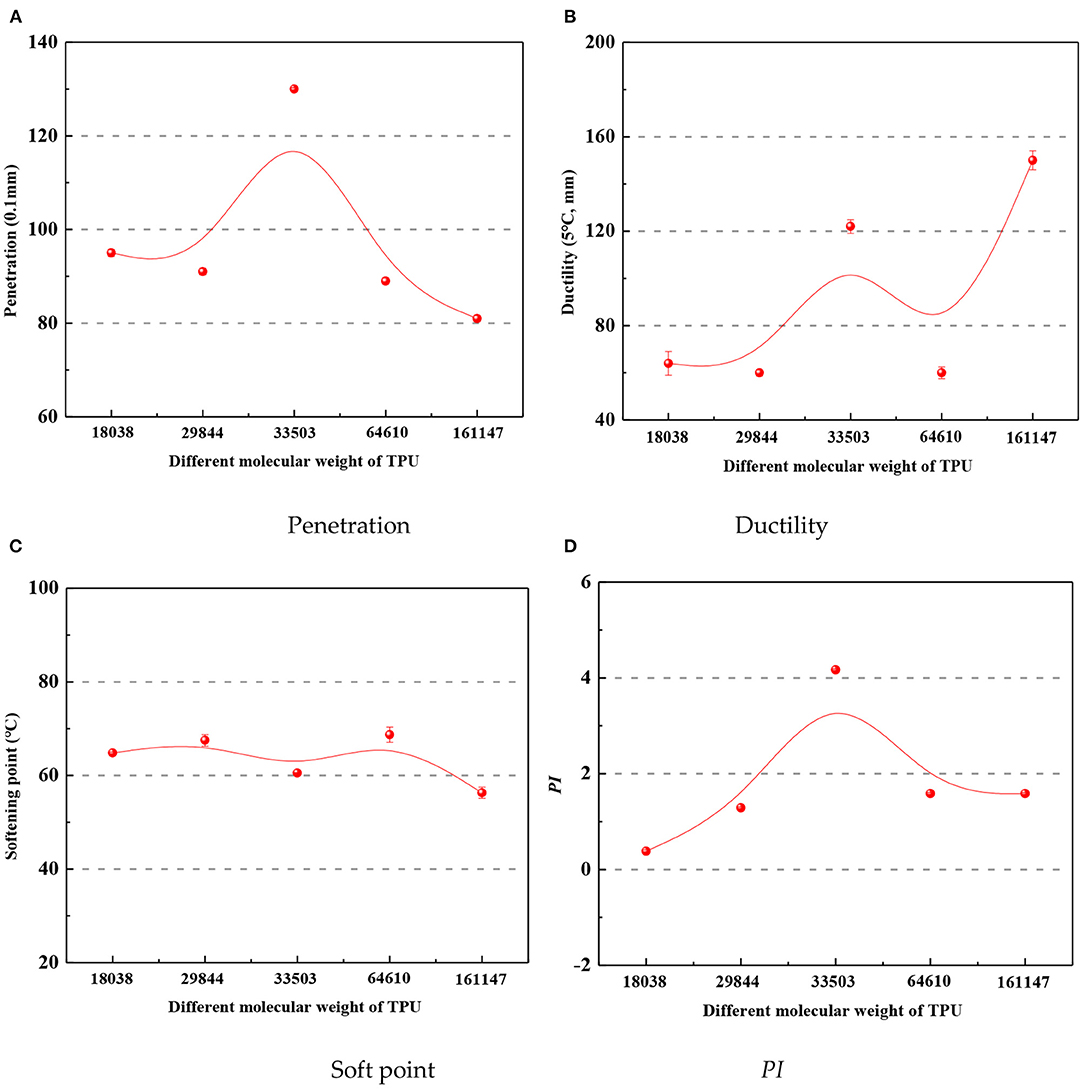
Figure 13. Polyester-based TPU-modified asphalt. (A) Penetration. (B) Ductility. (C) Soft point. (D) PI.
The performance indexes of polyether-based TPU-modified asphalt with different molecular weights are shown in Figure 14. As the molecular weight of TPU increased, both the penetration and ductility of polyether-based TPU-modified asphalt first increased and then decreased. The softening point first decreased, and then increased. PI declined and then increased.
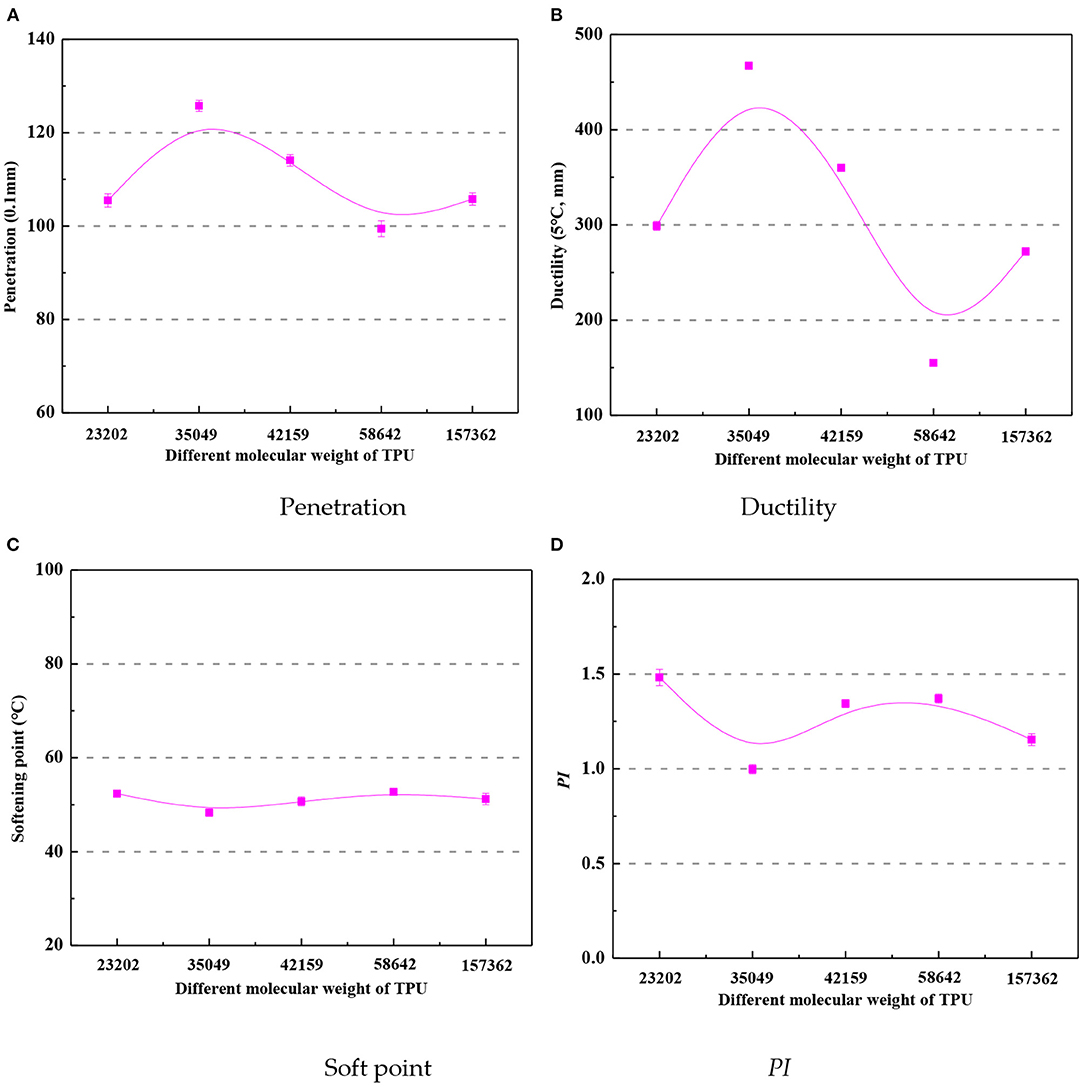
Figure 14. Polyether-based TPU-modified asphalt. (A) Penetration. (B) Ductility. (C) Soft point. (D) PI.
The rotational viscosity results of polyester-based TPU and polyether-based TPU modified with different molecular weights are shown in Figure 15. With the increase of molecular weight, the rotational viscosity of polyester-based TPU-modified asphalt had no corresponding change rule at 135°C. The maximum viscosity of polyester-based TPU-modified asphalt with the molecular weight of 64,610 was 0.754Pa·s. There is also no corresponding change rule of polyether-based TPU-modified asphalt on rotational viscosity at 135°C. The maximum viscosity of polyester-based TPU-modified asphalt with the molecular weight of 58,642 was 0.463 Pa·s. At 175°C, the viscosity of polyester TPU and polyether TPU-modified asphalt increased gradually with the increase of molecular weight, but the change was not obvious.
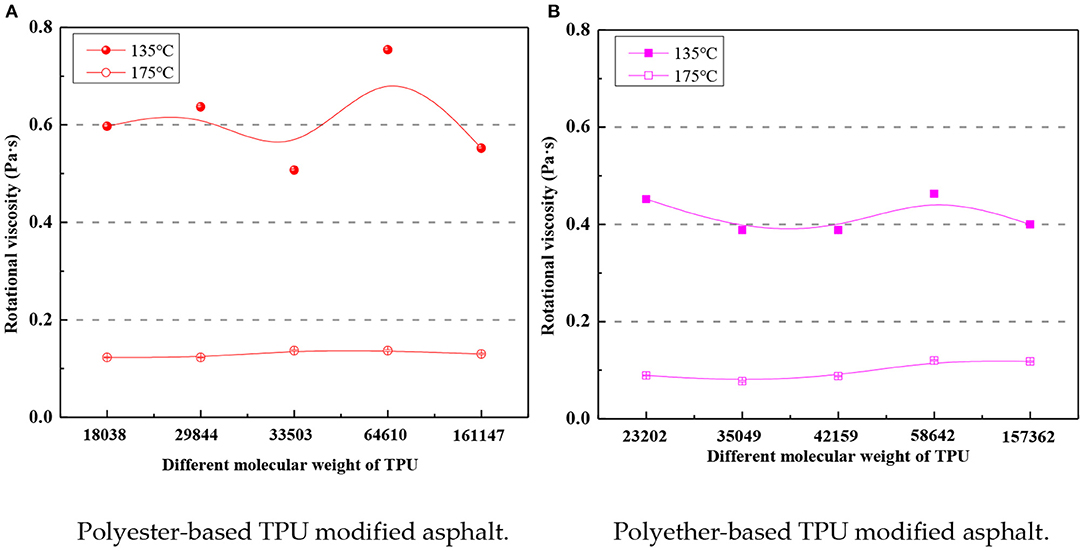
Figure 15. Rotational viscosity. (A) Polyester-based TPU-modified asphalt. (B) Polyether-based TPU-modified asphalt.
