Peptide self-assembled nanomedicine induces antitumor immunity by blocking the PD-1/PD-L1 axis
- 1Department of Breast Disease, Henan Breast Cancer Center, The Affiliated Cancer Hospital of Zhengzhou University and Henan Cancer Hospital, Zhengzhou, China
- 2Department of Pharmacology, School of Basic Medical Sciences, Zhengzhou University, Zhengzhou, Henan, China
- 3Department of Radiation Oncology, The Affiliated Cancer Hospital of Zhengzhou University and Henan Cancer Hospital, Zhengzhou, China
The development of immune checkpoint inhibitors (ICIs) revolutionizes cancer treatment, which has been applied in the treatment of several kinds of malignancies in the clinic. Due to their specific affinity and controllable cost, the emerging peptide-based ICI therapeutics have attracted great attention. However, peptide-based drugs generally show poor bioavailability and a short in vivo half-life. Peptide-based nanomedicine is a promising approach to increase the efficacy of therapeutic peptides. In this work, an amphiphilic peptide based on the reported D-peptide for ICIs has been developed. The constructed amphiphilic peptide can self-assemble into a stable nanostructure (noted as CD-NPs). The prepared CD-NPs have a spherical shape with a hydrodynamic size of about 35 nm. In vivo studies show that the prepared nanomedicine can effectively inhibit tumor growth in 4T1 tumor-bearing mice. Moreover, we found that the CD-NPs increase the infiltration of effector T cells and natural killer cells as well as the immune cytokines in the tumor tissues, implying that they activated the immune response for antitumor activity. Finally, no obvious changes in the major organs, tissues, or the body weight caused by the nanomedicine can be observed, demonstrating their good biocompatibility. Taken together, this study provides a basic idea for designing a novel peptide-based nanomedicine for enhanced antitumor efficacy by enhancing the blockade of ICIs and activating the immune response.
Introduction
Checkpoint blockade-based immunotherapy that evokes the host immunity to fight against cancers has resulted in unprecedented rates of effective responses in increasing kinds of malignancies (such as colon cancer and breast cancer) (Marin-Acevedo et al., 2018; Robert, 2020; Singh et al., 2020). Immune checkpoint inhibitors (ICIs) mainly suppressed the activity of negative regulatory molecules of immunity (such as cytotoxic T-lymphocyte-associated protein 4) or blocked the interactions between the checkpoint molecules (e.g., programmed cell death 1 (PD-1) or programmed cell death 1 ligand 1 (PD-L1)) and their corresponding ligands (Mahoney et al., 2015; Meng et al., 2015; Rezvani et al., 2017; Gong et al., 2021; Hu et al., 2021). Upon the checkpoint being blocked, the immune recognition and cancer surveillance of host immunity will be restored (Chen and Mellman, 2013; Dempke et al., 2017; Darvin et al., 2018). Among these ICI-based therapeutic drugs, PD-1 or PD-L1 antibodies have the most outstanding clinical treatment achievements (Acurcio et al., 2018; Li et al., 2020; Li et al., 2022). Despite encouraging clinical efficacy, the non-negligible safety risk caused by immune-related adverse events and high costs limited the population of patients who benefited from these therapeutics.
By virtue of the flexibility of sequence design, controllable preparation cost, and high affinity to specific biomarkers, peptides have plenteous application potential in mimicking antibodies in the clinic (Cui et al., 2010; Gautam et al., 2014; Delfi et al., 2021; Gong et al., 2021). Numerous efforts have been devoted to developing ICI-based peptides. With the effective phase display technology, Gao and coworkers screened a DPPA-1 peptide, which can competitively bind to PD-L1 and consequently block the PD-1/PD-L1 axis to restore the recognition and killing ability of immunity to cancer cells (Blank and Mackensen, 2007; Chang et al., 2015; Sun et al., 2018). However, facing the challenge in the pharmacokinetics of free peptides, such as short half-life time and vulnerability to serum peptidase in vivo, the developed DPPA-1 must be administered intratumorally, which limited its translational potential in the clinic.
Considering the weakness of free therapeutic peptides, chemical modifying of the hydrophilic peptide to be amphiphilic and consequently self-assembling into stable nanomicelles under physiological conditions are a practicable strategy (Zhao et al., 2014; Cheng et al., 2018; Du et al., 2018; Yu et al., 2021). By virtue of the advantage of nanosize, the self-assembled nanomicelles can accumulate in the tumor tissue by enhanced permeability and retention effect (Eskandari et al., 2017; Robert, 2020; Taleb et al., 2021). In this work, DPPA-1 was covalently modified with hydrophobic octadecanoic acid, and an amphiphilic peptide C18-DPPA-1 was obtained. As shown in Scheme 1A, the prepared C18-DPPA-1 can self-assemble into a stable spherical nanomicelle (noted as CD-NPs) with a hydrodynamic size of about 35 nm. After intravenously administered into 4T1 tumor-bearing mice, the CD-NPs can effectively suppress the growth of the tumor. Further research revealed that the CD-NPs can activate the host immunity of mice, including improving the infiltration of T lymphocytes (T cells) and natural killer (NK) cells in tumor tissues, along with increasing the level of antitumor immune cytokines, such as interferon-γ (IFN-γ) and interleukin 2 (IL-2) Scheme 1B. In addition, there is no obvious body weight loss in mice during the treatment period, and negligible changes in serum biochemical analysis and histopathological analysis of major organs can be observed. Taken together, this work developed a practical peptide-based nanomedicine for ICIs, which has an effective antitumor ability, as well as satisfying biocompatibility.
