- 1Clinical and Translational Research Center, School of Medicine, Shanghai First Maternity and Infant Hospital, Tongji University, Shanghai, China
- 2Department of Obstetrics, School of Medicine, Shanghai First Maternity and Infant Hospital, Tongji University, Shanghai, China
- 3Department of Reproductive Immunology, School of Medicine, Shanghai First Maternity and Infant Hospital, Tongji University, Shanghai, China
Premature ovarian failure (POF) and intrauterine adhesion (IUA) that easily lead to reduced fertility in premenopausal women are two difficult diseases to treat in obstetrics and gynecology. Hormone therapy, in vitro fertilization and surgical treatments do not completely restore fertility. The advent of hydrogels offers new hope for the treatment of POF and IUA. Hydrogels are noncytotoxic and biodegradable, and do not cause immune rejection or inflammatory reactions. Drug delivery and stem cell delivery are the main application forms. Hydrogels are a local drug delivery reservoir, and the control of drug release is achieved by changing the physicochemical properties. The porous properties and stable three-dimensional structure of hydrogels support stem cell growth and functions. In addition, hydrogels are promising biomaterials for increasing the success rate of ovarian tissue transplantation. Hydrogel-based in vitro three-dimensional culture of follicles drives the development of artificial ovaries. Hydrogels form a barrier at the site of injury and have antibacterial, antiadhesive and antistenosis properties for IUA treatment. In this review, we evaluate the physicochemical properties of hydrogels, and focus on the latest applications of hydrogels in POF and IUA. We also found the limitations on clinical application of hydrogel and provide future prospects. Artificial ovary as the future of hydrogel in POF is worth studying, and 3D bioprinting may help the mass production of hydrogels.
Introduction
In obstetrics and gynecology, both premature ovarian failure (POF) and intrauterine adhesion (IUA) are difficult diseases that often cause infertility in premenopausal women. POF is defined as a primary ovarian defect characterized by premature follicular depletion or impaired folliculogenesis before the age of 40 years (Beck-Peccoz and Persani, 2006). Known causes of POF mainly include autoimmune diseases, chromosomal abnormalities, radiation and chemotherapy for tumors, infections, and surgical injuries, these causes are difficult to avoid (Goswami and Conway, 2005). POF patients experience early menopause and are susceptible to cardiovascular disease, type 2 diabetes and osteoporosis, and this susceptibility may be related to a decrease in estrogen (Wu et al., 2014). Hormone replacement therapy (HRT) replenishes ovarian hormone deficiencies and improves menopausal syndrome, but may increase the risk of breast cancer and stroke (Sullivan et al., 2016). A low pregnancy rate (5%–10%) is the biggest problem for POF patients (van Kasteren and Schoemaker, 1999). HRT and gonadotropin-releasing hormone agonists are not effective in restoring fertility (Demeestere et al., 2016; Ishizuka et al., 2021). In vitro fertilization (IVF) may allow POF patients to have healthy children, but the success rate is low especially after the age of 35 (Ishizuka et al., 2021).
IUA is defined as adhesion in uterine cavity resulting from endometrial damage, characterized by amenorrhea, infertility, pregnancy loss and abnormal placentation (Dreisler and Kjer, 2019). IUA is mainly due to surgical operations in the uterine cavity, and extensive fibrosis of the uterine cavity leads to failure of decidualization and embryo implantation (Hou et al., 2019). Transcervical resection of adhesion (TCRA) is a standardized treatment that loosens adhesion and improves fertility. TCRA itself is also an intrauterine surgical operation, adhesion in the uterine cavity can be removed by TCRA, but there is also a risk of damage to the endometrium caused by the operation of TCRA, and the risk of re-adhesion after surgery is difficult to minimize (March 2011). The recurrence rate of severe IUA patients even exceeds 60% after TCRA (Capella-Allouc et al., 1999). The clinical pregnancy rate of IUA patients does not exceed 20% after TCRA (20%) (Wang Z. et al., 2021), transdermal estrogen treatment (16.67%) (Chi et al., 2018) and IVF (15.3%) (Mao et al., 2020).
Hydrogels are a cross-linked form of hydrophilic polymers (Tibbitt and Anseth, 2009). The structure and composition of hydrogels are similar to those of the extracellular matrix; thus, hydrogels show great potential for biological and medical applications (Slaughter et al., 2009). Hydrogel-based treatments have emerged in many diseases, including bone injury, skin injury, kidney diseases, heart failure, etc (Mohapatra et al., 2021). Hydrogels have been extensively studied in skin injury. To date, at least 43 clinical trials have reported that hydrogel dressings increased the cure rate of skin injury and relieved pain, and in these trials no adverse effects were found (Zhang et al., 2019). The safety and efficacy in the treatment of skin injury has driven the application of hydrogels in other diseases. The application of hydrogels in other diseases is still at the stage of animal experiment. In animal experiment the therapeutic effects and safety of hydrogels have been exhibited. Both drug-loaded and stem cell-loaded hydrogels promoted the regeneration of bone tissue (Hasani-Sadrabadi et al., 2020; Zuo et al., 2022). IL-10-loaded hydrogels reduced the fibrosis of chronic kidney disease (Rodell et al., 2015), and mitigated the local and systemic inflammation of acute kidney injury (Soranno et al., 2016). After myocardial infarction, necrotic cardiomyocytes are replaced by fibroblasts, resulting in impaired electrical signal conduction. Conductive hydrogels effectively improved electrical signal transmission and protected ventricular function (Bao et al., 2017; Zhang, C et al., 2020).
In obstetrics and gynecology, the main application of hydrogel is in the treatment of POF and IUA. Hydrogels have been studied for ovarian tissue transplantation and artificial ovary, and the three-dimensional structure of hydrogels provides support for follicle growth and morphology maintenance, making ovarian tissue and follicle autologous transplantation possible (Shikanov et al., 2009; Yoon et al., 2021). The application of hydrogels in IUA is mainly focused on drug delivery and stem cell delivery therapy (Mettler et al., 2008; Xue et al., 2019). Hydrogels are capable of controlling drug release and extending stem cell retention (Zhang S. et al., 2017; Huang et al., 2022). In this review, we discuss the physicochemical properties of hydrogels and the latest applications of hydrogels in POF and IUA. Applications of hydrogels in POF and IUA were studied mainly in animal models, and hydrogels have shown therapeutic effects in animal models, but we still have a long way to go for clinical application; hence, we discuss the limiting factors that hinder clinical application and we highlight the future prospects for the applications of hydrogels.
Physicochemical Properties
Swelling is a phenomenon whereby the volume of hydrogels rapidly increases after they absorb a solvent. The dried hydrogels were immersed in phosphate buffer solution (PBS) (Qi et al., 2020), medium (Qi et al., 2020), double-distilled water (DDW) (Zheng et al., 2021) or deionized water (Sutthasupa et al., 2021) according to different experimental designs. Then swollen hydrogels were removed from the solvent at a certain time, and weighed after gently blotting water on the surface. The swelling ratio (SR) was calculated by the following equation: SR=(Ws-Wi)/Wi×100 (Wi: the weight of initial dried hydrogel; Ws: the weight of swollen hydrogels) (Qi et al., 2020; Sutthasupa et al., 2021; Zheng et al., 2021). SR is associated with the hydroxyl groups, hydrophilic groups and carboxyl groups (Lv et al., 2021), and the pH and ionic strength of solutions also affect the SR (Ganguly et al., 2017). The swelling property confers a swollen three-dimensional (3D) structure resembling the extracellular matrix in which cells physiologically live; therefore, researchers increasingly regard hydrogels as novel cell culture media (Jackson et al., 2009; Xu et al., 2009; West et al., 2007a). Traditional 2D culture achieve the survival of follicles in vitro, but cannot maintain the normal morphology of follicles (Desai et al., 2012; Choi et al., 2013), the 3D structure of the swollen hydrogels maintains the normal morphology of the follicles in vitro. Hydrogels are promisingly applied to treat IUA, because hydrogels are not easily expelled due to the strong adhesion to uterine walls (Zhang, W et al., 2020; Yang et al., 2020), and the stable 3D structure due to swelling prevents adjacent uterine walls from directly contacting (Mettler et al., 2008; Wang, Z. et al., 2021).
Rheology, which is defined by Eugene Bingham, is the study of the flow and deformation of materials under applied forces (Dawn and Kumari., 2018; Wilson, 2018). The rheology of hydrogels is measured by a rheometer. The storage modulus (G′) and the loss modulus (G″) parameters are always used, the storage modulus (G′) reflects viscous behavior and loss modulus (G″) reflects elastic behavior (Müller et al., 2011; Zheng et al., 2021). The viscous behavior facilitates the injection of the hydrogel from the syringe into the uterine cavity and adhesion to the uterine cavity; moreover, the elastic behavior facilitates the retention of the hydrogel in the uterine cavity (Xu HL. et al., 2017). Hydrogels that exhibit more viscous behavior under large frequency and exhibit more elastic behavior under low frequency are appropriately applied to IUA (Müller et al., 2011). Thermosensitive hydrogels exhibit more viscous behavior at 4°C. After the hydrogels are injected into the uterine cavity, the temperature increases gradually and reaches the gelation temperature, and hydrogels exhibit more elastic behavior (Xu HL. et al., 2017).
Biocompatibility is the ability of biomaterials to perform without adverse host responses in their application (Naahidi et al., 2013). Sterility is a prerequisite for clinical applications, and the assurance of no introduced pathogens is imperative after hydrogels are implanted. Hydrogels are sterilized by high pressure steam, 75% ethanol and UV light according to their physicochemical properties (Müller et al., 2011; Zheng et al., 2021). Some hydrogels, such as antibacterial electroactive self-healing hydrogels, have antibacterial and antioxidant properties in their own composition, but aseptic operation is also necessary in the preparation process (Zhao X. et al., 2017). Cell-loaded hydrogels are applied to treat diseases; however, encapsulated cells may lead to host immune rejection. MHC molecules are the critical bridge for the recognition of allogeneic cells through host lymphocytes; therefore, host immune rejection can be examined by detecting the expression of MHC molecules (Yuan et al., 2011). In addition, the one-way mixed lymphocyte reactions (MLR) assay can also detect host immune rejection (Yuan et al., 2010). The cytotoxicity of hydrogels to encapsulated cells and host cells is not negligible, and the migration and viability of cells reflect whether hydrogels are cytotoxic to cells (Zhao X. et al., 2017; Cui et al., 2019). In addition, the growth of encapsulated cells requires the hydrogels to complete the exchange of oxygen, nutrients and metabolites (Slaughter et al., 2009). Many factors affect the biocompatibility of hydrogels; for natural hydrogels, natural sources may contain heavy metals, endotoxins, and polyphenolic compounds (Lee and Mooney, 2012), and the purification process (Kong et al., 2002), the structure and formulation of the biomaterials affect biocompatibility (Tabata et al., 1999).
