- 1Department of Chemistry, Sree Narayana College, Kannur, India
- 2Hybrid Microsystems, Microsystems LTCC and HTCC, Fraunhofer Institute for Ceramic Technology, Dresden, Germany
- 3Department of Chemistry, Malabar Christian College, Calicut, India
BaZr0.2[Ti(1-x)Mgx/3Ta2x/3]0.8O3 (x = 0.0, 0.05, 0.1, 0.2, 0.4, 0.6, 0.8, 1.0) (BZTMT) ceramics were prepared through conventional solid state ceramic route. The crystal structure and microstructure of the compounds were investigated respectively using XRD and SEM. The dielectric properties were measured in the frequency range of 100 Hz–1 MHz. The ferroelectric Curie temperature of BaZr0.2Ti0.8O3 (x = 0) shifted from 21°C to -10°C with the addition of minute amount of dopant (x = 0.025) and the paraelectric transition temperature is diffusive in nature. The relative permittivity and dielectric loss of BZTMT at 1 MHz varies from 5205 to 24 and 1.8 × 10–2 to 2.0 × 10–5 respectively, as the value of x increases from 0 to 1.
Introduction
BaTiO3 based ferroelectric materials have received a great deal of attention from scientific community as an alternative for lead free materials for extensive applications in capacitors, piezoelectric devices and transducers(Wang et al., 2012; Chen et al., 2014; Magnone et al., 2016; Li et al., 2017; Co et al., 2020; Venkatachalam et al., 2020). The high curie temperature (128°C) and intrinsically low relative permittivity in pure BaTiO3 make it unsuitable in practical device applications(Hennigs and Schnell, 1982). Electrical properties of BaTiO3 can be tailored by chemically substituting both dodecahedral Ba2+ sites and the octahedral Ti4+ sites(Hennigs and Schnell, 1982; Sangwan et al., 2018). Ti4+ site is generally substituted by Zr4+, which is more chemically stable than that of Ti4+. The resulting material, BaZrxTi1-xO3 (BZT) generally exhibits very high and broad curie maxima and are often found application as relaxor ferroelectrics(Li et al., 2014). As in the case of BaTiO3, three ferroelectric phase transitions are observed in BZT solid solution for x < 0.13. On increasing the Zr content the three transition points and the corresponding dielectric maxima move closer together and finally coalesce in to single broad maxima(Hennigs and Schnell, 1982). For composition 0.26 < x < 0.42, the temperature dependence of the real part of dielectric constant at transition temperature is broad and frequency dependent (Maiti et al., 2008; Li et al., 2014). Until now many researchers have studied the dielectric properties of BZT system(Buscaglia et al., 2000; Weber et al., 2001; Maiti et al., 2008).
Substitution for both isovalent and aliovalent cation in the host BZT perovskite lattice plays a very important role in the modification of dielectric properties. Buscaglia et al. had reported that the aliovalent cation substitution in perovskite lattice can serve either as donors or as acceptors, which will affect the electrical characteristics greatly(Buscaglia et al., 2000). Many aliovalent compositional alterations in BZT have been studied either with higher valance substitution (donors), or with lower valance ions (acceptors). Donor dopants induce cationic defects while occupying octahedral site of perovskite lattice(Ravez and Simon, 2000; Surendran and Sebastian, 2005). Rout et al. observed a decrease in Curie temperature (Tc) by substituting small amount of Mg2+ in place of Ba2+ in BZT, which was ascribed to decrease in the grain size(Rout et al., 2007). The cation ordering and dielectric properties of Ba(Mg1/3Ta2/3)1-xTixO3 system was reported by Surendran et al (Surendran and Sebastian, 2005) They found that as the concentration of Ti4+ is x > 0.2, the cation ordering shifted to disordered state. Moreover the microwave resonance character of Ba(Mg1/3Ta2/3)O3 vanishes completely when the concentration exceeds x = 0.6(Penn and McN Alford, 2000).