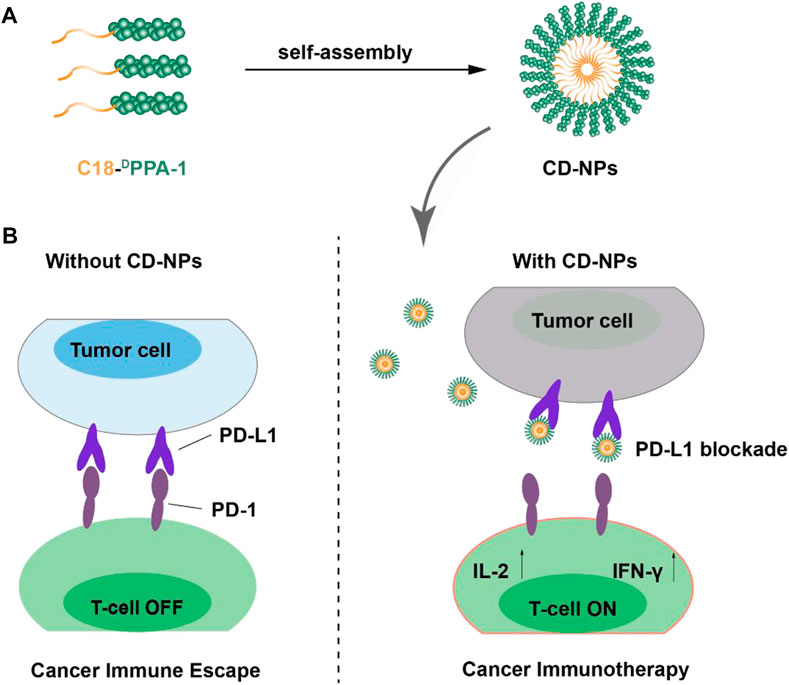
SCHEME 1. Schematic illustration of the preparation of CD-NPs and the antitumor mechanism of CD-NP-mediated cancer immunotherapy.
Materials and methods
Materials and reagents
Sterile PBS, RPMI-1640 cell culture media, and tissue-fixing buffer were purchased from Servicebio (Wuhan, China). The DPPA-1 and C18-DPPA-1 peptides were purchased from Anhui Guoping Pharmaceutical Co., Ltd. (Hefei, China). Trypsin and pentobarbital sodium were purchased from Solarbio Science & Technology Co., Ltd. (Beijing, China). The matrix gel was purchased from Corning (United States). Heparin sodium was purchased from Yuanye Bio-Technology Co., Ltd. (Shanghai). The fixation buffer, perm/wash, PE anti-mouse CD8α antibody, FITC anti-mouse CD3 antibody, PE/Cyanine7 anti-mouse IFN-γ antibody, Alexa Fluor® 488 anti-mouse CD49b antibody, and Alexa Fluor® 647 anti-mouse CD69 antibody were purchased from BioLegend (United States), and fetal bovine serum was purchased from Biological Industries. IFN-γ and IL-2 ELISA kits were purchased from Pengpai Bio-Technology Co., Ltd. (Shanghai).
Equipment
A 0–150 mm caliper (DeguoMNT), 5810R ultra-speed centrifuge (Eppendorf, German), DV215CD digital balance (OHAUS, United States), IVIS (PerkinElmer, United States), inverse microscope (Olympus, Japan), and digital sonicator (Jiekang, Dongguan) were used for this experiment.
Animals
BALB/c mice weighing 18–22 g (female, 6–8 weeks old) were purchased from the Animal Center of Zhengzhou University (Henan, China).
Preparation of self-assembled peptide nanomedicine
A measure of 1 mg of C18-DPPA-1 peptide was dissolved in 10 µL DMSO. Subsequently, the peptide was slowly distributed into 1 ml of PBS using a 1-ml syringe, followed by sonication for 15 min. Then, the mixture was placed at room temperature for 1–2 h to form peptide self-assembled nanomedicine.
Characterization of the peptide self-assembled nanomedicine
The morphology of the peptide self-assembled nanomedicine was characterized by transmission electron microscopy (TEM) as follows: briefly, 10 µL of the nanomedicine was dropped on the carbon-supported copper grid and allowed to sit for 10–15 min for air-drying. Then, 7 µl of uranyl acetate dihydrate was added onto the sample for staining purpose. After 3 min, the extra uranyl acetate dihydrate was absorbed gently using a piece of filter paper. When the dye dried, the sample on the copper grid was placed into the sample chamber of TEM for morphological study.
Antitumor efficiency study
To investigate the antitumor efficiency by the nanomedicine in vivo, the 4T1 tumor-bearing mouse model was constructed. When the tumor volume reached about 200 mm3, mice were separated into three groups randomly (n = 5), which were treated with PBS, DPPA-1 peptide, or CD-NPs every other day within 2 weeks. Before each injection of nanomedicine or controls, the body weights of mice and the lengths (L) and widths (W) of tumors were measured and recorded. The tumor volume was calculated using the equation: (L × W2)/2. In addition, luciferase substrate was intraperitoneally injected at days 7, 14, and 21 after tumor planting to monitor the tumor volume via the IVIS imaging system. After therapy, mice were euthanized for tumor weight and volume measurement.
Study of the population of T cells and NK cells in the tumor
After the treatment, mice were sacrificed. Tumors were harvested, and a small section of tumor in each group was lysed with tissue lysis buffer containing collagenase, hyaluronidase, and 1% trypsin at 37°C for 30 min, followed by termination of lysis with cell culture media containing 2% FBS to obtain the cell mixture, which was filtered through 70-µm mesh sieves for single-cell suspension. The single-cell suspension was stored for the analysis of immune cell populations. Briefly, a part of the single-cell suspension was incubated with anti-CD3-FITC and anti-CD8α antibodies on ice in the dark for 40 min. Then, the cells were washed twice with FBS-free media and permeabilized with 500 µL perm/wash buffer for 10 min. Cells were pelleted by centrifugation at 2, 000 g for 5 min. Next, the anti-IFN-γ-PE/Cy7 antibody was added according to the manufacturer’s protocol and incubated for another 40 min in the dark. Subsequently, the cells were washed twice and resuspended in 200 µl FBS-free media for the analysis of T-cell populations with CD8α+ or IFN-γ expression via flow cytometry. Similarly, another portion of the single-cell suspension was incubated with anti-mouse CD69-Alexa Fluor 647 and anti-mouse CD49b-Alexa Fluor 488 antibodies for the analysis of the NK-cell population via flow cytometry as mentioned previously.