Biodegradability provides space for cell growth and new tissue formation (Li et al., 2012). High-quality hydrogels require proper biodegradability, and the degradation products are not harmful to tissues and cells. Nondegradable biomaterials accumulate in the mononuclear phagocytic system and are difficult to excrete; this accumulation is permanent and may be potentially harmful to the body (Owens and Peppas, 2006). Natural hydrogels, such as chitosan, alginate, gelatin and heparin, are mainly derived from natural sources and are readily degraded by cells in vivo (Yamaguchi et al., 2007). The following factors affect hydrogel degradability: different crosslinking methods (Jeon et al., 2009), water content (Tabata et al., 1999) and the ratio of each component (Yu et al., 2010).
Premature Ovarian Failure
Premature ovarian failure (POF) is defined as amenorrhea due to ovarian hypofunction before the age of 40 years (Goswami and Conway, 2005). A wide range of causes lead to POF, including chemotherapy, autoimmune diseases, infection and genetic aberrations; however, most causes are not clear and known causes are difficult to avoid (Goswami and Conway, 2005). The most difficult problem of POF is the reduced fertility of premenopausal women; in addition, the dyspareunia due to decreased sexual function and frequent night sweats due to abnormal vasodilation also cause distress in patients (Schover, 2008). Hormone replacement therapy (HRT) is the main treatment strategy for POF, but hormone therapy may lead to an increased probability of breast cancer and stroke (Rossouw et al., 2002); moreover, HRT only relieves the uncomfortable symptoms of POF and cannot fully restore the function of the ovaries (Sullivan et al., 2016). In vitro oocyte maturation successfully restores fertility in POF patients, but these are case reports, and more statistical data are needed to confirm the efficiency of this technique (Grynberg et al., 2020; Lucie et al., 2021). The fertility rate after IVF is low, and the success rate is related to age and disease duration (Ishizuka et al., 2021); therefore, novel treatment strategies are urgently needed.
The Application of Hydrogels in POF
Hydrogels are introduced in POF treatment in the following four ways (shown in Figure 1). The first method is transplantation of ovarian tissues. POF may occur in cancer patients after radiation and chemotherapy, and the autologous transplantation of ovarian tissue that are frozen before radiation and chemotherapy improved the patient’s menopausal symptoms (Fabbri et al., 2019); however, the patient’s pregnancy rate is low. One report showed only seven pregnancies in 21 patients who received autologous transplantation (Jadoul et al., 2017), and fertility was not fully restored despite orthotopic transplantation of ovarian tissue (von Wolff et al., 2009). Hydrogel-coated ovarian tissue transplantation was superior to autologous transplantation alone in promoting follicular proliferation in animal models; however, there are no reports of fertility restoration due to the difficulty of achieving orthotopic transplantation in animal models. Tavana et al. (2016) encapsulated ovarian tissues in a hyaluronic acid (HA) hydrogel containing VEGF and fibroblast growth factor (bFGF), and the hydrogel promoted follicle proliferation and restoration of ovarian function and inhibited apoptosis after transplantation. Tanaka et al. (2018) wrapped human ovarian tissues in a gelatin hydrogel containing bFGF and transplanted them into immunodeficient mice, found that primordial and primary follicular density significantly increased. Ovarian tissue allotransplantation may be achieved by hydrogel encapsulation because hydrogel-encapsulated ovarian tissue transplantation did not cause follicle apoptosis or immune rejection. Gao et al. (2013) performed allotransplantation with fibrin hydrogels containing bFGF-coated ovarian tissues, and found that the number of apoptotic follicles was significantly reduced. Day et al. (2019) demonstrated that poly (ethylene-glycol) (PEG) hydrogel-coated ovarian tissue prevents allotransplantation immune rejection.
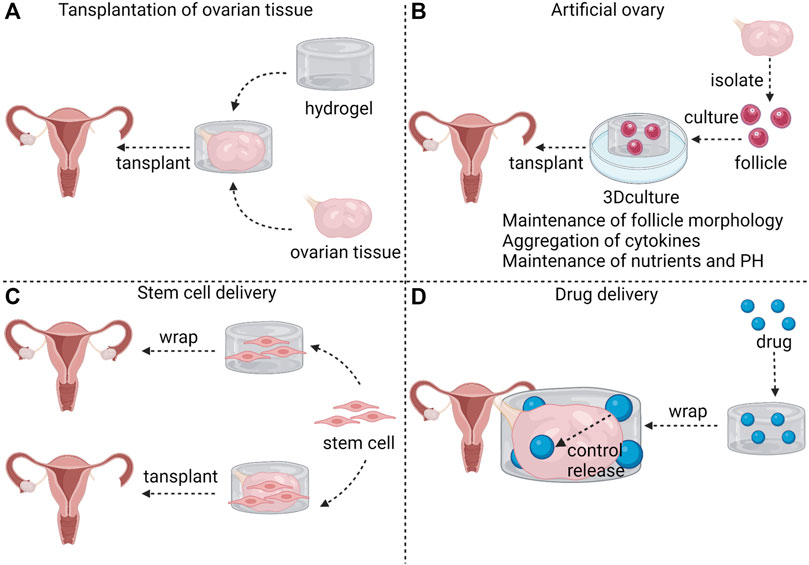
FIGURE 1. The application of hydrogels in POF. Hydrogel can be used as a carrier for ovarian tissue transplantation (A). Hydrogel 3D culture is characterized by maintenance of follicle morphology, aggregation of cytokines and maintenance of nutrients and PH; thus, hydrogel can be used as a material for artificial ovaries (B). Stem cells can be encapsulated in hydrogel to wrap ovaries in POF patients to exert therapeutic effects; Stem cells can also be encapsulated in hydrogel along with ovarian tissues for ovarian transplantation (C). Hydrogels surrounding ovaries control the rate of drug release (D).
The second method of hydrogel application is artificial ovary. Artificial ovary mainly consists of isolated follicles and biocompatible and biodegradable biomaterials. Transplanting ovarian tissues is a major method through which to restore fertility in cancer patients, but tissue transplantation easily reintroduces tumor cells (Camboni et al., 2013). Follicular basal lamina does not have capillaries and nerves from the granulosa layer; thus, tumors metastasis to the follicles is not possible (Rodgers et al., 2003). Isolated follicles may be transplanted into patients instead of ovarian tissue, and the storage and culture of follicles in vitro is the foundation for artificial ovary feasibility (See The Application of Hydrogels in Follicle Culture for details). Studies have shown that the transplantation of artificial ovaries promotes the survival and maturation of follicles in vivo (Rios et al., 2018), improves local vascularization (Rajabzadeh et al., 2020), and restores hormones to a level at which the endometrium regenerates (Yoon et al., 2021).
The third method is stem cell delivery. Mesenchymal stem cells (MSCs) treatment in POF increased the number of follicles, restored plasma sex hormone levels (Zhang et al., 2021b), reduced ovarian inflammation and reduced granulosa cell apoptosis (Ling et al., 2017; Deng et al., 2021). Stem cells are mainly administered by caudal intraperitoneal injection or ovarian orthotopic injections (Zhang, Q et al., 2017; Woo et al., 2012). These traditional injection methods are associated with problems of low efficiency and growth restriction in target organs (Liu, S et al., 2016). Hydrogels enrich stem cells in the target organ and provide a suitable environment for the growth of stem cells. A study found that co-transplantation of fibrin-collagen hydrogel-coated MSCs and ovarian tissue increased the transplantation success rate (Mehdinia et al., 2020). Huang et al. (2021) embedded human amniotic epithelial cells (hAECs) in sodium alginate-bioglass (SA-BG) hydrogel and wrapped ovarian tissues with the hAECs-loaded hydrogel in POF mice. The SA-BG hydrogel not only enhanced the viability of hAECs in ovary, but also with the help of hAECs restored follicle development and enhanced angiogenesis in POF ovaries. To date, there are insufficient studies on the treatment of hydrogels combined with stem cells for POF; thus, whether this combination enhances efficacy for POF is worth investigating.
The fourth method is drug delivery. Hydrogels enrich drugs in the local tissue. This local delivery maintains the high concentration of drugs in the local tissue and reduces adverse effects (Bhattarai et al., 2010). Drugs are released from hydrogels in a controlled manner, avoiding burst release (Jiang et al., 2014). The long-term sustained release avoids multiple-dose regimens. Drug release is determined by molecular mass, degradation rate and affinity between drugs and hydrogels (Li and Guan., 2011). In addition, environment-stimulated release can also modulate the drug release. Temperature-responsive hydrogels are a good example, drug release is slow when the temperature is below the thermal transition temperature of the hydrogel. Once the temperature rises above the thermal transition temperature, the drug is released rapidly due to hydrogel contraction (Li and Guan, 2011). Electricity-response and magnetism-response hydrogels control the “on” and “off” of drug release by applying and withdrawing electric and magnetic fields (Murdan 2003; Ganguly and Margel, 2021). Excessive activation of mammalian target of rapamycin (mTOR) induces POF (Reddy et al., 2008). Rapamycine, a mTOR inhibitor, prolongs ovarian lifespan (Dou et al., 2017), but mTOR inhibitors may have adverse effects on the immune system (Araki et al., 2009). Ala-Glu-Ala-Ala-Leu-Tyr- Lys-Asn-Leu-Leu-His-Ser-OH (Inh), a peptide sequence, competitively inhibits the autophosphorylation of receptor tyrosine kinases (RTKs), which are the upstream kinases of mTOR (Thömmes et al., 1999). Hydrogels are able to control the release and ensure the enrichment of Inh, and Shi et al. (2021) reported that the Inh embedded in hydrogels delayed ovarian aging.
