- 1Beijing Key Laboratory of Traffic Engineering, College of Metropolitan Transportation, Faculty of Architecture, Civil, and Transportation Engineering, Beijing University of Technology, Beijing, China
- 2Professor, School of Highway, South Erhuan Middle Section, Chang’an University, Xi’an, China
- 3Professor, School of Civil Engineering and Transportation, Beijing University of Civil Engineering and Architecture, Beijing, China
- 4Professor, Department of Civil and Environmental Engineering, Michigan Technological University, Houghton, MI, United States
Asphalt mixtures are commonly used in pavement engineering, especially for highway construction. The mechanism exploration and analysis of pavement distress are the main challenges for researchers and industry managers. Thereby, it is important to understand their properties and interaction mechanisms in asphalt mixtures. It is difficult to conduct some sophisticated or microscale tests in the laboratory, and numerical simulation and virtual tests can be solutions for these cases with low costs. The Discrete Element Method (DEM) is a promising tool for researchers to undertake these tasks. This paper mainly summarized and analyzed the research progress and development prospect of DEMs in asphalt mixtures from a series of technical sections. The laboratory test results were often used to calibrate the DEM simulations as well as Two-dimensional (2D) and Three-dimensional (3D) modeling. Several modeling methods were developed to generate digital samples, like user-defined, image-based, random-modeling. In addition, the conclusions can be referenced by researchers for the development of numerical simulations.
1 Introduction
Generally, the behaviors of materials can be evaluated from the performance of asphalt mixtures, such as high-temperature stability, low-temperature crack resistance, durability, workability, etc., The laboratory test method is a good way to understand the properties of asphalt mixtures. Also, another analysis way is the numerical simulation method to get the fundamental views and insights. Different numerical simulation methods are gradually emerged to study the properties of asphalt mixtures, such as the Finite Element Method (FEM), Discrete Element Method (DEM), Molecular Dynamics (MD) method, etc., Three numerical methods focus on the different scales. The time scale is from femtosecond to second, and the length scale is from meter to Angstrom. The time scale and length scale of the DEM is microsecond and micrometer, respectively. The DEM is a suitable numerical simulation method to analyze the interactions in asphalt mixtures at the microscale. Many tests cannot deal with the interaction between materials at the microscale, but the DEM is a good way to explore mechanisms at the microscale. Therefore, many scholars used the DEM to research the properties of asphalt mixtures in recent years. The following discussions relate to applications of the DEM in asphalt mixtures.
Generally speaking, the research on the performance of asphalt mixture mainly starts from the macroscopic and microscopic aspects. Firstly, from the macroscopic perspective, the experimental method is a more traditional method to study the performance of asphalt mixtures, and it is also the most widely used method. It is controlled by humans in the laboratory, and its advantage is strong intuitiveness. 1) Although some errors cannot be avoided during the test process, the test results are still generally more reliable from realistically thinking. However, the costs of some tests are high and the operation process is also complicated and time-consuming. 2) During data collection in the tests, indirect and direct measures can be collected, like stress and strain data. However, the stress and strain values of each point in the entire material cannot be obtained directly and the route of the force transfer cannot be directly demonstrated. It indicates that the mechanisms or operation ways are not visible for the researchers during laboratory tests. Therefore, different numerical methods are invented and developed for different purposes.
Compared with the experimental method, the advantages of numerical simulations are 1) the cost is low and most cases in the tests can be simulated using the computer resource; 2) any type or amount of loading can be applied without worrying about the actual loss caused by the excessive load; 3) some extreme situations cannot be achieved and done by the experimental method and they can also be applied in the numerical methods. On the other hand, the numerical simulation method can analyze the stress of each area and measuring point of the test materials, and get the change of stress and strain, as well as the force and displacement distributions. The test results can be obtained quickly through certain operation settings, but the numerical simulation method is not perfect, and there are some shortcomings, such as abstraction, intuition, credibility, etc., The accuracy of the simulation can make certain predictions about the experiment results. The numerical simulation method mainly refers to the finite element method and DEM, which are the most commonly used. The DEM is used to solve the problems of discontinuous media by analyzing the interlocking contacts of discrete elements. The constitutive relation of the contact is found out and the physical and mechanical model of contact is established. Then the discontinuous and discrete elements are simulated according to Newton’s second law of motion. The DEM can better explain the stress or loading types, deformation mechanism, and failure processing of the asphalt mixtures from the meso and micro perspectives. It can also be used to predict rutting initiations, fatigue mechanisms, and engineering evaluation indexes for asphalt mixtures. However, the finite element method is not good for large and discontinuous deformations and is more suitable for the study of continuum problems. Therefore, the researchers tend to use the DEM to simulate the stress-strain relationships, damage situations, and fracture processing of the asphalt mixtures. The examples of specific studies on discrete elements are discussed as follows:
The parameters of the generalized Burgers model fitted by Mao (2018) were used to simulate the uniaxial test of asphalt mortars with a three-dimensional (3D) DEM. Although the results had deviations from the tests, this simulation provided a good attempt to understand the asphalt mortars and their behaviors. The scholars used the 3D DEM to simulate the porosity changes of asphalt mixtures under the dynamic loading and summarize the changing laws Zhang and Li (2017). The results showed that the DEM was an effective way to simulate elastic-plastic properties of the asphalt mixtures, and simulation results were also of great help to improve the durability and drainage performance of the asphalt pavement. Hou et al. (2015) established discrete element specimens for asphalt mastic macadam mixtures, SMA-13, and applied vehicle loads to study its meso-mechanical response under loading. Huang et al. (2008) carried out a series of biaxial compression tests on sand granular materials under different densities and confining pressures, and further analyzed their mechanical properties through the response of sand characteristics from simulation tests. Compared with laboratory test methods, the DEM simulation can provide the details of meso-structural changes in the loading process. The researchers simulated the Compaction Flow Test (CFT) of asphalt mixtures based on the 3D DEM (Roozbahany et al. (2019) and analyzed several factors, including the fluidity of asphalt mixture particles, mold size, loading strip geometry and loading rate, etc., Kusumawardani and Wong (2020) carried out the DEM simulation and laboratory tests on the mechanical properties of Porous Asphalt Mixtures (PAM) with three aggregates shapes, and also analyzed the influence of the aggregate shape on the accumulation of mixtures. Other studies tried to simulate the rutting test of asphalt mixtures with 3D DEM and analyze the influence of internal structural characteristics of the mixtures on the rutting behavior (Zhang et al., 2018). The three-stage simulations (gravity drop, static, and rotary compaction) were performed on three different asphalt mixtures to analyze the internal consistency of the mixtures (Zhou et al., 2020). The randomly generated algorithm was used to establish a model to simulate the aggregate penetration test and the uniaxial creep test. Compared with the experimental tests, it was proved that the established microstructure models were correct and simulation results are feasible (Ma et al., 2016b). The static indirect tensile test of the asphalt mixture was simulated, and the laboratory experiment was carried out to verify the model. The crack propagation mechanism of asphalt mixtures was explained through simulation and test results (Dan et al., 2018). There are many scholars to publish more manuscripts related to the DEM and they are trying to solve many kinds of engineering problems through numerical simulations. According to the currently statistical survey and literature review (main databases), research on the application of the DEM showed an increasing trend, and also the topic of the literature mainly focused on asphalt mixtures. This trend can be seen from Figure 1.
This paper briefly introduced the historical status and development of the DEM, and demonstration of its advantages and disadvantages, as well as differences between the laboratory test method and DEM in performance analysis of asphalt mixtures. The key point was to summarize characteristics of the DEM and learn from others. The DEM applications and shortcomings were also mentioned, as well as prospects of its future development in civil engineering.
2 Historical Overview and Discrete Element Method
2.1 Basic Overview of Discrete Element Method
The numerical simulation is a more advanced analysis method based on computer resources and it is different from the traditional laboratory test method. The numerical simulation mainly included the finite element method and boundary element method before the invention of the DEM (Wang and Li, 2005). As a new numerical simulation method, the DEM was applied to various studies by researchers. Compared with the former two methods, the DEM focused on solutions of discontinuous media based on Newton’s second law of motion (F = ma) (Wang et al., 2019). The DEM software PFC (Particle Flow Code) was more suitable and widely used (Liu et al., 2020). The PFC was divided into two-dimensional (2D) and three-dimensional (3D) PFC programs. The PFC was based on the theories of Newton’s second laws and relationship between force and displacement, when simulating the related model of asphalt materials (You and Liu, 2010).
2.2 Historical Overview
The DEM was originally proposed by Prof. Cundall (1971) as a method for the analysis of granular discrete materials. It was originally used to analyze geotechnical problems. He also realized the purpose of interactive simulations after inputting and outputting geometric data on the computer (Cundall, 1974), and developed a 2D plane “Ball” program to simulate the behavior of granular media (Cundall, 1978). Cundall and Strack (1979) carried out the numerical simulation in 1979 and compared them with the real experimental data. The use of the DEM can carry out more realistic simulations of specimens. However, due to the immaturity of the technology and the limitations of the conditions at that time, the research was carried out based on the 2D plane. The 3D program was not developed because of the complexity and advanced computer technology. After the development of the computer algorithms, the DEM was gradually accepted to solve the problem of discontinuous media. One of DEM companies is called “ITASCA,” and it developed many discrete element programs including the 2D discrete element program—UDEC (Universal Distinct Element Code), and 3D discrete element program—3DEC (3D Distinct Element Code). These programs aimed to solve problems from rock mechanics and mining engineering.
Wang (1986a) introduced the basic principle and application cases of DEMs to the field of rock mechanics and engineering in China at the first National Symposium. The DEM can also be generalized for other research areas (Wang, 1986b), like mining or other engineering fields (Wang and Xing, 1986; Wang et al., 1987; Xing and Wang, 1988; Wang and Xing, 1991; Wang and Xing1993a; Wang and Xing1993b). The DEM progress was summarized from application examples (Wang and Xing, 1989; Wang, 1990). The 2D and 3D DEMs were also promoted for the simulation of civil engineering materials (Wang and Xing, 1990; Xing and Wang, 1990). One of the discrete element programs was called “MatDEM” based on MATLAB and it was developed by Liu et al. (2020) for 3D DEM. This program can build the virtual particles of asphalt mixtures with 2D and 3D models. Another discrete element simulation software “StreamDEM” was developed and it improved the simulation speed and efficiency based on the GPU operation. It met the calculation requirement of the large-scale and large number of particles (million levels), and can be commonly used in the engineering fields (Zhang and Zhang, 2016; Zhang et al., 2017).