Detection of immune cytokines in tumor
A small section of tumor tissue was lysed with highly efficient lysis buffer supplemented with a proteinase inhibitor cocktail and phosphatase inhibitor (10:1:1). After homogenization, the tissue suspension was centrifuged at 12,000 g for 20 min. The supernatant was reserved for ELISA assays to detect the immune-related factors, including IFN-γ and IL-2, according to the manufacturer’s protocol.
Assessment of the biosafety of CD-NPs
To evaluate the biosafety of CD-NPs, the body weights of mice were recorded every other day during the treatment within 2 weeks, and a curve was generated to monitor the weight changes. Moreover, murine blood was acquired before mice were harvested for the hematological and histopathological examination. Briefly, the blood was centrifuged at 4°C for 20 min, and plasma was reserved for blood biochemical analysis, including alanine aminotransferase (ALT) and aspartate aminotransferase (AST) as well as urea (UREA) and creatinine (CREA). In addition, the tumor was fixed and embedded with paraffin, which was cut for hematoxylin and eosin (H&E) staining to investigate histopathological changes at the end of the therapy.
Data analysis and statistics
All experiments were performed with five parallel samples unless elsewhere indicated, and the result was presented as the mean ± standard deviation (SD). All results were analyzed using GraphPad Prism 7, and Student’s t-test was used for statistical analysis (*p < 0.05; **p < 0.01; and ***p < 0.001).
Results and discussion
The preparation and characterization of CD-NPs
After modified with hydrophobic octadecanoic acid, the obtained C18-DPPA-1 becomes amphiphilic. When interacted with water molecules, the amphiphilic C18-DPPA-1 tends to expose its hydrophilic segments on the periphery to form an interface with water molecules, while keeps hydrophobic segments inward, consequently assembling into nanostructures. The morphology of the peptide self-assembled nanomedicine was characterized by TEM, and the results showed that the C18-DPPA-1 peptide can form homogenous nanoparticles with a spherical shape (Figure 1A). Also, the hydrodynamic sizes of these nanoparticles were measured with an average size of 35 nm (Figure 1B). The zeta potential of CD-NPs was measured to be c.a. −12.9 ± 1.8 mV. Furthermore, we evaluated the storage stability of CD-NPs. As shown in Figures 1D,E, there are negligible changes in size and zeta potential after incubation in PBS (pH 7.4) for 5 days, implying the good storage stability of CD-NPs.
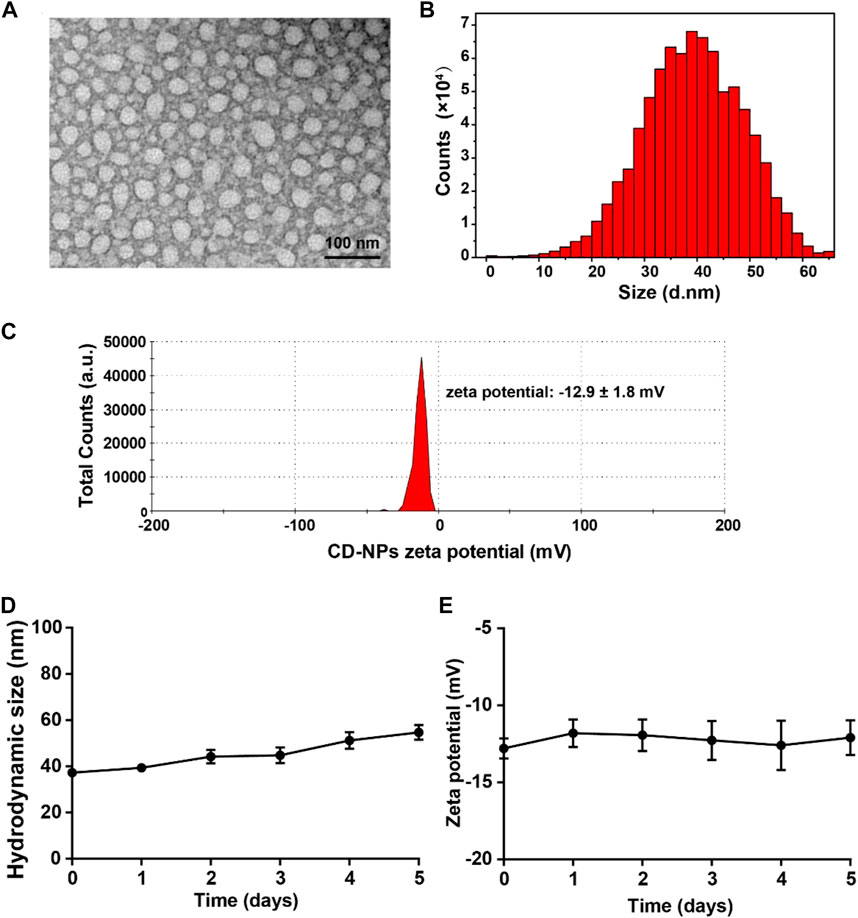
FIGURE 1. Characterization of the peptide self-assembled nanomedicine. (A) TEM images of prepared CD-NPs. (B) Hydrodynamic size distribution and (C) zeta potential of CD-NPs in PBS measured by DLS. (D) Changes in hydrodynamic size and (E) zeta potential of CD-NPs incubated in PBS for 5 days.