The Application of Hydrogels in Follicle Culture
Hydrogel-based in vitro three-dimensional (3D) culture is significantly superior to traditional two-dimensional (2D) culture, promoting the development of follicle science and advancing the emergence of artificial ovary. The advantages of 3D culture are shown in Figure 1. Traditional 2D culture fail to fully simulate the ovarian microenvironment for the following reasons: 1) The 3D structure of follicle cannot be easily maintained in 2D culture. Granulosa cells and theca cells attach to the dish surface and lose physiological morphology, and flat follicle disrupt intercellular communication (Desai et al., 2012; Choi et al., 2013); 2) Endogenous autocrine and paracrine factors are diluted, which affects cell development (West et al., 2007a); 3) Consumption of nutrients and accumulation of metabolites lead to pH fluctuations in the medium (Duval et al., 1992). Regular medium changes improve the culture environment, but increase the possibility of contamination and culture cost. The 3D structure of hydrogels simulates the native microenvironment and maintains follicle morphology. The follicular cavity, a closed and filled-fluid cavity, is an indicator of progressive follicular maturation. The normal morphology and expansion of the follicular cavity have been demonstrated in a fibrin-alginate hydrogel (Shikanov et al., 2009). The limited volume of the hydrogels slows down the escape of cellular secretions (West et al., 2007a), and the porous property of the hydrogels ensures the exchange of cellular secretions. The nutrients necessary for cell development can be encapsulated in a responsive polyurea hydrogel based on organic sol–gel chemistry, and the nutrients are constantly released to compensate for cellular consumption, thus maintaining a dynamic balance of nutrients. In addition, this hydrogel is able to simultaneously neutralize lactic acid and maintain pH (Skory et al., 2015).
Natural hydrogels are the most commonly used hydrogels for follicle culture. Alginate, mainly obtained from brown algae cell walls, is a linear water-soluble high swelling natural source. With the goal of facilitating better water solubility in cell culture and disease treatment, alginates are commonly converted into the form of monovalent salts, such as sodium alginates or calcium alginates (Kang et al., 2021). The concentration and stiffness of alginates influence follicle growth and development. Min Xu. et al isolated mouse follicles and demonstrated that 0.25% (w/v) and 0.5% (w/v) alginates promoted secondary follicle growth and follicular cavity expansion more rapidly than 1.0% (w/v) and 1.5% (w/v) alginates, and 0.25% (w/v) alginate was the optimal concentration given the high production of estradiol and mature oocytes (Xu et al., 2006). Alginates (0.3% w/v) are suitably applied in human follicle culture. More than half of the preantral follicles were viable and most of them were in a fast growing state in a 0.3% (w/v) alginate hydrogel (Yin et al., 2016); moreover, for primary and secondary follicles, steroid and peptide hormone secretion patterns similar to those observed in vivo were induced after the sequential addition of follicle-stimulating hormone (FSH), human chorionic gonadotrophin (hCG) and epidermal growth factor (EGF) (Skory et al., 2015). Erin R West. et al reduced the stiffness of alginate via radiation and chemical oxidation, and found that cell differentiation, steroid production and oocyte quality were superior when follicles were grown on low-stiffness alginates (West et al., 2007b). In addition to follicle culture, alginates are also applied in follicle cryopreservation. Vanacker et al. (2013) showed that human preantral follicles embedded in alginate hydrogel were successfully cryopreserved and that the viability of frozen follicles was comparable to that of fresh follicles in in vitro culture; moreover, ME2SO was found to be superior to ethylene glycol for cryopreservation of follicles embedded in alginate hydrogel (Camboni et al., 2013). Jamalzaei et al. (2020) compared the effects of three hydrogels (hyaluronic acid-alginate hydrogel, alginate and fibrin-alginate hydrogel) in preantral follicles cultures and demonstrated that hyaluronic acid-alginates hydrogel has more advantages over the other two in terms of follicle-secreted estradiol levels and the expression of differentiation genes .
Collagen is abundant in ovarian tissues; thus, collagen hydrogels are biocompatible and biodegradable (Berkholtz et al., 2006). Anthony Atala. et al encapsulated follicles in collagen hydrogels from 1% to 7% (w/v) and demonstrated that follicles had the best viability in 5% collagen hydrogels and 17 β-estradiol levels remained increasing during 20-days culture; therefore, 5% may be the optimal concentration for follicle growth (Joo et al., 2016). Gelatin is derived from collagen, Laronda et al. (2017) found that the shape of pores in gelatin hydrogels affected the survival of the follicle. 3D printing can control the advancing angle to change the shape of the pores, and advancing angles of 30° and 60° were more favorable for follicle survival than 90°.
Intrauterine Adhesion
Traumatic intrauterine adhesions were reported in 1894 (Fritsch, 1894). Asherman linked amenorrhea to IUA in 1948 by observing 29 patients, and in 1950 Asherman found that miscarriages and menstrual disorders also were caused by IUA (Asherman, 1950). Later, IUA with symptoms (e.g., amenorrhea, chronic pain, menstrual disorders) is called Asherman’s syndrome. Although some researchers agreed that Asherman’s syndrome should be distinguished from asymptomatic IUA (Dreisler and Kjer, 2019), the Asherman’s syndrome and IUA are interchangeable in the clinic. In addition to mechanical injuries (e.g., curettage, hysteroscopy), infection, genetic susceptibility and uterine malformation may also cause IUA (Yu et al., 2008). Histologically, fibrosis, which is not conducive to embryo implantation, occurs after the endometrium is damaged. Numerous fibroblasts replace endometrial mesenchymal cells, resulting in a decreased decidualization in response to hormonal stimulation (Hou et al., 2019). The demarcation between the functional and basal layers becomes unclear, and fibrotic endometrium is usually avascular and exhibits a reduced gland number (Foix et al., 1966).
Transcervical resection of adhesions (TCRA) is a primary choice for IUA (March 2011), but TCRA itself may cause damage to the uterine cavity and lead to re-adhesion; thus, adjuvant treatments are often used in conjunction with TCRA to prevent postoperative re-adhesion in clinical treatment. Oral administration of 9 mg/diet estrogen before TCRA and 10 mg/diet after TCRA improved pregnancy rates and menstrual cycles in patients with moderate or severe IUA (Liu AZ et al., 2016); however, estrogen therapy may increase the risk of cancer (Rossouw et al., 2002) and venous thrombosis (Bracamonte and Miller, 2001). Balloon dilatation after TCRA and early second-look hysteroscopy improved pregnancy outcomes (Sun et al., 2020), and the appropriate delay of balloon placement in the uterine cavity prevented re-adhesion but was not effective in severe IUA (Zhang et al., 2021a). The dilated balloon is a nonvariable sphere that is not able to be plasticized according to the shape of the uterine cavity, resulting in ineffective improvement of adhesions in the uterine horns (Sun et al., 2020). IVF after TCRA improved reproductive outcomes, but increased the miscarriage rate (Wang, Y et al., 2021).
The Application of Hydrogels Combined With Drugs in IUA
Drug and cell delivery are two main hydrogel applications in IUA (shown in Figure 2). Hydrogels maintain the shape of the uterus after being introduced into the uterine cavity, and hydrogels cling more completely to the uterine wall compared with balloons, supporting every part of the uterus and effectively preventing re-adhesion. Natural hydrogels, such as collagen and chitosan, have the ability to carry drugs. Leukemia inhibitory factor (LIF) is increased at the site of injury and may be associated with tissue regeneration. LIF/collagen hydrogel restored uterine structure and function of uterine in rat models with full-thickness injury (Xue et al., 2019). Basic fibroblast growth factor (bFGF) plays an important role in wound repair and angiogenesis. The bFGF/collagen hydrogel partially restored endometrial thickness and fertility in IUA infertile patients in a clinical trial (Jiang et al., 2019). Chitosan is a nature polysaccharide, The chitosan-heparin hydrogel carries stromal cell-derived factor-1α (SDF-1α) and releases it at the site of injury, accelerating the repair of the damaged endothelium (Qi et al., 2020). The exact biocompatibility and biodegradability are advantages for natural hydrogels, but achieving controlled drug release is difficult. Synthetic hydrogels are capable of controlling drug release by changing the type and ratio of materials. Keratinocyte growth factor (KGF) is a factor associated with epithelial repair. The binding of KGF to thermosensitive heparin-poloxamer (HP) hydrogels prolonged the retention of KGF in the uterus, and the KGF-HP hydrogel promoted the proliferation of epithelial cells (Xu HL et al., 2017). ε-polylysine (EPL) was added as a functional excipient to the HP hydrogel, and the release rate of KGF and the rheology of the hydrogel were easily changed by adjusting the concentration of EPL; moreover, angiogenesis was also significantly increased compared with the KGF-HP hydrogel group (Xu H et al., 2017). HP released β-estradiol (E2) at a constant rate in vivo, resulting in a significantly longer retention time of E2 in the uterine cavity compared to the E2 solution injection (Zhang S et al., 2017). The E2-HP hydrogel effectively improved the fertility rate, and the effect may be due to the expression of kisspeptin through the MAPK p38 and ERK1/2 signaling pathways (Zhang S et al., 2020). Wang B et al. (2021) synthesized injectable poly (ethylene glycol)-b-poly (l-phenylalanine) (PEBP)/poly (ethylene glycol) (PEG) hydrogel according to the π−π accumulation effect, and the ratio of methoxy-poly (ethylene glycol)-amine (MeO-PEG-NH2) and l-phenylalanine N-carboxy anhydride (L-Phe-NCA) in PEBP affected the release of L-Phe. At a ratio of two to eight (feed mass ratio of MeO-PEG-NH2 via L-Phe NCA), most L-Phe was released; however, considering the extended retention time of the PEBP/PEG hydrogel in the uterus, a ratio of three to seven is considered more appropriate. The L-Phe released by the PEBP/PEG hydrogel effectively reduced the area of fibrosis in the damaged endothelium in a rat model . The release of E2 in the injectable aloe/poloxamer (AP) hydrogel is independent of pH; thus, the E2-AP hydrogel is suitable for administration in the presence of cervical mucus. The E2-AP hydrogel restored the number of estrogen receptors and promoted endometrial regeneration (Yao et al., 2020).
