- 1Key Laboratory of Eco-Textile, Ministry of Education, School of Textiles and Clothing, Jiangnan University, Wuxi, China
- 2School of Textile and Clothing, Yancheng Institute of Technology, Yancheng, China
- 3Electrochemical Innovation Lab, Department of Chemical Engineering, University College London, London, United Kingdom
Application of SiO2 aerogel in thermal protective clothing has been limited due to its brittle nature, ordinary mechanical properties, and poor film forming performance. This work is aimed to develop thermal protective cotton fabrics by coating blended OPU/SiO2 aerogel with enhanced mechanical properties and thermal protection performance. The OPU/SiO2 aerogel composites with different ratio were applied onto cotton fabrics by knife-coating. The morphology, chemical component, crystalline structure, thermal stability and compression strength were characterized by scanning electron microscopy (SEM), Fourier transfer Infrared spectroscopy (FTIR), X-ray diffraction (XRD), thermogravimetric analysis (TG) and compression test, respectively. Besides, the warmth retention performance and heat protection performance together with air and moisture permeability of the coated fabrics were studied. The results showed that OPU/SiO2 aerogel were successfully coated onto cotton fabrics with enhanced mechanical properties and thermal stability together with better film forming capacity. The heat transfer coefficient of the coated cotton fabrics was distinctly dropped due to the synergistic effect of OPU and SiO2 aerogel, which resulted in higher warmth retention. The OPU/SiO2 aerogel coated fabrics exhibited obvious heat insulation performance with its surface temperate almost 4 °C than the uncoated fabrics. This work demonstrates a new strategy of fabricating stronger thermal insulating textiles using OPU/SiO2 aerogel composites.
Introduction
Thermal protective clothing is essential for wearers who are subject to threats from environments such as extremely cold weather, special low temperature industrial environment and/or geometrically challenging locations (e.g., the polar region of the earth; Kothari and Chakraborty, 2016; Udayraj et al., 2016a, Udayraj et al., 2016b; Bogerd et al., 2017; Su et al., 2020). Heat is generally transferred via thermal conduction, thermal radiation and thermal convection (Abbasi et al., 2016; Hayat et al., 2016; Dai et al., 2018; Ge et al., 2019). The clothing system serves as the barrier between environment and human body, in which the fibrous materials and fibrous structure dominate the heat transfer from body to environment. Conventional thermal protective clothing is based on thermal insulating fibers, e.g., down fiber, with highly porous structure in the clothing system (Shu et al., 2017). These thermal protective products are functionally competent for daily wearing such as winter coat. However, there is a big challenge in protecting human from extreme environmental conditions, as under extreme environmental conditions the traditional thermal protective clothing is far from enough to ensure the safety and wellbeing of human. Thus, it is important to develop new thermal insulating materials with outstanding thermal insulation performance to withstand both thermal conduction and thermal radiation (Hu et al., 2018). Due to the limit of fiber materials in high level thermal protection, novel materials with excellent thermal protection performance are widely used in textiles to provide extra thermal protection. Coating of materials with extra performance onto textiles has been widely applied in protective clothing (Tian et al., 2016; Tao et al., 2017; Yang et al., 2017; Liu et al., 2018a; Asim et al., 2020). Active nanomaterials with excellent thermal protection have also been applied in the coated layers, providing nanocomposite solutions to the development of next-generation thermal protective clothing (Wang and Fan, 2014; Zábranský et al., 2016; Kim et al., 2017; Xu et al., 2017; Pakdel et al., 2019; Zheng et al., 2019).
SiO2 aerogel has been well noticed for its light weight, porosity and network structure, and it has shown a great potential in developing thermal insulating materials (Liu et al., 2016; Günay et al., 2018; Liu et al., 2018b; Liu et al., 2019; Lu et al., 2020). However, SiO2 aerogel shows low thermal blocking effects against Infrared radiation. To overcome this issue, additives to blocking Infrared radiation were added into SiO2 aerogel (Tang et al., 2016). It has always been a challenging proposition to load of SiO2 aerogel onto textiles and realize its application (Liu et al., 2016; Ye et al., 2016). In theory, SiO2 aerogel can achieve thermal protection due to its excellent performance. However, in practice SiO2 aerogel coated textiles have poor durability due to the imperfect combination between SiO2 aerogel and fibers (Rostami et al., 2021). Furthermore, SiO2 aerogel has a different shrinkage rate in the process of drying, resulting in cracks on the surface of the coated fabrics. Adhesives are widely used to overcome these issues, but the additive itself has a high thermal conductivity. As a result, finding a proper adhesive to enhance the cohesiveness of the coated layer with fibers and to maintain the excellent thermal protection of SiO2 aerogel is still a challenge.
Polyurethane has been widely used as coating layers for various objects, and it can be simply coated on textiles for protection against different threats from environment, e.g., wind, water, chemicals and fire (Moiz et al., 2016, 2017). However, the protection as provided by polyurethane is not enough for some professionals due to the limit of polyurethane in high-level protection against different threats from either environment or working locations. Thus, modification of polyurethane, such as nanocomposites (Lee and Saito, 2018), combining with other mechanisms and surface modification, has been the research topic in the area of protective clothing. As an example, optically active polyurethane (OPU) has been synthesized to enhance the thermal stability especially the thermal radiative protection of polyurethane. OPU has evident blocking and refracting effects to Infrared and visible lights, resulting in thermal radiative protection performance to the coated materials (Sedlacik et al., 2019). OPU has the potential to be an ideal adhesive film on textiles to serve different purposes.