3 Research Methods for Asphalt Mixtures
3.1 Analysis of the Laboratory Test Method
The traditional tests were employed to evaluate the properties of the asphalt binders and mixtures with different modifiers at different conditions based on the Marshall and Superpave systems. The recycled materials are the hotspot for the development of asphalt mixtures. Recently, Li et al. (2021) recycled SMA-13 asphalt mixtures for the pavement reconstruction project. Song (2021) utilized waste materials to reduce emissions and increase waste consumption, as well as the polypropylene fibers and glass. Yao et al. (2021) used comparative experiments to analyze performance with other waste materials, such as red mud. It was mixed into asphalt mixtures by the replacement of the mineral powder. Based on the test results, it is a good way to use the waste resources in the pavement engineering. In addition, different modifiers were used for performance improvement of asphalt mixtures. The polyester fibers can also effectively improve performance of asphalt mixtures with the Reclaimed Asphalt Pavement (RAP) (Zhong and Zhang, 2021). The comparative tests were also used to determine the optimum content of these modifiers, like the Freeze-Thaw Test, Four-Point Bending Beam, Dynamic Shear Rheometer (DSR) and Bending Beam Rheometer (BBR) test (Gao, 2021). Meng et al. (2021) studied the effect of the snow melting agent on the adhesion of asphalt mixtures. The use of the snow melting agent (chloride) did not affect the adhesion between asphalt mixtures, but the negative effect on performance of asphalt mixtures may occur by the increase of the agent content. The Styrene-butadiene-styrene (SBS) modified asphalt binders were used for the preparation of the composite modified asphalt binders to enhance the low-temperature performance (Xia et al., 2021). Figures 2A,B show the dynamic modulus test samples and curve of stress and strain changes with time in asphalt mixtures (Jia et al., 2021).
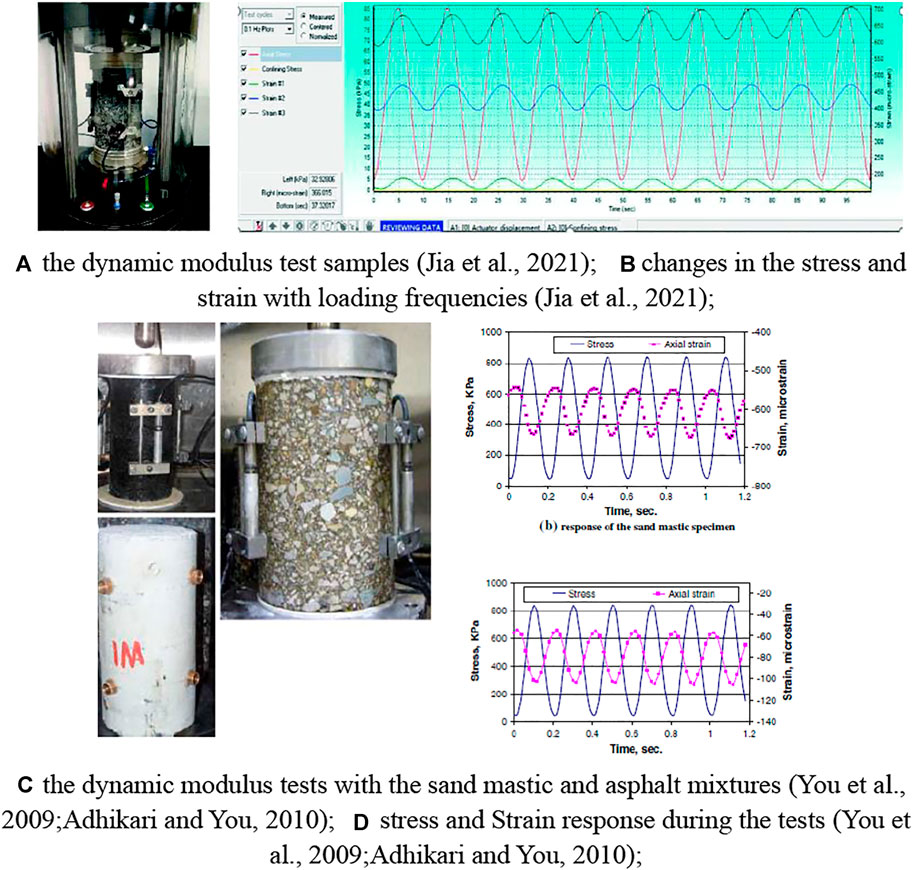
FIGURE 2. Laboratory tests and processing for the dynamic modulus of the asphalt mixtures (Jia et al., 2021; You et al., 2009; Adhikari and You, 2010). (A) the dynamic modulus test samples (Jia et al., 2021); (B) changes in the stress and strain with loading frequencies (Jia et al., 2021); (C) the dynamic modulus tests with the sand mastic and asphalt mixtures (You et al., 2009; Adhikari and You, 2010); (D) stress and Strain response during the tests(You et al., 2009; Adhikari and You, 2010).
The dynamic modulus test is conducted under the haversine or sinusoid loading conditions. The output parameters (the dynamic modulus and phase angle) are measured at different temperatures and frequencies. Before testing, the samples are mixed by asphalt binders and aggregates with the certain gradation. The mixtures are compacted by the Superpave Gyratory Compactor (SGC) with a targeted air void. The samples are compacted and cut with the specified dimension (100 mm in diameter and 150 mm in height); The detailed procedure can be found from the specification AASHTO T378. The indirect tensile test is measured by the Universal Testing Machine (UTM), and this test is destructive and used to evaluate the moisture susceptibility. This test results can also be used to predict the performance of anti-stripping and fracture toughness. The range of the air void in the asphalt mixture is around 6%–8%. The dimension of the sample is 100 mm in height and 63.5 mm in diameter. One group of the samples is cured with different conditions and another group is stored in the dry environment. The procedure of this test can be found from the specification AASHTO T283. These are the details for the dynamic modulus and indirect tensile test.
3.2 Discrete Element Analysis
3.2.1 Two-Dimensional Discrete Element Method
In many early studies of the discrete element modeling method, the 2D model was commonly used and the advantage of the 2D model is high calculation speed for the complex system. Sometimes, although simulation results of some relatively complex experiments are not consistent with the actual measured data, the 2D model program is still advantageous for the experiments with the simple internal structure of the mixture and the analysis of the general trend without requiring accurate results. The asphalt mixtures with a 2D discrete element program as an example (Nian et al., 2021; Peng and Sun 2015a) are shown in Figure 3.
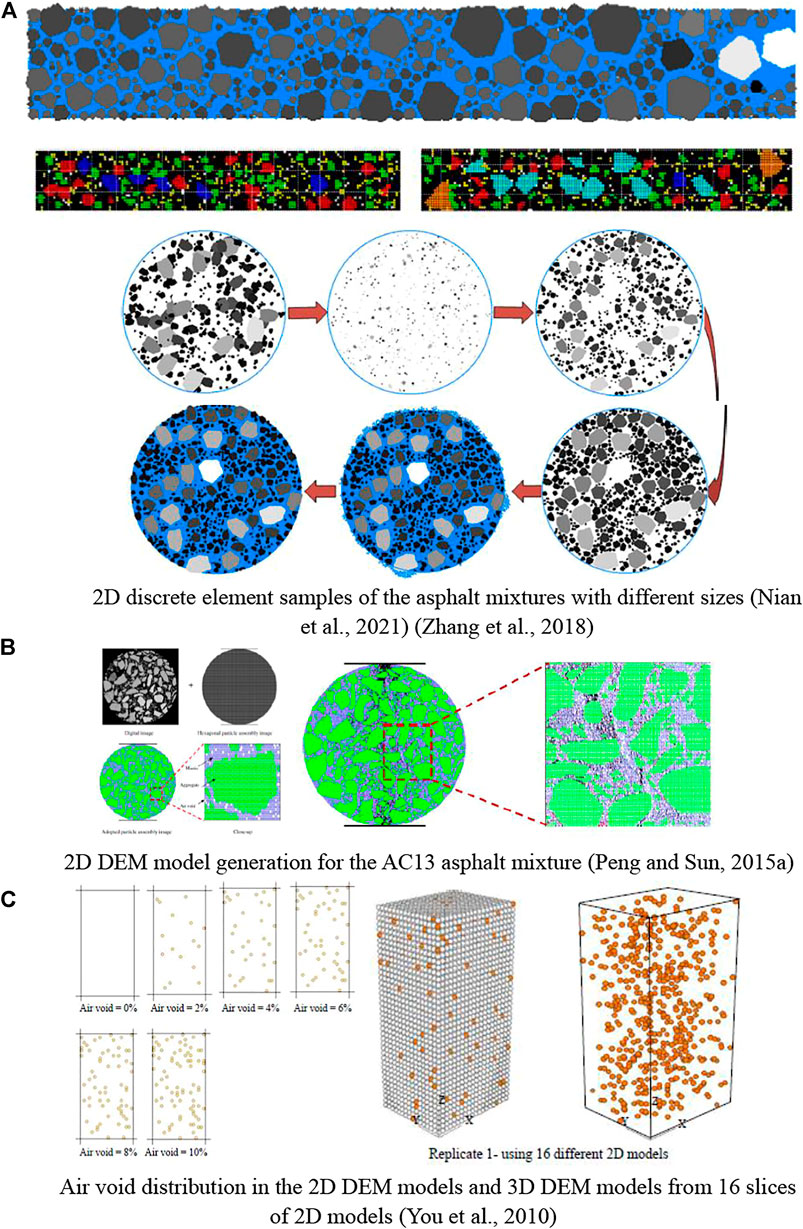
FIGURE 3. 2D DEM samples with different gradation and air void distributions (Nian et al., 2021; Zhang et al., 2018; Peng and Sun, 2015a; You et al., 2010). (A) 2Ddiscrete element samplesof the asphalt mixtureswith different sizes (Nian et al., 2021; Zhang et al., 2018). (B) 2D DEM model generation for the AC13 asphalt mixture (Peng and Sun, 2015a). (C) Air void distribution in the 2D DEM models and 3D DEM models from 16 slices of 2D models (You et al., 2010).
Liu et al. (2009) established a 2D discrete element model to focus on the viscoelastic properties of asphalt mixtures with the burger model. Through the DEM simulation of the asphalt mixtures, the dynamic modulus and phase angle of the asphalt mixtures were predicted under the sinusoidal loading, and the viscoelastic models were verified by the measured results. There is only a little research on the influence of aggregate uniformity on the splitting strength test of asphalt mixtures. The simulation results showed that whether the aggregates are uniform or not has little effect on the average splitting strength. However, it was closely related to the variability of splitting strength, which showed that the coefficient of variation of the splitting strength decreases with a better aggregate uniformity (Peng Y. and Sun L., 2015a). The variation relationship of the splitting strength under different loading rates was that the higher the loading rate was, the higher the splitting strength was with a logarithmic growth (Pei et al., 2010). The cracking and failure properties of asphalt mixtures with three different gradations were analyzed and compared under the splitting test simulations using the 2D DEM. The simulation results demonstrated that the large nominal maximum particle size and huge porosities of aggregates with low asphalt contents caused low splitting strength in asphalt mixtures. The crack resistance of the OLSM-25 (Open-graded Large Stone Asphalt Mixes) asphalt mixture was better than that of AC-16 and AC-25 (Asphalt Concrete) (Guo and Chen, 2014; Guo and Chen, 2015). The splitting test process of four different aggregates was simulated by the 2D DEM and the influence of aggregate properties on splitting strength was also analyzed with laboratory tests. It was concluded that the high stiffness and strength of aggregates lead to a strong bearing capacity of the asphalt mixture models (Xiong, 2013). The indirect tensile test of the asphalt mixture was simulated by 2D DEM to analyze the influence of the bond strength ratio and loading rate on the splitting strength of the asphalt mixture. The results showed that the splitting strength decreased with the increase of the bond strength; the splitting strength increases with the increase of loading rate, and it is proved that the performance of asphalt mixtures plays the most important role in enhancing the crack resistance of asphalt mixture (Chang et al., 2011). Four kinds of aggregates with different properties and gradations were selected (Yu, 2015) for laboratory tests and simulations of the splitting test with the 2D DEM. The feasibility of discrete element simulation was studied and the influence of aggregates with different properties on the cracking of asphalt mixtures was analyzed. The results demonstrated that the DEM simulation may provide more intuitive and effective details compared to the laboratory test processing, like loading changes, displacements, and cracking propagations during testing. However, this study also revealed the limitations of the 2D DEM, such as the lack of geometric information. Figure 4 shows the loading-displacement relationship of the AC-16 gradation section at different temperatures when simulating the splitting test of the asphalt mixture by a 2D DEM (Peng and Sun 2015b). In addition, the 2D DEM can be also used in many other studies, like other Marshall system tests of asphalt mixtures.