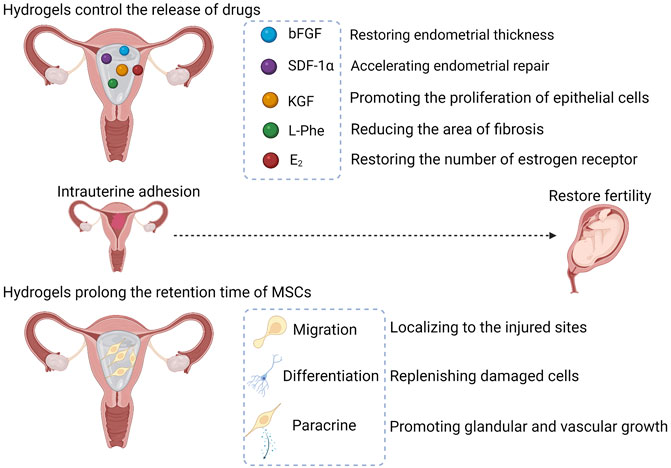
FIGURE 2. The applications of hydrogels in IUA. The swollen hydrogels form a physical barrier to prevent adhesions. Hydrogels control the release of drugs in the uterine cavity, and drugs exert different therapeutic effects. Hydrogels prolong the retention time of MSCs in the uterus, giving MSCs the opportunity to exert its therapeutic effects. The therapeutic effects are dependent on migration, differentiation, and paracrine function of MSCs. MSCs, mesenchymal stem cells; bFGF, basic fibroblast growth factor; SDF-1α, stromal cell-derived factor-1α; KGF, Keratinocyte growth factor; L-Phe, l-Phenylalanine; E2, β-estradiol.
The Application of Hydrogels Combined With MSCs in IUA
Hydrogels are a good three-dimensional medium to meet the growth needs of cells, allowing cells to be maintained at the site of injury for a long time. Proliferation and connectivity were not inhibited when cells were encapsulated in hydrogels (Sara et al., 2021; Kim Y et al., 2019), and hydrogels prolonged cell retention in the uterus compared with cell injection alone (Xiao et al., 2019). Therefore, the combination of hydrogels and MSCs is receiving increasing attention, and many animal and clinical trials have shown that the combination of both effectively treats IUA and restoresfertility.
Mesenchymal stem cells (MSCs) are capable of promoting the proliferation of glands and blood vessels, reducing the area of fibrosis and restoring fertility (Nagori et al., 2011; Santamaria et al., 2016; Wang et al., 2016; Zheng et al., 2020). This successful treatment stems from the low antigenicity, pleiotropic differentiation, strong migration, and paracrine signaling of MSCs. Low antigenicity is the key for clinical application, making allogeneic transplantation possible. MSCs express low levels of major histocompatibility complex class I (MHC I) molecules and hardly express major histocompatibility complex class II (MHC II) and costimulatory molecules (Kim K et al., 2019; Tsuchiya et al., 2019). A meta-analysis showed that allogeneic MSCs did not cause significant adverse effects (Lalu et al., 2012). Multidirectional differentiation is a characteristic of stem cells, and MSCs can be induced to differentiate into osteoblasts, chondrocytes, adipocytes and neurons in vitro (Ding et al., 2014; Yang et al., 2021). MSCs differentiate into epithelial and endothelial cells in the injured uterus, providing a cellular source for vascular and endometrial renewal (Shao et al., 2019; Zheng et al., 2020). MSCs are capable of accumulating in the injured uterus by tail vein injection or intraperitoneal injection in rats (Wang et al., 2016; Liu et al., 2018; Zheng et al., 2020), and migration allows MSCs to localize to the injured uterus for multidirectional differentiation and paracrine signaling.
Researchers believe that the repair function of MSCs is mainly derived from paracrine signaling (Bao and He, 2021; Zhou et al., 2022). MSCs secrete a variety of cytokines (e.g., VEGF-A, TGF-β1, FGF-2) to promote glandular and vascular growth and degradation of fibrous scars (Ding et al., 2014; Xin et al., 2019; Xu, L et al., 2017). Collagen increases the number of endometrial glands and endometrial thickness, reduces the area of fibrosis and improves pregnancy rates in a rat model (Ding et al., 2014; Liu et al., 2020). Compared with collagen alone, combining hUCMSCs more significantly increased the number of glands and reduced the area of fibrosis (Liu et al., 2020), and combining BMSCs was more effective in improving pregnancy rates, as well as in myometrium recovery and revascularization (Ding et al., 2014). These significant improvements may be due to the secretion of factors that promote vascular and glandular neogenesis by MSCs. Poly (glycerol sebacate) (PGS) restored soft tissue deformation without causing mechanical damage and porous PGS favored the attachment and growth of cells (Mi et al., 2017). Xiao et al. (2019) found that although no difference in pregnancy rates was observed between the PGS/BMSCs group and the collagen/BMSCs groups, higher concentrations of growth factors (e.g., bFGF, IGF-1, TGF-β1, VEGF) were detected in the endometrium treated with PGS/BMSCs than with collagen/BMSCs.
The beneficial effects of autologous stem cell therapy and hydrogel-encapsulated autologous cell therapy in clinical patients have been reported (shown in Table 1). Autologous cell therapy is effective in avoiding autoimmune system attacks, but the extraction of autologous cells is always invasive and difficult to extract in large quantities. hUCMSCs are derived from discarded umbilical cords and can be easily and abundantly extracted; Therefore, hUCMSCs are a potential alternative in allogeneic cell therapy. In clinical trials, synthetic hydrogels are rarely used in IUA treatment. researchers mostly chose natural hydrogels to encapsulate the cells, probably because the safety of synthetic hydrogels in clinical IUA treatment is still difficult to guarantee and ethical approval is difficult to pass. Future experiments on the application synthetic hydrogels in IUA should pay more attention to safety assessments.
Limitations and Future Prospects
Artificial Ovary
The development of artificial ovary can be divided into three stages, cellular experiment stage, animal experiment stage, the clinical experiment stage. The current development is in the first two stages, and these two stages is not mature enough to support the development of the clinic stage. Follicle isolation and in vitro culture has been established. Hydrogel 3D culture is significantly better than traditional 2D culture (See The Application of Hydrogels in Follicle Culture for details), maintenance of follicular morphology has been achieved in hydrogel 3D culture; however, ovulation in vitro 3D culture was rarely reported. Preantral follicles can develop into antral follicles in artificial ovary, but ovulation and natural-mating offspring is unsatisfactory (see Table 2). Ovulation is the key to pregnancy. Successful ovulation is difficult to achieve without the help of other cells of the ovary (Ren et al., 2016). In the ovarian cortex, stromal cells, vascular endothelial cells and epithelial cells were the top 3 cell types (approximately 83%, 10%, and 5%, respectively) (Wagner et al., 2020). Artificial ovary was created mainly to ensure that cancer patients preserve fertility after radiotherapy. In order to avoid reintroduction of tumor cells, most previous studies only added follicles in the artificial ovary, and removed the stromal cells, vascular endothelial cells, and epithelial cells (see Table 2). To make the artificial environment of the follicles closer to the physiological environment and avoid reintroduction of tumor cells, adding allogeneic stromal cells, vascular endothelial cells, and epithelial cells to artificial ovary may be alternative. Hydrogels exhibited the ability to prevent allotransplantation immune rejection in ovarian tissue transplantation (Day et al., 2019); thus, allogeneic transplantation of artificial ovary is not a pipe dream.
The presence of blood vessels in the graft is essential for the long-term survival of the artificial ovary. Neovascularization in artificial ovary has been reported (Telfer et al., 1990), adding pro-angiogenic factors to hydrogels is beneficial for neovascularization (Kniazeva et al., 2015; Rajabzadeh et al., 2015), but whether a complete blood circulation system can be developed is unclear due to short grafting time. How to prolong the degradation time is the problem to achieve long-term artificial ovary. Fibrin, collagen, gelatin, hyaluronic acid and alginate are common materials used to build artificial ovaries (see Table 2). Doxycycline, a matrix metalloproteinase (MMP) inhibitor, effectively prolonged the degradation of fibrin and collagen in vivo (Wassenaar et al., 2016). Integrating photocuring 3D bioprint and lyophilization techniques successfully decreased the degradation rate of gelatin and hyaluronic acid (Xia et al., 2018). Integrating alginate into N,O-18 carboxymethyl chitosan-aldehyde hyaluronic acid network significantly prolonged degradation time (Le et al., 2020). Collagen and alginate were added to fibrin hydrogel to slow down degradation, but these additions were detrimental to follicle survival and development (Kniazeva et al., 2015); thus, balancing hydrogel degradation and follicle survival is important in exploring long-term artificial ovaries.
Fresh follicles were used in majority of animal experiments, and cryopreserved follicles do not develop as well as fresh follicles in hydrogel (see Table 2). Cryopreserved follicles, however, are more readily available than fresh follicles in clinic; hence, how to build artificial ovary using cryopreserved follicles is worth studying. More research is needed on which hydrogel is best for follicle growth and development. Some additives may help cryopreserved follicle recovery. Cryopreservation has an inhibitory effect on the expression of estrogen receptor β (Depalo et al., 2009); thus, adding estrogen to hydrogels may help estrogen receptor recovery. FSH alleviated depletion of the resting follicle pool in cryopreserved ovary (von Schönfeldt et al., 2012); thus, Follicle stimulating hormone (FSH) is also a promising additive. Addressing the above problems may advance the development of artificial ovaries to the clinical stage.
3D Bioprinting in Hydrogel Synthesis
Although there are numerous studies of hydrogels in regenerative medicine, and most of them suggest good therapeutic effects, there are few hydrogel products on the market available for clinical applications. The biosafety of hydrogels in clinical applications cannot be fully guaranteed (Tonbul et al., 2014). In addition, hydrogels are synthesized in small batches at the pre-clinical stage, and to realize the clinical application of hydrogels, achieving large-batch production of high-quality hydrogels is an inevitable problem (Mandal et al., 2020).
3D bioprinting, which allows for controllable material structure through computer-controlled formation of continuous layers of material, has been used for the synthesis of cell-loaded hydrogels (Elkhoury et al., 2021). 3D bioprinting technology is developing rapidly. Compared with traditional inkjet 3D bioprinting, microextrusion 3D bioprinting is suitable for high viscosity materials and yields higher cell density (Billiet et al., 2014; Pedde et al., 2017). Stereolithography (SLA) 3D bioprinting has a higher resolution and higher speed, and cells are not damaged by external forces during the printing process, ensuring higher cell viability (Wang et al., 2018). 3D bioprinting enables mechanical controllability of the hydrogel synthesis process, a reduction in human errors and a more uniform distribution of cells in hydrogels; thus, 3D printing is a potential method for large-batch production of cell-loaded hydrogels and may advance the clinical application of hydrogels in POF and IUA therapy.