In this study, thermal protective cotton fabrics were fabricated by coating blended OPU/SiO2 aerogel with enhanced mechanical properties and thermal protection performance. OPU has great film formation capacity, which makes up for the weakness of SiO2 aerogel poor mechanical properties. SiO2 aerogel has high thermal stability, and the combination with OPU improves the thermal stability and thermal protection performance of polyurethane. The OPU/SiO2 aerogel paste was then applied onto cotton fabrics by knife coating. The morphology, chemical component, crystalline structure, thermal stability and compression strength were characterized, and the warmth retention performance, heat protection performance and air and moisture permeability of the coated fabrics were all investigated in detail. The combination of OPU and SiO2 aerogel has synergistically enhanced the performance of both polyurethane and aerogel, resulting in a thermal insulating layer on cotton fabrics. The research results provide a technical basis for the preparation of aerogel insulation coated fabric and propose an approach to improve the thermal insulation performance of the coated fabric.
Experimental
Materials
The sample fabrics were pure cotton in woven structure. Optically active polyurethane and SiO2 aerogel were self-prepared according to the method as reported (Gurav et al., 2010; Yang et al., 2016). Dispersion agent SN-5040, wetting dispersant PE-100, thickening agent ASE-60 and defoaming agent were purchased from Kexingde Chemical Co., Ltd. (Yixing, China).
Preparation
First of all, 450 g deionized water, 5 g dispersion agent and 3 g wetting dispersant were added to a flask followed by high-speed dispersion for 10 min. SiO2 aerogel was then added under high-speed stirring for 1 h. After that, OPU was added under stirring at 40°C for 1 h. Then, thickening agent and defoaming agent were added to the mixture under low speed stirring for 1 h, resulting in OPU/SiO2 aerogel blended paste. The weight ratio of SiO2 aerogel and OPU in the blend was adjusted to be 1:5, 1:10, 1:15, 1:20, and 1:25, coded as SiO2/OPU-1, SiO2/OPU-2, SiO2/OPU-3, SiO2/OPU-4, and SiO2/OPU-5, accordingly.
Secondly, the cotton fabrics were knife-coated with the OPU/SiO2 aerogel blended paste. Cotton fabrics were immersed in the OPU/SiO2 aerogel blended paste under mechanical stirring at 40°C for 30 min. The fabrics were then placed on a Teflon molding sheet and the remaining OPU/SiO2 aerogel blended paste was poured on the surface of the fabrics. A knife was moving along the surface of the fabrics to ensure an even coating. The Teflon sheet with coated fabrics were then placed in an oven at 40°C for drying. In the end, the coated fabrics were peeled off from the molding sheet. The fabrication process of OPU/SiO2 aerogel coated fabrics is illustrated in Figure 1.
Characterization and Measurements
SEM images were obtained from Nova NanoSEM 450 field emission scanning electron microscope. The samples were gold-coated in a sputter coater before observation.
FTIR spectra were obtained from NEXUS-670 FTIR spectroscopy with ATR, wavelength range was 400–4,000 cm–1 and resolution <0.09 cm–1.
XRD patterns were obtained from X’Pert3 Powder X-ray diffraction tester (PANalytical, Netherland), with 2θ = 5°–80°.
TG curves were obtained from STA 449C TG-DSC thermal analyzer with nitrogen protection and temperature rising rate of 10°C/min in the range of 20–1,000°C.
Compression module was tested on RGD-5B compression module testing machine. Samples were in cylinder with radius of 20 mm and height of 20 mm, and the load was 0.5 kN/s. The force-displacement curves were drawn from the extracted data in a data analysis software (Origin).
The warmth retention of coated fabrics was tested on a plate warmth retention tester (YG606, Ningbo Textile Equipment Company, Ningbo, China). In the test, the size of the sample was 30 cm × 30 cm, and the temperature was 38 ± 0.5°C. The test was repeated for 5 times and the results were averaged. The warmth retention rate was calculated as the difference (in percentage) of heat loss of a hotplate due to covering of the coated fabrics, according to the testing standard GB11048-89.
The heat protection test was performed on a purpose-built apparatus in which the fabrics were mounted on the hotplate with a constant temperature of 46°C, and the surface temperature of fabrics was monitored during the test. The environment conditions were set as 25 ± 2% RH and 20°C.
The air permeability was tested on a fabric permeability tester (YG-461E, Ningbo Textile Equipment Company, Ningbo, China). The size of nozzle was 0.8 mm, power of suction ventilator 1,100 W, flow rate 120 m3/h. The size of sample was 20 cm2 and the pressure difference was 200 Pa. The air permeability was tested as the volume of the transported air through the unit area of the fabrics in a given timeframe (unit mL/cm2⋅s).
The moisture permeability was tested on the YG(B)216-II fabric moisture permeability tester (Wenzhou Darong Textile Equipment Co., Ltd., Wenzhou, China). The size of sample was 90 mm in diameter (round shape) and the environment conditions were 38°C and 90% RH with airflow speed of 0.3–0.5 m/s. The moisture permeability was expressed as the vapor diffusion rate through a unit of the surface of the tested fabric (unit g/m2⋅d).