Overall, the physical properties of polyester-based TPU and polyether-based TPU-modified asphalt had no obvious effect on the molecular weight. This may be because the modifier was fully sheared and had a high degree of refinement in the preparation process after the modifier was sheared at high speed by the shearing machine in the preparation process, and the TPU macromolecular chain segment was destroyed (Yu et al., 2018).
Chemical Characterization of TPU Modified Asphalt
The influences of Ch and r value on the micro morphology of the polyester-based TPU and polyether-based TPU-modified asphalt were investigated to reveal the micro-mechanism of the TPU modified asphalt. For the understanding and direct characterization of the micro-structure of the composite-modified asphalt with different dosages of modifier, the amount of TPU was chosen as 5%.
FTIR
The infrared spectrum of base asphalt and modified asphalt is shown in Figure 16A, and the detailed overview of the characteristic peak of infrared spectrum of base asphalt is shown in Table 8.
The characteristic groups in the TPU molecules are ammonia ester group, hydrocarbon group, phenyl group, ester group, ether group, hydroxyl group, isocyanate ester group, urea group, amide group, biuret group, allophanate, etc. (Liu, 2012; Alamawi et al., 2019; Xu et al., 2019). The FTIR results of polyester-based TPU and polyether-based TPU is shown in Figure 16B. There is no difference in peak shape, peak size, and peak outlet position of all samples; the broad absorption band is centered at ~3,343 cm−1, which was caused either by —OH or —NH stretching. The strong stretching in the C—H bond of CH3 or CH2 occurred at frequencies between 2,917 and 2,847 cm−1 which were due to asymmetric stretching vibration and symmetric vibration. Many weak peaks from 2,159 to 2,023 cm−1 were the interference peaks of CO2 in the air. The absorption peak at 1,717 cm−1 was —NHCOO—, the absorption peak at 1,457–1,580 cm−1 can be assigned to the stretching vibration of C=O in ammonia ester group, urea group, or amide group. 1,375 cm−1 was the absorption peak of C—O bond or the hydroxyl group connected to C in ester group. A wide absorption peak at 1,126 and 1,106 cm−1 correspond to the vibration mode of S=O bond compound. In the fingerprint region, the absorption peak between 500 and 900 cm−1 were assigned to the vibration of H in the aromatic ring in isocyanate.
The FTIR results of polyether-based TPU-modified asphalt are shown in Figure 17A; the characteristic peak at 3,362 cm−1 was the characteristic absorption peak of —NH (free NH stretching band) or the overlap of the —OH. The wide absorption peaks at 2,918 and 2,847 cm−1 were due to the asymmetric stretching vibration or the symmetric vibration of CH3 or CH2. The strong peak at around 3,078 cm−1 was the characteristic absorption peak of —NH (secondary amine). Some weak peaks between 2,358 and 2,341 cm−1 were due to the related vibration peak of CO2 in the air which belonged to the interference peak. The absorption peak at 1,729 cm−1 was —NHCOO—, at 1,729 cm−1 was C=O stretching. The peak near 1,150 cm−1 was the absorption peak of —O— (ether group) in ammonia ester group. The absorption peak near 1,109 cm−1 was produced by S=O bond compound. In the fingerprint region, the vibration of benzene ring in isocyanate was the absorption peak in the range of 500–900 cm−1.
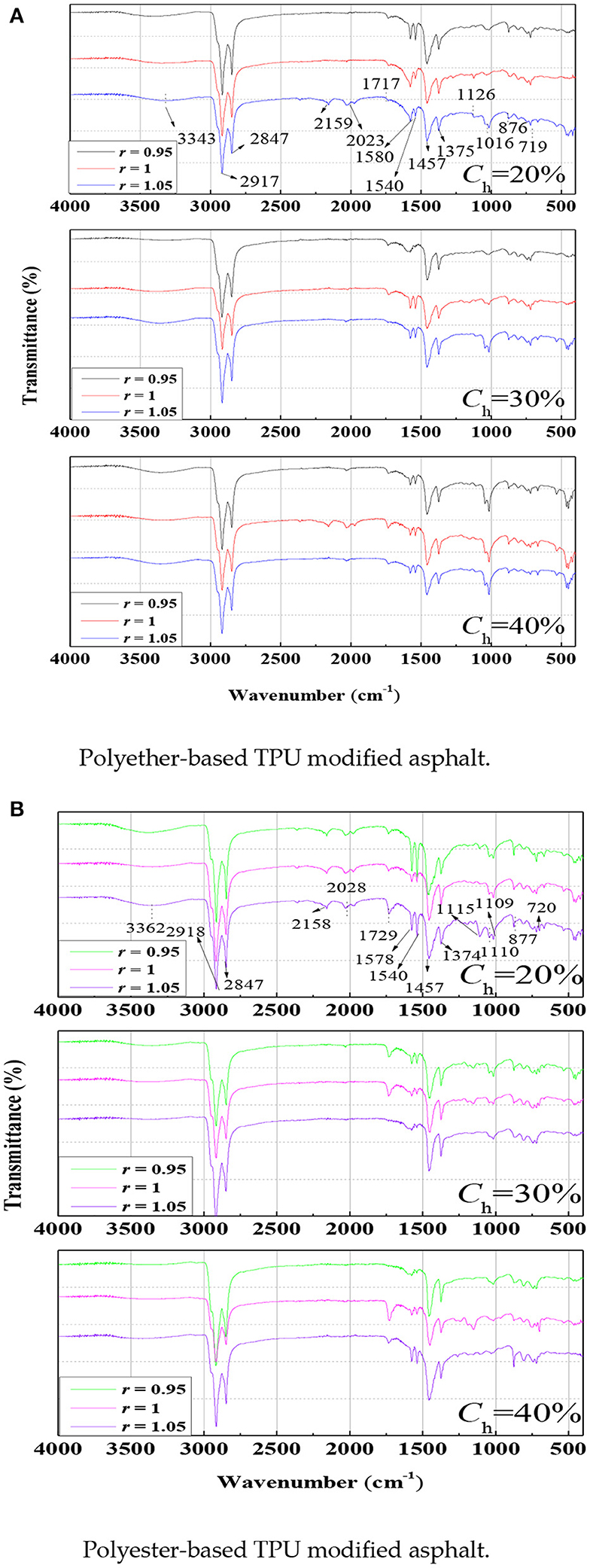
Figure 17. FTIR of different modified asphalt. (A) Polyether-based TPU-modified asphalt. (B) Polyester-based TPU-modified asphalt.
Combined with the base performance tests results, it can be concluded that the formation and structure of polyester-based TPU-modified asphalt (see Figure 17B) and polyether-based TPU-modified asphalt in asphalt had an extremely important influence on the properties of the modified asphalt. The curves of polyester-based TPU modified asphalt and polyether-based TPU-modified asphalt from 3,343 to 2,847 cm−1 were similar but distinct from base asphalt which indicates that the characteristic peak was generated by modified asphalt. The characteristic absorption of ester group is at 1,375 cm−1 for polyester-based TPU-modified asphalt. And the absorption peak of polyether-based TPU of —O— (ether group) in ammonia ester group was near 1,150 cm−1. By comparing the characteristic peaks of polyester-based TPU and polyether-based TPU, it was found that the characteristic peaks of TPU modified asphalt had changed a lot. The most prominent one was the characteristic peak of —NHCOO— at 1,717 and 1,729 cm−1 in the FTIR curve of polyester-based TPU-modified leachate and polyether-based TPU-modified leachate. In the synthesis process of TPU modifier, -NCO group itself is excessive, but there is no obvious isocyanate characteristic peak in the FTIR of TPU-modified asphalt, then a new -NHCOO characteristic peak appears, which indicated that both polyester TPU and polyether TPU can react with some active functional groups in base asphalt, and new compounds are generated (Sun et al., 2018). The main reactions in TPU-modified asphalt were the isocyanate and polyol to form carbamate, furthermore, the isocyanate and aromatic compounds to form halohydrins in the asphalt by an addition reaction. There are mainly two reactions in the TPU-modified asphalt. One is the reaction between the isocyanate and polyol to form carbamate, the other is the addition reaction between the isocyanate and aromatic compounds in the asphalt (Sun et al., 2019).
Fluorescence Microscope
The morphology, phase, and structure of polyester-based TPU and polyether-based TPU in asphalt were observed by fluorescence microscope with the purpose to evaluate the mechanical properties of the two modified asphalt more effectively, and thus to establish the relationship between the microstructure and the macroscopic mechanical properties of polyurethane modified asphalt.
Figure 18 shows the distribution of polyurethane in the base asphalt was clearly observed by fluorescence microscope, and the polyurethane modifier and asphalt were two-phase continuous structures. The distribution area of the modifier increased gradually along with the content of TPU; it was also observed that the polyester-based TPU had a homogeneous distribution of large and small particles in the base asphalt, modifier swelling effect and good dispersion, asphalt polyurethane with each other, forming a mixture of space network structure, which belongs to the typical two phases structure in a row. Thus, the rationality of the preparation process is proved.