Evaluation of the antitumor efficacy of CD-NPs in vivo
It is well known that the blood clearance time is critical for drugs to work in vivo. Before evaluating the antitumor effect, we study the pharmacokinetics of prepared CD-NPs. The CY5 fluorescence dye was used to covalently label the DPPA-1 peptide and CD-NPs. After being intravenously injected into mice, the CY5-labeled DPPA-1 was quickly cleared (Figure 2A). Significantly, the prepared CD-NPs prolonged the circulation time with a half-life time of 1.45 h (CY5-CD-NPs) vs. 0.44 h (CY5-DPPA-1), which facilitates the antitumor response in vivo (Figure 2B). To visually monitor the antitumor effect, the bioluminescence images of mice were recorded during the whole trials. As shown in Figure 2C, the tumors in each group had similar size before the treatment and revealed distinct growing rates during the treatment. In addition, the analysis of bioluminescence signals demonstrated that the luciferase signal in the DPPA-1 group was 1.3 times higher than that in the CD-NP group, indicating that the DPPA-1 has the antitumor effect but not as good as the CD-NPs, which only had about half of the luciferase signal of the saline group with statistical significance in the tumor inhibition (Figure 2D). According to the tumor growth curves, we found that the tumor volumes in the group treated with saline reached about 1000 mm3 at the end of therapy, while the tumor volumes in the group treated with DPPA-1 were about 560 mm3, showing a mild tumor inhibition rate. In contrast, the tumor volumes of the group treated with CD-NPs were only about 350 mm3 (Figure 2E), which further demonstrated that the CD-NPs indeed had better antitumor efficacy. Similar inhibition tendency was observed in the tumor weight, as the results showing the average tumor weight in the CD-NP groups was only 1/2 and 3/5 of that in the saline group and DPPA-1 group, respectively (Figure 2F).
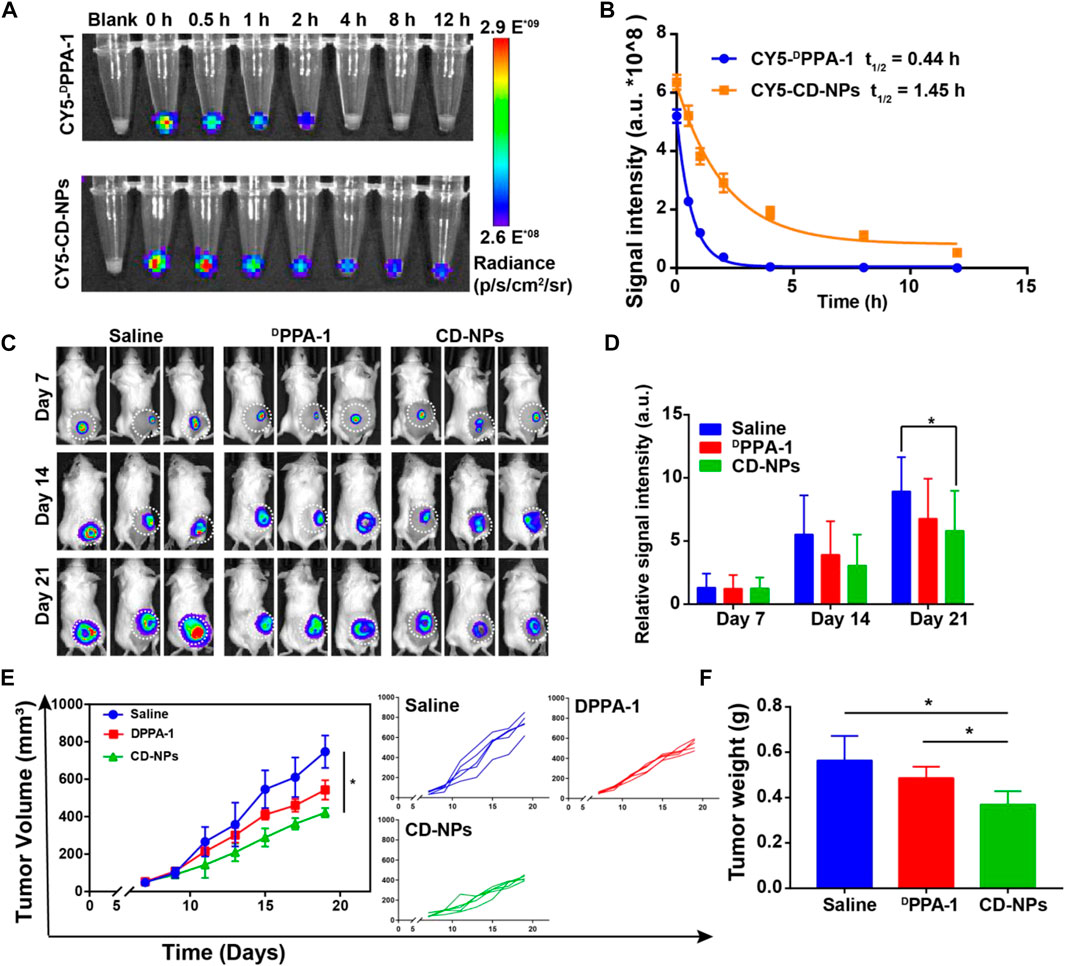
FIGURE 2. Pharmacokinetics and antitumor effects of CD-NPs in vivo. (A) Fluorescence images and (B) semi-quantitative analysis of blood collected at indicated time points from 4T1-luci tumor-bearing mice injected with CY5-labeled DPPA-1 or CD-NPs (n = 3). (C) Bioluminescence images and (D) corresponding semi-quantitative analysis of 4T1-luci tumor-bearing mice at indicated time points after treatment with different formulations (n = 3). (E) Growth curves of tumors from mice treated with various formulations. (F) Tumor weights of mice treated with various formulations (n = 5). *p < 0.05.