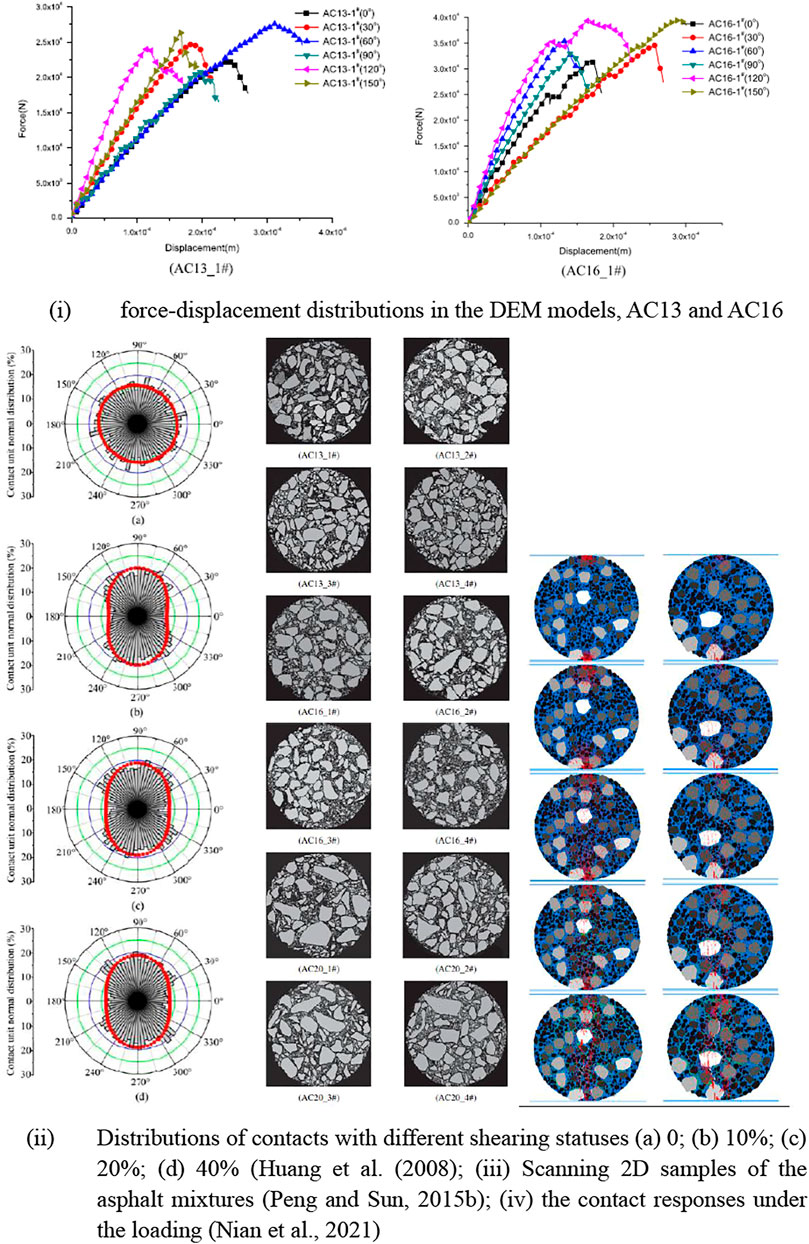
FIGURE 4. Load-displacement curves of the splitting test (indirect tensile test) and contact distributions (Peng and Sun, 2015b; Huang et al., 2008; Nian et al., 2021). (i) force-displacement distributions in the DEM models, AC13 and AC16. (ii) Distributions of contacts with different shearing statuses (A) 0; (B) 10%; (C) 20%; (D) 40% (Huang et al., 2008); (iii)Scanning 2D samples of the asphalt mixtures(Peng and Sun, 2015b); (iv) the contact responses under the loading (Nian et al., 2021).
3.2.2 Three-Dimensional Discrete Element Method
From the morphology perspective, the effect of the 3D model is close to the real experiment compared to the 2D one and the 3D model has more complex structures and interactions. During the generation and optimization of 3D models, the calculation time is longer than that of the 2D model and the time depends on the element number in the simulation system. Sometimes, the computing time can be greatly reduced using the temperature-frequency superposition method, but the computation time is still relatively long compared to the 2D model. The temperature-frequency superposition method specifically is to amplify or adjust the normal loading frequency from the available test results and convert Burger’s model parameters under the normal frequency into parameters under the virtual frequency. The model can be established with the magnified virtual frequency and is matched by the modified Burger’s model parameters. The comparative test results of the dynamic modulus of asphalt mixtures at different temperatures and frequencies showed that the simulation results were close to measured results under the temperature-frequency superposition method. However, the calculation time was greatly reduced. When the computation time took months or even years in the traditional method, it can be reduced to only hours, and the calculation speed increased rapidly (Liu and You, 2011a). It has been used most by scholars in recent years.
Due to the limitation of the computer technology in the early stage, the research with the 3D DEM is far less than the 2D one. Dr. You’s research team members conducted much research on the 3D DEM, like a 3D DEM model to predict the dynamic modulus and phase angle of asphalt mixtures, and the relative errors between the laboratory and simulation results were within an acceptable range (You and Liu, 2010). Another 3D DEM model was established to simulate the creep test of the asphalt mixture to study the creep stiffness and response (You et al., 2011), and also, it was used to analyze whether the element size affected the performance of the asphalt mixtures (especially in mechanics) (Liu et al., 2012). The dynamic modulus of the asphalt mixture was predicted and the influence of the aggregate size was analyzed by comparing the predicted results with the measured data (Adhikari and You, 2011). The fitting curves of the dynamic modulus and phase angle of asphalt mixtures are shown in Figure 5, and the relationship between the 2D and 3D DEM simulation values and laboratory-measured values was demonstrated (Liu and You, 2011b). Both 2D and 3D DEM coincide with the test results. Zhang et al. (2015) modeled rubber particles and aggregates, respectively, to simulate the splitting test of the asphalt mixture by the 3D DEM. The simulation results were in good agreement with the test results. It can be seen that it was successful to use the DEM to simulate the test processing from the initial loading and cracking to the failure of the asphalt mixture. In addition, the contact model in the DEM is composed of three built-in parts: “stiffness model, slip model and bonding model.” These models constitute other complicate models. The slip model in the DEM means the frictional properties between contact points. The bonding model represents shear and normal strength between contact points. The stiffness model addresses the relationship between the contact forces and displacements of elements. The viscoelastic models or properties of asphalt mixtures can be expressed by the Burger’s model and this model can be constituted from three parts (stiffness model for asphalt mastics and aggregates or within asphalt mastics; slip model for all contacts; bonding model for all contacts except between aggregates). Thereby, different viscoelastic models can be further formed from three contact parts in the DEM (Liu et al., 2012).
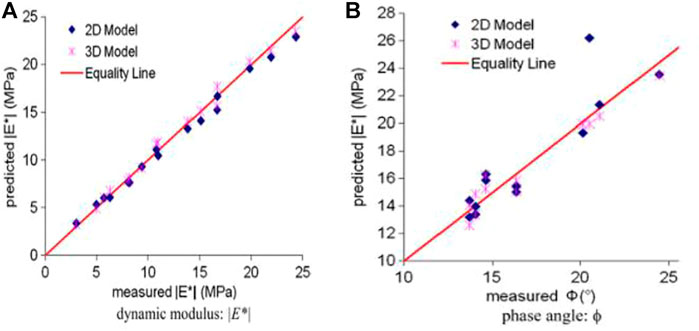
FIGURE 5. Fitting curves between the simulation data and measured values in the laboratory with the 2D and 3D DEMs, (A) the dynamic modulus; (B) the phase angle (Liu and You, 2011b).
The Digital Image Processing Technology and Computerized Tomography (CT) Scanning Technology were also employed and assisted for the DEM simulation to explore the interaction mechanisms in the asphalt mixtures. Different factors were analyzed through the splitting test of the asphalt mixture with the 3D DEM by Wan (2016), including the aggregate gradation, asphalt contents, temperatures, and loading rates. The research results were consistent with the previous analysis results. A possible reason for the error between simulation and measured results is the selection of parameters in the DEM models. It can be concluded and referred that 3D DEM simulation is more stable and reliable than the 2D one through the comparison between microstructures of the 2D and 3D DEM models (Peng et al., 2016). The accuracy of the simulation model was also improved from the 2D model to the 3D model (Peng and Bao, 2018). The influence of the aggregate gradation and temperature on the splitting strength of asphalt mixtures was also analyzed with the 3D DEM by Peng et al. (2019). The DEM simulation data trend of the asphalt mixtures was consistent with the laboratory test results. However, minor errors can be found in the simulation data. Further studies were needed for the improvement method and fundamental interactions. Figure 6 shows the 3D asphalt mixture DEM model (Shan et al., 2019; Liu and You, 2011b; Ma et al., 2016b; You et al., 2008; Zhou et al., 2020; Liu et al., 2012).
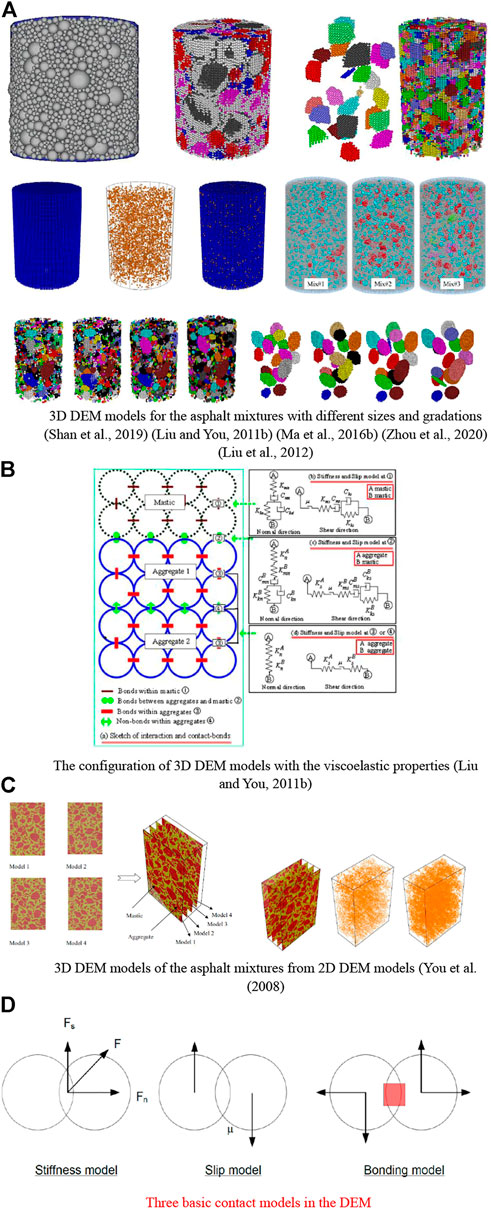
FIGURE 6. Three-dimensional discrete element model and DEM viscoelastic configurations of the asphalt mixtures (Shan et al., 2019; Liu and You, 2011b; Ma et al., 2016b; Zhou et al., 2020; You et al., 2008; Liu et al., 2012). (A) 3D DEM models for the asphalt mixtures with different sizes and gradations (Shan et al., 2019; Liu and You, 2011b; Ma et al., 2016b; Zhou et al., 2020; You et al., 2008; Liu et al., 2012). (B) The configuration of 3D DEM models with the viscoelastic properties (Liu and You, 2011b). (C) 3D DEM models of the asphalt mixtures from 2D DEM models(You et al., 2008). (D) Three basic contact models in the DEM.