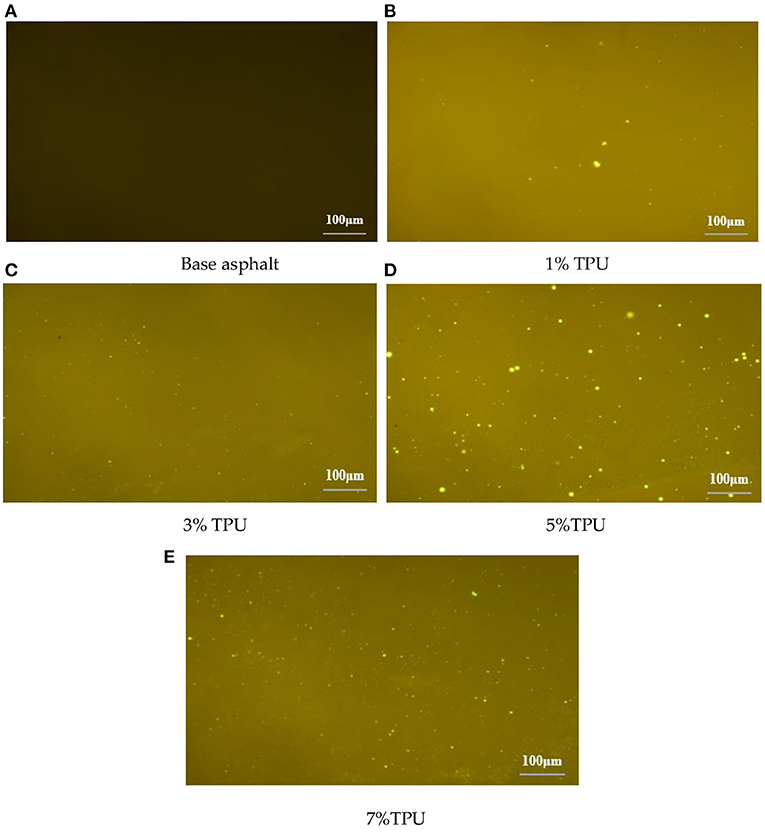
Figure 18. Fluorescence micrographs of polyester-based TPU-modified asphalt. (A) Base asphalt. (B) 1% TPU. (C) 3% TPU. (D) 5%TPU. (E) 7%TPU.
It can be seen from Figure 19 that the polyurethane modifier is uniformly dispersed in asphaltenes without obvious agglomeration distribution. With the increase of hard segment content and r value, the refinement degree of polyester-based TPU modifier particles decreased, while the large particles increased significantly, which indicated that with the increase of hard segment content and r value, the adhesion between polyester-based TPU molecules was far greater than the penetration force of asphalt, and particle agglomerates were free in asphalt. On the other hand, polyester-based TPU surface can strengthen the tendency of asphalt light components to move closer to polyester-based TPU particles and shrink into a relatively stable spherical energy state with the increase of temperature which has better high temperature performance.
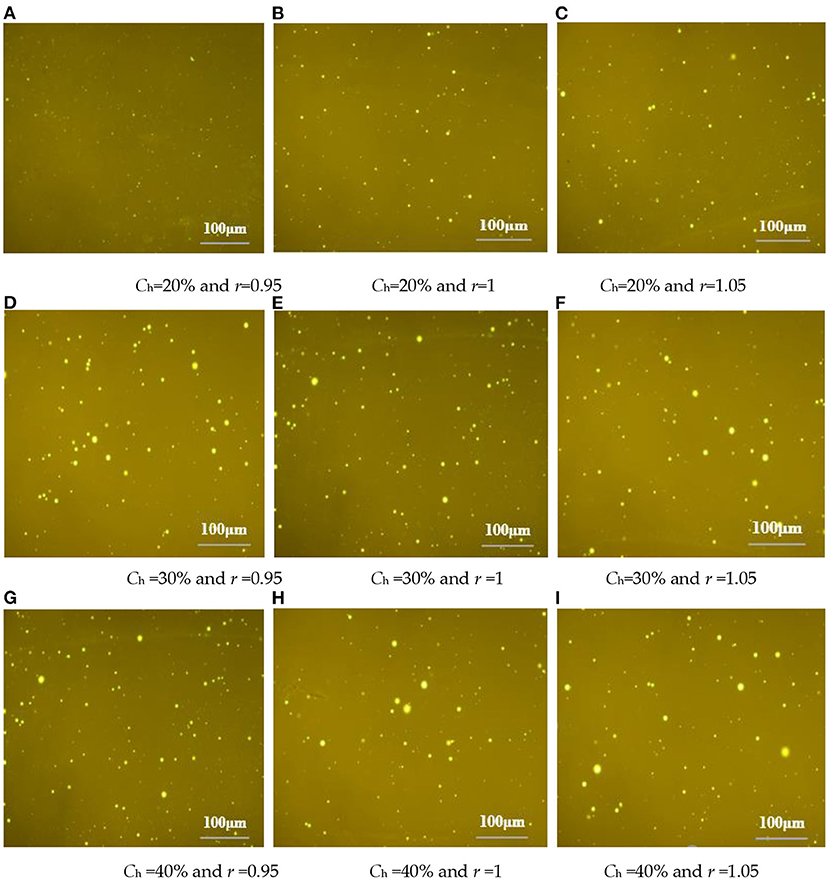
Figure 19. Fluorescence micrographs of polyester-based TPU-modified asphalt. (A) Ch = 20% and r = 0.95. (B) Ch = 20% and r = 1. (C) Ch = 20% and r = 1.05. (D) Ch = 30% and r = 0.95. (E) Ch = 30% and r = 1. (F) Ch = 30% and r = 1.05. (G) Ch = 40% and r = 0.95. (H) Ch = 40% and r = 1. (I) Ch = 40% and r = 1.05.
As can be seen from Figure 20, which is similar to polyester-based TPU modified asphalt, the distribution area of the modifier in polyether-based TPU-modified asphalt also increased with the increase of the modifier content. However, with the increase of the content of polyether-based TPU, the small particles that were originally uniformly dispersed were aggregated into relatively large clusters. It is proven that the organic molecular chain in the modifier can be embedded into the layered structure, which can promote the formation of the cross-linked structure in polyether-based TPU, so as to improve the low temperature resistance of the asphalt binder (Gallu et al., 2020).
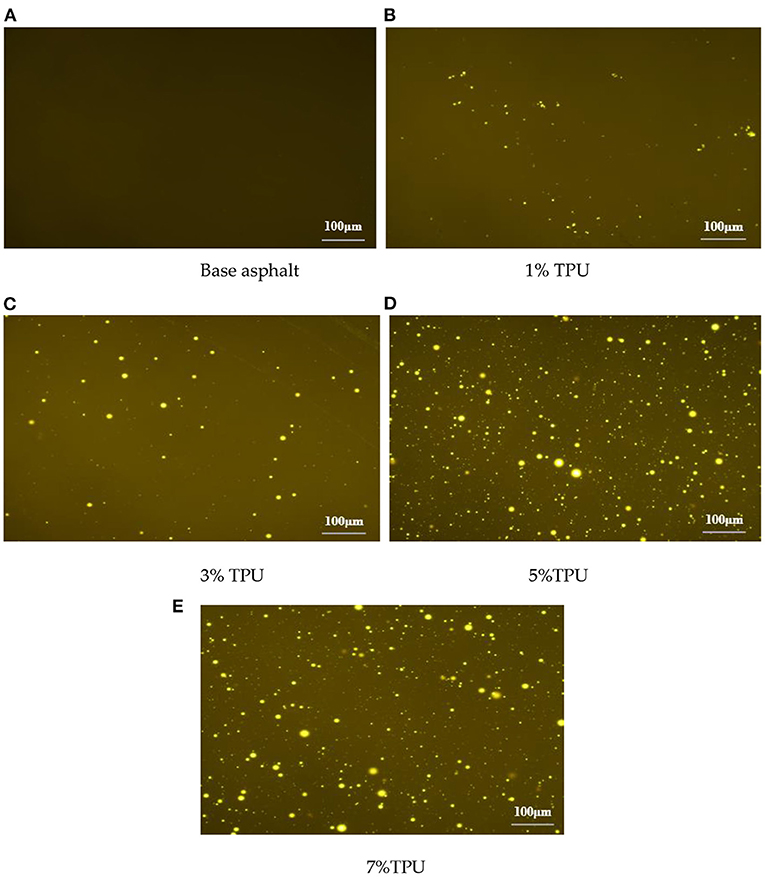
Figure 20. Fluorescence micrographs of polyether-based TPU-modified asphalt. (A) Base asphalt. (B) 1% TPU. (C) 3% TPU. (D) 5%TPU. (E) 7%TPU.
Similar to polyester-based TPU-modified asphalt, large particles increased obviously with the Ch and r value increasing, only the internal small particles did not reduce which is proof that the polyether polyols in polyether-based TPU could suppress the crystallinity increase with the content of MDI increasing. Therefore, the polyether-based TPU has better resistance to low temperature performance compared to a polyester-based TPU-modified asphalt (see Figure 21).