In the present investigation Ti4+ octahedral site in ferroelectric BaZr0.2Ti0.8O3 is systematically replaced with (Mg1/3Ta2/3)4+ ions. The dielectric properties of the materials were investigated both in low frequency and microwave regions. The effect of (Mg1/3Ta2/3)4+ substitution on the curie temperature of the materials were also investigated. To the best of our knowledge no useful work has been done on the aspect of simultaneous substitution of Mg and Ta in the Ti site of BZT. The present paper describe the variation of microwave dielectric properties of BaZr0.2 [Ti(1-x)Mgx/3Ta2x/3]0.8O3 as a function of x.
Materials and Methods
Materials Synthesis
The BaZr0.2 [Ti(1-x)Mgx/3Ta2x/3]0.8O3 [ x = 0.0, 0.05, 0.1, 0.2, 0.4, 0.6, 0.8, 1.0] solid solution by varying the concentrations of (MgTa)4+ were prepared by the conventional solid state ceramic route. High-purity (>99.9%) powders of barium carbonate (BaCO3), magnesium carbonate hydroxide penta hydrate (MgCO3Mg(OH)2-5H2O), titanium dioxide (TiO2), zirconium dioxide (ZrO2) (Aldrich Chemicals), and tantalum pentoxide (Ta2O5) (NFC, Hyderabad) were used as the starting materials. Stoichiometric proportions of these chemicals were mixed from a ball milling unit for 24 h. The slurry obtained was dried in a hot air oven at 100°C for12 h and then calcined at 1,200°C for 8 h at a heating rate of 5°C/min. The calcined powders were ground well and mixed with 4 wt% aqueous solution of Poly vinyl alcohol. The powder was uniaxially pressed into cylindrical pellets having diameter 11 mm and thickness 1.5 mm under a pressure of 100 MPa. These sample pellets were fired to 600°C at a rate of 5°C/min, kept at 600°C for 30 min to expel binder and then sintered at 1,600°C for 2 h in air. The samples were then cooled to 800°C at a slow rate of 3.5°C/min. The bulk densities of the sintered samples were measured using dimensional method.
Characterization Techniques
The phase purity of the as synthesized BZTMT solutions was analyzed by X-ray diffraction technique using Cu-Kα radiation (Philips X’pert Prro diffractometer). The sintered samples were thermally etched, and their surface morphology and grain sizes were studied using a scanning electron microscope (JEOL-JSM 5600 LV, Japan). The sintered and polished thin pellets were electroded by coating silver paste on both sides and the dielectric properties in low frequency regions (500 Hz–1 MHz) were measured using an LCR meter (HIOKI 3532-50 LCR HiTESTER). The temperature dependence of relative permittivity in the range of -25–70°C was also determined from the same instrument at 1 MHz frequency. The dielectric properties of the materials in the microwave frequency range were measured by the resonance method using a Vector Network Analyzer (Agilent 8753 ET, Palo Alto, CA). TE01δ mode of resonance is used for the measurements(Krupka et al., 1998). The cavity method is ideal for measuring the Q factor since the electric field is symmetrical with geometry of the cavity and the dielectric(Krupka et al., 1998). The temperature coefficient of resonant frequency (τf) was measured in the temperature range 25–70°C. The resonance frequency was measured at regular time intervals of temperature ramping and the τf was measured by the expression,
where f1isthe resonant frequency at temperature T1 and f2 is the resonant frequency at temperature T2.
Results and Discussion
X-Ray Diffraction
The room temperature powder X-ray diffraction patterns of BZTMT solid solution (x = 0, 0.2, 0.6, 1.0) are shown in Figure 1. The crystal structure of pure BaZr0.2Ti0.8O3 (x = 0) is indexed to the cubic symmetry phase (00360019), due to the fact that above 21°C (Curie temperature) the BZT changes from the non-centro symmetric tetragonal phase to the centro-symmetric cubic phase. Unit cell parameter of pure BZT calculated from diffraction studies is a = 4.04 Ǻ. As the concentration of (MgTa)4+ increases unit cell parameter increases and reaches a maximum of 4.10 Ǻ at x = 1 (Table 1).