The aggregate uniformity of asphalt mixtures was also investigated with the 3D DEM through the low-temperature splitting strength, and the Cohesive Zone Model (CZM) was also used in the DEM model to explore the relationship between the aggregate uniformity and splitting strength of asphalt mixtures. In the study of Liu et al. (2018), the same conclusion was obtained as the previous study (Yu, 2015) did. In recent years, the 3D DEM and the cohesive zone model were also used by many scholars to study the splitting strength of asphalt mixtures. The asphalt and aggregate models were reconstructed by Liang et al. (2019) to simulate the splitting test with the 3D DEM and MATLAB, and the cohesive model in this model was applied. The cohesive model was also combined with the finite element analysis in six kinds of asphalt mixtures. The results showed that the splitting strength trends of the asphalt mixtures were the same as the experimental results roughly. The DEM simulation was feasible for the asphalt mixtures and provided a reference for the fundamental research. The applicability of the contact bond and parallel bond models was explored by Shan et al. (2019) and Sheng et al. (2018) through the 2D model and 3D DEM models with different parameters. The results showed that it was reasonable to adapt the parallel bond model when simulating the mechanical properties of asphalt mixtures with the load-displacement behavior. The splitting test is commonly used for the evaluation of water susceptibility and permanent deformation resistance of asphalt mixtures. The dynamic modulus test of the asphalt mixture is also used for the evaluation of the high- and low-temperature performance. The 3D DEM is a promising way to understand the microscopic behavior of the asphalt mixtures, as well as the splitting test and dynamic modulus test of asphalt mixtures. More fundamental understanding between the materials may be revealed and induced from the DEM simulations in the near future.
4 Discrete Element Modeling Method and Simulation Process
4.1 Modeling Method
Generally speaking, there are three modeling methods to construct the microstructure of asphalt mixtures with DEM, including the idealized model, randomly generated irregular shape model and image-based model. The idealized model cannot represent the geometric properties of aggregates and mixtures, and does not have authenticity; the image-based model can truly simulate the characteristic structures of asphalt mixtures with the help of computer scanning technology. However, it is dependent on the laboratory conditions. The scanning equipment is expensive and the cost is relatively high. The testing process is long and complex; the randomly generated irregular shape model is independent of the laboratory, which is more convenient and faster than the former two modeling methods and consumes less manpower and material resources. The above has been emphatically mentioned in many kinds of literature. The idealized model is idealized, which is not consistent with the real experimental results, the latter two models are mostly used in general research. Therefore, only the latter two modeling methods are introduced in this article.
4.1.1 Based on Scanning and Image Processing Technology
A 3D viscoelastic model of the asphalt mixture was established by You et al. (2008) based on X-ray image scanning technology to simulate and restore the creep flexibility test. A time-temperature superposition principle was developed to improve the calculation time. The microstructure reconstruction of asphalt mixtures with the 3D DEM was completed by You and Liu (2010) based on images from the X-ray computed tomography. The frequency-temperature superposition principle was used to shorten the simulation time. The advantage of this image processing technology is that it can truly show most of the structural characteristics of asphalt mixtures. The dynamic modulus and phase angle of the asphalt mixtures were predicted and compared with the laboratory test results. The results showed that most of the predicted values were close to the measured values. The 3D discrete element model of the asphalt mixture was reconstructed through the scanned 2D model of the aggregates and the related research was carried out to analyze the influence factors of the modulus (You et al., 2011). Also, the 3D structured light scanning technology was used to scan the aggregate particles and the aggregate information was compiled with the help of the MATLAB program. The 3D model of asphalt mixtures was established based on the aggregated information in PFC5.0 to study the multiscale influence factors of volume characteristics (Li et al., 2020). X-ray CT technology was used to scan aggregates for the microstructure of aggregates. The MATLAB program and PFC3D were employed to establish a 3D model of the asphalt mixture to simulate the uniaxial penetration test to explore how aggregate affected the shear performance (Wu et al., 2021). The scanned internal images of aggregates were processed by Guo (2020) and Peng et al. (2021)with image information acquisition software with the help of CT scanning. The aggregate characteristics were imported into PFC3D to generate a 3D discrete element model of the asphalt mixture to study the effect of the gap distribution on the shear fatigue life. the relevant information of aggregate particles was obtained by Shi (2020) from the laser scanning and established a PFC3D model of the asphalt mixture. The model was verified with the help of the laboratory experimental data and it was used to analyze the aggregate characteristics. The particle images of aggregates were intercepted by Lu (2020) with CT scanning technology and processed them with the MATLAB program to establish a 3D model of the asphalt mixture in PFC3D. The virtual splitting test (indirect tensile test) and uniaxial penetration test of the asphalt mixtures were carried out and verified with real experimental data for further research. The 2D mixture image and the scanning process of 3D specimens obtained with the help of scanning technology are shown in Figure 7 (Liu, 2011) You et al., 2009; Adhikari and You, 2010) and Figure 8 (Ge et al., 2021) (Adhikari and You, 2010).
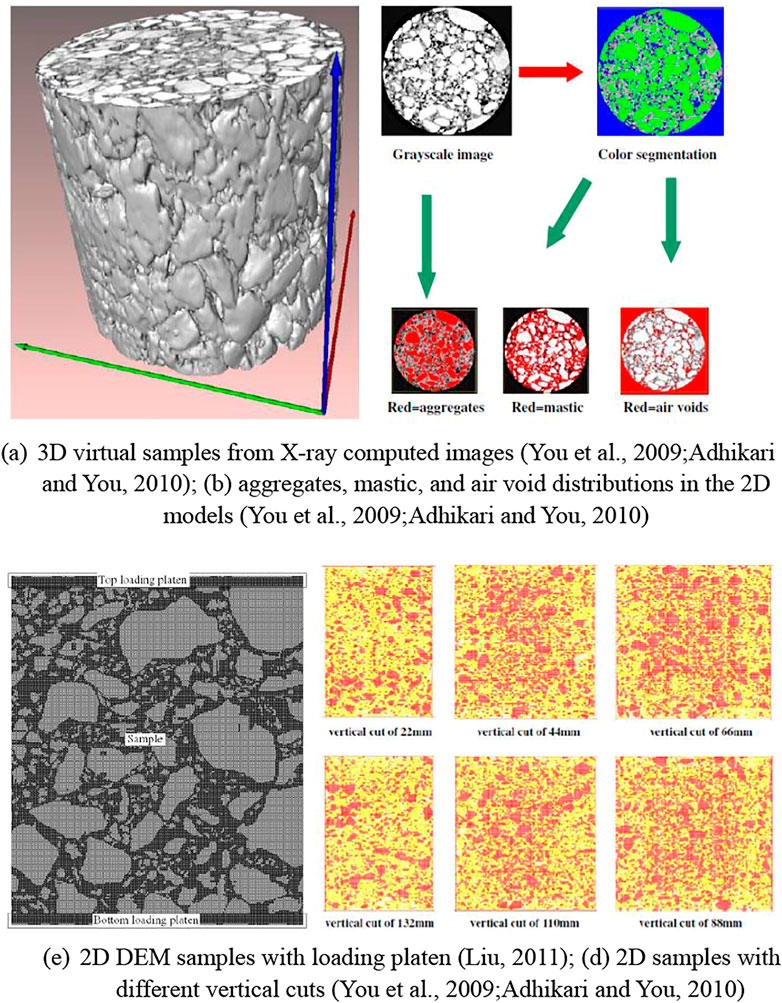
FIGURE 7. Virtual samples of the asphalt mixtures (Liu, 2011; You et al., 2009; Adhikari and You, 2010). (A) 3D virtual samples from X-ray computedimages (You et al., 2009; Adhikari and You, 2010); (B) aggregates, mastic,and air void distributions in the 2D models (You et al., 2009; Adhikari and You, 2010). (C) 2D DEM samples with loading platen(Liu, 2011); (D) 2D samples with different vertical cuts(You et al., 2009; Adhikari and You, 2010).
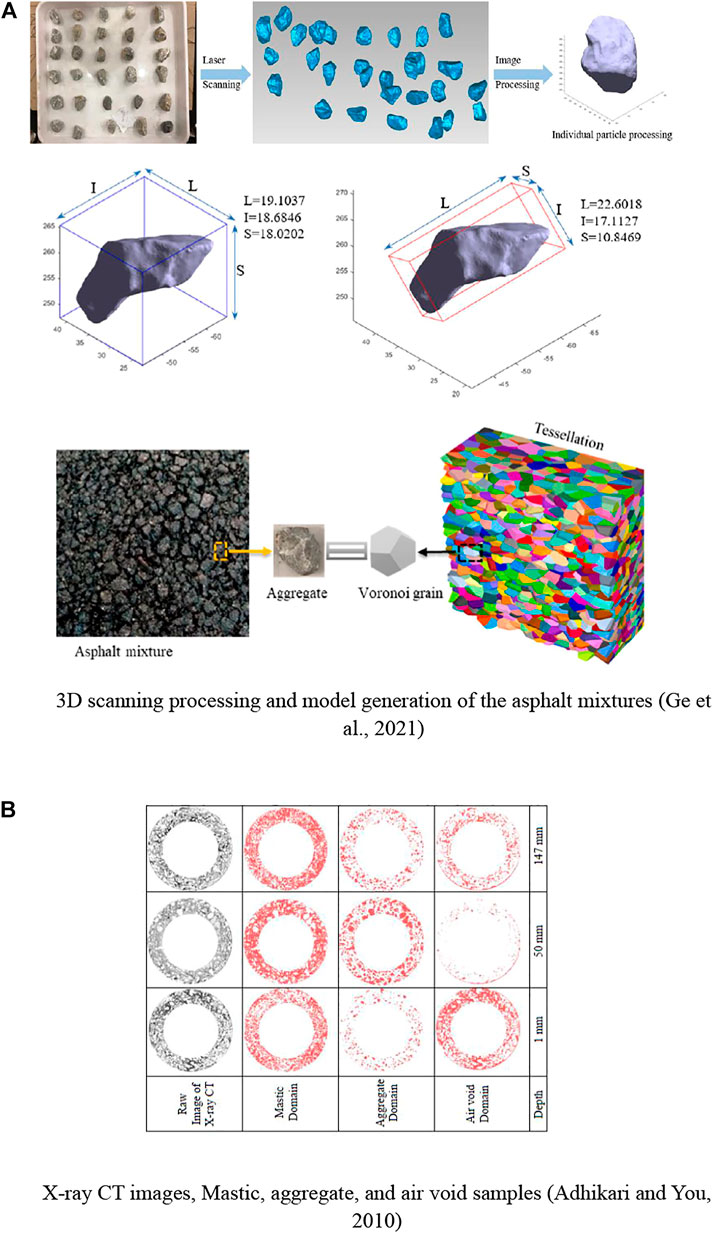
FIGURE 8. 3D DEM scanning samples and digital generation specimens (Ge et al., 2021; Adhikari and You, 2010). (A) 3D scanning processingand model generation of the asphalt mixtures (Ge et al., 2021). (B) X-ray CT images, Mastic, aggregate, andair void samples (Adhikari and You, 2010).