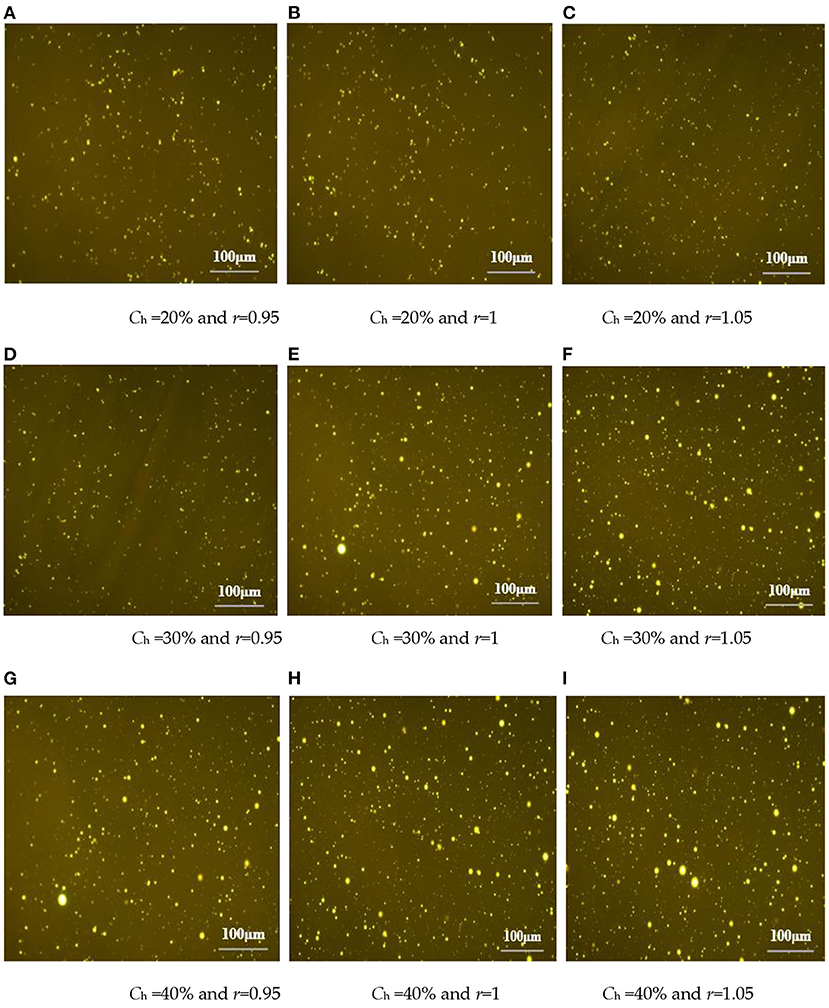
Figure 21. Fluorescence micrographs of polyether-based TPU-modified asphalt. (A) Ch = 20% and r = 0.95. (B) Ch = 20% and r = 1. (C) Ch = 20% and r = 1.05. (D) Ch = 30% and r = 0.95. (E) Ch = 30% and r = 1. (F) Ch = 30% and r = 1.05. (G) Ch = 40% and r = 0.95. (H) Ch = 40% and r = 1. (I) Ch = 40% and r = 1.05.
SEM
There is no accumulation at the interface, and the fracture mode of polyester-based TPU-modified asphalt under external force was a typical brittle fracture. At 500 times, some modifier particles were exposed to the outside and could not be completely covered by the base asphalt. The surface of the particles was relatively smooth, the cleavage was clear, and there were fine particle clusters attached at 5,000 times. This was due to the addition of excessive polyester-based TPU resulting in poor interface adhesion, affecting its adhesion. Thus, the low temperature performance of modified asphalt was affected (Figure 22).
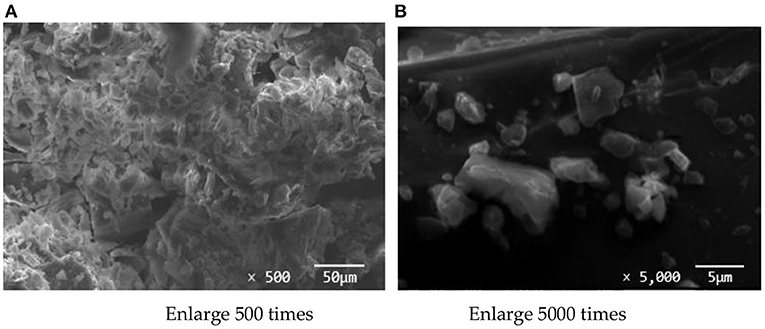
Figure 22. SEM images of polyester-based TPU-modified asphalt. (A) Enlarge 500 times. (B) Enlarge 5000 times.
It can be seen in Figure 23 that the expansion volume of polyether-based TPU can effectively improve the working performance of base asphalt, and the network structure of polyether-based TPU was formed in asphalt, which was helpful when changing the low temperature mechanical performance of asphalt. With magnification at 5,000, the surface of polyether-based TPU-modified asphalt was rough, and there was distribution of fine grain and white block. The structure of polyether-based TPU in asphalt had a very important influence on the properties of the modified asphalt, it was found that some filiform and soft curly branched chain combined with each other after the polyether-based TPU reaction with hot mixed asphalt which can form the three-dimensional network structure, and fold up after the asphalt overlapped together, thus expanding the viscoelastic domain category, so as to improve the performance (Jiang et al., 2018). In addition, the network strongly restricts the transfer between asphalt, restricts the mobility of asphalt colloid, and enhanced the ability to resist external forces. Only by applying large external forces can the asphalt produce relative displacement, so the low temperature resistance of asphalt was significantly improved.
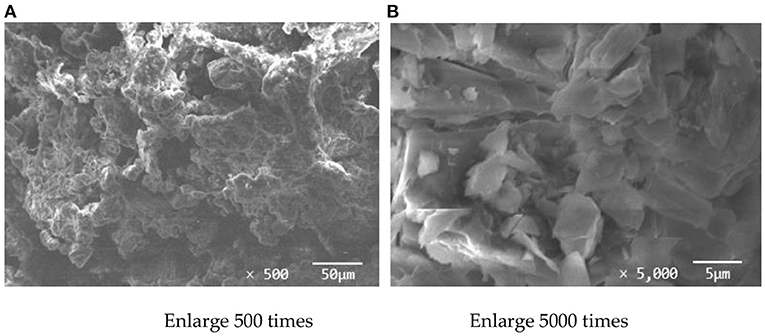
Figure 23. SEM images of polyether-based TPU-modified asphalt. (A) Enlarge 500 times. (B) Enlarge 5000 times.
TG
The TG curves of polyester-based TPU-modified asphalt obtained by the test are shown in Figure 24. As can be seen from Figure 24, TG curves of all samples show similar weight loss characteristics, and there is only one weight loss process. The thermal decomposition of the polyester-based TPU-modified asphalt was divided into two stages, on account of the TPU modifier used in this experiment belonging to a two-phase structure. The mass hardly changed in the temperature range from room temperature to 240°C, which indicated that it was relatively stable in this temperature range without material decomposition. In the vicinity of 240°C, the polyester-based TPU-modified asphalt started to break down, which is mainly caused by the overflow of light molecular weight in asphalt. After the decomposition of each asphalt sample at 500°C, the mass remains basically unchanged with the temperature continuing to rise, which formed the thermal hysteresis. Combined with the FTIR spectrum of TPU, it was speculated that urea and allophanate might cause differences in the melting peaks of the hard phase crystallization zone.
The T5% and T10% of all substances obtained by TG curves are shown in Table 9. As can be seen from Table 9, both T5% and T10% of polyester TPU-modified asphalt gradually increased with the increase of Ch or r value, which indicated that in polyester TPU-modified asphalt, the increase of MDI content can effectively improve the thermal stability of the modified asphalt, which is roughly the same as the temperature scanning test results.
As Figure 25 shows, the weight loss of polyether-based TPU-modified asphalt and polyester-based TPU-modified asphalt were similar with the change trend of temperature, which indicated that the polyurethane modifiers composed of the same hard section have similarities in the essential properties of matrix asphalt modification (Wen et al., 2020). But compared with polyester-based TPU-modified asphalt, the thermal decomposition temperature of polyether-based TPU-modified asphalt was slightly lower, there was no weight loss from room temperature to 200°C. The largest weight loss occurred over the temperature range of 300–500°C, which was attributed to the macromolecules splitting into small molecules and eventually becoming a gaseous and volatile substance.
The T5% and T10% of all substances obtained by TG curves are shown in Table 10. It is proven that the content of MDI in the synthesized polyurethane modifier was an important factor affecting the high-temperature stability of the modified asphalt. The polyester-based TPU modifier had a better high temperature performance than polyether-based TPU modifier.
DSC
Asphalt molecular mass generally will not exceed 6,000, the bonds between the molecules are weak due to the smaller molecular weight, so it is difficult to maintain a state under the action of a force. A mixture of asphalt is complex and morphologic changes often occur at different temperatures, from the size of Tg, it can be seen that the proportion of saturated fraction and asphaltenes in asphalt is distributed, the higher the saturation content, the less asphaltene and the lower the Tg will have. The midpoint of Tg can reflect the average situation of the entire glass transition region. Therefore, Tg is selected in part for the vitrification transition analysis, with the purpose of studying the influence of design parameters of polyurethane modifier on the thermal performance of asphalt.
Both the polyester-based TPU-modified asphalt and polyether-based TPU-modified asphalt began to absorb heat with the increase of temperature (see Figure 26). The peak value appeared on the DSC test curves, indicating that the chain segment began to move. As the temperature continues to rise, the material began to melt. This indicated that the phase state of all asphalt had changed. The asphalt is composed of a variety of complex components; different components have different phase transition temperatures, peak values, and temperature ranges. According to the theory of thermal characteristics, temperature causes the aggregation state of different molecules and components of the molecular structure in asphalt to change from the glass state to the rubber state and finally to the viscous state. From Figure, polyester-based TPU-modified asphalt by DSC curves show that when the Ch = 20 and Ch = 30%, there are two irregular strong peaks in the melting area; the first peak is caused by imperfect hard segment crystallization, namely, the material hard segment of the arrangement was not neat which made internal hydrogen bond and the cohesion of the drops melt first (Bazmara et al., 2018), and then the crystal with a neatly arranged area began to melt. The absorption (discharge) heat peak on the DSC test curve represents the aggregation state of asphalt, with a ratio of solid to liquid. In the aggregation state, the larger the peak value, the more intense the reaction, and then the worse thermal stability gets. By comparing the DSC curves of Figure 26A, it is obvious that the peak area of polyether-based TPU-modified asphalt was slightly larger than that of polyester-based TPU-modified asphalt which indicates that the high-temperature stability of polyester-based TPU-modified asphalt was better than that of polyether-based TPU-modified asphalt. However, the high-temperature stability of the two were similar, which is consistent with the results of TG analysis.