Results and Discussion
Morphology
The OPU/SiO2 aerogel coating layer was formed on the surface of cotton fabric with the knife. The organic/inorganic blending of OPU and SiO2 aerogel has greatly enhanced the mechanical properties and membrane forming of the coated layer on cotton fabrics. Polyurethane has great film formation capacity to form an intact membrane on the surface of fabrics and to trap the SiO2 aerogel particles inside without falling off during usage.
Figure 2 shows the SEM images of pure SiO2 aerogel and OPU/SiO2 aerogel with different OPU concentrations. Pure SiO2 aerogel shows very small particle size with uniform distribution (Figure 2a). Coating of OPU/SiO2 aerogel on cotton fabrics has resulted in a membrane on the surface of the fabrics. However, larger particles of SiO2 aerogel can be observed in the blends exhibits, suggesting a low level of aggregation of SiO2 aerogel in the blends. As a typical phenomenon of nanoparticles in composites, the particles tend to aggregate due to the phase separation and poor interface connections in the composites. The SiO2 aerogel was subjected to extensive stirring in accompany with dispersion agent and water. Si-OH groups were subsequently formed on the surface of aerogel and the instability of these groups forced the aerogel to move to areas with lower surface tension, resulting in coupling between Si-OH groups and aggregations.
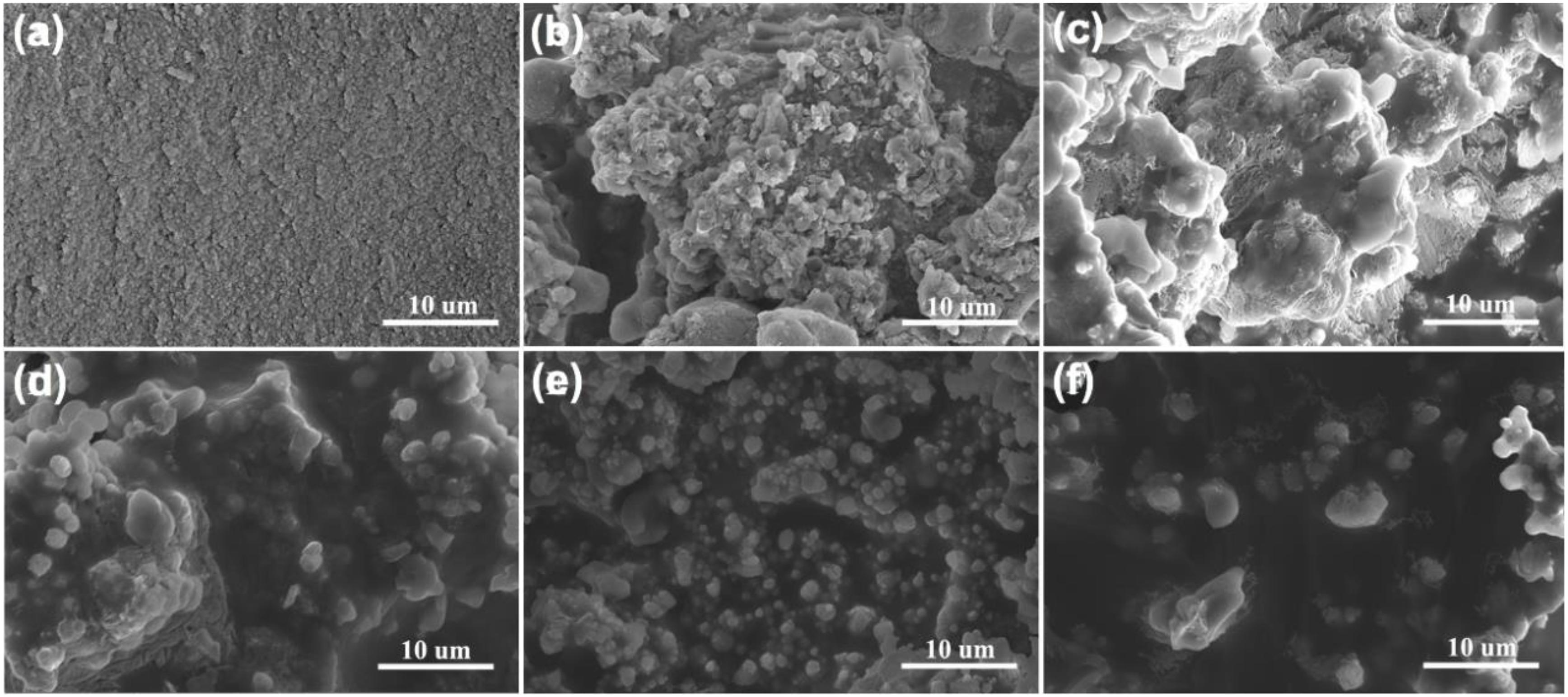
Figure 2. SEM images of pure SiO2 aerogel (a), SiO2/OPU-1 (b), SiO2/OPU-2 (c), SiO2/OPU-3 (d), SiO2/OPU-4 (e), and SiO2/OPU-5 (f).