4.1.2 User-Defined Method
The randomly generated irregular shape model refers to the aggregates of the asphalt mixtures represented by irregular polygonal particles in the discrete element model established by a random polygon algorithm (Liu and You, 2008). A self-defined discrete element model was established by Chen et al. (2012) and the cyclic loading uniaxial compression tests of asphalt mixtures were carried out. The model was verified to predict the dynamic modulus and phase angle of asphalt mixtures and analyze factors for the viscoelastic characteristics of asphalt mixtures. four kinds of typical aggregate gradations were selected by Zhou (2016) to carry out the dynamic modulus test and generate the numerical model to analyze the DEM feasibility for the virtually mechanical test of asphalt mortars and mixtures. The classically viscoelastic model (Burger’s self-defined constitutive contact model) was adopted in the discrete element model. The digital cylinder specimen was composed of multiple discrete elements with different sizes and the discrete element model was used for the dynamic modulus test. The randomly generated PFC3D models of asphalt mixtures were used to simulate the creep experiments to study the influence of void-related factors at different temperatures (Ma et al., 2016a). A 3D user-defined model was established by Du et al. (2021) to simulate the indirect tensile test to analyze the gradation effect on the crack propagation at low temperatures, as well as the virtual performance test of different gradation asphalt mixtures. Different mixture gradations were adapted for the performance analysis and improvement measurements (Ling et al., 2020). the user-defined method was used by Wang et al. (2021) to establish PFC2D and PFC3D models of asphalt mixtures and simulated the dynamic creep and rutting tests. Other tests were modeled with the PFC2D program to study the factors of asphalt mixtures for the low-temperature performance, such as the Semicircular Bending Test (SCB) (Figure 9), Splitting Test (indirect tensile test), and Three-point Bending Test (Figure 10) (Liang et al., 2021; Nian et al., 2021).
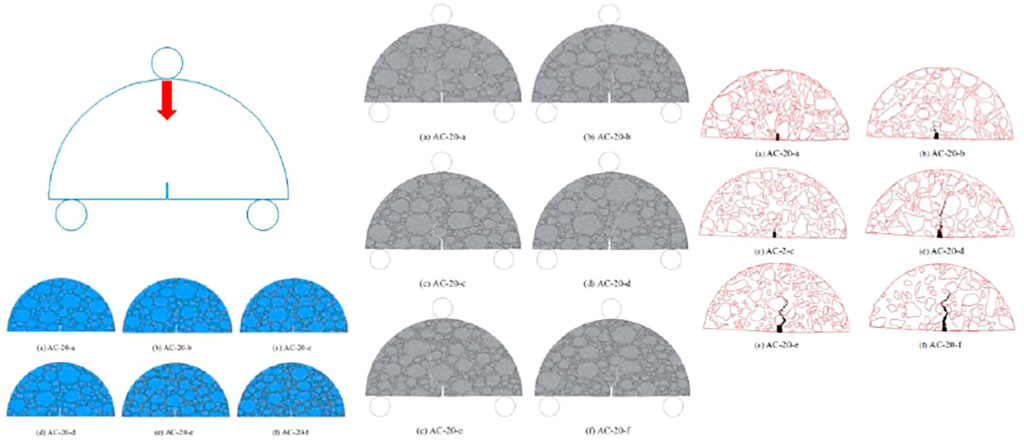
FIGURE 9. SCB digital samples, loading setting and deformations of the asphalt mixtures (Liang et al., 2021; Nian et al., 2021).
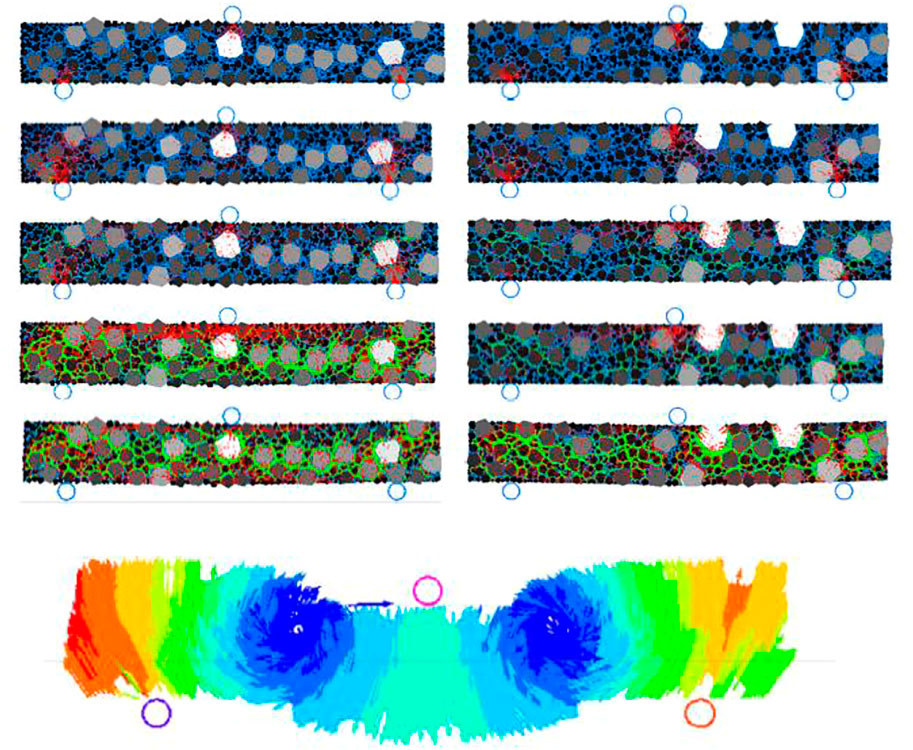
FIGURE 10. Splitting test (indirect tensile test) and three-point bending test samples of the asphalt mixtures with the DEM models (Nian et al., 2021).
4.2 Simulation Process
4.2.1 Modeling
The basic steps of the discrete metamodels (Randomly Generated Models) for asphalt mixtures in PFC3D were roughly divided into the following steps (Liu, 2011) (You et al., 2009; Adhikari and You, 2010). 1) the digital size and aggregate gradations were defined, and the asphalt content and air voids were set based on the volume characteristics of asphalt mixtures; 2) balls with the same size were created and a polyhedron was randomly created; 3) A two-phase system of the aggregate and mortar was generated; 4) the volume characteristics of the two-phase system were verified. The air voids were randomly generated by deleting the balls in the two-phase system. Finally, a digital PFC sample of the asphalt mixture was generated with the air void by the random method. The Aggregate Imaging Measurement System (AIMS) was used to measure the aggregate particles to get the image characteristics. Three methods were adopted to form the virtual aggregate samples, including the Radius Expansion (RE), Interior Filling (IF), and Contour Filling (CF) Methods (Ding et al., 2019). The relationship between the contact points was also demonstrated and defined in the literature (Ding et al., 2019) (Cundall and Strack (1979). Three parts (asphalt mastic, aggregates and air voids) was combined to generate the asphalt mixture samples in PFC3D with the user-defined procedure. The asphalt mixture samples were made for the wheel tracking test (Ma et al., 2018). The complex processes and interaction behaviors of these methods are shown in Figure 11 (Liu, 2011; You et al., 2009; Adhikari and You, 2010; Cundall and Strack,1979; Ding et al., 2019; Ma et al., 2018).
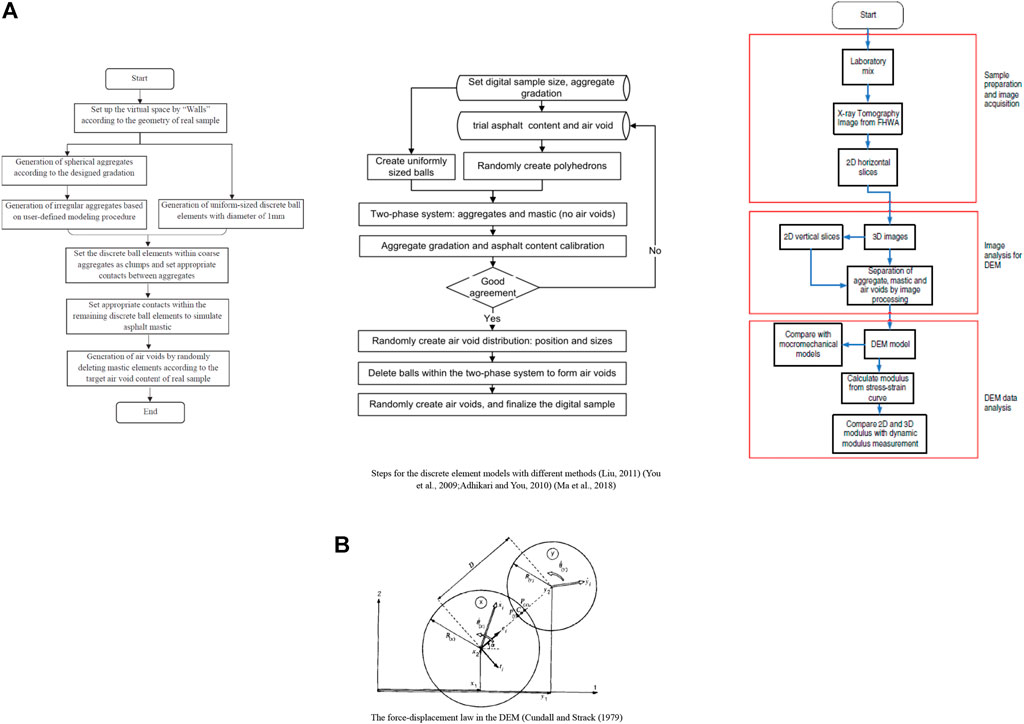
FIGURE 11. Dem modelling steps and interaction behaviors between balls (Liu, 2011; You et al., 2009; Adhikari and You, 2010; Cundall and Strack,1979; Ding et al., 2019; Ma et al., 2018). (A) Steps for the discrete element models with different methods(Liu, 2011; You et al., 2009; Adhikari and You, 2010; Ma et al., 2018). (B) The force-displacement law in the DEM(Cundall and Strack,1979).