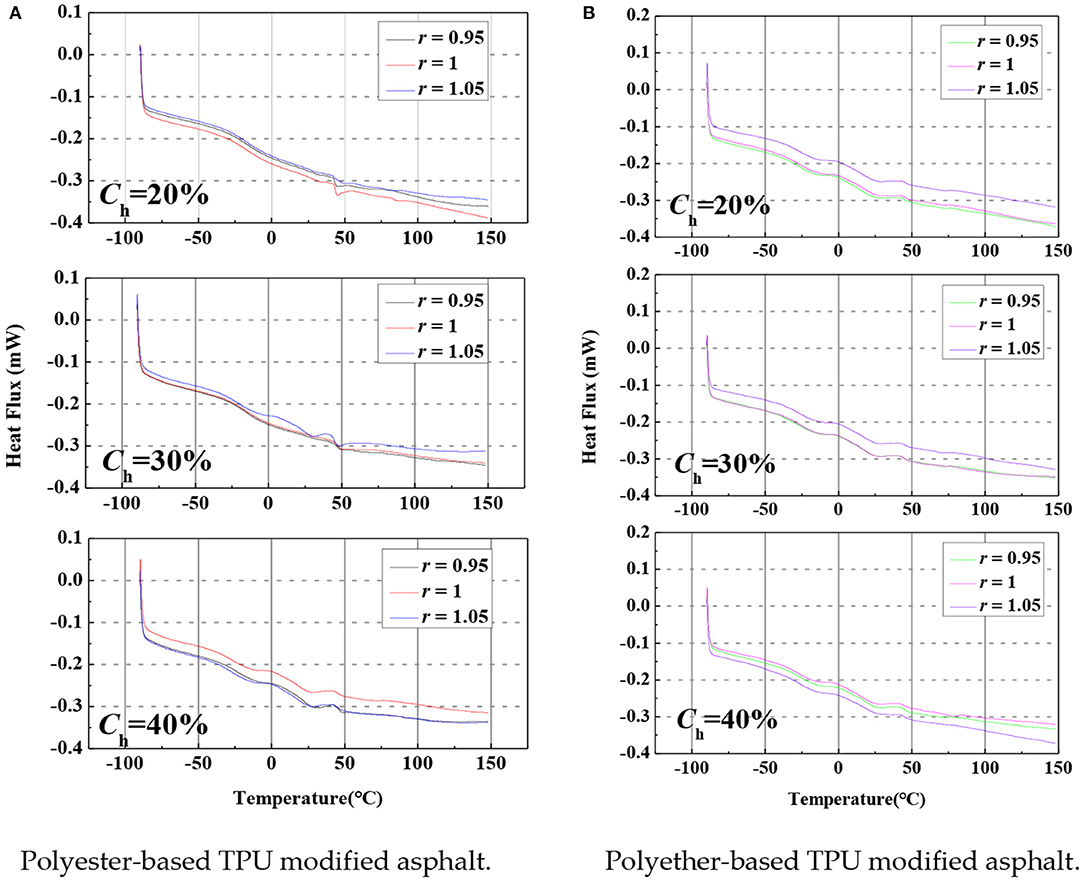
Figure 26. DSC curves of TPU-modified asphalt. (A) Polyester-based TPU-modified asphalt. (B) Polyether-based TPU-modified asphalt.
In fact, vitrification transformation is the phenomenon of molecular chain fracture in asphalt (Hao and Liu, 2003). As shown in Figure 27, asphalt manifested a viscoelastic property above Tg and a brittle fracture property below Tg. Although the heat absorption peak of polyester-based TPU-modified asphalt and polyether-based TPU-modified asphalt were similar, the variation trend of glass transition temperature was obviously different between each other. The reason for this is because the polyester-based TPU's molecular chain, of which the main chain is composed of a saturated single bond of polymer, can rotate within a fixed single bond. Steric hindrance of the matrix asphalt side chain substituents increased along with the increase of MDI content, rotating hampered when the molecular chain increases, and the Tg increased. However, when the Ch of polyether-based TPU-modified asphalt was constant, Tg decreased first and then increased with the increase of r value. When r value was unchanged and the increase of Ch, Tg had no obvious change regularity, the polyether polyol chain segment had great flexibility compared to polyester polyol. When r ≤ 1 and Ch <40%, the addition of polyether polyols can effectively offset the MDI chain rigidity and improve the viscoelastic property of asphalt, so as to improve the low temperature performance of asphalt. It should be noted that with a large polarity of polyester-based TPU, the polyester diol inside can not only form a hydrogen bond between hard segment, and the polar groups can also be part of the soft segment to form hydrogen bonds with the polar groups on the hard segment, but the hard phase can be more evenly distributed in soft phase crystallization phase formation, causing asphalt materials to become hard and brittle, thus affecting the mechanical properties of the asphalt. The soft segment of polyether showed a weak polarity, and the main chain structure of the molecule contains ether bonds, hydroxyl groups at the end of the base band of alcohol polymers, or oligomers. Due to the low cohesion and easy rotation of the ether bond in structure, the low temperature compliance was better, but the mechanical properties were not as good as polyester-based TPU. The above factors indicated that polyester-based TPU can affect the low temperature performance of based asphalt to a certain extent, while polyether-based TPU can improve the low temperature performance of base asphalt.
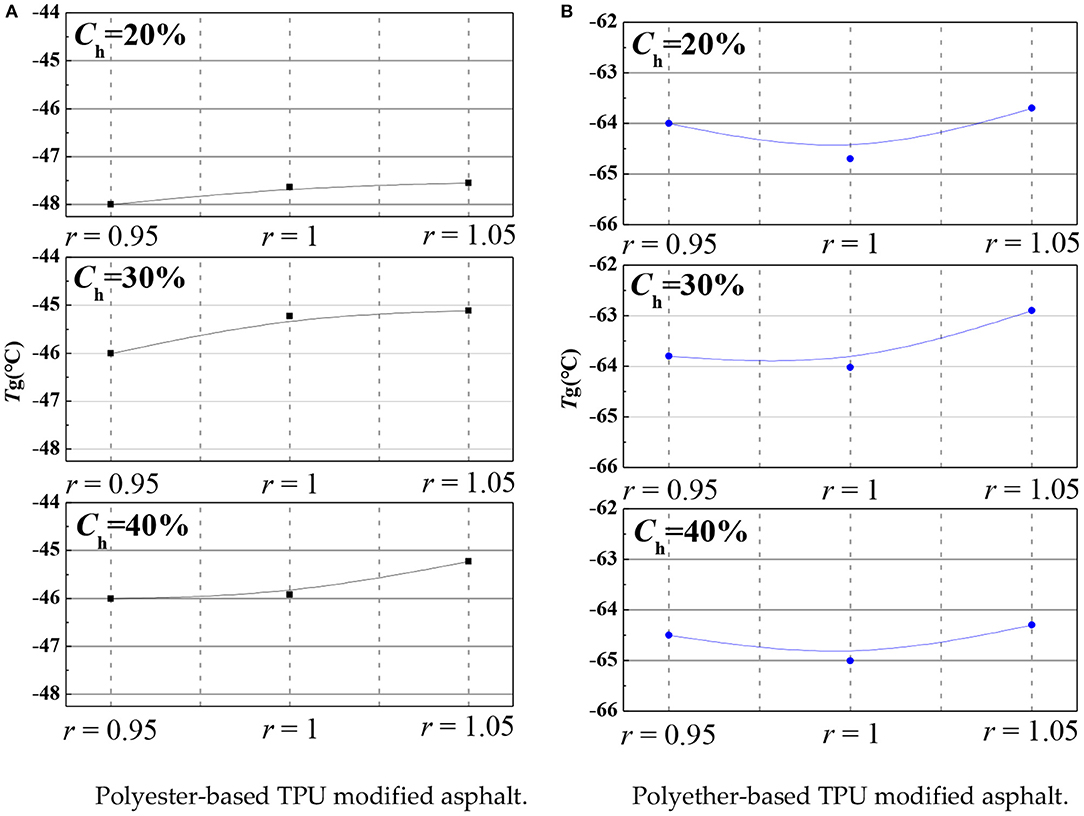
Figure 27. Tg of different samples. (A) Polyester-based TPU-modified asphalt. (B) Polyether-based TPU-modified asphalt.
Rheological Properties
DSR
The high temperature rheological properties of asphalt binders are closely related to the rutting resistance of the asphalt mixture. The rutting factor is the characteristic index of the anti-rutting performance of the asphalt binder (Zhou et al., 2020). The temperature sweep test results of the TPU-modified asphalt are summarized in Figure 28; a rapid fall at the start stage in the G*/sinδ of polyester-based TPU-modified asphalt and polyether-based TPU-modified asphalt samples were observed with the increasing temperature from 46 to 64°C, and then the temperature curves smoothed as the temperature increased. When Ch was 40%, the high temperature grade of both types of TPU-modified asphalt can reach 76°C. In addition, it is displayed that the G*/sinδ of polyester-based TPU-modified asphalt and polyether-based TPU-modified asphalt gradually increased with the same Ch as r value increased from 0.95 to 1.05. When Ch was <40%, G*/sinδ of polyester-based TPU-modified asphalt showed little difference from that of polyether-based TPU-modified asphalt, indicating that Ch played a crucial role in improving the high-temperature performance of modified asphalt compared with the molar ratio of NCO to OH. This is mainly due to the increase of MDI content, which will increase the crystallinity of modified asphalt, thus improving the high temperature rheological properties of modified asphalt. It is found that when Ch reached 40%, in detail, the G*/sinδ of modified asphalt under medium-temperature and high-temperature conditions can be improved relatively well. Taking into account the above results also verified the thermal properties from a rheological view.