Conclusion
Suitable swelling and rheology as well as good biocompatibility and degradability are the key physicochemical properties of hydrogels for the application in POF and IUA treatment. Hydrogels are involved in POF treatment in four ways: transplantation of ovarian tissues, artificial ovary, stem cell delivery and drug delivery. 3D hydrogels mimic the physiological environment of the follicle and are more suitable for in vitro culture of follicles than the traditional 2D culture, providing a basis for the development of artificial ovaries. Hydrogels are involved in IUA treatment in two main ways: drug delivery and cell delivery. Hydrogels control the release of drugs and increase the retention time of drugs in the uterine cavity. MSCs themselves are capable of improving tissue repair through differentiation and paracrine secretion, and the therapeutic effect of MSCs combined with hydrogels may be more remarkable. The effect of hydrogels in POF and IUA treatment has been confirmed in many animal experiments, but there are still many stumbling blocks to the clinical application of hydrogels; thus, more safety evaluations and efficacy validations are needed.
Author Contributions
DZ: literature search, manuscript writing. CD: manuscript editing and revise. QZ: manuscript revise and project supervision. TD: project supervision and final approval.
Funding
The National Natural Science Foundation of China (82071663).
Conflict of Interest
The authors declare that the research was conducted in the absence of any commercial or financial relationships that could be construed as a potential conflict of interest.
Publisher’s Note
All claims expressed in this article are solely those of the authors and do not necessarily represent those of their affiliated organizations, or those of the publisher, the editors and the reviewers. Any product that may be evaluated in this article, or claim that may be made by its manufacturer, is not guaranteed or endorsed by the publisher.
References
Araki, K., Turner, A. P., Shaffer, V. O., Gangappa, S., Keller, S. A., Bachmann, M. F., et al. (2009). mTOR Regulates Memory CD8 T-Cell Differentiation. Nature 460 (7251), 108–112. doi:10.1038/nature08155
Asherman, J. G. (1950). Traumatic Intra-uterine Adhesions. BJOG:An Int. J. O&G 57 (6), 892–896. doi:10.1111/j.1471-0528.1950.tb06053.x
Bao, C., and He, C. (2021). The Role and Therapeutic Potential of MSC-Derived Exosomes in Osteoarthritis. Archives Biochem. Biophysics 710, 109002. doi:10.1016/j.abb.2021.109002
Bao, R., Tan, B., Liang, S., Zhang, N., Wang, W., and Liu, W. (2017). A π-π Conjugation-Containing Soft and Conductive Injectable Polymer Hydrogel Highly Efficiently Rebuilds Cardiac Function after Myocardial Infarction. Biomaterials 122, 63–71. doi:10.1016/j.biomaterials.2017.01.012
Beck-Peccoz, P., and Persani, L. (2006). Premature Ovarian Failure. Orphanet J. Rare Dis. 1, 9. doi:10.1186/1750-1172-1-9
Berkholtz, C. B., Lai, B. E., Woodruff, T. K., and Shea, L. D. (2006). Distribution of Extracellular Matrix Proteins Type I Collagen, Type IV Collagen, Fibronectin, and Laminin in Mouse Folliculogenesis. Histochem Cell Biol. 126 (5), 583–592. doi:10.1007/s00418-006-0194-1
Bhattarai, N., Gunn, J., and Zhang, M. (2010). Chitosan-based Hydrogels for Controlled, Localized Drug Delivery. Adv. Drug Deliv. Rev. 62 (1), 83–99. doi:10.1016/j.addr.2009.07.019
Billiet, T., Gevaert, E., De Schryver, T., Cornelissen, M., and Dubruel, P. (2014). The 3D Printing of Gelatin Methacrylamide Cell-Laden Tissue-Engineered Constructs with High Cell Viability. Biomaterials 35 (1), 49–62. doi:10.1016/j.biomaterials.2013.09.078
Bracamonte, M. P., and Miller, V. M. (2001). Vascular Effects of Estrogens: Arterial Protection versus Venous Thrombotic Risk. Trends Endocrinol. Metabolism 12 (5), 204–209. doi:10.1016/s1043-2760(01)00406-4
Camboni, A., Van Langendonckt, A., Donnez, J., Vanacker, J., Dolmans, M. M., and Amorim, C. A. (2013). Alginate Beads as a Tool to Handle, Cryopreserve and Culture Isolated Human Primordial/primary Follicles. Cryobiology 67 (1), 64–69. doi:10.1016/j.cryobiol.2013.05.002
Cao, Y., Sun, H., Zhu, H., Zhu, X., Tang, X., Yan, G., et al. (2018). Allogeneic Cell Therapy Using Umbilical Cord MSCs on Collagen Scaffolds for Patients with Recurrent Uterine Adhesion: a Phase I Clinical Trial. Stem Cell Res. Ther. 9 (1), 192. doi:10.1186/s13287-018-0904-3
Capella-Allouc, S., Morsad, F., Rongières-Bertrand, C., Taylor, S., and Fernandez, H. (1999). Hysteroscopic Treatment of Severe Asherman's Syndrome and Subsequent Fertility. Hum. Reprod. 14 (5), 1230–1233. doi:10.1093/humrep/14.5.1230
Chi, Y., He, P., Lei, L., Lan, Y., Hu, J., Meng, Y., et al. (2018). Transdermal Estrogen Gel and Oral Aspirin Combination Therapy Improves Fertility Prognosis via the Promotion of Endometrial Receptivity in Moderate to Severe Intrauterine Adhesion. Mol. Med. Rep. 17 (5), 6337–6344. doi:10.3892/mmr.2018.8685
Chiti, M. C., Dolmans, M. M., Orellana, R., Soares, M., Paulini, F., Donnez, J., et al. (2016). Influence of Follicle Stage on Artificial Ovary Outcome Using Fibrin as a Matrix. Hum. Reprod. 31 (2), dev299–435. doi:10.1093/humrep/dev299
Choi, J. K., Agarwal, P., and He, X. (2013). In Vitro culture of Early Secondary Preantral Follicles in Hanging Drop of Ovarian Cell-Conditioned Medium to Obtain MII Oocytes from Outbred Deer Mice. Tissue Eng. Part A 19 (23-24), 2626–2637. doi:10.1089/ten.TEA.2013.0055
Cui, X., Lee, J. J. L., and Chen, W. N. (2019). Eco-friendly and Biodegradable Cellulose Hydrogels Produced from Low Cost Okara: towards Non-toxic Flexible Electronics. Sci. Rep. 9 (1), 18166. doi:10.1038/s41598-019-54638-5
Dawn, A., and Kumari, H. (2018). Low Molecular Weight Supramolecular Gels under Shear: Rheology as the Tool for Elucidating Structure-Function Correlation. Chem. Eur. J. 24 (4), 762–776. doi:10.1002/chem.201703374
Day, J. R., David, A., Barbosa, M. G. d. M., Brunette, M. A., Cascalho, M., and Shikanov, A. (2019). Encapsulation of Ovarian Allograft Precludes Immune Rejection and Promotes Restoration of Endocrine Function in Immune-Competent Ovariectomized Mice. Sci. Rep. 9 (1), 16614. doi:10.1038/s41598-019-53075-8
Demeestere, I., Brice, P., Peccatori, F. A., Kentos, A., Dupuis, J., Zachee, P., et al. (2016). No Evidence for the Benefit of Gonadotropin-Releasing Hormone Agonist in Preserving Ovarian Function and Fertility in Lymphoma Survivors Treated with Chemotherapy: Final Long-Term Report of a Prospective Randomized Trial. J. Clin. Oncol. 34 (22), 2568–2574. doi:10.1200/JCO.2015.65.8864
Deng, T., He, J., Yao, Q., Wu, L., Xue, L., Wu, M., et al. (2021). Human Umbilical Cord Mesenchymal Stem Cells Improve Ovarian Function in Chemotherapy-Induced Premature Ovarian Failure Mice through Inhibiting Apoptosis and Inflammation via a Paracrine Mechanism. Reprod. Sci. 28 (6), 1718–1732. doi:10.1007/s43032-021-00499-1
Depalo, R., Lorusso, F., Bettocchi, S., Selvaggi, L., Cavallini, A., Valentini, A. M., et al. (2009). Assessment of Estrogen Receptors and Apoptotic Factors in Cryopreserved Human Ovarian Cortex. Syst. Biol. Reproductive Med. 55 (5-6), 236–243. doi:10.3109/19396360903046761
Desai, N., Abdelhafez, F., Calabro, A., and Falcone, T. (2012). Three Dimensional Culture of Fresh and Vitrified Mouse Pre-antral Follicles in a Hyaluronan-Based Hydrogel: a Preliminary Investigation of a Novel Biomaterial for In Vitro Follicle Maturation. Reprod. Biol. Endocrinol. 10 (1), 29. doi:10.1186/1477-7827-10-29
Ding, L., Li, X. a., Sun, H., Su, J., Lin, N., Péault, B., et al. (2014). Transplantation of Bone Marrow Mesenchymal Stem Cells on Collagen Scaffolds for the Functional Regeneration of Injured Rat Uterus. Biomaterials 35 (18), 4888–4900. doi:10.1016/j.biomaterials.2014.02.046
Dou, X., Sun, Y., Li, J., Zhang, J., Hao, D., Liu, W., et al. (2017). Short-term Rapamycin Treatment Increases Ovarian Lifespan in Young and Middle-Aged Female Mice. Aging Cell 16 (4), 825–836. doi:10.1111/acel.12617
Dreisler, E., and Kjer, J. J. (2019). Asherman's Syndrome: Current Perspectives on Diagnosis and Management. Int. J. Womens Health 11, 191–198. doi:10.2147/IJWH.S165474
Duval, D., Demangel, C., Miossec, S., and Geahel, I. (1992). Role of Metabolic Waste Products in the Control of Cell Proliferation and Antibody Production by Mouse Hybridoma Cells. Hybridoma 11 (3), 311–322. doi:10.1089/hyb.1992.11.311
Elkhoury, K., Morsink, M., Sanchez-Gonzalez, L., Kahn, C., Tamayol, A., and Arab-Tehrany, E. (2021). Biofabrication of Natural Hydrogels for Cardiac, Neural, and Bone Tissue Engineering Applications. Bioact. Mater. 6 (11), 3904–3923. doi:10.1016/j.bioactmat.2021.03.040
Fabbri, R., Seracchioli, R., Vicenti, R., Paradisi, R., Rossi, S., De Meis, L., et al. (2019). Successful Achievement after Heterotopic Transplantations of Long-Term Stored Ovarian Tissue in Hodgkin's Lymphoma Survivor. Gynecol. Endocrinol. 35 (6), 470–472. doi:10.1080/09513590.2018.1549218
Foix, A., Bruno, R. O., Davison, T., and Lema, B. (1966). The Pathology of Postcurettage Intrauterine Adhesions. Am. J. Obstetrics Gynecol. 96 (7), 1027–1033. doi:10.1016/0002-9378(66)90452-2
Fritsch, H. (1894). Ein Fall von volligem Schwund der Gebarmutterhohle nach Auskratzung. Zentralbl Gynaekol 18, 1337–1342.