Determining the optimized blending ratio of SiO2:OPU is the key to ensure that both OPU and SiO2 aerogel can play their roles in the composites. From Figures 2b–f it is clear that the aerogel turns to distribute more evenly under a high OPU concentration. As seen from Figures 2b,c, the aerogel exists mainly in aggregation form with a very uneven distribution. With the increase of OPU content in the composites, the size of aerogel became smaller with a more even distribution, without evident aggregation (Figures 2d–f). The reason is probably due to the stronger coupling effect between -NCO and H hydrion as compared to that with Si-OH, which disperses the aggregated aerogel during the preparation stage of OPU/SiO2 aerogel. Besides, under a high OPU ratio the excellent film forming capacity of polyurethane can slightly overcome the aggregation of aerogel with an improved film forming capacity of the coated layer. However, the viscosity of OPU/SiO2 aerogel increased gradually with the increase of the ratio of OPU in the composites. A ratio of higher than 1:25 for SiO2:OPU would result in too viscous blend for subsequent coating applications. From morphology study it can be found that a ratio of 1:20 is more suitable for preparing OPU/SiO2 aerogel with uniform distribution of aerogel and without aggregation.
FTIR Spectra
Figure 3A shows the FTIR spectra of OPU/SiO2 aerogel coated cotton fabrics with different blending ratios. All the spectra show a similar pattern with characteristic peaks of SiO2 and polyurethane. The obvious peak at 1,800 cm–1 is due to the stretching of Si-O-Si. The peak at 800 cm–1 is due to Si-C stretching, and the one at 1,245 cm–1 is corresponding to the C-H in Si-CH3. The peaks between 1,537 and 1,704 cm–1 are attributed to the absorbing peaks of benzene ring. The peak around 930 cm–1 is the absorbing peak of Si-H vibration, whereas the one at 2,963 cm–1 is the absorbing peak of the antisymmetric stretching vibration of C-H from aerogel. Besides, the absorbing peaks at 1,245 and 3,415 cm–1 are due to the stretching vibration of N-H, C=O and C-O from the urethane groups. FTIR analysis has revealed that both the chemical components of OPU and SiO2 aerogel can be detected from the coated fabrics, suggesting that both OPU and SiO2 aerogel were successfully loaded onto the fabrics.
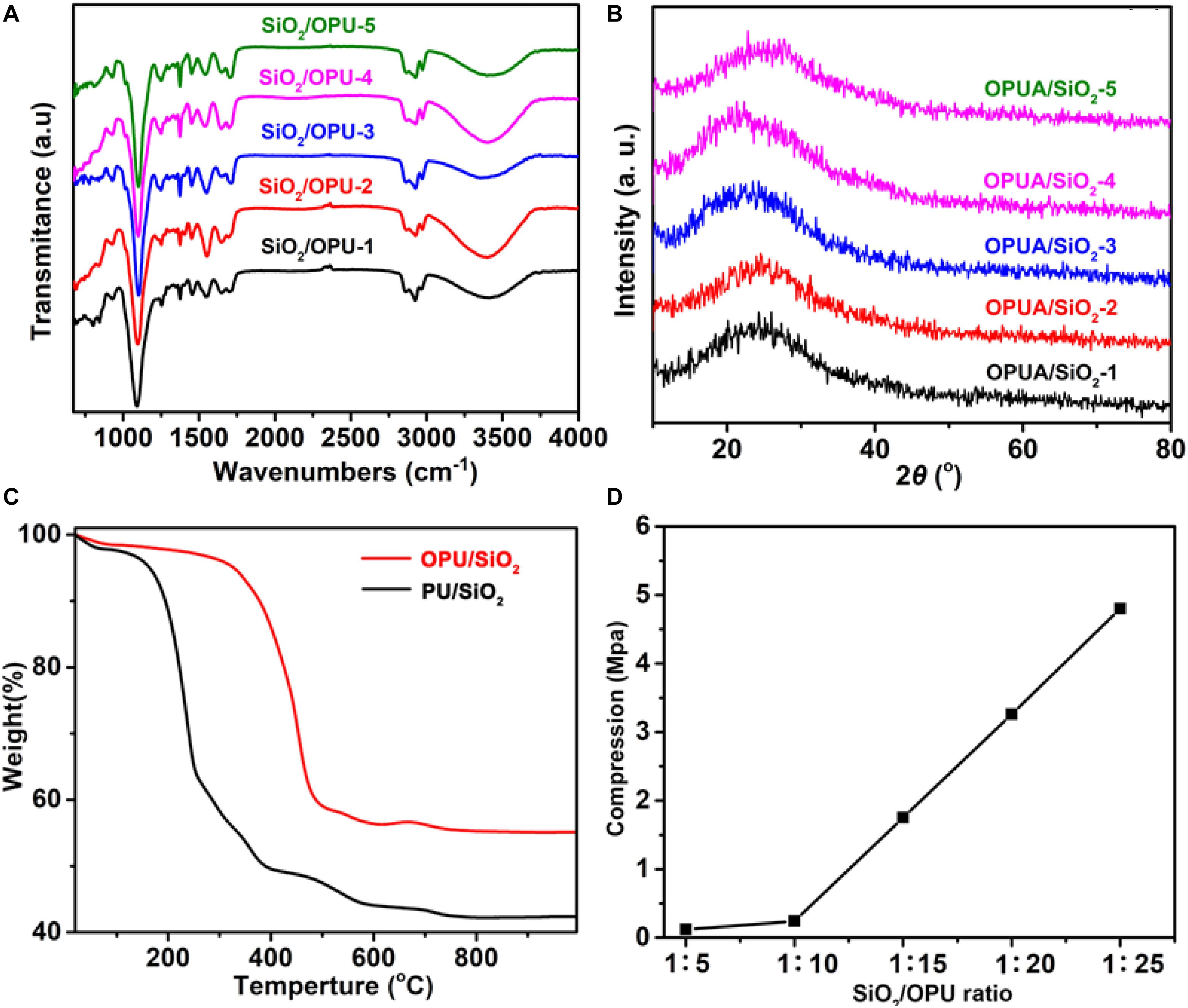
Figure 3. FITR spectra (A), XRD patterns (B), thermogravimetric curves (C), and compression strength (D) of SiO2/OPU.