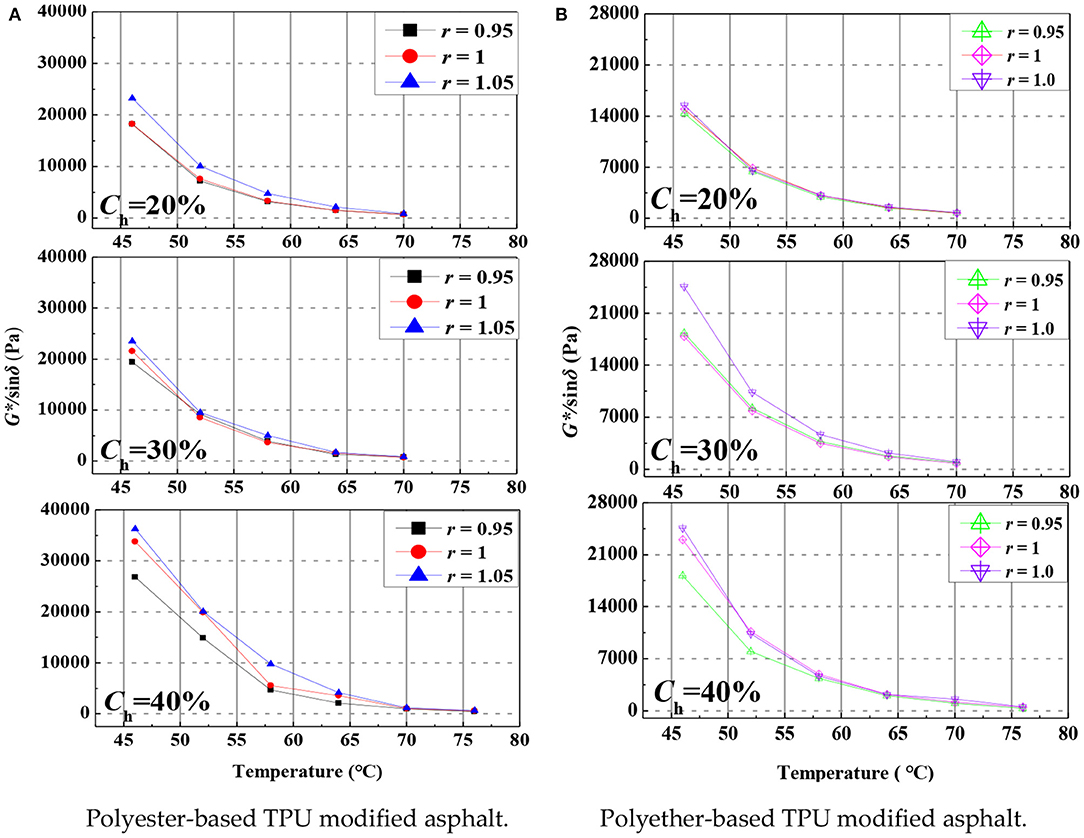
Figure 28. DSR test results of different samples. (A) Polyester-based TPU-modified asphalt. (B) Polyether-based TPU-modified asphalt.
BBR
In this study, BBR test was used and the test temperature was from −10 to −34°C. The creep stiffness (S) and creep rate (m-value) obtained from the test were used to evaluate the low-temperature performance of TPU-composite-modified asphalt.
The relationship between S or m-value with temperature of the polyester-based TPU-modified asphalt is shown in Figure 29. The S of the polyester-based TPU-modified asphalt exhibited a rapid increase with the decreasing temperature when the temperature was in the range from −16 to −34°C. With a temperature range between −10 and −16°C, the S of the polyester-based TPU-modified asphalt gradually leveled off. For an identical Ch, the influence of r value on S and m-value of polyester-based TPU-modified asphalt had no regularity. And the r value was unchanged, with the increase of Ch the S increased and m-value declined as the temperature increased. This indicates that the higher the content of polyester-based TPU, the worse the low-temperature crack resistance of the modified asphalt will get. The main reason is that with the increase of Ch, the vitrification transition temperature of the modified system increased gradually, however, the molecular chain rigidity of the modified system became more and more rigid with the continuous decrease of temperature. This means the low temperature deformation resistance of the modified asphalt was affected.
The BBR test results of the polyether-based TPU-modified asphalt are depicted in Figure 30, the results showed that the variation trend of polyester-based TPU-modified asphalt was similar to that of polyester-based TPU-modified asphalt. Within the temperature range from −16 to −34°C, the S of all samples increased rapidly with the decrease of temperature. S gradually became stable in the temperature range of −10 to −16°C. When Ch remained the same, S first increased and then decreased with the increase of r value, however m-value did not increase with the increase of Ch as r value was different, Therefore, it can be concluded that the low temperature ductility and self-healing ability of polyether-based TPU-modified asphalt will improve after fatigue failure. When r value reached 1, the m-value of modified asphalt can be improved to the greatest extent, which also reflected that most of the internal stress generated by the modified asphalt can be effectively absorbed and dispersed by TPU when the modified asphalt was deformed by external forces, and then the low-temperature cracking resistance of the base asphalt can be significantly enhanced. This is due to the polyether polyols being favorable for decreasing the interaction of molecules and improving the chain flexibility. Although the content of hard segment increased, ether group can offset the negative effect of the increase of hard segment and the decrease of soft segment on low temperature rheological energy. Also, it was shown that polyether-based TPU-modified asphalt can improve the rheological properties of base asphalt more effectively than polyester-based TPU-modified asphalt at low temperatures.
PG
Based on the DSR and BBR test results, the PG of the PU-composite-modified asphalt was conducted according to the method of SHRP asphalt performance specification (G*/sinδ≥1kPa, S ≤ 300MPa, m-value≥0.3), and the results are shown in Tables 11, 12. It can be seen that the higher the content of MDI, the better the high-temperature performance of modified asphalt will be. When Ch was 40% and r was 0.95, the PG grading of polyester-based TPU-modified asphalt was the best while the PG grading of polyether-based TPU-modified asphalt was better as Ch reached 40% and r was 1. Besides, polyether-based TPU-modified asphalt had the same high-temperature grade as polyester-based TPU-modified asphalt, but the low temperature grade was obviously higher than polyester-based TPU-modified asphalt.
MSCR Test
Figure 31 displays the MSCR test results of the PU-composite-modified asphalt at shear stress level of 0.1 and 3.2 kPa. Short-term aging of the TPU-modified asphalt samples were achieved using the rolling thin-film oven test (RTFOT) to simulate aging during construction according to AASHTO T 240, and the fatigue resistance of the specimens was obtained from the time-strain curves at 64°C. The change trend of polyester-based TPU-modified asphalt was consistent with that of polyether-based TPU-modified asphalt. As r increased from 0.95 to 1.05, the strain of two types of modified asphalt increased first and then decreased. When the Ch was certain, the irrecoverable strain increased first and then decreased with the increase of r value from 0.95 to 1.05. When r value was the same, the irrecoverable strain gradually decreased with the increase of Ch, and Ch reached 40%, and the irrecoverable strain was the smallest when r value was 1.05.
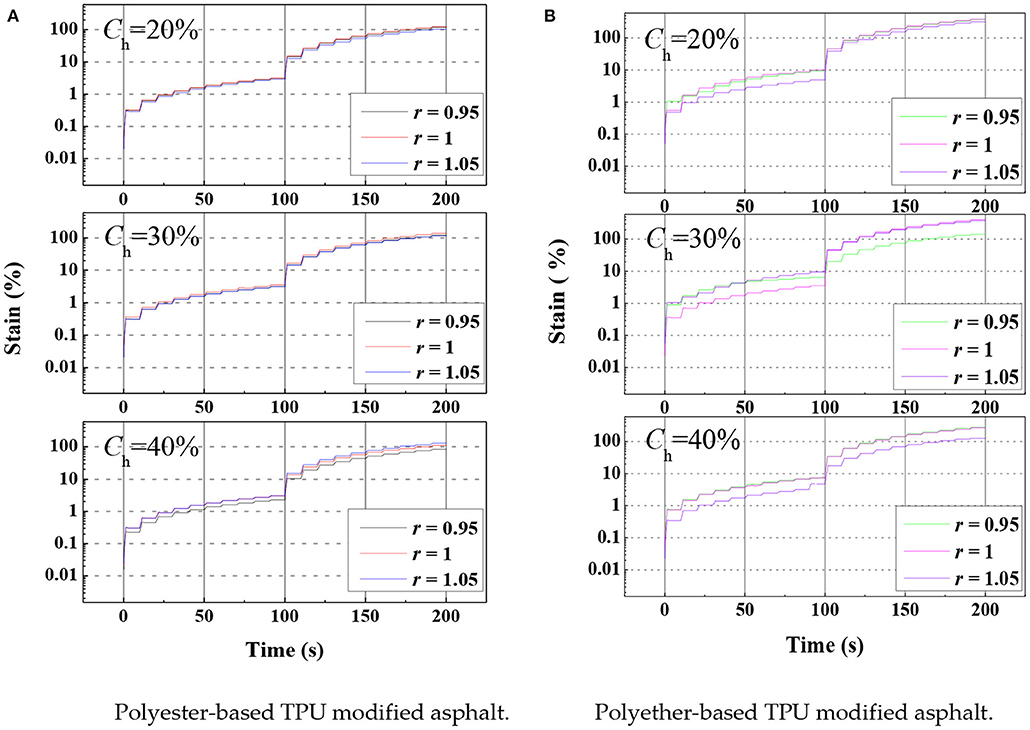
Figure 31. Time-irreversible strain curves at 64°C. (A) Polyester-based TPU-modified asphalt. (B) Polyether-based TPU-modified asphalt.