Evaluation of the antitumor immune response of CD-NPs in vivo
Given that the antitumor effect of nanomedicine is closely associated with the tumor-infiltrating immune cells in the tumor tissues, we further analyzed the tumor-infiltrating immune cell populations with flow cytometry to elucidate the mechanism of the higher antitumor efficacy of CD-NPs than the DPPA1 peptide observed in vivo. As shown in the results, CD8α-positive (CD8α+) T cells and IFN-γ-producing CD8α+ T-cell populations were obviously elevated to a level of 17.64% and 20.08%, respectively, which is approximately 4 times and 1.5 times of those in saline and DPPA-1 groups (Figures 3A–D). The enhanced infiltration of tumor immune cells could benefit from the unique characteristics of nanomedicine that they can extend the half-life and the retention in the tumor tissues. In addition, we observed a higher population of NK cells (CD69+ CD49b+) in the CD-NP group, which is 2.6 times and 1.8 times of that in the groups treated with saline and DPPA1, respectively (Figures 3E,F). Taken together, the peptide self-assembled nanomedicine could activate the immune response and increase the effector T cells and NK cells in the tumor microenvironment.
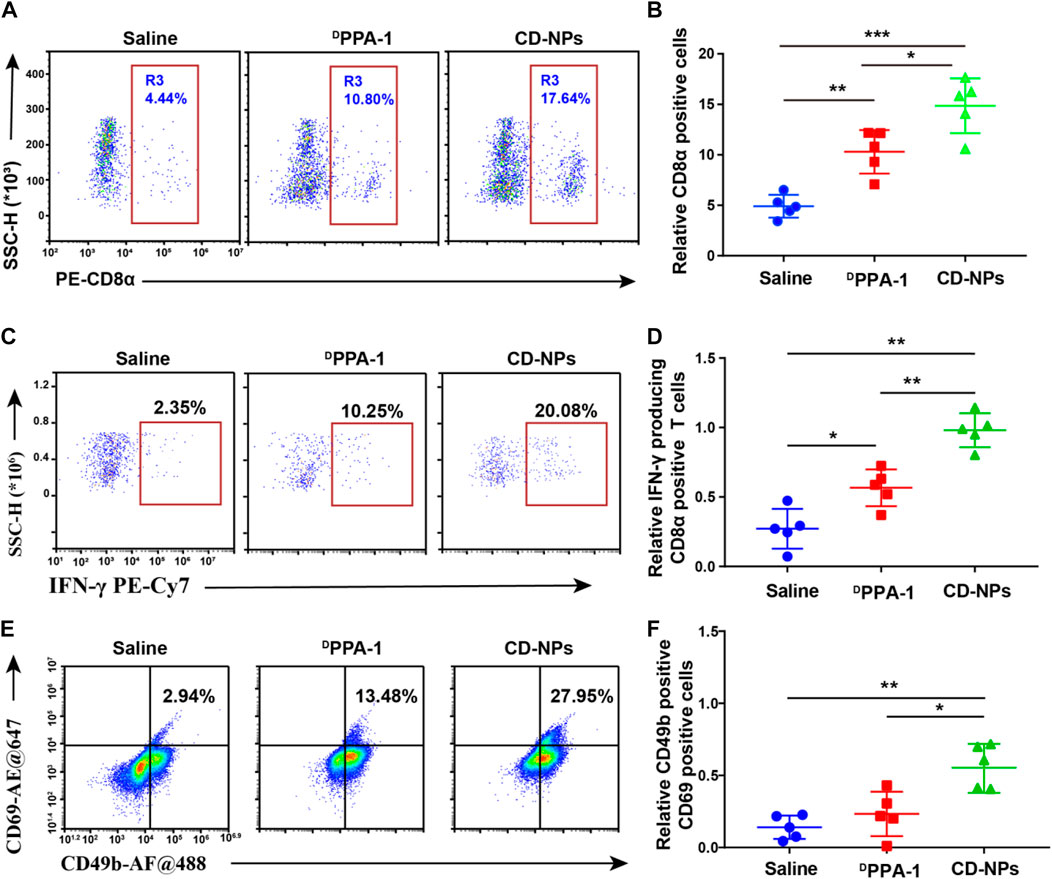
FIGURE 3. Evaluation of antitumor immune responses within tumor tissues. (A) Flow cytometry and (B) corresponding quantitative analysis of CD8α-positive T cells in the tumor tissue with indicated treatments. (C) Flow cytometry and (D) corresponding quantitative analysis of IFN-γ-producing CD8α-positive T cells in tumor tissue with indicated treatments. (E) Flow cytometry and (F) corresponding quantitative analysis of both CD69-positive and CD49-positive NK cells in the tumor tissue with indicated treatments. Data are shown as the mean ± SD; *p < 0.05, **p < 0.01, ***p < 0.001.
We further detected the intratumor immune-related cytokines to reveal the antitumor immune response activation of nanomedicine. The DPPA-1 was found to mildly increase the levels of IFN-γ and IL-2, while CD-NPs caused significant elevation in these cytokines (Figures 4A,B). This result demonstrated that the peptide-based nanomedicine can block the PD-1/PD-L1 interaction to enhance the antitumor effect by activating the immune response and increasing the immune cytokines in the tumor microenvironment.