XRD Patterns
Figure 3B shows the XRD patterns of OPU/SiO2 aerogel coated cotton fabrics with different blending ratios. Overall, the XRD patterns suggest the amorphous crystalline structure of the coated fabrics, which is typical crystalline structure for fibrous materials. From the XRD pattern curves, there is a broad non-crystalline characteristic peak at around 20°, and the intensity of the peak has found to be decreased with the increase of the amount of OPU in the blend. Apart from the peak at 20°, there are no other obvious crystalline peaks as observed from the patterns. Therefore, adding SiO2 aerogel to OPU hasn’t altered the amorphous crystalline structure of OPU. The coupling among Si-OH groups or between -NCO and H hydrion only resulted in changing of the size and aggregation form of aerogel but not the overall crystalline structure of OPU/SiO2 aerogel. Coating as a physical method to apply extra materials onto fabrics has little effect on the crystalline structure, thus the XRD patterns suggest no evident changes after coating.
TG Analysis
OPU has a better thermal stability than polyurethane due to its evident blocking and refracting effects to Infrared and visible lights (Sedlacik et al., 2019)., as a result OPU/SiO2 aerogel composites are supposed to exhibit better thermal stability than widely used PU/SiO2 aerogel. Figure 3C shows the TG curves of both OPU/SiO2 aerogel (1:15 ratio) and PU/SiO2 aerogel. Both curves show a weight loss at around 60°C owing to the evaporation of water and solvents. The main weight loss (47%) of PU/SiO2 aerogel occurred between 154 and 254°C, and in this stage the polymer chain of PU broke up and decomposed. However, the main weight loss (38%) of OPU/SiO2 aerogel occurred at the temperature of 385–490°C. The significance difference is due to the excellent thermal stability of OPU, which leads to a higher decomposing temperature of OPU/SiO2 aerogel. At a given weight loss of 60%, the decomposing temperature for PU/SiO2 aerogel and OPU/SiO2 aerogel is 280 and 485°C, respectively. These results show the enhanced thermal stability of OPU/SiO2 aerogel than SiO2/PU aerogel.
Compression Strength
The compression modulus of OPU/SiO2 aerogel was investigated, as shown in Figure 3D. It can be seen that the compression modulus of OPU/SiO2 aerogel has increased almost linearly with the increase of OPU ratio from 1:10 to 1:25. The compression modulus of OPU/SiO2 aerogel at 1:25 ratio is almost 5 MPa, which is almost 150 times of the compression modulus of pure SiO2 aerogel.
In OPU/SiO2 aerogels, the deformation is large whereas the compressive strength is small during the early compression process, which mainly due to the macroscopic pores in OPUS and SiO2 aerogels. The subsequent continuous compression may cause the collapse of SiO2 aerogel framework and chain break, resulting in crack and structure damage, and the compression strength and compressive strength gradually increase. After that, all the pores in the composite aerogel disappeared and the compressive strength showed a linear maximum. Besides, OPU also shows outstanding tenacity to further withstand external pressure. The OPU withstands the force to protect the brittle SiO2 aerogel in the composites, leading to a greatly enhancement of mechanical properties for OPU/SiO2 aerogel. Besides, OPU has tenacity to further withstand external pressure.
Warmth Retention
Fabrics coated with OPU/SiO2 aerogel showed excellent warmth retention with a low heat transfer coefficient. As presented in Figure 4A, the warmth retention rate for uncoated fabrics is 29.05%. However, the warmth retention rate of OPU/SiO2 aerogel coated fabrics is higher than 50%. Besides, the warmth retention increases gradually with the increase of OPU ratio in OPU/SiO2 aerogel. On the other hand, the heat transfer coefficient drops distinctly when the fabrics have been coated with OPU/SiO2 aerogel, and the coefficient decreases gradually with further increasing OPU ratio (Figure 4B).
It is noted that there is a fluctuation in both curves with the ratio increasing from 1:5 to 1:10. This is probably related to the heat transfer ways and the distribution together with the aggregation of SiO2 aerogel in the coated layer. When the OPU ratio is low, the thermal retention is mostly determined by SiO2 aerogel. The distribution of the aerogel has great impact on the overall thermal retention. As shown in the morphology study (Figure 2), evident aggregation can be found at the ratio of 1:5–1:10, leading to an uncertain thermal retention capacity. Besides, SiO2 aerogel has no evident thermal protection against radiant transfer, thus the heat transfer is slightly high when the OPU ratio is low. With the increase of OPU in the SiO2 aerogel, the thermal retention capacity is largely determined by OPU. OPU has an evident blocking effect to infrared, leading to its excellent thermal protection against radiation. Furthermore, the distribution of SiO2 aerogel becomes more even without obvious aggregation at a higher OPU ratio. As a result, the thermal protection effect of SiO2 aerogel has been strengthened. In the end, both OPU and SiO2 aerogel work collaboratively to generate excellent thermal retention capacity as shown in Figure 4.