Additionally, the result from the MSCR test presented a superior consistency with the DSR test. The comparison of Jnr 0.1 (the corresponding unrecoverable compliance at 0.1kPa) and Jnr 3.2 (the corresponding unrecoverable compliance at 3.2kPa) are shown in Figures 32, 33. According to R and Jnr calculated by experiments, it was found that when the r value increased from 0.95 to 1.05 with the same Ch, the R of both polyester-based TPU-modified asphalt and polyether-based TPU-modified asphalt first increased and then decreased, which showed that the increase of MDI content can improve the elastic recovery ability of modified asphalt. For TPU-modified asphalt whose hard segment was composed by MDI type, the elastic recovery ability was the best when the molar ratio of NCO to OH was 1. When r value was certain, R gradually increased with the increase of Ch. Compared with polyester-based TPU-modified asphalt, the R of polyether-based TPU-modified asphalt was significantly improved as the polyether-based TPU had more elastic components than polyester-based TPU which can better improve the recovery and deformation capacity of base asphalt.
The overall trend of polyester-based TPU-modified asphalt was the same as that of polyether-based TPU-modified asphalt. The non-recoverable creep compliance decreased with the increase of Ch, which indicated that the increase of MDI content in TPU made the high-temperature resistance to permanent deformation of modified asphalt stronger. However, when the Ch was fixed and the r value gradually increased, the unrecoverable creep compliance of polyester-based TPU-modified asphalt first increased and then decreased while that of polyether-based TPU-modified asphalt gradually increased. Nevertheless, the unrecoverable creep flexibility of polyether-based TPU-modified asphalt was significantly lower than that of polyester-based TPU-modified asphalt, indicating that the elastic recovery ability of TPU-modified asphalt with soft segment polyether polyol is obviously better than that with soft segment polyester polyol. In conclusion, it can be concluded that polyether-based TPU-modified asphalt has more excellent resistance to permanent deformation.
Figures 34, 35 showed the influence rules of different types of TPU modifiers on Rdiff and Jnr−diff. It can be seen that all modified asphalt samples meet the standard requirements of AASHTO MP 19-10 being ≤ 75%. When Ch was fixed, the Rdiff and Jnr−diff of both polyester-based TPU-modified asphalt and polyether-based TPU-modified asphalt showed little effect as the change of r value. However, when r value was unchanged, Rdiff and Jnr−diff showed a downward trend with the increase of Ch. Rdiff and Jnr−diff of polyester-based TPU-modified asphalt showing the smallest as Ch and r value were 40% and 0.95, respectively. When the Ch of polyether-based TPU-modified asphalt reached 40% and r = 1, Rdiff and Jnr−diff were at a minimum, indicating that the stress sensitivity was the least, which also means that the line viscoelasticity was more significant. The Rdiff and Jnr−diff of polyether-based TPU-modified asphalt was greater than those of polyester-based TPU-modified asphalt, indicating that the addition of polyether-based TPU can more reduce the stress sensitivity of base asphalt.
At last, in combination with conventional performance indexes, microscopic performance characterization, and high and low temperature rheological properties test results, polyether-based TPU is recommended as an ideal asphalt modifier with a hard section content of 40%, and r = 1 is preferred.
Conclusions
In summary, the polyester-based TPU and polyether-based TPU-modified asphalt samples were successfully produced by a newly developed preparation process, and then the laboratory performance, including rheological properties and micro-characteristics, were investigated and discussed. Based on the study conducted, the following conclusions can be drawn:
(1) For the use of polyester-based TPU in the modified asphalt, there was an increased content of TPU from 1 to 7%, the penetration increased 3 to 75%, and the ductility increased −35 to 300%, however, the softening point exhibited a slight variation from 0 to 35%. As the polyether-based TPU raised from 1 to 7%, the softening point of the modified asphalt increased −4 to 27%, whereas the penetration and ductility increased −14 to 69% and 2 to 419%, respectively. The modified asphalt with 5% TPU exhibited a favorable performance in terms of penetration, ductility, softening point, and rotational viscosity. With the TPU composed of MDI, the increased Ch and r value can improve the high-temperature deformation resistance of base asphalt. The molecular weight showed little influence on the physical properties of the polyester-based TPU and polyether-based TPU-modified asphalt. The polyether-based TPU is more suitable to be used as a modifier of asphalt.
(2) The FTIR test further proved that both polyester TPU and polyether TPU can react with some active functional groups in the base asphalt, and new compounds, such as generated isocyanate, in the modified asphalt reacted with phenol and carboxylic acid in asphalt. Moreover, the isocyanate and polyol reacted to form carbamate in the TPU-modified asphalt and the isocyanate and aromatic compounds reacted to form halohydrins in the asphalt.
(3) From the morphology and microstructure analysis, it was observed that the polyester-based TPU showed large and small particles were uniformly distributed in the base asphalt after the modifier had been sheared. The modifier had good swelling and dispersion effect. The asphalt and polyurethane cooperate with each other to form a typical continuous with two-phase structure mixture of spatial network structure. It was also found that the organic molecular chain in the modifier of the polyether-based TPU-modified asphalt can be embedded into the layered structure which can promote the formation of the cross-linked structure in polyether-based TPU, so as to improve the low temperature resistance of asphalt binder.
(4) The polyester-based TPU-modified asphalt exhibited a similar improvement as compared to the polyether-based TPU-modified asphalt with regard to the thermal stability. However, the polyether-based TPU can increase the low-temperature performance. The addition of polyester-based TPU into the PU-modified asphalt reduced the low-temperature performance and increased the formation of hydro-keys. In addition, the content of MDI in the synthesized polyurethane modifier was an important factor affecting the high-temperature stability of the modified asphalt. The polyester-based TPU modifier showed a better high temperature performance than the polyether-based TPU modifier.
(5) The polyester-based TPU modifier has a better high temperature performance than the polyether-based TPU modifier. The polyether polyol chain segment showed a great flexibility compared to polyester polyol; the addition of polyether polyols can be effectively offset with MDI chain rigidity as r ≤ 1 and Ch <40%, which improved the viscoelastic property of asphalt. When Ch = 40% and r = 0.95, the polyether-based TPU-modified asphalt exhibited the same high-temperature grade as the polyester-based TPU-modified asphalt, the polyether-based TPU modified asphalt showed a higher low temperature grade, which also verified the investigation results exhibited for the thermal properties.
(6) The permanent deformation resistance of the TPU-modified asphalt was improved with the inclusion of MDI, and the polyester polyol performed a better resistance to permanent deformation than the polyether polyol. The unrecoverable creep compliance at 3.2 kPa stress combining with the high temperature PG can more accurately evaluate the resistance to permanent deformation of the TPU-modified asphalt. The Rdiff and Jnr−diff of polyether-based TPU-modified asphalt was greater than those of polyester-based TPU-modified asphalt, indicating that the addition of polyether-based TPU can better reduce the stress sensitivity of base asphalt. Overall, the TPU-modified asphalt added 5% polyether-based TPU with Ch = 40% and r =1, and can be regarded as an appropriate composition as it demonstrated a favorable performance based on the investigation conducted in this study.
Data Availability Statement
The original contributions presented in the study are included in the article/supplementary material, further inquiries can be directed to the corresponding author/s.
Author Contributions
XJ, SS, and NG: conceptualization and data curation. SS, XJ, SH, and YT: methodology. NG: validation and resources. SS, XJ, SH, and NG: formal analysis. XJ: investigation. XJ and NG: writing—review and editing. NG and ZY: supervision. All authors have read and agreed to the published version of the manuscript.
Funding
This study was funded by the Scientific Innovation Fund of Dalian Maritime University, the Dalian technology Bureau of science and technology innovation fund (Grant no. 2020JJ26SN062), Liaoning Highway Administration Bureau under (Grant no. 201701), and National Natural Science Funding of China (Grant no. 51308084), and the Dalian Maritime University Double First-class Construction Project (Grant no. BSCXXM021).
Conflict of Interest
SS and SH were employed by Liaoning Provincial Transportation Science Research Institute Co. Ltd.
The remaining authors declare that the research was conducted in the absence of any commercial or financial relationships that could be construed as a potential conflict of interest.
References
Alamawi, M. Y., Khairuddin, F. H., Md.Yusoff, N. I., Badri, K., and Halil, C. (2019). Investigation on physical, thermal and chemical properties of palm kernel oil polyol bio-based binder as a replacement for bituminous binder, Constr. Build. Mater. 204, 122–131. doi: 10.1016/j.conbuildmat.2019.01.144
Apostolidis, P., Liu, X., Kasbergen, C., van de Ven, M. F. C., Pipintakos, G., and Scarpas, A. (2018). Chemo-rheological study of hardening of epoxy modified bituminous binders with the finite element method. Transp. Res. Rec. 2672, 190–199. doi: 10.1177/0361198118781377
Athira, P. K., Atul Narayan, S. P., Krishnan, J. M., and Jain, P. K. (2020). Comparison of binder and mixture tests to characterize permanent deformation of elastomer and terpolymer modified binders. Constr. Build. Mater. 264:120138. doi: 10.1016/j.conbuildmat.2020.120138
Barrioni B. R. de Carvalho S. M. Oréfice R. L. de Oliveira A. A. R. Pereira M. M. (2015). Synthesis and characterization of biodegradable polyurethane films based on HDI with hydrolyzable crosslinked bonds and a homogeneous structure for biomedical applications. Mater. Sci. Eng. C. 52, 22–30. doi: 10.1016/j.msec.2015.03.027
Bazmara, B., Tahersima, M., and Behravan, A. (2018). Influence of thermoplastic polyurethane and synthesized polyurethane additive in performance of asphalt pavements, Constr. Build. Mater. 166, 1–11. doi: 10.1016/j.conbuildmat.2018.01.093
Cao, Z., Huang, X., Yu, J., Han, X., Wang, R., and Li, Y. (2020). Study on all-components regeneration of ultraviolet aged SBS modified asphalt for high-performance recycling. J. Clean. Prod. 276:123376. doi: 10.1016/j.jclepro.2020.123376
China Communications Press (2011). JTG E 20-2011 Standard Test Methods of Bitumen and Bituminous Mixtures for Highway Engineering [S]. Beijing: China Communications Press.