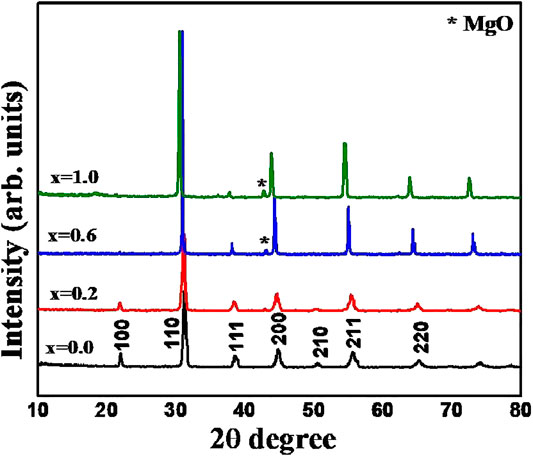
FIGURE 1. XRD patterns of BaZr0.2[Ti(1-x)Mgx/3Ta2x/3]0.8O3 solid solution with x = 0.0, 0.2, 0.6 and 1.0 compositions.

TABLE 1. Unit cell parameter and dielectric properties of BaZr0.2[Ti(1-x)Mgx/3Ta2x/3]0.8O3 solid solution phase.
SEM Analysis
The SEM micrographs of polished and thermally etched surfaces of the sintered BaZr0.2[Ti(1-x)Mgx/3Ta2x/3]0.8O3 (x = 0, 0.1, 0.4, 0.6) is shown in Figure 2. The average grain size of pure BZT (x = 0) is about 25–30 µm. It is interestingly noted that as the concentration of Mg and Ta increases, the grain size decreases. The average grain size of x = 0.6 composition was found to be 4–6 µm. For BZTMT compositions with x ≥ 0.6, the presence of unreacted MgO phase is clearly visible from the SEM image (Figure 2D) as evidenced from the XRD studies.
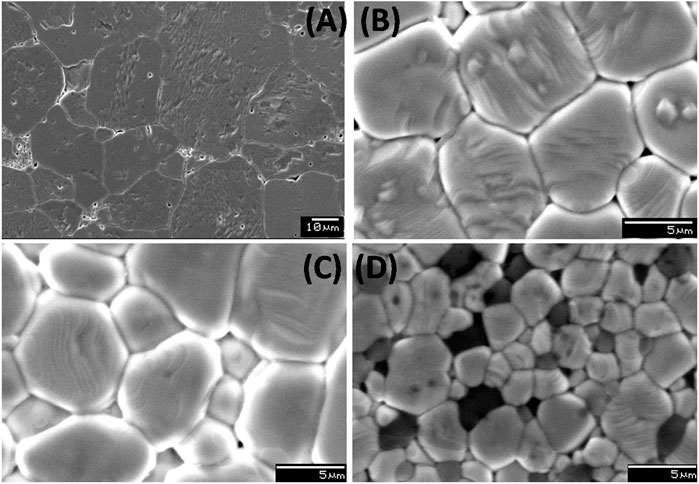
FIGURE 2. SEM images of BaZr0.2[Ti(1-x)Mgx/3Ta2x/3]0.8O3 solid solution: (A) x = 0.0, (B) x = 0.1, (C) x = 0.4, (D) x = 0.6.