Heat Protection
Figure 5 shows the surface temperature of OPU/SiO2 aerogel coated fabrics when they are attached to the hotplate of 46°C. It can be found that the surface temperature of the control fabrics increases immediately from 23.9 to 31.2°C after 10 min, and then the temperature further increases gradually to 41.1°C after 30 min. A similar trend can be found for the surface temperature of OPU/SiO2 aerogel coated fabrics. In the first 5–10 min, the surface temperature of OPU/SiO2 aerogel coated fabrics is almost the same and sometimes higher than that of the control fabrics.
However, the surface temperature of coated fabrics increases much slower than the rate for the control fabrics. As a result, the surface temperature of coated fabrics is lower than that of the control fabrics, and the difference becomes bigger and bigger with the increase of testing time. For the control fabrics, the surface temperature is 41.1°C after 30 min, a difference of 4.9°C to the hotplate (which is 46°C). But for the coated fabrics, the surface temperature can be as low as 37.2°C after 30 min, suggesting a difference of 8.8°C. It can be concluded that the surface temperature of coated fabrics is almost 4°C lower than that of uncoated fabrics. These results show that the OPU/SiO2 aerogel coated fabrics exhibit obvious heat insulation performance.
It is evident that the coated fabrics showed excellent warmth retention and heat protection as per the results. The mechanism of thermal protection performance of the coated fabrics can be explained as a combined effect of OPU and SiO2 aerogel. The membrane forming capacity of OPU has provided thermal protection to some extent as the pores of fabrics were largely blocked and thermal exchange between the fabrics and environment was decreased. The excellent thermal stability of SiO2 aerogel has provided thermal protection effect to the coated layer. Besides, the even distribution of SiO2 aerogel in the coated layer has maximized the thermal protection effect of SiO2 aerogel.
Air and Moisture Permeability
The permeability of fabrics is greatly affected by their thickness, pore size and porous structure of the coated layer. Figure 6A shows the air permeability of OPU/SiO2 aerogel coated fabrics. It can be seen that the air permeability decreases gradually with the increase of OPU ratio. Polyurethane has good membrane forming properties, thus the increase of OPU ratio in the OPU/SiO2 aerogel will result in a film on the fabric surface with most of the porous structure in SiO2 aerogel blocked. Besides, the thickness of coated fabrics increased from 0.54 to 0.61 mm with the increase of OPU ratio in the OPU/SiO2 aerogel. Thicker fabrics tend to exhibit a lower air permeability when other parameters are the same.
Figure 6B shows the moisture permeability of the coated fabrics. Similarly, the moisture permeability declines evidently with the increase of OPU ratio in the OPU/SiO2 aerogel. The excellent film forming performance of polyurethane has formed an intact membrane on the surface of fabrics after coating, and the porous structure of SiO2 aerogel has been altered with most of the pores blocked. The existence of OPU endows excellent water repellent performance to the coated fabrics. Therefore, the OPU/SiO2 aerogel coating has led to deteriorated moisture repellent capacity as per the results.
From the above results it can be seen that the coated fabrics have excellent compression strength, partly preserved permeability, and excellent warmth retention together with heat protection. These properties and performance have granted the coated fabrics with application potential as heat protective clothing, sportswear (for cold weather) and technical textiles.
Conclusion
OPU/SiO2 aerogel were successfully coated on cotton fabrics with enhanced mechanical properties and membrane forming capacity. Existence of OPU has significantly improved the film forming capacity and distribution of SiO2 aerogel in the coated layer. OPU/SiO2 aerogel coated fabrics showed a similar FTIR pattern with characteristic peaks of SiO2 and polyurethane. Adding SiO2 aerogel to OPU hasn’t altered the amorphous crystalline structure of OPU as seen from XRD patterns. The excellent thermal stability of OPU has led to a higher decomposing temperature and enhanced thermal stability of OPU/SiO2 aerogel as compared with PU/SiO2 aerogel. The compression modulus of OPU/SiO2 aerogel has increased almost linearly with the increase of OPU ratio from 1:10 to 1:25, reaching to almost 150 times of the compression modulus of pure SiO2 aerogel. The warmth retention increased gradually with the increase of OPU ratio in OPU/SiO2 aerogel, and the heat transfer coefficient decreased gradually with the increase of OPU ratio. The OPU/SiO2 aerogel coated fabrics exhibited obvious heat protection performance with its surface temperate almost 4°C than the uncoated fabrics. The air permeability of OPU/SiO2 aerogel coated fabrics decreased gradually with the increase of OPU ratio. Besides, the moisture permeability of OPU/SiO2 aerogel coated fabrics declined evidently with the increase of OPU ratio in the OPU/SiO2 aerogel. The development of OPU/SiO2 aerogel coated fabrics has showed new strategy of fabricating stronger thermally insulating textiles using OPU/SiO2 aerogel composites.
Data Availability Statement
The original contributions presented in the study are included in the article/supplementary material, further inquiries can be directed to the corresponding author/s.
Author Contributions
LL: data curation, investigation, and writing—original draft. ZL: methodology. CW: supervision. CW and WL: review and editing. All authors contributed to the article and approved the submitted version.
Funding
This work was supported by Natural Science Foundation of Jiangsu Province (BK20191045), the Natural Science Foundation of the Jiangsu Higher Education Institutions of China (19KJB150040), and the Industry-university-research Cooperation Projects (2020072109).