Cong, L., Yang, F., Guo, G., Ren, M., and Tan, L. (2019). The use of polyurethane for asphalt pavement engineering applications: a state-of-the-art review. Constr. Build. Mater. 166, 1012–1025. doi: 10.1016/j.conbuildmat.2019.07.213
Gallu, R., Méchin, F., Dalmas, F., Gérard, J., Perrin, R., and Loup, F. (2020). Rheology-morphology relationships of new polymer-modified bitumen based on thermoplastic polyurethanes (TPU). Constr. Build. Mates. 259:120404. doi: 10.1016/j.conbuildmat.2020.120404
Gong, J., Liu, Y., Wang, Q., Xi, Z., Cai, J., Ding, G., et al. (2019). Performance evaluation of warm mix asphalt additive modified epoxy asphalt rubbers. Constr. Build. Mater. 204, 288–295. doi: 10.1016/j.conbuildmat.2019.01.197
Hao, P. W., and Liu, T. (2003). SHRP asphalt binder standards are used for evaluation of in-service performance. J. Highw. Transport. Res. Dev. 1, 11–13.
Jiang, L., Zhou, Y., Wang, Y., Jiang, Z., Zhou, F., Chen, S., et al. (2018). Fabrication of dielectric elastomer composites by locking a pre-stretched fibrous TPU network in EVA. Materials 11:1687. doi: 10.3390/ma11091687
Jin, X., Guo, N. S., You, Z. P., Wang, L., Wen, Y. K., and Tan, Y. Q. (2020). Rheological properties and micro-characteristics of polyurethane composite modified asphalt. Constr. Build. Mater. 234:117395. doi: 10.1016/j.conbuildmat.2019.117395
Kang, Y., Song, M., Pu, L., and Liu, T. (2015). Rheological behaviors of epoxy asphalt binder in comparison of base asphalt binder and SBS modified asphalt binder. Constr. Build. Mater. 76, 343–350. doi: 10.1016/j.conbuildmat.2014.12.020
Król, P. (2007). Synthesis methods, chemical structures and phase structures of linear polyurethanes. properties and applications of linear polyure-thanes in polyurethane elastomers, copolymers and ionomers. Prog. Mater. Sci. 52, 915–1015. doi: 10.1016/j.pmatsci.2006.11.001
Li, C., Liu, J.W, Li, J. J., Shen, F., Huang, Q., and Xu, H. (2012). Studies of 4,4′-diphenylmethane diisocyanate (MDI)/1,4-butanediol (BDO) based TPUs by in situ and moving-window two-dimensional correlation infrared spectroscopy: understanding of multiple DSC endotherms from intermolecular interactions and motions level. Polymer 53, 5423–5435. doi: 10.1016/j.polymer.2012.09.030
Liu, J., Yan, K. L., You, L., Ge, D., and Wang, Z. (2016). Laboratory performance of warm mix asphalt binder containing polyphosphoric acid. Constr. Build. Mater. 106, 218–227. doi: 10.1016/j.conbuildmat.2015.12.126
Liu, S., Zhou, S., and Peng, A. (2020). Evaluation of polyphosphoric acid on the performance of polymer modified asphalt binders. J. Appl. Polym. Sci. 137:48984. doi: 10.1002/app.48984
Liu, X., Gu, X., Sun, J., and Zhang, S. (2017). Preparation and characterization of chitosan derivatives and their application as flame retardants in thermoplastic polyurethane. Carbohydr. Polymers. 167, 356–363. doi: 10.1016/j.carbpol.2017.03.011
Lu, Q., and Bors, J. (2015). Alternate uses of epoxy asphalt on bridge decks and roadways. Constr. Build. Mater. 78, 18–25. doi: 10.1016/j.conbuildmat.2014.12.125
Mao, Z. Q., Zhang, B., Guo, X. Y., Shi, H. C., Yao, W. Q., and Zhang, M. G. (2017). Synthesis and properties of polyester-polyether mixed soft segment type polyurethane resin. Chem. Adhes. 39, 1–3.
Pang, K. W., Li, Y. F., Qu, Z. M., and Wu, Y. Q. (2007). Processing characteristics of MDI casting polyurethane elastomer. Polyureth. Ind. 22, 32–34.
Parnell, S., and Min, K. (2005). Reactive blending of thermoplastic polyurethane in situ with poly(vinylchloride). Polym. Eng. Sci. 45, 876–887. doi: 10.1002/pen.20334
Rosu, D., Tudorachi, N., and Rosu, L. (2010). Investigations on the thermal stability of a MDI based polyurethane elastomer. J. Anal. Appl. Pyrolysis 89, 152–158. doi: 10.1016/j.jaap.2010.07.004
Shen, K. Y. (2020). Unsaturated Polyester Resin and Its Application, 2nd Edn. Beijing: Chemical Industry Press.
Sheng, X., Wang, M., Xu, T., and Chen, J. (2018). Preparation, properties and modification mechanism of polyurethane modified emulsified asphalt, Constr. Build. Mater. 189, 375–383. doi: 10.1016/j.conbuildmat.2018.08.177
Shirzad, S., Hassan, M., and Mohammad, L. N. (2020). Rheological and mechanical evaluation of polyurethane prepolymer-modified asphalt mixture with self-healing abilities. J. Mater. Civ. Eng. 32:04020231. doi: 10.1061/(ASCE)MT.1943-5533.0003307
Sun, M., Zheng, M., Qu, G., Yuan, K., Bi, Y., and Wang, J. (2018). Performance of polyurethane modified asphalt and its mixtures, Constr. Build. Mater. 191, 386–397. doi: 10.1016/j.conbuildmat.2018.10.025
Sun, M., Zheng, M. L., Bo, Y. F., Zhu, L. L., and Gao, Y. (2019). Modification mechanism and performance of polyurethane modified asphalt. J. Traff. Transport. Eng. 19, 49–58.
Wang, C., Wang, H., Zhao, L., and Cao, D. (2017). Experimental study on rheological characteristics and performance of high modulus asphalt binder with different modifiers, Constr. Build. Mater. 155, 26–63. doi: 10.1016/j.conbuildmat.2017.08.058
Wen, Y., Chen, C., Feng, Y., Xue, Z., Zhou, X., Xie, X., et al. (2020). Effects of selective distribution of alumina micro-particles on rheological, mechanical and thermal conductive properties of asphalt/SBS/alumina composites. Composit. Sci. Technol. 186:107917. doi: 10.1016/j.compscitech.2019.107917
Wu, H. H., Xie, H. P., Tian, X. X., Sun, Y., Liu, X., and Yang, Y. (2019). Preparation and properties of polyester-polyether polyurethane. Eng. Plastic Appl. 47, 17–21.
Xing, C., Liu, L., and Li, M. (2020). Chemical composition and aging characteristics of linear SBS modified asphalt binders. Energy Fuels. 34, 4194–4200. doi: 10.1021/acs.energyfuels.9b04523
Xu, C., Zhang, Z., Zhao, F., Liu, F., and Wang, J. (2019). Improving the performance of RET modified asphalt with the addition of polyurethane prepolymer (PUP). Constr. Build. Mater. 206, 560–575. doi: 10.1016/j.conbuildmat.2019.02.101
Xue, H. X., Tan, Y. Q., and Sha, A. M. (2020). Adhesive characteristic and mechanism of ballastless track sealant in hydrolysis condition. Front. Mater. 7:535483. doi: 10.3389/fmats.2020.535483
Yang, Y. L., Lu, X., and Wang, W. W. (2017). A tough polyurethane elastomer with self-healing ability. Mater. Design 127, 30–s36. doi: 10.1016/j.matdes.2017.04.015
Yu, R., Zhu, X., Zhang, M., and Fang, C. (2018). Investigation on the short-term aging-resistance of thermoplastic polyurethane-modified asphalt binders. Polymers. 10:1189. doi: 10.3390/polym10111189
Zhang, J., Kong, Q., Yang, L., and Wang, D. Y. (2016). Few layered Co(OH)2 ultrathin nanosheet-based polyurethane nanocomposites with reduced fire hazard: from eco-friendly flame retardance to sustainable recycling. Green Chem. 18, 3066–3074. doi: 10.1039/C5GC03048J
Zhang, J. P., Fan, Z. P., Hu, D. L., Pei, J. Z., and Kong, W. C. (2018). Evaluation of asphalt-aggregate interaction based on the rheological properties, Int. Pavement Eng. J. 19, 586–592. doi: 10.1080/10298436.2016.1199868
Zhou, T., Zhou, J., Li, Q., and Li, B. (2020). Aging properties and mechanism of microwave-activated crumb rubber modified asphalt binder. Front. Mater. 7:603938. doi: 10.3389/fmats.2020.603938
Keywords: thermoplastic polyurethane elastomers, synthesis parameters, modified asphalt, rheological properties, micro-characteristics
Citation: Jin X, Sun S, Guo N, Huang S, You Z and Tan Y (2021) Influence on Polyurethane Synthesis Parameters Upon the Performance of Base Asphalt. Front. Mater. 8:656261. doi: 10.3389/fmats.2021.656261
Received: 20 January 2021; Accepted: 08 March 2021;
Published: 09 July 2021.
Edited by:
Sher Bahadar Khan, King Abdulaziz University, Saudi ArabiaReviewed by:
Nafisa Gull, University of the Punjab, PakistanJyotishkumar Parameswaranpillai, King Mongkut's University of Technology North Bangkok, Thailand
Copyright © 2021 Jin, Sun, Guo, Huang, You and Tan. This is an open-access article distributed under the terms of the Creative Commons Attribution License (CC BY). The use, distribution or reproduction in other forums is permitted, provided the original author(s) and the copyright owner(s) are credited and that the original publication in this journal is cited, in accordance with accepted academic practice. No use, distribution or reproduction is permitted which does not comply with these terms.
*Correspondence: Naisheng Guo, bmFpc2hlbmdndW9AMTI2LmNvbQ==
†These authors have contributed equally to this work and share first authorship