Ganguly, S., Maity, T., Mondal, S., Das, P., and Das, N. C. (2017). Starch Functionalized Biodegradable Semi-IPN as a pH-Tunable Controlled Release Platform for Memantine. Int. J. Biol. Macromol. 95, 185–198. doi:10.1016/j.ijbiomac.2016.11.055
Ganguly, S., and Margel, S. (2021). Design of Magnetic Hydrogels for Hyperthermia and Drug Delivery. Polymers 13 (23), 4259. doi:10.3390/polym13234259
Gao, J.-M., Yan, J., Li, R., Li, M., Yan, L.-Y., Wang, T.-R., et al. (2013). Improvement in the Quality of Heterotopic Allotransplanted Mouse Ovarian Tissues with Basic Fibroblast Growth Factor and Fibrin Hydrogel. Hum. Reprod. 28 (10), 2784–2793. doi:10.1093/humrep/det296
Goswami, D., and Conway, G. S. (2005). Premature Ovarian Failure. Hum. Reprod. Update 11 (4), 391–410. doi:10.1093/humupd/dmi012
Grynberg, M., Jacquesson, L., and Sifer, C. (2020). In Vitro maturation of Oocytes for Preserving Fertility in Autoimmune Premature Ovarian Insufficiency. Fertil. Steril. 114 (4), 848–853. doi:10.1016/j.fertnstert.2020.04.049
Hasani-Sadrabadi, M. M., Sarrion, P., Pouraghaei, S., Chau, Y., Ansari, S., Li, S., et al. (2020). An Engineered Cell-Laden Adhesive Hydrogel Promotes Craniofacial Bone Tissue Regeneration in Rats. Sci. Transl. Med. 12 (534), eaay6853. doi:10.1126/scitranslmed.aay6853
Hou, X., Liu, Y., Streuli, I., Dällenbach, P., Dubuisson, J., Ansaldi, Y., et al. (2019). Endometrial Regeneration in Asherman's Syndrome: Clinical and Translational Evidence of Stem Cell Therapies. Curr. Stem Cell Res. Ther. 14 (6), 454–459. doi:10.2174/1574888X14666190213100528
Huang, J., Zhang, W., Yu, J., Gou, Y., Liu, N., Wang, T., et al. (2022). Human Amniotic Mesenchymal Stem Cells Combined with PPCNg Facilitate Injured Endometrial Regeneration. Stem Cell Res. Ther. 13 (1), 17. doi:10.1186/s13287-021-02682-2
Huang, Y., Ma, Z., Kuang, X., Zhang, Q., Li, H., and Lai, D. (2021). Sodium Alginate-Bioglass-Encapsulated hAECs Restore Ovarian Function in Premature Ovarian Failure by Stimulating Angiogenic Factor Secretion. Stem Cell Res. Ther. 12 (1), 223. doi:10.1186/s13287-021-02280-2
Ishizuka, B., Furuya, M., Kimura, M., Kamioka, E., and Kawamura, K. (2021). Live Birth Rate in Patients with Premature Ovarian Insufficiency during Long-Term Follow-Up under Hormone Replacement with or without Ovarian Stimulation. Front. Endocrinol. 12, 795724. doi:10.3389/fendo.2021.795724
Jackson, K. S., Inoue, K., Davis, D. A., Hilliard, T. S., and Burdette, J. E. (2009). Three-dimensional Ovarian Organ Culture as a Tool to Study Normal Ovarian Surface Epithelial Wound Repair. Endocrinology 150 (8), 3921–3926. doi:10.1210/en.2008-1674
Jadoul, P., Guilmain, A., Squifflet, J., Luyckx, M., Votino, R., Wyns, C., et al. (2017). Efficacy of Ovarian Tissue Cryopreservation for Fertility Preservation: Lessons Learned from 545 Cases. Hum. Reprod. 32 (5), 1046–1054. doi:10.1093/humrep/dex040
Jamalzaei, P., Rezazadeh Valojerdi, M., Montazeri, L., and Baharvand, H. (2020). Applicability of Hyaluronic Acid-Alginate Hydrogel and Ovarian Cells for In Vitro Development of Mouse Preantral Follicles. Cell J. 22 (Suppl. 1), 49–60. doi:10.22074/cellj.2020.6925
Jeon, O., Bouhadir, K. H., Mansour, J. M., and Alsberg, E. (2009). Photocrosslinked Alginate Hydrogels with Tunable Biodegradation Rates and Mechanical Properties. Biomaterials 30 (14), 2724–2734. doi:10.1016/j.biomaterials.2009.01.034
Jiang, G., Sun, J., and Ding, F. (2014). PEG-g-chitosan Thermosensitive Hydrogel for Implant Drug Delivery: Cytotoxicity,in Vivodegradation and Drug Release. J. Biomaterials Sci. Polym. Ed. 25 (3), 241–256. doi:10.1080/09205063.2013.851542
Jiang, P., Tang, X., Wang, H., Dai, C., Su, J., Zhu, H., et al. (2019). Collagen-binding Basic Fibroblast Growth Factor Improves Functional Remodeling of Scarred Endometrium in Uterine Infertile Women: a Pilot Study. Sci. China Life Sci. 62 (12), 1617–1629. doi:10.1007/s11427-018-9520-2
Joo, S., Oh, S.-H., Sittadjody, S., Opara, E. C., Jackson, J. D., Lee, S. J., et al. (2016). The Effect of Collagen Hydrogel on 3D Culture of Ovarian Follicles. Biomed. Mat. 11 (6), 065009. doi:10.1088/1748-6041/11/6/065009
Kang, S.-M., Lee, J.-H., Huh, Y. S., and Takayama, S. (2021). Alginate Microencapsulation for Three-Dimensional In Vitro Cell Culture. ACS Biomater. Sci. Eng. 7 (7), 2864–2879. doi:10.1021/acsbiomaterials.0c00457
Kim, J., Perez, A. S., Claflin, J., David, A., Zhou, H., and Shikanov, A. (2016). Synthetic Hydrogel Supports the Function and Regeneration of Artificial Ovarian Tissue in Mice. NPJ Regen. Med. 1, 16010. doi:10.1038/npjregenmed.2016.10
Kim, K., Bou-Ghannam, S., Thorp, H., Grainger, D. W., and Okano, T. (2019). Human Mesenchymal Stem Cell Sheets in Xeno-free Media for Possible Allogenic Applications. Sci. Rep. 9 (1), 1–12. doi:10.1038/s41598-019-50430-7
Kim, Y. Y., Park, K.-H., Kim, Y. J., Kim, M. S., Liu, H. C., Rosenwaks, Z., et al. (2019). Synergistic Regenerative Effects of Functionalized Endometrial Stromal Cells with Hyaluronic Acid Hydrogel in a Murine Model of Uterine Damage. Acta Biomater. 89, 139–151. doi:10.1016/j.actbio.2019.03.032
Kniazeva, E., Hardy, A. N., Boukaidi, S. A., Woodruff, T. K., Jeruss, J. S., and Shea, L. D. (2015). Primordial Follicle Transplantation within Designer Biomaterial Grafts Produce Live Births in a Mouse Infertility Model. Sci. Rep. 5, 17709. doi:10.1038/srep17709
Kong, H.-J., Lee, K. Y., and Mooney, D. J. (2002). Decoupling the Dependence of Rheological/mechanical Properties of Hydrogels from Solids Concentration. Polymer 43 (23), 6239–6246. doi:10.1088/1748-605X/ac4d7b10.1016/s0032-3861(02)00559-1
Lalu, M. M., McIntyre, L., Pugliese, C., Fergusson, D., Winston, B. W., Marshall, J. C., et al. (2012). Safety of Cell Therapy with Mesenchymal Stromal Cells (SafeCell): A Systematic Review and Meta-Analysis of Clinical Trials. PloS one 7 (10), e47559. doi:10.1371/journal.pone.0047559
Laronda, M. M., Rutz, A. L., Xiao, S., Whelan, K. A., Duncan, F. E., Roth, E. W., et al. (2017). A Bioprosthetic Ovary Created Using 3D Printed Microporous Scaffolds Restores Ovarian Function in Sterilized Mice. Nat. Commun. 8, 15261. doi:10.1038/ncomms15261
Le, A. N. M., Nguyen, T. T., Ly, K. L., Luong, T. D., Ho, M. H., Minh-Phuong Tran, N., et al. (2020). Modulating Biodegradation and Biocompatibility of In Situ Crosslinked Hydrogel by the Integration of Alginate into N, O-Carboxylmethyl Chitosan–Aldehyde Hyaluronic Acid Network. Polym. Degrad. Stab. 180, 109270. doi:10.1016/j.polymdegradstab.2020.109270
Lee, K. Y., and Mooney, D. J. (2012). Alginate: Properties and Biomedical Applications. Prog. Polym. Sci. 37 (1), 106–126. doi:10.1016/j.progpolymsci.2011.06.003
Li, Y., Rodrigues, J., and Tomás, H. (2012). Injectable and Biodegradable Hydrogels: Gelation, Biodegradation and Biomedical Applications. Chem. Soc. Rev. 41 (6), 2193–2221. doi:10.1039/c1cs15203c
Li, Z., and Guan, J. (2011). Thermosensitive Hydrogels for Drug Delivery. Expert Opin. Drug Deliv. 8 (8), 991–1007. doi:10.1517/17425247.2011.581656
Ling, L., Feng, X., Wei, T., Wang, Y., Wang, Y., Zhang, W., et al. (2017). Effects of Low-Intensity Pulsed Ultrasound (LIPUS)-pretreated Human Amnion-Derived Mesenchymal Stem Cell (hAD-MSC) Transplantation on Primary Ovarian Insufficiency in Rats. Stem Cell Res. Ther. 8 (1), 283. doi:10.1186/s13287-017-0739-3
Liu Az, A. Z., Zhao, H. G., Gao, Y., Liu, M., and Guo, B. Z. (2016). Effectiveness of Estrogen Treatment before Transcervical Resection of Adhesions on Moderate and Severe Uterine Adhesion Patients. Gynecol. Endocrinol. 32 (9), 737–740. doi:10.3109/09513590.2016.1160375