Conflict of Interest
The authors declare that the research was conducted in the absence of any commercial or financial relationships that could be construed as a potential conflict of interest.
References
Abbasi, F. M., Shehzad, S. A., Hayat, T., and Alhuthali, M. S. (2016). Mixed convection flow of jeffrey nanofluid with thermal radiation and double stratification. J. Hydrodynam. 28, 840–849. doi: 10.1016/s1001-6058(16)60686-8
Asim, M. T., Paridah, M., Chandrasekar, M., Shahroze, R., Jawaid, M., Nasir, M., et al. (2020). Thermal stability of natural fbers and their polymer composites. Iran. Polym. J. 29, 625–648. doi: 10.1007/s13726-020-00824-6
Bogerd, C. P., Langenberg, J. P., and DenHartog, E. A. (2017). A novel adjustable concept for permeable gas/vapor protective clothing: balancing protection and thermal strain. Ann. Work Expo. Health 62, 232–242. doi: 10.1093/annweh/wxx101
Dai, G. L., Shang, J., and Huang, J. P. (2018). Theory of transformation thermal convection for creeping flow in porous media: cloaking, concentrating, and camouflage. Phys. Rev. E. 97:022129. doi: 10.1103/physreve.97.022129
Ge, W. A., Zhao, C. Y., and Wang, B. X. (2019). Thermal radiation and conduction in functionally graded thermal barrier coatings. Part II: experimental thermal conductivities and heat transfer modeling. Int. J. Heat Mass Transf. 134, 166–174. doi: 10.1016/j.ijheatmasstransfer.2019.01.017
Günay, A., Kim, H., Nagarajan, N., Lopez, M., Kantharaj, R., Alsaati, A., et al. (2018). Optically optically transparent thermally insulating silica aerogels for solar thermal insulation. ACS Appl. Mater. Interfaces 10, 12603–12611.
Gurav, J. L., Jung, I. K., Park, H. H., Kang, E. S., and Nadargi, D. Y. (2010). Silica aerogel: synthesis and applications. J. Nanomater. 2010:409310.
Hayat, T., Nisar, Z., Yasmin, H., and Alsaedi, A. (2016). Peristaltic transport of nanofluid in a compliant wall channel with convective conditions and thermal radiation. J. Mol. Liq. 220, 448–453. doi: 10.1016/j.molliq.2016.04.080
Hu, F., Wu, S. Y., and Sun, Y. G. (2018). Hollow-structured materials for thermal insulation. Adv. Mater. 31:1801001. doi: 10.1002/adma.201801001
Kim, H., Ra, H. N., Kim, M., Kim, H. G., and Kim, S. S. (2017). Enhancement of barrier properties by wet coating of epoxy-ZrP nanocomposites on various inorganic layers. Prog. Org. Coat. 108, 25–29. doi: 10.1016/j.porgcoat.2017.04.001
Kothari, V. K., and Chakraborty, S. (2016). Protective performance of thermal protective clothing assemblies exposed to different radiant heat fluxes. Fiber. Polym. 17, 809–814. doi: 10.1007/s12221-016-5656-z
Lee, J. Y., and Saito, R. (2018). Polyurethane-silica nanocomposites provided from perhydropolysilazane: polymerization mechanism. Polymer 135, 251–260.
Liu, D. P., Ouyang, Q., Jiang, X. F., Ma, H. B., Chen, Y. S., and He, L. (2018a). Thermal properties and thermal stabilization of lignosulfonate-acrylonitrile-itaconic acid terpolymer for preparation of carbon fiber. Polym. Degrad. Stab. 150, 57–66. doi: 10.1016/j.polymdegradstab.2018.02.013
Liu, H. G., Xu, Y. D., Tang, C. L., Li, Y., and Chopra, N. (2019). SiO2 aerogel-embedded carbon foam composite with Co-Enhanced thermal insulation and mechanical properties. Ceram. Int. 45, 23393–23398. doi: 10.1016/j.ceramint.2019.08.041
Liu, H. L., Li, S. X., Li, H. Y., Chen, Z., Li, J., and Li, Y. J. (2018b). HNTs/SiO2 dual-network aerogels with improved strength and thermal insulation. J. Solgel Sci. Technol. 88, 519–527. doi: 10.1007/s10971-018-4851-3
Liu, Z. H., Ding, Y. D., Wang, F., and Deng, Z. P. (2016). Thermal insulation material based on SiO2 aerogel. Constr. Build. Mater. 122, 548–555.