4.2.2 Loading Model
The shapes and sizes of the asphalt mixture models were relatively different from the test samples. The dynamic modulus test models of asphalt mixtures were cylindrical; the splitting strength test models of asphalt mixtures were cylindrical with the same size as the actual Marshall specimen, and the four-point bending beam test model is rectangularly trabecular. It is necessary to apply the load in the model with “force” after the generation of the mixture model. The specific operation was summarized as follows: setting several different gradations; writing the servo control program in the Fish language; and achieving the function of applying load to the top and bottom of the model specimen. It is worth noting that the force on the “wall” cannot be directly controlled in the program. Instead, it is necessary to control the speed of the “wall” through commands. The “wall” at the bottom of the specimen is not moving and a downward speed is applied to the “wall” at the top of the specimen to realize the loading of the model, and the control of the speed of the “wall” is realized using the servo control program to apply the periodic load to the model specimen. The PFC2D and PFC3D models of the asphalt mixtures are generated as shown in Figure 12 (Shan et al., 2019; Peng and Bao, 2018; Ma et al., 2016b).
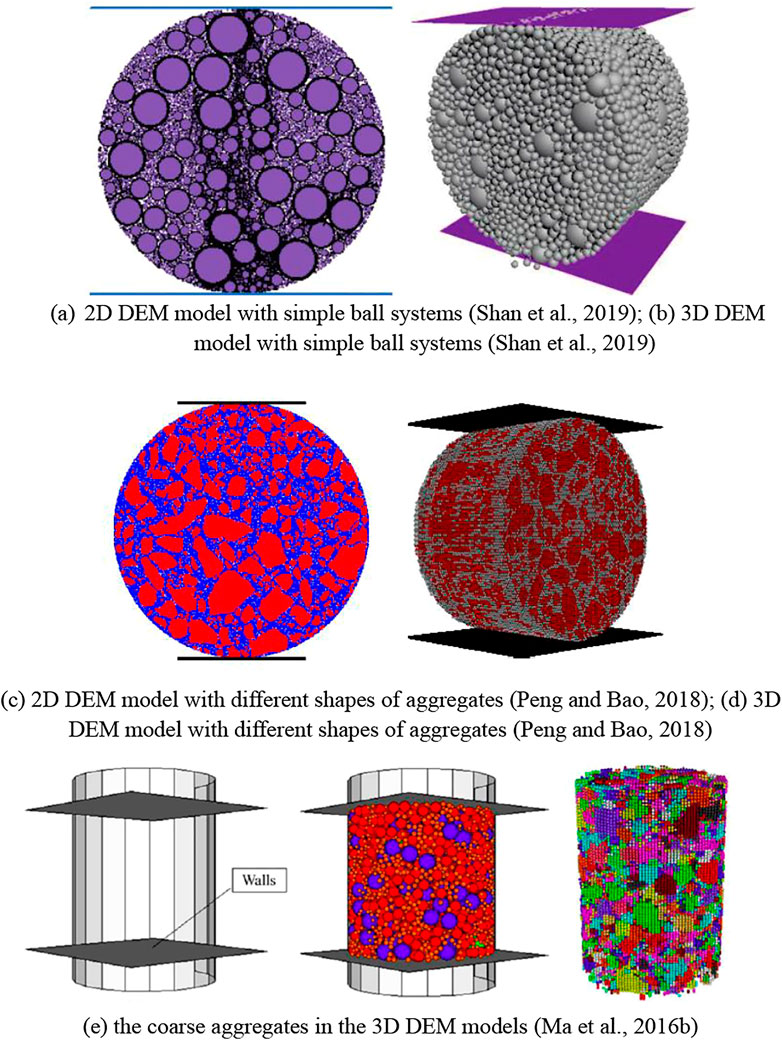
FIGURE 12. 2D and 3D DEM simulations for the splitting test (indirect tensile test) of the asphalt mixtures (Shan et al., 2019; Peng and Bao, 2018; Ma et al., 2016b). (A) 2D DEM model with simple ball systems (Shan et al., 2019); (B) 3D DEM model with simple ball systems (Shan et al., 2019). (C) 2D DEM model with different shapes of aggregates(Peng and Bao, 2018); (D) 3D DEM model with different shapes of aggregates(Peng and Bao, 2018). (E) the coarse aggregates in the 3D DEM models (Ma et al., 2016b).
5 Discussions
(1) The DEM was designed for the analysis of rock problems and it was extended to solve the pavement problems later, as well as to explore the mechanical behaviors of asphalt mixtures or cement. The difference between the continuum-based method and DEM is the system topology and construction manner, as well as the solution strategies and material behaviors. During the development of discrete elements, the DEM algorithms were invented and developed for different scenarios of materials at the micro scales, such as the boundary types, contact-point constitutions, force transfers, and calculation, sample generation, particle shape and types, fluid flow mechanics, geomaterial micromechanics, etc. Many algorithm blocks and methods were investigated to fully satisfy different research proposes and objectives, such as the discrete fracture network, the Navier-Stokes equation, deformation analysis, stiffness matrix, heat conduction, stress wave propagation, various moduli, tensile test, etc. There are many advantages and disadvantages for the laboratory test method and the discrete element numerical simulation method to evaluate the performance and analyze the interaction between elements. The virtual laboratory test plan is the main reason for us to use the simulation to conduct the test; Another reason is that the simulation can solve the problems and it is hard to deal with or figure out them through the laboratory test. Most importantly, the simulations can avoid most laboratory risks during real testing in the laboratory, and it is safe that the hazardous materials are also processed with the simulations. Thereby, more scholars tried to use different simulations to solve the problems with different scales. In addition, the required simulation time is shorter than that used in the laboratory. Some tests in the laboratory may take as long as several years, like the creep test for concretes. For instance, during the dynamic modulus simulations of asphalt mixtures, the calculation time in the DEM simulation can be shortened to several days or even hours using the temperature-frequency superposition method (Liu and You, 2011a). It can be found that most DEM simulations are verified by real experimental data from different studies. The computational and numerical simulations may be the promising way to understand the behavior of materials based on the fast development of computer technologies. Some advantages are highlighted compared to laboratory testing, like the fast calculation, low cost, less labor, less risk, no damage to human health, and so on. Most importantly, the numerical simulation can explore the interactions between materials, which cannot easily realize by the experiments, such as the interaction at the nano- and micro-scale between materials compositions. In addition, experimental data is still needed in the DEM simulations of asphalt mixtures to ensure the relative accuracy of the results.
(2) Generally speaking, there are two ways to simulate different tests or models of asphalt mixtures with discrete element programs, PFC2D for 2D mixture models and PFC3D for 3D mixture models. It also means that two aspects and perspectives are available to explore the digital materials. The PFC3D program is developed and extended from the PFC2D. It is difficult to reflect the simulation authenticity with the PFC2D under different conditions and research proposals. Thereby, the PFC3D programs are produced and introduced with more accurate simulation results, and the programs restore the actual actions between materials. The 2D and 3D discrete element models of asphalt mixtures are established, respectively, and the two-type models are compared from these aspects, such as the calculation speed and simulation effect compared with the laboratory-measured data. Some example models can be described as follows: Prediction for the dynamic modulus of the asphalt mixture with DEMs (You et al., 2009; Adhikari and You, 2010); Influence of voids and its distribution laws in asphalt mixtures with DEMs (You et al., 2010). After comparison, the effect of 3D model simulation is better than that of the 2D model in most cases. It is relatively close to the laboratory results.
(3) The generation methods or algorithms of DEM samples were discussed here, like the image-based, user-defined, idealized and random models. Three methods had their advantages and the application scenarios depend on the research purposes. Integrating the current simulation databases and computer resources, the image-based method seemed to be more likely to be real samples. However, the image-based model was restricted by the image capturing technology and image numbers or databases. More images of samples needed to be captured and processed under different conditions and these are not transferable. Compared to the idealized models, the other two methods (image-based and user-defined models) are commonly used in 2D or 3D discrete element programs. Through the study of the literature (Yang et al., 2016), the image-based DEM models are inseparable from the scanning equipment and image processing technology, and these are needed to be completed in the laboratory with the high cost and long time-consuming processing. The advantage of this method is that the simulation effect is close to the real status for materials, which can accurately present the shape, position, and distribution of aggregates, and also reflect the real structures of the asphalt mixtures. In contrast, the user-defined model method is used to build the model according to the basic data of the simulation test in the discrete element program with the help of the “Fish” language in the program. It is not expensive and also not time-consuming, as well as the independence of the laboratory restriction. However, sometimes, the accuracy of the simulation data is less than that of the image-based model method. It is difficult to fully show the internal structures of the aggregates in the asphalt mixtures.
6 Conclusion
After the above discussions, we can understand that:
(1) The application of DEMs in pavement engineering, especially in asphalt mixtures, was also studied from the function realization to the mechanism discussion. Many contact/connectivity patterns and behaviors were generated and developed for the interaction discovery of asphalt and aggregates. Many tests of asphalt mixtures were simulated to explain and investigate the performance by the DEM, like the splitting test (indirect tensile test), dynamic modulus test, three-point, and four-point beam test, compressive test, etc., Those were demonstrated partially in this manuscript. There are still more questions and mechanisms needed to be discovered and explored in the DEM simulation, for instance, 1) interaction between the water and mixtures under different conditions, 2) damage patterns of different shapes and forces under different conditions in the pavement, 3) contribution rates of different aggregates and asphalt in asphalt mixtures under different conditions, 4) void distributions in asphalt mixtures and formation laws, 5) water propagations in asphalt mixtures, etc., Sometimes, these questions may be divided into different pieces and can be solved from different views and sights. In addition, it is common that the DEM simulation explores from 2D to 3D for the reality demonstration and links the microscale to macro scale. Also, there are some different advantages in the 2D and 3D simulations and researchers need to integrate your aims and thoughts to make decisions.
(2) The user-defined and random models also have more potential to be developed for virtual laboratory tests, 1) The difficulty of the methods is the useful and universal databases for the virtual sample generation; 2) The shape and texture properties of aggregates can be precisely described and added in the virtual samples, as well as other properties in the models; 3) The interaction between materials, like asphalt and aggregates in asphalt mixtures, can be accurately expressed in the models; 4) The physical and chemical reactions can be strictly presented in the models; 5) The DEM calculation speed and element numbers also affect the simulation effect and generalization. 6) the contact model between the elements may be updated for the constitutive models in DEM model, and this update may provide an option for the solution of the plastic deformation problems in the DEM simulation. It helps to understand behaviors of visco-elastic plastic materials. These points are needed to be considered for us to generate good models, as well as other possible factors.
Therefore, the DEM is a promising tool for us to explore the micro behaviors of asphalt mixtures. It is also affordable for researchers to conduct the tests in the virtual laboratory. It can achieve different test simulations at different dimensions and explore mechanisms under extreme conditions. These operations can not be easily realized in the laboratory or fields. The researchers benefit from these advantages and program the simulation with their purposes. The research results can directly guide the industry application and production, and also, accelerate the research development for the engineering field.
Author Contributions
HY and MX wrote the manuscript; JL and YL helped revise the manuscript; JJ and ZY provided the technical support for the manuscript.
Funding
The authors appreciate the financial support of the Hunan Department of Transportation (No. 202152) and Hunan Expressway Group Co. Ltd. The authors also appreciate the funding support from the National Natural Science Foundation of China (No. 51778038), Beijing Key Laboratory of Traffic Engineering (No. 2018BJUT-JTJD007), and Beijing Municipal Commission of Transportation (Grant Number: 2018-kjc-01-213). Any opinion, finding, and conclusion expressed in this paper are those of the authors and do not necessarily represent the view of any organization. The funder was not involved in the study design, collection, analysis, interpretation of data, the writing of this article or the decision to submit it for publication. All authors declare no other competing interests.