Dielectric Measurements
Hard pellets with bulk density above 85% were used for dielectric measurements. Figure 3 show the variation of relative permittivity of BZTMT solid solution at a frequency of 1 MHz. The relative permittivity of the BaZr0.2[Ti(1-x)Mgx/3Ta2x/3]0.8O3 solid solution decreases with increase in (MgTa)4+ content. The room temperature relative permittivity of bare BZT was found to be 5,160 which decreases to 23 when the entire Ti4+ is replaced by (MgTa)4+ ions (x = 1.0). As the Ti4+ is replaced by (MgTa)4+ ions in BaZr0.2[Ti(1-x)Mgx/3Ta2x/3]0.8O3 the solid solution finally shifted to BaZr0.2[Mgx/3Ta2x/3]0.8O3 phase with very low relative permittivity value(Xu and Li, 2020). The microwave dielectric properties of the BZTMT solid solutions were recorded and tabulated in Table 1. The compositions from x = 0.0 to 0.04, which contains lower concentration of (MgTa)4+, does not show any resonance in the microwave region (marked as NR) due to the very high dielectric loss contribution from these paraelectric phases. However, as the concentration of (MgTa)4+ increases the material shows appreciable resonance and the x = 1.0 composition shows an excellent quality factor, Quxf of 66,500 GHz.
The dependence of frequency on the relative permittivity of BZTMT solid solutions were recorded for compositions x = 0, 0.05, 0.1, 0.2, 0.4 and depicted as Figure 4. All the compositions of BaZr0.2[Ti(1-x)Mgx/3Ta2x/3]0.8O3 ceramics shows frequency dependent relative permittivity, however the variation of relative permittivity with frequency is very small in with x = 0.4 composition. At low frequency region, all the polarization mechanisms such as interfacial, dipolar, ionic and electronic polarizations are active and results in high relative permittivity; as the frequency increases to 1 MHz the permittivity of all the samples decreases due to suppression of interfacial and dipolar polarization mechanisms(Mollá et al., 1999).
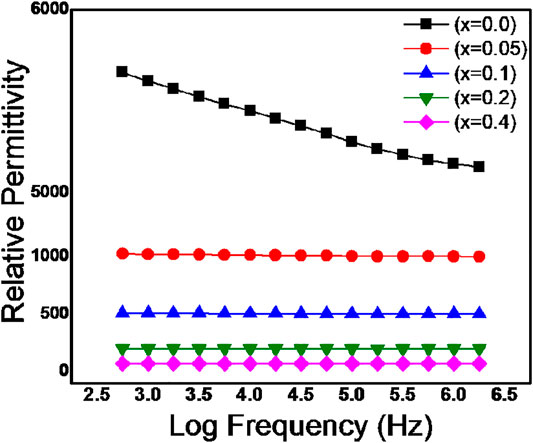
FIGURE 4. Variation of Relative Permittivity of BaZr0.2[Ti(1-x)Mgx/3Ta2x/3]0.8O3 for 0.0 < x < 0.4 with frequency.
The temperature dependence of relative permittivity of BaZr0.2[Ti(1-x)Mgx/3Ta2x/3]0.8O3 at various (MgTa)4+ concentrations were recorded at a frequency of 1 MHz and plotted as Figure 5. In BaZr0.2Ti0.8O3 (x = 0), the relative permittivity value increases with increase in temperature and reaches a maximum of 6,521 at 21°C and thereafter decreases. The temperature corresponding to this maximum value of relative permittivity is the Curie temperature (21°C) of this material which corresponds to the transition from tetragonal (ferroelectric) to cubic (paraelectric) phase of the material. However, it is seen that as the (MgTa)4+concentration increases curie temperature has shifted to lower temperature region and the corresponding relative permittivity value decreases. The relative permittivity at Curie temperature, -10°C for x = 0.025 was found to be 5,086. Instead of getting a single sharp Curie peak a broad maxima is observed for both composition which is due to the inhomogeneous distribution of M4+ ions in Ti4+ octahedral sites and the corresponding mechanical stresses created in the grains(Xu and Li, 2020). The ferroelectric to paraelectric phase transition for x = 0.05 composition has shifted to very low temperature region, thus the paraelectric phase is only visible at experimental temperature range (-50–80°C). The temperature dependence of relative permittivity is visible only upto x = 0.1 composition and above this concentration the material shows any temperature dependence.