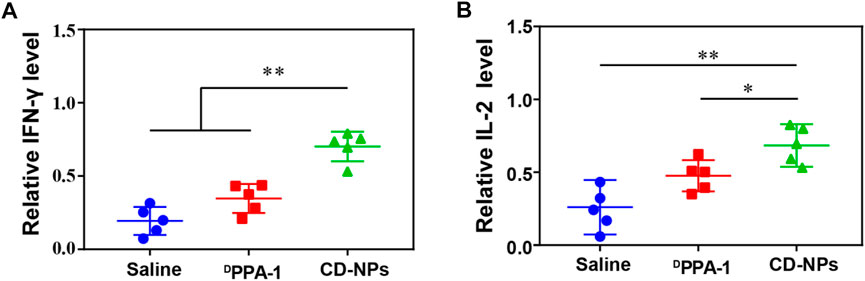
FIGURE 4. Analysis of intratumor immune cytokines. (A) Relative levels of IFN-γ and (B) IL-2 in the tumor tissue from mice treated with different formulations. Data are shown as the mean ± SD (n = 5); *p < 0.05, **p < 0.01.
Evaluation of the biosafety of CD-NPs in vivo
To assess the biosafety of CD-NPs in vivo, the body weights of mice in each group were recorded during the treatment, and no obvious changes were found in any groups during the treatment (Figure 5), indicating that CD-NPs barely impact the normal growth of mice.
In addition, murine blood was collected for the hematological and histopathological examination for monitoring the liver and kidney function. We found that there were no obvious changes in ALT and AST as well as the CREA and UREA levels (Figure 6), implying that the liver and kidney function was not impacted by CD-NPs. This result demonstrates that the CD-NPs do not cause liver or kidney toxicity.
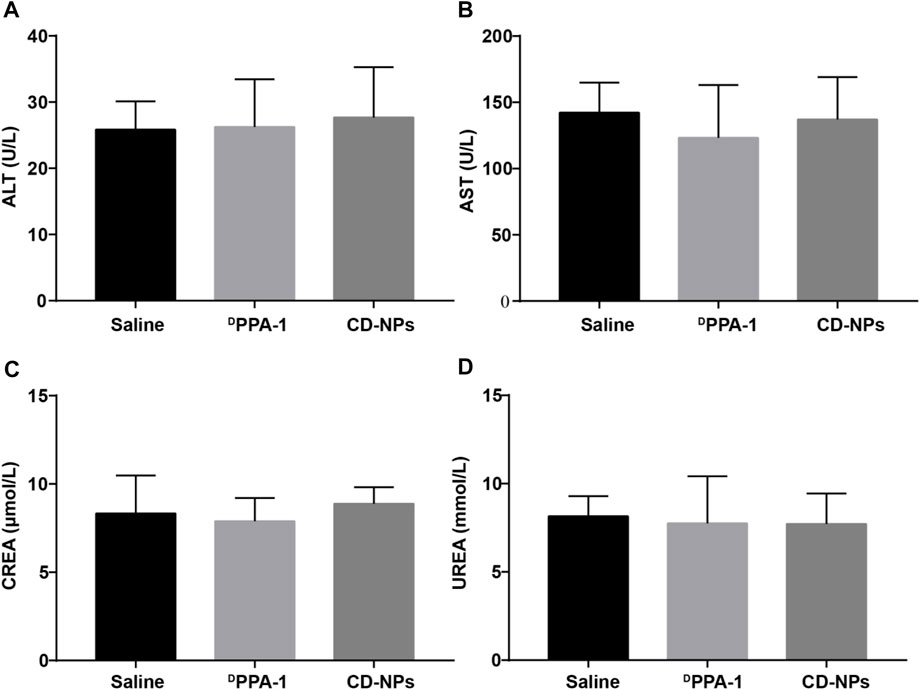
FIGURE 6. Blood biochemical level analysis of mice after treatments. Levels of (A) alanine aminotransferase (ALT) and (B) aspartate aminotransferase (AST) as well as (C) serum creatinine (CREA) and (D) urea (UREA) in blood from mice treated with different formulations. Data are shown as the mean ± SD (n = 3).
At the end of therapy, mice were sacrificed, and tissues including the heart, liver, spleen, lung, and kidney were harvested for hematoxylin and eosin (H&E) staining to investigate histopathological changes. No obvious changes in the tissues were observed (Figure 7), indicating that no harm to the major tissues was caused by CD-NPs during the treatment. Taken together, CD-NPs have good biocompatibility with minimal toxicity in vivo.
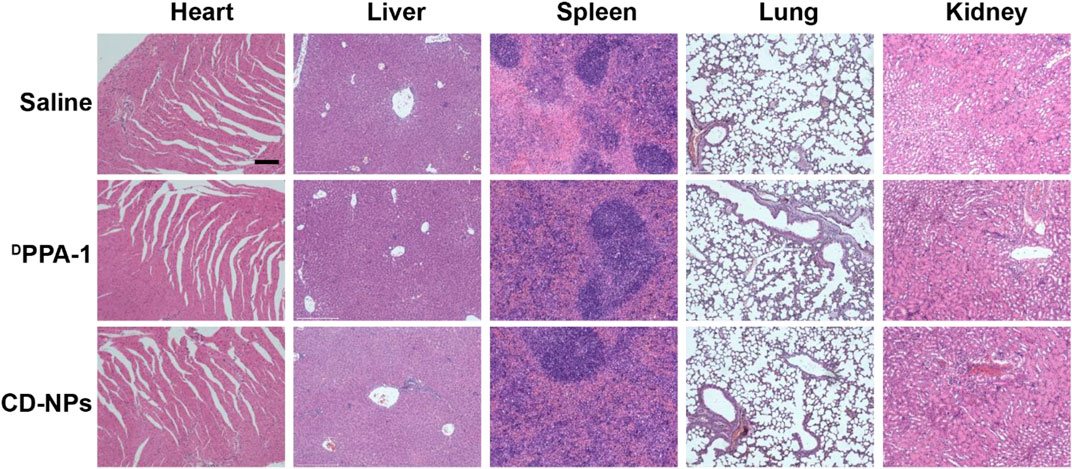
FIGURE 7. Representative images of H&E staining of hearts, livers, spleens, lungs, and kidneys of mice treated with different formulations. Scale bars, 200 μm.