Conflict of Interest
The authors declare that the research was conducted in the absence of any commercial or financial relationships that could be construed as a potential conflict of interest.
Publisher’s Note
All claims expressed in this article are solely those of the authors and do not necessarily represent those of their affiliated organizations, or those of the publisher, the editors and the reviewers. Any product that may be evaluated in this article, or claim that may be made by its manufacturer, is not guaranteed or endorsed by the publisher.
References
Adhikari, S., and You, Z. (2010). 3D Discrete Element Models of the Hollow Cylindrical Asphalt concrete Specimens Subject to the Internal Pressure. Int. J. Pavement Eng. 11. doi:10.1080/10298436.2010.489114
Adhikari, S., and You, Z. (2011). Investigating the Sensitivity of Aggregate Size within Sand Mastic by Modeling the Microstructure of an Asphalt Mixture. J. Mater. Civil Eng. 23. doi:10.1061/(asce)mt.1943-5533.0000212
Chang, M., Pei, J., and Zhang, J. (2011). “Influences of Mesoscopic Parameters on Crack Resistance of Asphalt Mixture,” in ICCTP 2011: Towards Sustainable Transportation Systems, 3324–3332. doi:10.1061/41186(421)329
Chen, J., Pan, T., Chen, J., Huang, X., and Lu, Y. (2012). Predicting the Dynamic Behavior of Asphalt concrete Using Three-Dimensional Discrete Element Method. J. Wuhan Univ. Technol. Mater. Sci. Edition 27. doi:10.1007/s11595-012-0470-y
Cundall, P. (1974). A Computer Model for Rock Mass Behavior Using Interactive Graphics for the Input and Output of Geometrical Data. A Report Prepared under Contract Number DACW 45-74-C-006. Washington, D.C.: The National Academies of Sciences, Engineering, and Medicine.
Cundall, P. A. (1971). “A Computer Model for Simulating Progressive, Large-Scale Movement in Blocky Rock System,” in Symposium of the International Society for Rock Mechanics.
Cundall, P. A. (1978). BALL–A Program to Model Granular media Using the Distinct Element Method. London, United Kingdom: Dames & Moore. Advanced Technology Group". Technical Note No. TN-LN.
Cundall, P. A., and Strack, O. D. L. (1979). A Discrete Numerical Model for Granular Assemblies. Géotechnique 29, 47–65. doi:10.1680/geot.1979.29.1.47
Dan, H.-C., Zhang, Z., Chen, J.-Q., and Wang, H. (2018). Numerical Simulation of an Indirect Tensile Test for Asphalt Mixtures Using Discrete Element Method Software. J. Mater. Civ. Eng. 30, 04018067. doi:10.1061/(asce)mt.1943-5533.0002252
Ding, X., Ma, T., and Huang, X. (2019). Discrete-Element Contour-Filling Modeling Method for Micromechanical and Macromechanical Analysis of Aggregate Skeleton of Asphalt Mixture. J. Transp. Eng. Part. B: Pavements 145, 04018056. doi:10.1061/jpeodx.0000083
Du, J., Ren, D., Ai, C., and Qiu, Y. (2021). Study on Low Temperature Crack Propagation and Evolution of Asphalt Mixture[J/OL]. J. Building Mater., 1–10.
Gao, Y. (2021). Experimental Study on Rheological Properties of Sulfur SBS Modified Asphalt and Pavement Performance of its Mixture[J]. Synth. Mater. Aging Appl. 50, 67–69+22.
Ge, H., Quezada, J. C., Houerou, V. L., and Chazallon, C. (2021). Three-dimensional Simulation of Asphalt Mixture Incorporating Aggregate Size and Morphology Distribution Based on Contact Dynamics Method. Construction Building Mater. 302, 124124. doi:10.1016/j.conbuildmat.2021.124124
Ghafoori Roozbahany, E., Partl, M. N., and Jelagin, D. (2019). Modelling the Flow of Asphalt under Simulated Compaction Using Discrete Element. Construction Building Mater. 227, 116432. doi:10.1016/j.conbuildmat.2019.07.158
Guo, H., and Chen, S. (2014). Discrete Element Numerical Analysis of Splitting Test of Open-Graded Large-Size Asphalt Macadam Mixture[J]. J. Highw. Transportation Res. Dev. 31, 22–26+40.
Guo, H., and Chen, S. (2015). Numerical Analysis on the Splitting Test of Open-Graded Large Stone Asphalt Mixture Based on Discrete Element Method. J. Highw. Transportation Res. Dev. 9. doi:10.1061/jhtrcq.0000434
Guo, Z. (2020). Influence of Void Distribution Based on Discrete Element Method on Shear Fatigue Life of Asphalt Mixture[J]. Low Temperature Architecture Tech. 42, 11–14.
Hou, S., Zhang, D., Huang, X., and Zhao, Y. (2015). Investigation of Micro-mechanical Response of Asphalt Mixtures by a Three-Dimensional Discrete Element Model. J. Wuhan Univ. Technol.-Mat. Sci. Edit. 30, 338–343. doi:10.1007/s11595-015-1150-5
Huang, Z.-y., Yang, Z.-x., and Wang, Z.-y. (2008). Discrete Element Modeling of Sand Behavior in a Biaxial Shear Test. J. Zhejiang Univ. Sci. A. 9, 1176–1183. doi:10.1631/jzus.a0720059
Jia, H., Sheng, Y., Lv, H., Kim, Y. R., Zhao, X., Meng, J., et al. (2021). Effects of Bamboo Fiber on the Mechanical Properties of Asphalt Mixtures[J]. Construction Building Mater. 289. doi:10.1016/j.conbuildmat.2021.123196
Kusumawardani, D. M., and Wong, Y. D. (2020). The Influence of Aggregate Shape Properties on Aggregate Packing in Porous Asphalt Mixture (PAM). Construction Building Mater. 255, 119379. doi:10.1016/j.conbuildmat.2020.119379
Li, Q., Wu, C., Fang, Y., Zhong, M., Huang, H., and Han, Q. (2021). Performance Research and Application of SMA-13 Asphalt Mixture with Plant Mixed Hot Recycling[J/OL]. Highw. Eng., 1–12.
Li, Z., Jing, H., and Wang, Z. (2020). Multi-factor Interaction of Asphalt Mixture Volume Characteristics Based on Discrete Element Method[J]. J. Guangxi Univ. (Natural Sci. Edition) 45, 1301–1314.
Liang, H., Shi, L., Wang, D., Xiao, X., and Deng, K. (2021). Influence of Graded Coarse Aggregate Content and Specific Surface Area on the Fracture Properties of Asphalt Mixtures Based on Discrete Element Simulations and Indoor Tests. Construction Building Mater. 299, 123942. doi:10.1016/j.conbuildmat.2021.123942
Liang, H., Wang, D., Deng, Z., Liang, X., and Xu, C. (2019). Application of Cohesive Zone Model in Discrete Element Simulation of Foamed Asphalt Mixture[J]. J. Hunan Univ. (Natural Sciences) 46, 115–123.
Ling, T., Yang, Y., Zhao, C., Zhu, L., and Chen, Q. (2020). Design of Asphalt Mixture with Low Voidage Based on PFC3D[J]. J. Chongqing Jiaotong Univ. (Natural Science) 39, 73–80.
Liu, C., Le, T., Shi, B., and Zhu, Y. (2020). Discussion on Three Major Problems of Engineering Application of the Particle Discrete Element Method. Chin. J. Rock Mech. Eng. 39, 1142–1152.
Liu, G., Dai, J., Liu, Y., Gao, H., and Peng, Y. (2018). Numerical Simulation of Aggregate Uniformity Influence on Low Temperature Splitting Strength of Asphalt Mixtures[J]. Low Temperature Architecture Tech. 40, 12–16.
Liu, Y., Dai, Q., and You, Z. (2009). Viscoelastic Model for Discrete Element Simulation of Asphalt Mixtures. J. Eng. Mech. 135. doi:10.1061/(asce)0733-9399(2009)135:4(324)
Liu, Y. (2011). Discrete Element Methods for Asphalt concrete: Development and Application of User-Defined Microstructural Models and a Viscoelastic Micromechanical Model. Houghton, MI: Michigan Technological University.
Liu, Y., and You, Z. (2011a). Accelerated Discrete-Element Modeling of Asphalt-Based Materials with the Frequency-Temperature Superposition Principle. J. Eng. Mech. 137, 355–365.
Liu, Y., and You, Z. (2011b). Accelerated Discrete-Element Modeling of Asphalt-Based Materials with the Frequency-Temperature Superposition Principle. J. Eng. Mech. 137, 355–365. doi:10.1061/(asce)em.1943-7889.0000234
Liu, Y., and You, Z. (2008). “Simulation of Cyclic Loading Tests for Asphalt Mixtures Using User Defined Models within Discrete Element Method,” in GeoCongress 2008: Characterization, Monitoring, and Modeling of Geosystems, 742–749.
Liu, Y., You, Z., and Zhao, Y. (2012). Three-dimensional Discrete Element Modeling of Asphalt concrete: Size Effects of Elements. Construction Building Mater. 37, 775–782. doi:10.1016/j.conbuildmat.2012.08.007
Lu, J. (2020). Study on Numerical Simulation of Asphalt Mixture Based on CT Technology and Three-Dimensional Discrete Element Method[D]. Master. Guangzhou, China: South China University of Technology.
Ma, T., Zhang, D.-y., Zhang, Y., Zhao, Y.-l., and Huang, X.-m. (2016b). Microstructure Modeling and Virtual Test of Asphalt Mixture Based on Three-Dimensional Discrete Element Method. J. Cent. South. Univ. 23, 1525–1534. doi:10.1007/s11771-016-3204-z
Ma, T., Zhang, D., Zhang, Y., Wang, S., and Huang, X. (2018). Simulation of Wheel Tracking Test for Asphalt Mixture Using Discrete Element Modelling. Road Mater. Pavement Des. 19, 367–384. doi:10.1080/14680629.2016.1261725
Ma, T., Zhang, D., Zhang, Y., Zhao, Y., and Huang, X. (2016a). Effect of Air Voids on the High-Temperature Creep Behavior of Asphalt Mixture Based on Three-Dimensional Discrete Element Modeling. Mater. Des. 89, 304–313. doi:10.1016/j.matdes.2015.10.005
Mao, Z. (2018). The Research on Creep of Asphalt Mortar Based on Three-Dimensional Discrete Element[J]. Low Temperature Architecture Tech. 40, 4–6+13.
Meng, L., Zhang, W., and Wang, X. (2021). Research on the Effect of Chlorine Salt Snow-Melting Agent on Asphalt concrete Performance[J]. J. Qingdao Univ. Tech. 42, 45–51.
Nian, T., Ge, J., Li, P., Wang, M., and Mao, Y. (2021). Improved Discrete Element Numerical Simulation and experiment on Low-Temperature Anti-cracking Performance of Asphalt Mixture Based on PFC2D. Construction Building Mater. 283, 122792. doi:10.1016/j.conbuildmat.2021.122792
Pei, J., Chang, M., Chen, S., and Wang, B. (2010). Numerical Simulation of Indirect Tensile Test for Asphalt Mixture[J]. J. Chang'an Univ. (Natural Sci. Edition) 30, 6–10.