Liu S, S., Zhou, J., Zhang, X., Liu, Y., Chen, J., Hu, B., et al. (2016). Strategies to Optimize Adult Stem Cell Therapy for Tissue Regeneration. Int. J. Mol. Sci. 17 (6), 982. doi:10.3390/ijms17060982
Liu, Y., Cai, J., Luo, X., Wen, H., and Luo, Y. (2020). Collagen Scaffold with Human Umbilical Cord Mesenchymal Stem Cells Remarkably Improves Intrauterine Adhesions in a Rat Model. Gynecol. Obstet. Invest. 85 (3), 267–276. doi:10.1159/000505691
Liu, Y., Tal, R., Pluchino, N., Mamillapalli, R., and Taylor, H. S. (2018). Systemic Administration of Bone Marrow-Derived Cells Leads to Better Uterine Engraftment Than Use of Uterine-Derived Cells or Local Injection. J. Cell Mol. Med. 22 (1), 67–76. doi:10.1111/jcmm.13294
Lucie, C.-D., Elisabeth, R., Chloé, D., Volcy, S., Claude, H., Clément, J., et al. (2021). Successful Live Birth after In Vitro Maturation Treatment in a Patient with Autoimmune Premature Ovarian Failure: a Case Report and Review of the Literature. Gynecol. Endocrinol. 37 (12), 1138–1142. doi:10.1080/09513590.2021.1928065
Lv, H., Wu, B., Song, J., Wu, W., Cai, W., and Xu, J. (2021). Hydrogel, a Novel Therapeutic and Delivery Strategy, in the Treatment of Intrauterine Adhesions. J. Mater Chem. B 9 (33), 6536–6552. doi:10.1039/d1tb01005k
Mandal, A., Clegg, J. R., Anselmo, A. C., and Mitragotri, S. (2020). Hydrogels in the Clinic. Bioeng. Transl. Med. 5 (2), e10158. doi:10.1002/btm2.10158
Mao, X., Tao, Y., Cai, R., Zhang, J., Gao, H., Chen, Q., et al. (2020). Cross-linked Hyaluronan Gel to Improve Pregnancy Rate of Women Patients with Moderate to Severe Intrauterine Adhesion Treated with IVF: a Randomized Controlled Trial. Arch. Gynecol. Obstet. 301 (1), 199–205. doi:10.1007/s00404-019-05368-6
March, C. M. (2011). Management of Asherman's Syndrome. Reprod. Biomed. Online 23 (1), 63–76. doi:10.1016/j.rbmo.2010.11.018
Mehdinia, Z., Ashrafi, M., Fathi, R., Taheri, P., and Valojerdi, M. R. (2020). Restoration of Estrous Cycles by Co-transplantation of Mouse Ovarian Tissue with MSCs. Cell Tissue Res. 381 (3), 509–525. doi:10.1007/s00441-020-03204-x
Mettler, L., Hucke, J., Bojahr, B., Tinneberg, H. R., Leyland, N., and Avelar, R. (2008). A Safety and Efficacy Study of a Resorbable Hydrogel for Reduction of Post-operative Adhesions Following Myomectomy. Hum. Reprod. 23 (5), 1093–1100. doi:10.1093/humrep/den080
Mi, H., Jing, X., Napiwocki, B. N., Hagerty, B. S., Chen, G., and Turng, L. (2017). Biocompatible, Degradable Thermoplastic Polyurethane Based on Polycaprolactone-Block-Polytetrahydrofuran-Block-Polycaprolactone Copolymers for Soft Tissue Engineering. J. Mater Chem. B 5 (22), 4137–4151. doi:10.1039/C7TB00419B
Mohapatra, S., Mirza, M. A., Hilles, A. R., Zakir, F., Gomes, A. C., Ansari, M. J., et al. (2021). Biomedical Application, Patent Repository, Clinical Trial and Regulatory Updates on Hydrogel: An Extensive Review. Gels 7 (4), 207. doi:10.3390/gels7040207
Müller, S. A., Weis, C., Odermatt, E. K., Knaebel, H. P., and Wente, M. N. (2011). A Hydrogel for Adhesion Prevention: Characterization and Efficacy Study in a Rabbit Uterus Model. Eur. J. Obstet. Gynecol. Reprod. Biol. 158 (1), 67–71. doi:10.1016/j.ejogrb.2010.11.008
Murdan, S. (2003). Electro-responsive Drug Delivery from Hydrogels. J. Control Release 92 (1-2), 1–17. doi:10.1016/s0168-3659(03)00303-1
Naahidi, S., Jafari, M., Edalat, F., Raymond, K., Khademhosseini, A., and Chen, P. (2013). Biocompatibility of Engineered Nanoparticles for Drug Delivery. J. Control Release 166 (2), 182–194. doi:10.1016/j.jconrel.2012.12.013
Nagori, C. B., Panchal, S. Y., and Patel, H. (2011). Endometrial Regeneration Using Autologous Adult Stem Cells Followed by Conception by In Vitro Fertilization in a Patient of Severe Asherman's Syndrome. J. Hum. Reprod. Sci. 4 (1), 43–48. doi:10.4103/0974-1208.82360
Owens, D. E., and Peppas, N. A. (2006). Opsonization, Biodistribution, and Pharmacokinetics of Polymeric Nanoparticles. Int. J. Pharm. 307 (1), 93–102. doi:10.1016/j.ijpharm.2005.10.010
Paulini, F., Vilela, J. M., Chiti, M. C., Donnez, J., Jadoul, P., Dolmans, M. M., et al. (2016). Survival and Growth of Human Preantral Follicles after Cryopreservation of Ovarian Tissue, Follicle Isolation and Short-Term Xenografting. Reprod. Biomed. Online 33 (3), 425–432. doi:10.1016/j.rbmo.2016.05.003
Pedde, R. D., Mirani, B., Navaei, A., Styan, T., Wong, S., Mehrali, M., et al. (2017). Emerging Biofabrication Strategies for Engineering Complex Tissue Constructs. Adv. Mater 29 (19), 1606061. doi:10.1002/adma.201606061
Qi, W., Xu, L., Zhao, S., Zheng, J., Tian, Y., Qi, X., et al. (2020). Controlled Releasing of SDF-1α in Chitosan-Heparin Hydrogel for Endometrium Injury Healing in Rat Model. Int. J. Biol. Macromol. 143, 163–172. doi:10.1016/j.ijbiomac.2019.11.184
Rajabzadeh, A., Jahanpeyma, F., Talebi, A., Moradi, F., Hamidieh, A. A., and Eimani, H. (2020). Fibrin Scaffold Incorporating Platelet Lysate Enhance Follicle Survival and Angiogenesis in Cryopreserved Preantral Follicle Transplantation. Galen. Med. J. 9, e1558. doi:10.31661/gmj.v9i0.1558
Rajabzadeh, A. R., Eimani, H., Mohseni Koochesfahani, H., Shahvardi, A. H., and Fathi, R. (2015). Morphological Study of Isolated Ovarian Preantral Follicles Using Fibrin Gel Plus Platelet Lysate after Subcutaneous Transplantation. Cell J. 17 (1), 145–152. doi:10.22074/cellj.2015.521
Reddy, P., Liu, L., Adhikari, D., Jagarlamudi, K., Rajareddy, S., Shen, Y., et al. (2008). Oocyte-specific Deletion of Pten Causes Premature Activation of the Primordial Follicle Pool. Science 319 (5863), 611–613. doi:10.1126/science.1152257
Ren, Y. A., Liu, Z., Mullany, L. K., Fan, C. M., and Richards, J. S. (2016). Growth Arrest Specific-1 (GAS1) Is a C/EBP Target Gene that Functions in Ovulation and Corpus Luteum Formation in Mice. Biol. Reprod. 94 (2), 44. doi:10.1095/biolreprod.115.133058
Rios, P. D., Kniazeva, E., Lee, H. C., Xiao, S., Oakes, R. S., Saito, E., et al. (2018). Retrievable Hydrogels for Ovarian Follicle Transplantation and Oocyte Collection. Biotechnol. Bioeng. 115 (8), 2075–2086. doi:10.1002/bit.26721
Rodell, C. B., Rai, R., Faubel, S., Burdick, J. A., and Soranno, D. E. (2015). Local Immunotherapy via Delivery of Interleukin-10 and Transforming Growth Factor β Antagonist for Treatment of Chronic Kidney Disease. J. Control Release 206, 131–139. doi:10.1016/j.jconrel.2015.03.025
Rodgers, R. J., Irving-Rodgers, H. F., and Russell, D. L. (2003). Extracellular Matrix of the Developing Ovarian Follicle. Reproduction 126 (4), 415–424. doi:10.1530/rep.0.1260415
Rossouw, J. E., Anderson, G. L., Prentice, R. L., LaCroix, A. Z., Kooperberg, C., Stefanick, M. L., et al. (2002). Writing Group for the Women's Health Initiative Investigators. Risks and Benefits of Estrogen Plus Progestin in Healthy Postmenopausal Women: Principal Results from the Women's Health Initiative Randomized Controlled Trial. JAMA 288 (3), 321–333. doi:10.1001/jama.288.3.321
Santamaria, X., Cabanillas, S., Cervelló, I., Arbona, C., Raga, F., Ferro, J., et al. (2016). Autologous Cell Therapy with CD133+ Bone Marrow-Derived Stem Cells for Refractory Asherman's Syndrome and Endometrial Atrophy: a Pilot Cohort Study. Hum. Reprod. 31 (5), 1087–1096. doi:10.1093/humrep/dew042