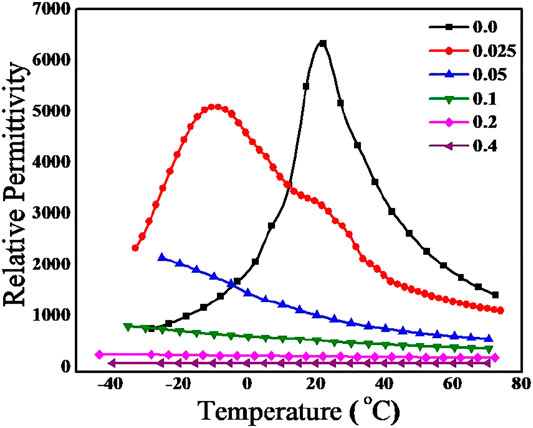
FIGURE 5. Variation of relative permittivity of BaZr0.2[Ti(1-x)Mgx/3Ta2x/3]0.8O3 for 0.0 < x < 0.4 with temperature at 1 MHz.
The stability of complex perovskite depends on the tolerance factor (tf) and is given by
Where, Ro is the radius of oxygen anion, RA and RB is the radius of A site and B site cation in ABO3 perovskite structure (Tejuca and Fierro, 1993; Reaney and Iddles, 2006) The tolerance factor calculated using Shannon’s ionic radii for all the compounds investigated in the present study are in the range of a stable perovskite (1.02< tf <1.05) (Shannon, 1976) The tolerance factors of all compositions are close to unity which is the characteristics of cubic perovskite structures. Figure 6 shows the variation of relative permittivity and quality factor of solid solution phases in BZTMT as a function of tolerance factors. It is evident from the figure that as the tolerance factor increases the quality factor gradually decreases. The composition with tolerance factors greater than 1.036 have high dielectric loss and do not resonate. It is evident that quality factor of the specimen increases with increase in concentration of Mg and Ta in BaZr0.2[Ti(1-x)Mgx/3Ta2x/3]0.8O3.
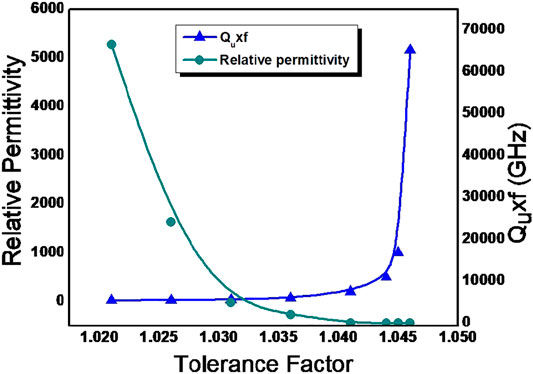
FIGURE 6. Variation of Relative permittivity and Quality factor with tolerance factor of BaZr0.2[Ti(1-x)Mgx/3Ta2x/3]0.8O3 solid solution phases.
Conclusion
The effect of (MgTa)4+ substitution on the ferroelectric to paraelectric transition and the microwave dielectric properties were investigated in BaZr0.2[Ti(1-x)Mgx/3Ta2x/3]0.8O3 [x = 0.0, 0.025, 0.05, 0.1, 0.2, 0.4, 0.6, 0.8, 1.0] solid solutions. The ferroelectric Curie temperature of BaZr0.2Ti0.8O3 (x = 0) has shifted from 21°C to -10°C on the addition of a small amount of dopant (x = 0.025). As the concentration of (MgTa)4+ increases the material changes from a lossy ferroelectric phase to a low loss dielectric phase. The microwave dielectric properties show that the temperature variation of resonant frequency (tf) and the quality factor (Quxf) of the composition with x = 1 are 15.8 and 66,500 GHz respectively.
Data Availability Statement
The original contributions presented in the study are included in the article/Supplementary Material, further inquiries can be directed to the corresponding authors.