Conclusion
In summary, this work constructed an amphiphilic peptide through a practical chemical modification based on DPPA-1 peptide. The amphiphilic peptide can self-assemble into a spherical nanomicelle (CD-NPs). The in vivo antitumor study showed that the prepared CD-NPs can effectively inhibit the growth of 4T1 tumors. Furthermore, immuno-analysis revealed that CD-NPs can evoke the host immunity through improving the infiltration of T cells and NK cells and elevating the level of antitumor immune cytokines in the tumor tissue. Moreover, good biocompatibility of CD-NPs was further confirmed through body weight monitoring, biochemical analysis, and histopathological examination. Taken together, this work developed practical peptide-based ICIs for tumor immunotherapy. Owing to the superiority of controllable cost, good storage stability, and significant antitumor activity, the prepared CD-NPs host great potentials for immunotherapy. However, the majority of tumor patients do not benefit from ICI-based therapy because of the “cold” tumor microenvironment and other tumor-associated factors. The development of a synergistic strategy to overcome the drawbacks of ICI-based therapy is of significance in the clinic in the future.
Data availability statement
The original contributions presented in the study are included in the article/Supplementary Material; further inquiries can be directed to the corresponding authors.
Ethics statement
The animal study was reviewed and approved by the Zhengzhou University Institutional Animal Care and Use Committee.
Author contributions
TH, YQ, and HG conceived and designed the experiments. XS, XM, MC, and YL performed the experiments and analyzed the data. TH, YQ, and SD wrote the original draft. YQ and HG supervised the project and revised the original draft. HG conceptualized and supervised the project. All authors have read and agreed to the published version of the manuscript.
Funding
This work was supported by the National Natural Science of Foundation of China (32000998), Natural Science Foundation of Chinese Postdoctoral (2019TQ0285 and 2019M662513), Science and Technology Joint Project of Henan Province (LHGJ20210210), and Science and Technology Development Project of Henan Province (222102310525 and 212102310138).
Acknowledgments
The authors would like to acknowledge the use of resources at the Center of Advanced Analysis and Gene Sequencing, Zhengzhou University, and the National Center for Nanoscience and Technology.
Conflict of interest
The authors declare that the research was conducted in the absence of any commercial or financial relationships that could be construed as a potential conflict of interest.
Publisher’s note
All claims expressed in this article are solely those of the authors and do not necessarily represent those of their affiliated organizations, or those of the publisher, the editors, and the reviewers. Any product that may be evaluated in this article, or claim that may be made by its manufacturer, is not guaranteed or endorsed by the publisher.
References
Acurcio, R. C., Scomparin, A., Conniot, J., Salvador, J. A. R., Satchi-Fainaro, R., Florindo, H. F., et al. (2018). Structure-function analysis of immune checkpoint receptors to guide emerging anticancer immunotherapy. J. Med. Chem. 61, 10957–10975. doi:10.1021/acs.jmedchem.8b00541
Blank, C., and Mackensen, A. (2007). Contribution of the PD-L1/PD-1 pathway to T-cell exhaustion: An update on implications for chronic infections and tumor evasion. Cancer Immunol. Immunother. 56, 739–745. doi:10.1007/s00262-006-0272-1
Chang, H. N., Liu, B. Y., Qi, Y. K., Zhou, Y., Chen, Y. P., Pan, K. M., et al. (2015). Blocking of the PD-1/PD-L1 interaction by a D-peptide antagonist for cancer immunotherapy. Angew. Chem. Int. Ed. 54, 11760–11764. doi:10.1002/anie.201506225
Chen, D. S., and Mellman, I. (2013). Oncology meets immunology: The cancer-immunity cycle. Immunity 39, 1–10. doi:10.1016/j.immuni.2013.07.012
Cheng, K., Ding, Y., Zhao, Y., Ye, S., Zhao, X., Zhang, Y., et al. (2018). Sequentially responsive therapeutic peptide assembling nanoparticles for dual-targeted cancer immunotherapy. Nano Lett. 18, 3250–3258. doi:10.1021/acs.nanolett.8b01071
Cui, H. G., Webber, M. J., and Stupp, S. I. (2010). Self-assembly of peptide amphiphiles: From molecules to nanostructures to biomaterials. Biopolymers 94, 1–18. doi:10.1002/bip.21328
Darvin, P., Toor, S. M., Nair, V. S., and Elkord, E. (2018). Immune checkpoint inhibitors: Recent progress and potential biomarkers. Exp. Mol. Med. 50, 1–11. doi:10.1038/s12276-018-0191-1
Delfi, M., Sartorius, R., Ashrafizadeh, M., Sharifi, E., Zhang, Y. P., De Berardinis, P., et al. (2021). Self-assembled peptide and protein nanostructures for anti-cancer therapy: Targeted delivery, stimuli-responsive devices and immunotherapy. Nano Today 38, 101119. doi:10.1016/j.nantod.2021.101119
Dempke, W. C. M., Fenchel, K., Uciechowski, P., and Dale, S. P. (2017). Second- and third-generation drugs for immuno-oncology treatment-The more the better? Eur. J. Cancer 74, 55–72. doi:10.1016/j.ejca.2017.01.001