Peng, Y., and Bao, J. (2018). Comparative Study of 2D and 3D Micromechanical Discrete Element Modeling of Indirect Tensile Tests for Asphalt Mixtures. Int. J. Geomechanics 18. doi:10.1061/(asce)gm.1943-5622.0001155
Peng, Y., Bao, J., and Wang, Z. (2016). A Comparison of Two-Dimensional and Three-Dimensional Micromechanical Discrete Element Modeling of the Splitting Tests for Asphalt Mixtures. DEStech Trans. Eng. Tech. Res.
Peng, Y., Gao, H., Wan, L., and Liu, G. (2019). Numerical Simulation of Influence Factors of Splitting Strength of Asphalt Mixtures[J]). J. Jilin Univ. (Engineering Tech. Edition) 49, 1521–1530.
Peng, Y., and Sun, L. (2015a). Aggregate Distribution Influence on the Indirect Tensile Test of Asphalt Mixtures Using the Discrete Element Method. Int. J. Pavement Eng. 18. doi:10.1080/10298436.2015.1121778
Peng, Y., and Sun, L. J. (2015b). Aggregate Distribution Influence on the Indirect Tensile Test of Asphalt Mixtures Using the Discrete Element Method [J]. Int. J. Pavement Eng. 18, 1–14. doi:10.1080/10298436.2015.1121778
Peng, Y., Yang, H., Lu, X., and Li, Y. (2021). Effect of Void Characteristics on Virtual Shear Fatigue Life of Asphalt Mixtures Using Discrete Element Method[J]. J. Jilin Univ. (Engineering Tech. Edition) 51, 956–964.
Shan, J., Du, Y., Fan, D., Guo, L., and Yang, X. (20192019). Determination of Parameters of the Discrete Element Bond Model for Asphalt Mixture Based on Splitting Test. Adv. Civil Eng. doi:10.1155/2019/5047214
Sheng, F., Hou, L., Wang, Y., Li, Z., and Shan, J. (2018). Studying on the Parameters of Bond-Model in DEM for Asphalt Mixture. IOP Conf. Ser. Mater. Sci. Eng. 392. doi:10.1088/1757-899x/392/2/022004
Shi, Y. (2020). Simulation Study on Split Test of Asphalt Mixture with Flat-Elongated Particles Using Discrete Element Method[D]. Master. Harbin, China: Harbin Engineering University.
Song, W. (2021). Influence of Polymer Fiber and Glass on the Mechanical Properties of Asphalt Mixture[J/OL]. Highway 66, 29–33.
Wan, L. (2016). Study on Asphalt Mixture Splitting Test Using Cohesive Zone Model and Three-Dimensional Discrete Element Method[D]. Master. Zhejiang, China: Zhejiang University.
Wang, H., Huang, W., Cheng, J., and Ye, G. (2021). Mesoscopic Creep Mechanism of Asphalt Mixture Based on Discrete Element Method. Construction Building Mater. 272, 121932. doi:10.1016/j.conbuildmat.2020.121932
Wang, W., and Li, X. (2005). A Review on Fundamentals of Distinct Element Method and its Applications in Geotechnical Engineering[J]. Beijing, China: Geotechnical Engineering Technique, 177–181.
Wang, X., Zheng, D., and Yu, C. (2019). Application of Discrete Element in the Teaching of "Asphalt and Asphalt Mixture. Education Modernization 6, 218–220.
Wang, Y. (1990). “Development of Discrete Element Method and its Application in Geotechnical Engineering[A],” in Engineering Application of Numerical Methods in Rock and Soil Mechanics——Proceedings of the Second National Symposium on Numerical Calculation and Model Experiments in Rock Mechanics, 44–54.
Wang, Y. (1986b). Discrete Element Method and its Application in Rock mechanics[J]. Shenyang, China: Metal Mine, 13–17+15.
Wang, Y. (1986a). Discrete Element Method—A Numerical Method Suitable for Analysis in Jointed Rocks. Proc. first Natl. Symp. Numer. calculation Model Test Rock Mech. 6.
Wang, Y., Liu, G., and Xing, J. (1987). Research on Applications of Discrete Element Method in Ore Drawing for Caving system[J]. Beijing, China: Non-ferrous metal, 20–26.
Wang, Y., and Xing, J. (1993a). Discrete Element Method and its Application in Coal Mining (Lecture 1)[J]. Coal Mining Tech., 55–58.
Wang, Y., and Xing, J. (1993b). Discrete Element Method and its Application in Coal Mining (Lecture 2)[J]. Coal Mining Tech., 56–58.
Wang, Y., and Xing, J. (1986). Discrete Element Method and its Applications in Mine Pressure research[J]. Fuxin, China: Journal of Fuxin Institute of Mining Technology, 1–12.
Wang, Y., and Xing, J. (1990). “Fully Deformed Discrete Element Method and its Application in Mining[A],” in Rock Mechanics Issues in Hydropower and Mining Engineering——Proceedings of the Academic Conference on Rock Mechanics and Engineering Applications in North China, 502–508.()
Wang, Y., and Xing, J. (1989). “Improvement and Application of Discrete Element Method[A],” in Engineering Application of Numerical Methods in Rock and Soil Mechanics——Proceedings of the Second National Symposium on Numerical Calculation and Model Experiments in Rock Mechanics, 254–259.
Wang, Y., and Xing, J. (1991). “New Progress of Numerical Methods and Their Engineering Applications[A],” in New Progress in Rock Mechanics, 168–192.()
Wu, W., Si, L., and Lu, J. (2021). Influence of Aggregate on Study on Shear Performance of Asphalt Mixture Based on Virtual Uniaxial Penetration Test[J]. J. Highw. Transportation Res. Dev. 38, 1–8.
Xia, Q., Gao, J., Zhang, Q., Li, Z., Yang, F., and Luo, H. (2021). Low-temperature Performance of Composite Modified Hard Asphalt Used in High Modulus Asphalt concrete[J/OL]. Changchun, China: Journal of Jilin University Engineering and Technology Edition, 1–9.
Xing, J., and Wang, Y. (1988). Discrete Element Simulation of Ore Drawing by Caving Method in Mining[J]. Shenyang, China: Journal of Northeast University of Technology, 148–153.
Xing, J., and Wang, Y. (1990). Improvement of Discrete Element Method and its Applications in Granular Media[J]. Chin. J. Geotechnical Eng., 51–57.
Xiong, Y. (2013). Analysis of the Influence of Aggregate Properties on Splitting Test of Asphalt Concrete Based on Discrete Element Method[J]. J. China Foreign Highw. 33, 318–322.
Yang, X., You, Z., Wang, Z., and Dai, Q. (2016). Review on Heterogeneous Model Reconstruction of Stone-Based Composites in Numerical Simulation. Construction Building Mater. 117, 229–243. doi:10.1016/j.conbuildmat.2016.04.135
Yao, L., Gao, W., Tao, F., and Wang, H. (2021). Effects of Bayer Red Mud as Filler on Properties of Asphalt Mixture[J/OL]. Highway 66, 61–68.
You, Z., Adhikari, S., and Dai, Q. (2010). Air Void Effect on an Idealised Asphalt Mixture Using Two-Dimensional and Three-Dimensional Discrete Element Modelling Approach. Int. J. Pavement Eng. 11. doi:10.1080/10298436.2010.488737
You, Z., Adhikari, S., and Dai, Q. (2008). Three-Dimensional Discrete Element Models for Asphalt Mixtures. J. Eng. Mech. 134. doi:10.1061/(asce)0733-9399(2008)134:12(1053)
You, Z., Adhikari, S., and Kutay, M. E. (2009). Dynamic Modulus Simulation of the Asphalt concrete Using the X-ray Computed Tomography Images. Mater. Structures 42. doi:10.1617/s11527-008-9408-4
You, Z., Liu, Y., and Dai, Q. (2011). Three-Dimensional Microstructural-Based Discrete Element Viscoelastic Modeling of Creep Compliance Tests for Asphalt Mixtures. J. Mater. Civil Eng. 23. doi:10.1061/(asce)mt.1943-5533.0000038
You, Z., and Liu, Y. (2010). Three-Dimensional Discrete Element Simulation of Asphalt Concrete Subjected to Haversine. Loading an Application of the Frequency-Temperature Superposition Technique. Road Mater. Pavement Des. 11, 273–290. doi:10.3166/rmpd.11.273-290
Yu, D. (2015). Splitting Test Modeling of Asphalt Mixture Using Particle Flow Code[J]. Mod. Transportation Tech. 12, 1–4.
Zhang, D., and Li, Y. (2017). Numerical Simulation of Asphalt Concrete’s Porosity Variation under Dynamic Loading[J]. J. Xuzhou Inst. Tech. (Natural Sci. Edition) 32, 73–76.
Zhang, H., Lian, H., and Yang, D. (2015). Split Test and Numerical Simulation of Crumb Rubber-Modified Asphalt Mixture[J]. Mater. Rep. 29, 145–148.
Zhang, Y., Ma, T., Ding, X., Chen, T., Huang, X., and Xu, G. (2018). Impacts of Air-Void Structures on the Rutting Tests of Asphalt concrete Based on Discretized Emulation. Construction Building Mater. 166, 334–344. doi:10.1016/j.conbuildmat.2018.01.141
Zhang, Y., and Zhang, B. (2016). “Blast Furnace for Bell Distribution Chute Research Based on StreamDEM[A],” in Proceedings of the 12th China CAE Engineering Analysis Technology Annual Conference, 141–143.
Zhang, Y., Zhong, X., Zhong, Y., and Zhang, B. (2017). “Study on the First Layer of Blast Furnace Fabric Based on StreamDEM[A],” in Proceedings of the 11th China Iron and Steel Annual Conference-S01 (Beijing, China: Ironmaking and Raw Materials), 830–834.
Zhong, X., and Zhang, Y. (2021). Influence of Fiber Type on Road Performance of High RAP Content Asphalt Mixture[J]. Highways & Transportation in Inner Mongolia, 29–33.
Zhou, J. (2016). Laboratory Test and Numerical Simulation for Dynamic Modulus of Asphalt Mortar[J]. Mod. Transportation Tech. 13, 1–5.
Keywords: pavement engineering, asphalt, asphalt mixtures, discrete element, modeling
Citation: Yao H, Xu M, Liu J, Liu Y, Ji J and You Z (2022) Literature Review on the Discrete Element Method in Asphalt Mixtures. Front. Mater. 9:879245. doi: 10.3389/fmats.2022.879245
Received: 19 February 2022; Accepted: 25 March 2022;
Published: 14 April 2022.
Edited by:
Antonio Caggiano, Darmstadt University of Technology, GermanyReviewed by:
Tao Ma, Southeast University, ChinaTuo Huang, Changsha University of Science and Technology, China
Augusto Cannone Falchetto, Aalto University, Finland
Copyright © 2022 Yao, Xu, Liu, Liu, Ji and You. This is an open-access article distributed under the terms of the Creative Commons Attribution License (CC BY). The use, distribution or reproduction in other forums is permitted, provided the original author(s) and the copyright owner(s) are credited and that the original publication in this journal is cited, in accordance with accepted academic practice. No use, distribution or reproduction is permitted which does not comply with these terms.
*Correspondence: Hui Yao, aHVpeWFvQG10dS5lZHU=