Author Contributions
JK: Material synthesis, Investigation, Writing. GK: Conceptualization, Writing, Review. JV: Microwave characterization and analysis.
Conflict of Interest
The authors declare that the research was conducted in the absence of any commercial or financial relationships that could be construed as a potential conflict of interest.
Acknowledgments
JK acknowledges Jose James (late) and M. T. Sebastian former scientist, CSIR-NIIST, Trivandrum for providing the necessary facilities.
References
Buscaglia, M. T., Buscaglia, V., Viviani, M., Nanni, P., and Hanuskova, M. (2000). Influence of Foreign Ions on the Crystal Structure of BaTiO3. J. Eur. Ceram. Soc. 20 (12), 1997–2007. doi:10.1016/s0955-2219(00)00076-5
Chen, L., Wang, H., Xiong, X., Meng, H., and Zhang, J. (2014). Influence of Thermal History on Relaxation Process in Barium Titanate Ceramics. Ceramics Int. 40 (4), 6241–6245. doi:10.1016/j.ceramint.2013.11.080
Co, K., Khassaf, H., and Alpay, S. P. (2020). Electrocaloric and Pyroelectric Properties of Barium Zirconate Titanate. J. Appl. Phys. 127 (17), 174102. doi:10.1063/5.0003000
Hennigs, D., and Schnell, A. (1982). Diffuse Ferroelectric Phase Transition in Ba(Ti1-yZry)O3 Ceramics. J. Am. Ceram. Soc. 65, 539–544.
Krupka, J., Derzakowski, K., Riddle, B., and Baker-Jarvis, J. (1998). A Dielectric Resonator for Measurements of Complex Permittivity of Low Loss Dielectric Materials as a Function of Temperature. Meas. Sci. Technol. 9, 1751–1756. doi:10.1088/0957-0233/9/10/015
Li, W.-B., Zhou, D., and Pang, L.-X. (2017). Enhanced Energy Storage Density by Inducing Defect Dipoles in Lead Free Relaxor Ferroelectric BaTiO3-Based Ceramics. Appl. Phys. Lett. 110 (13), 132902. doi:10.1063/1.4979467
Li, Y., Wang, R., Ma, X., Li, Z., Sang, R., and Qu, Y. (2014). Dielectric Behavior of Samarium-Doped BaZr0.2Ti0.8O3 Ceramics. Mater. Res. Bull. 49, 601–607. doi:10.1016/j.materresbull.2013.10.001
Magnone, E., Kim, J. R., and Park, J. H. (2016). The Effect of the Hydrothermal Synthesis Variables on Barium Titanate Powders. Ceramics Int. 42 (8), 10030–10036. doi:10.1016/j.ceramint.2016.03.106
Maiti, T., Guo, R., and Bhalla, A. S. (2008). Structure-Property Phase Diagram of BaZrxTi1−xO3 SystemStructure-Property Phase Diagram of BaZrxTi1−xO3System. J. Am. Ceram. Soc. 91 (6), 1769–1780. doi:10.1111/j.1551-2916.2008.02442.x
Mollá, J., González, M., Vila, R., and Ibarra, A. (1999). Effect of Humidity on Microwave Dielectric Losses of Porous Alumina. J. Appl. Phys. 85, 1727–1730. doi:10.1063/1.369317
Penn, S. J., and McN Alford, N. (2000). “High Relative Permittivity, Low Loss Dielectric Resonator Materials,” EPSRC Final Report. London: EEIE, South Bank University
Ravez, J., and Simon, A. (2000). Spontaneous Transition from Relaxor to Ferroelectric State in New Lead- Free Perovskite Ceramics. Ferroelectrics 240, 313–320. doi:10.1080/00150190008227985
Reaney, I. M., and Iddles, D. (2006). Microwave Dielectric Ceramics for Resonators and Filters in Mobile Phone Networks. J. Am. Ceram. Soc. 89 (7), 2063–2072. doi:10.1111/j.1551-2916.2006.01025.x