Du, C., Qi, Y., Zhang, Y., Wang, Y., Zhao, X., Min, H., et al. (2018). Epidermal growth factor receptor-targeting peptide nanoparticles simultaneously deliver gemcitabine and olaparib to treat pancreatic cancer with breast cancer 2 (BRCA2) mutation. Acs Nano 12, 10785–10796. doi:10.1021/acsnano.8b01573
Eskandari, S., Guerin, T., Toth, I., and Stephenson, R. J. (2017). Recent advances in self-assembled peptides: Implications for targeted drug delivery and vaccine engineering. Adv. Drug Deliv. Rev. 110, 169–187. doi:10.1016/j.addr.2016.06.013
Gautam, A., Kapoor, P., Chaudhary, K., Kumar, R., Raghava, G. P. S., and Open Source Drug Discovery, C. (2014). Tumor homing peptides as molecular probes for cancer therapeutics, diagnostics and theranostics. Curr. Med. Chem. 21, 2367–2391. doi:10.2174/0929867321666140217122100
Gong, N. Q., Sheppard, N. C., Billingsley, M. M., June, C. H., and Mitchell, M. J. (2021). Nanomaterials for T-cell cancer immunotherapy. Nat. Nanotechnol. 16, 25–36. doi:10.1038/s41565-020-00822-y
Hu, C., He, X. Q., Chen, Y. X., Yang, X. T., Qin, L., Lei, T., et al. (2021). Metformin mediated PD-L1 downregulation in combination with photodynamic-immunotherapy for treatment of breast cancer. Adv. Funct. Mat. 31, 2007149. doi:10.1002/adfm.202007149
Li, Z., Ding, Y., Liu, J., Wang, J., Mo, F., Wang, Y., et al. (2022). Depletion of tumor associated macrophages enhances local and systemic platelet-mediated anti-PD-1 delivery for post-surgery tumor recurrence treatment. Nat. Commun. 13, 1845. doi:10.1038/s41467-022-29388-0
Li, Z., Wang, Y., Shen, Y., Qian, C., Oupicky, D., and Sun, M. (2020). Targeting pulmonary tumor microenvironment with CXCR4-inhibiting nanocomplex to enhance anti–PD-L1 immunotherapy. Sci. Adv. 6, eaaz9240. doi:10.1126/sciadv.aaz9240
Mahoney, K. M., Freeman, G. J., and McDermott, D. F. (2015). The next immune-checkpoint inhibitors: PD-1/PD-L1 blockade in melanoma. Clin. Ther. 37, 764–782. doi:10.1016/j.clinthera.2015.02.018
Marin-Acevedo, J. A., Dholaria, B., Soyano, A. E., Knutson, K. L., Chumsri, S., and Lou, Y. Y. (2018). Next generation of immune checkpoint therapy in cancer: New developments and challenges. J. Hematol. Oncol. 11, 39. doi:10.1186/s13045-018-0582-8
Meng, X. J., Huang, Z. Q., Teng, F. F., Xing, L. G., and Yu, J. M. (2015). Predictive biomarkers in PD-1/PD-L1 checkpoint blockade immunotherapy. Cancer Treat. Rev. 41, 868–876. doi:10.1016/j.ctrv.2015.11.001
Rezvani, K., Rouce, R., Liu, E. L., and Shpall, E. (2017). Engineering natural killer cells for cancer immunotherapy. Mol. Ther. 25, 1769–1781. doi:10.1016/j.ymthe.2017.06.012
Robert, C. (2020). A decade of immune-checkpoint inhibitors in cancer therapy. Nat. Commun. 11, 3801. doi:10.1038/s41467-020-17670-y
Singh, S., Hassan, D., Aldawsari, H. M., Molugulu, N., Shukla, R., and Kesharwani, P. (2020). Immune checkpoint inhibitors: A promising anticancer therapy. Drug Discov. Today 25, 223–229. doi:10.1016/j.drudis.2019.11.003
Sun, Z. L., Zhang, Y., Cao, D., Wang, X. F., Yan, X. B., Li, H., et al. (2018). PD-1/PD-L1 pathway and angiogenesis dual recognizable nanoparticles for enhancing chemotherapy of malignant cancer. Drug Deliv. 25, 1746–1755. doi:10.1080/10717544.2018.1509907
Taleb, M., Atabakhshi-Kashi, M., Wang, Y., Rezavani Alanagh, H., Farhadi Sabet, Z., Li, F., et al. (2021). Bifunctional therapeutic peptide assembled nanoparticles exerting improved activities of tumor vessel normalization and immune checkpoint inhibition. Adv. Healthc. Mat. 10, 2100051. doi:10.1002/adhm.202100051
Yu, L., Dou, S. B., Ma, J. H., Gong, Q., Zhang, M. G., Zhang, X. Q., et al. (2021). An antimicrobial peptide-loaded chitosan/polyethylene oxide nanofibrous membrane fabricated by electrospinning technology. Front. Mat. 8. doi:10.3389/fmats.2021.650223
Keywords: immune checkpoint inhibitors (ICIs), nanomedicine, antitumor immunity, peptides, PD-L1, PD-1
Citation: Huang T, Sun X, Meng X, Chen M, Li Y, Du S, Qi Y and Ge H (2022) Peptide self-assembled nanomedicine induces antitumor immunity by blocking the PD-1/PD-L1 axis. Front. Mater. 9:1056600. doi: 10.3389/fmats.2022.1056600
Received: 29 September 2022; Accepted: 21 November 2022;
Published: 02 December 2022.
Edited by:
Ruirui Qiao, Australian Institute for Bioengineering and Nanotechnology, University of Queensland, AustraliaReviewed by:
Zhaoting Li, University of Wisconsin-Madison, United StatesHonglin Jin, Huazhong Agricultural University, China
Hao Song, Australian Institute for Bioengineering and Nanotechnology, University of Queensland, Australia
Copyright © 2022 Huang, Sun, Meng, Chen, Li, Du, Qi and Ge. This is an open-access article distributed under the terms of the Creative Commons Attribution License (CC BY). The use, distribution or reproduction in other forums is permitted, provided the original author(s) and the copyright owner(s) are credited and that the original publication in this journal is cited, in accordance with accepted academic practice. No use, distribution or reproduction is permitted which does not comply with these terms.
*Correspondence: Hong Ge, gehong616@126.com; Yingqiu Qi, qiyq@zzu.edu.cn