Lu, Z. M., Strobach, E., Chen, N. X., Ferralis, N., and Grossman, C. J. (2020). Passive sub-ambient cooling from a transparent evaporation-insulation bilayer. Joule 4, 2693–2701. doi: 10.1016/j.joule.2020.10.005
Moiz, A., Padhye, R., and Wang, X. (2017). Coating of TPU-PDMS-TMS on polycotton fabrics for versatile protection. Polymers 9:660. doi: 10.3390/polym9120660
Moiz, A., Vijayan, A., Padhye, R., and Wang, X. (2016). Chemical and water protective surface on cotton fabric by pad-knife-pad coating of WPU-PDMS-TMS. Cellulose 23, 3377–3388. doi: 10.1007/s10570-016-1028-5
Pakdel, E., Naebe, M., Sun, L., and Wang, X. G. (2019). Advanced functional fibrous materials for enhanced thermoregulating performance. ACS Appl. Mater. Interfaces 11, 13039–13057. doi: 10.1021/acsami.8b19067
Rostami, J., Khandel, O., Sedighardekani, R., Sahneh, A. R., and Ghahari, S. (2021). Enhanced workability, durability, and thermal properties of cement-based composites with aerogel and paraffin coated recycled aggregates. J. Clean. Prod. 297:126518. doi: 10.1016/j.jclepro.2021.126518
Sedlacik, M., Osicka, J., Pavlinek, V., and Fojtl, L. (2019). The influence of ultraviolet radiation on the optical properties of glass fibre reinforcements for polyurethane matrix composites. Color. Technol. 135, 510–515. doi: 10.1111/cote.12443
Shu, Y., Li, M. S., and Shen, X. J. (2017). Fractal approach to structure and thermal property of down fiber assembly. J. Nat. Fibers 16, 853–859. doi: 10.1080/15440478
CrossRef Full Text 2017. 1376300Google Scholar
Su, Y., Zhu, W., Tian, M., Wang, Y. Y., Zhang, X. G., and Li, J. (2020). Intelligent bidirectional thermal regulation of phase change material incorporated in thermal protective clothing. Appl. Therm. Eng. 174:115340. doi: 10.1016/j.applthermaleng.2020.115340
Tang, G. H., Zhao, Y., and Guo, J. F. (2016). Multi-layer graded doping in silica aerogel insulation with temperature gradient. Int. J. Heat Mass Transf. 99, 192–200. doi: 10.1016/j.ijheatmasstransfer.2016.03.093
Tao, X., Zhang, L. Y., Ma, X. H., Xu, X. J., Guo, A. R., Hou, F., et al. (2017). Preparation of a flexible high emissivity coating on quartz fiber fabric for thermal protection. Ceram. Int. 43, 14292–14300.
Tian, W. L., Qi, L. H., Su, C. Q., Liu, J., and Zhou, J. M. (2016). Effect of fiber transverse isotropy on effective thermal conductivity of metal matrix composites reinforced by randomly distributed fibers. Compos. Struct. 152, 637–644.
Udayraj, Talukdar, P., Das, A., and Alagirusamy, R. (2016a). Heat and mass transfer through thermal protective clothing – a review. Int. J. Therm. Sci. 106, 32–56. doi: 10.1016/j.ijthermalsci.2016.03.006
Udayraj, Talukdar, P., Das, A., and Alagirusamy, R. (2016b). Simultaneous estimation of thermal conductivity and specific heat of thermal protective fabrics using experimental data of high heat flux exposure. Appl. Therm. Eng. 100, 788–797. doi: 10.1016/j.applthermaleng.2016.07.051
Wang, X., and Fan, J. T. (2014). Use of aluminum-coated interlayers to develop a cold-protective fibrous assembly. J. Appl. Polym. 131:40205. doi: 10.1002/app.40205
Xu, Y. X., Chen, L., Liu, Z. Q., Pei, F., and Du, Y. (2017). Improving thermal stability of TiSiN nanocomposite coatings by multilayered epitaxial growth. Surf. Coat. Technol. 321, 180–185. doi: 10.1016/j.surfcoat.2017.04.057
Yang, B., Wang, S., and Tang, Q. (2017). Geometry modeling and permeability prediction for textile preforms with nesting in laminates. Polym. Compos. 39, 4408–4415. doi: 10.1002/pc.24526
Yang, Y., Sun, C., Zhou, Y., Wang, T., and Zhang, Y. (2016). Optically active polyurethane based on tyrosine: synthesis, characterization and study of hydrogen bonding. Polym. J. 48, 807–812. doi: 10.1038/pj.2016.33
Ye, J. K., Bu, C. H., Han, Z. H., He, G., Li, J. T., and Chen, Y. X. (2016). Microstructural evolution and infrared radiation property of Ca2+-Cr3+ doped LaAlO3 in the presence of SiO2. Mater. Lett. 171, 55–58. doi: 10.1016/j.matlet.2016.02.031
Zábranský, L., Buršíková, V., Souček, P., Vašina, P., Dugáček, J., St́ahel, P., et al. (2016). Thermal stability of hard nanocomposite Mo-B-C coatings. Vacuum 138, 199–204. doi: 10.1016/j.vacuum.2016.12.016
Keywords: optically active polyurethane, SiO2 aerogel, coating, thermal insulating textiles, cotton fabrics
Citation: Lin L, Li Z, Mao H, Li W and Wang C (2021) Optically Active Polyurethane/Silica Aerogel Coated Cotton Fabrics for Thermal Protection. Front. Mater. 8:681678. doi: 10.3389/fmats.2021.681678
Received: 17 March 2021; Accepted: 25 March 2021;
Published: 21 April 2021.
Edited by:
Bomou Ma, Donghua University, ChinaCopyright © 2021 Lin, Li, Mao, Li and Wang. This is an open-access article distributed under the terms of the Creative Commons Attribution License (CC BY). The use, distribution or reproduction in other forums is permitted, provided the original author(s) and the copyright owner(s) are credited and that the original publication in this journal is cited, in accordance with accepted academic practice. No use, distribution or reproduction is permitted which does not comply with these terms.
*Correspondence: Wenyao Li, bGl3ZW55YW8zMTRAZ21haWwuY29t; Chaoxia Wang, d2FuZ2NoYW94aWFAc29odS5jb20=