Rout, S. K., Sinha, E., and Panigrahi, S. (2007). Dielectric Properties and Diffuse Phase Transition in Ba1−xMgxTi0.6Zr0.4O3 Solid Solutions. Mater. Chem. Phys. 101, 428–432. doi:10.1016/j.matchemphys.2006.08.002
Sangwan, K. M., Ahlawat, N., Kundu, R. S., Rani, S., Rani, S., Ahlawat, N., et al. (2018). Improved Dielectric and Ferroelectric Properties of Mn Doped Barium Zirconium Titanate (BZT) Ceramics for Energy Storage Applications. J. Phys. Chem. Sol. 117, 158–166. doi:10.1016/j.jpcs.2018.01.051
Shannon, R. D. (1976). Revised Effective Ionic Radii and Systematic Studies of Interatomic Distances in Halides and Chalcogenides. Acta Cryst. Sect A. 32 (5), 751–767. doi:10.1107/s0567739476001551
Surendran, K. P., and Sebastian, M. T. (2005). Low Loss Dielectrics in Ba[Mg1/3Ta2/3)1-xTix]O3 and Ba[(Mg1-xZnx)Ta2/3]O3 Systems. J. Mat. Res. 20 (11), 784–790. doi:10.1557/jmr.2005.0384
Tejuca, L. G., and Fierro, J. L. G. (1993). Properties and Applications of Perovskite Type Oxides. 1st Ed. New York: Marcel Decker
Venkatachalam, V., Vaidhyanathan, B., and Binner, J. (2020). Synthesis of Nanocrystalline Barium Titanate: Effect of Microwave Power on Phase Evolution. J. Eur. Ceram. Soc. 40, 3974-3983. doi:10.1016/j.jeurceramsoc.2020.04.043
Wang, B., Pu, Y.-P., Xu, N., Wu, H.-D., and Chen, K. (2012). Dielectric Properties of Barium Titanate-Molybdenum Composite. Ceramics Int. 38, S37–S40. doi:10.1016/j.ceramint.2011.04.044
Weber, U., Greuel, G., Boettger, U., Weber, S., Hennings, D., and Waser, R (2001). Dielectric Properties of Ba(Zr,Ti)O3-Based Ferroelectrics for Capacitor Applications. J. Am. Ceram. Soc. 84 (4), 759–766. doi:10.1111/j.1151-2916.2001.tb00738.x
Keywords: ferroelectrics, paraelectrics, solid solution, barium zirconium titanate, ceramics
Citation: Kavil J, Varghese J and Kovummal GR (2021) Dielectric Properties of BaZr0.2[Ti(1-x)Mgx/3Ta2x/3]0.8O3 Solid Solution. Front. Mater. 8:664675. doi: 10.3389/fmats.2021.664675
Received: 05 February 2021; Accepted: 27 April 2021;
Published: 12 May 2021.
Edited by:
Ashutosh Goel, Rutgers, The State University of New Jersey, United StatesReviewed by:
Andrei Kholkin, University of Aveiro, PortugalAhmad Safari, Rutgers, The State University of New Jersey, United States
Anupinder Singh, Guru Nanak Dev University, India
Copyright © 2021 Kavil, Varghese and Kovummal. This is an open-access article distributed under the terms of the Creative Commons Attribution License (CC BY). The use, distribution or reproduction in other forums is permitted, provided the original author(s) and the copyright owner(s) are credited and that the original publication in this journal is cited, in accordance with accepted academic practice. No use, distribution or reproduction is permitted which does not comply with these terms.
*Correspondence: Govind Raj Kovummal, Z292aW5kQG1jY2NsdC5hYy5pbg==; Jithesh Kavil, aml0aGVzaGthdmlsQHNuY29sbGVnZWthbm51ci5hYy5pbg==