Sara, L.-M., Hannes, C., Lucía, de M.-G, L., Amparo, F., Alfredo, T. N., Ana, D., et al. (2021). A Natural Xenogeneic Endometrial Extracellular Matrix Hydrogel toward Improving Current Human In Vitro Models and Future In Vivo Applications. Front. Bioeng. Biotechnol. 9, 639688. doi:10.3389/fbioe.2021.639688
Schover, L. R. (2008). Premature Ovarian Failure and its Consequences: Vasomotor Symptoms, Sexuality, and Fertility. J. Clin. Oncol. 26 (5), 753–758. doi:10.1200/JCO.2007.14.1655
Shao, X., Ai, G., Wang, L., Qin, J., Li, Y., Jiang, H., et al. (2019). Adipose-derived Stem Cells Transplantation Improves Endometrial Injury Repair. Zygote 27 (6), 367–374. doi:10.1017/S096719941900042X
Shi, Z., Li, X., Wei, M., Chen, P., Zhang, T., Ling, X., et al. (2021). Receptor Tyrosine Kinases-Instructed Release of its Inhibitor from Hydrogel to Delay Ovarian Aging. Biomaterials 269, 120536. doi:10.1016/j.biomaterials.2020.120536
Shikanov, A., Xu, M., Woodruff, T. K., and Shea, L. D. (2009). Interpenetrating Fibrin-Alginate Matrices for In Vitro Ovarian Follicle Development. Biomaterials 30 (29), 5476–5485. doi:10.1016/j.biomaterials.2009.06.054
Singh, N., Mohanty, S., Seth, T., Shankar, M., Bhaskaran, S., and Dharmendra, S. (2014). Autologous Stem Cell Transplantation in Refractory Asherman's Syndrome: A Novel Cell Based Therapy. J. Hum. Reprod. Sci. 7 (2), 93–98. doi:10.4103/0974-1208.138864
Skory, R. M., Xu, Y., Shea, L. D., and Woodruff, T. K. (2015). Engineering the Ovarian Cycle Using In Vitro Follicle Culture. Hum. Reprod. 30 (6), 1386–1395. doi:10.1093/humrep/dev052
Slaughter, B. V., Khurshid, S. S., Fisher, O. Z., Khademhosseini, A., and Peppas, N. A. (2009). Hydrogels in Regenerative Medicine. Adv. Mater 21 (32-33), 3307–3329. doi:10.1002/adma.200802106
Smith, R. M., Shikanov, A., Kniazeva, E., Ramadurai, D., Woodruff, T. K., and Shea, L. D. (2014). Fibrin-mediated Delivery of an Ovarian Follicle Pool in a Mouse Model of Infertility. Tissue Eng. Part A 20 (21-22), 3021–3030. doi:10.1089/ten.TEA.2013.0675
Soranno, D. E., Rodell, C. B., Altmann, C., Duplantis, J., Andres-Hernando, A., Burdick, J. A., et al. (2016). Delivery of Interleukin-10 via Injectable Hydrogels Improves Renal Outcomes and Reduces Systemic Inflammation Following Ischemic Acute Kidney Injury in Mice. Am. J. Physiol. Ren. Physiol. 311 (2), F362–F372. doi:10.1152/ajprenal.00579.2015
Sullivan, S. D., Sarrel, P. M., and Nelson, L. M. (2016). Hormone Replacement Therapy in Young Women with Primary Ovarian Insufficiency and Early Menopause. Fertil. Steril. 106 (7), 1588–1599. doi:10.1016/j.fertnstert.2016.09.046
Sun, J., Shi, C., Liang, Y., Niu, J., Guo, S., and Cheng, Z. (2020). Effects of Early Second-Look Hysteroscopy Combined with Intrauterine Balloon Dilatation on Reproductive Outcomes for Women with Intrauterine Adhesions. Int. J. Gynaecol. Obstet. 149 (2), 192–196. doi:10.1002/ijgo.13108
Sutthasupa, S., Padungkit, C., and Suriyong, S. (2021). Colorimetric Ammonia (NH3) Sensor Based on an Alginate-Methylcellulose Blend Hydrogel and the Potential Opportunity for the Development of a Minced Pork Spoilage Indicator. Food Chem. 362, 130151. doi:10.1016/j.foodchem.2021.130151
Tabata, Y., Nagano, A., and Ikada, Y. (1999). Biodegradation of Hydrogel Carrier Incorporating Fibroblast Growth Factor. Tissue Eng. 5 (2), 127–138. doi:10.1089/ten.1999.5.127
Tanaka, A., Nakamura, H., Tabata, Y., Fujimori, Y., Kumasawa, K., and Kimura, T. (2018). Effect of Sustained Release of Basic Fibroblast Growth Factor Using Biodegradable Gelatin Hydrogels on Frozen-Thawed Human Ovarian Tissue in a Xenograft Model. J. Obstet. Gynaecol. Res. 44 (10), 1947–1955. doi:10.1111/jog.13726
Tavana, S., Valojerdi, M. R., Azarnia, M., and Shahverdi, A. (2016). Restoration of Ovarian Tissue Function and Estrous Cycle in Rat after Autotransplantation Using Hyaluronic Acid Hydrogel Scaffold Containing VEGF and bFGF. Growth factors. 34 (3-4), 97–106. doi:10.1080/08977194.2016.1194835
Telfer, E., Torrance, C., and Gosden, R. G. (1990). Morphological Study of Cultured Preantral Ovarian Follicles of Mice after Transplantation under the Kidney Capsule. J. Reprod. Fertil. 89 (2), 565–571. doi:10.1530/jrf.0.0890565
Thömmes, K., Lennartsson, J., Carlberg, M., and Rönnstrand, L. (1999). Identification of Tyr-703 and Tyr-936 as the Primary Association Sites for Grb2 and Grb7 in the C-Kit/stem Cell Factor Receptor. Biochem. J. 341 (Pt 1Pt 1), 211–216.
Tibbitt, M. W., and Anseth, K. S. (2009). Hydrogels as Extracellular Matrix Mimics for 3D Cell Culture. Biotechnol. Bioeng. 103 (4), 655–663. doi:10.1002/bit.22361
Tonbul, M., Adas, M., Bekmezci, T., and Kara, A. D. (2014). Intra-articular Polyacrylamide Hydrogel Injections Are Not Innocent. Case Rep. Orthop. 2014, 150709. doi:10.1155/2014/150709
Tsuchiya, A., Takeuchi, S., Watanabe, T., Yoshida, T., Nojiri, S., Ogawa, M., et al. (2019). Mesenchymal Stem Cell Therapies for Liver Cirrhosis: MSCs as "conducting Cells" for Improvement of Liver Fibrosis and Regeneration. Inflamm. Regen. 39 (1), 1–6. doi:10.1186/s41232-019-0107-z
van Kasteren, Y. M., and Schoemaker, J. (1999). Premature Ovarian Failure: a Systematic Review on Therapeutic Interventions to Restore Ovarian Function and Achieve Pregnancy. Hum. Reprod. Update 5 (5), 483–492. doi:10.1093/humupd/5.5.483
Vanacker, J., Dolmans, M.-M., Luyckx, V., Donnez, J., and Amorim, C. A. (2014). First Transplantation of Isolated Murine Follicles in Alginate. Regen. Med. 9 (5), 609–619. doi:10.2217/rme.14.33
Vanacker, J., Luyckx, V., Amorim, C., Dolmans, M. M., Van Langendonckt, A., Donnez, J., et al. (2013). Should We Isolate Human Preantral Follicles before or after Cryopreservation of Ovarian Tissue? Fertil. Steril. 99 (5), 1363–1368. e2. doi:10.1016/j.fertnstert.2012.12.016
von Schönfeldt, V., Chandolia, R., Ochsenkühn, R., Nieschlag, E., Kiesel, L., and Sonntag, B. (2012). FSH Prevents Depletion of the Resting Follicle Pool by Promoting Follicular Number and Morphology in Fresh and Cryopreserved Primate Ovarian Tissues Following Xenografting. Reprod. Biol. Endocrinol. 10, 98. doi:10.1186/1477-7827-10-98
von Wolff, M., Donnez, J., Hovatta, O., Keros, V., Maltaris, T., Montag, M., et al. (2009). Cryopreservation and Autotransplantation of Human Ovarian Tissue Prior to Cytotoxic Therapy-Aa Technique in its Infancy but Already Successful in Fertility Preservation. Eur. J. Cancer 45 (9), 1547–1553. doi:10.1016/j.ejca.2009.01.029
Wagner, M., Yoshihara, M., Douagi, I., Damdimopoulos, A., Panula, S., Petropoulos, S., et al. (2020). Single-cell Analysis of Human Ovarian Cortex Identifies Distinct Cell Populations but No Oogonial Stem Cells. Nat. Commun. 11 (1), 1147. doi:10.1038/s41467-020-14936-3
Wang B, B., Feng, C., Dang, J., Zhu, Y., Yang, X., Zhang, T., et al. (2021). Preparation of Fibroblast Suppressive Poly(ethylene Glycol)-B-Poly(l-phenylalanine)/Poly(ethylene Glycol) Hydrogel and its Application in Intrauterine Fibrosis Prevention. ACS Biomater. Sci. Eng. 7 (1), 311–321. doi:10.1021/acsbiomaterials.0c01390
Wang, J., Ju, B., Pan, C., Gu, Y., Zhang, Y., Sun, L., et al. (2016). Application of Bone Marrow-Derived Mesenchymal Stem Cells in the Treatment of Intrauterine Adhesions in Rats. Cell Physiol. Biochem. 39 (4), 1553–1560. doi:10.1159/000447857
Wang Y, Y., Yao, Z., Zhao, H., Yue, C., Yu, Q., Zhang, Y., et al. (2021). Reproductive Outcomes of In Vitro Fertilization-Intracytoplasmic Sperm Injection after Transcervical Resection of Adhesions: A Retrospective Cohort Study. J. Minim. Invasive Gynecol. 28 (7), 1367–1374. doi:10.1016/j.jmig.2020.10.029
Wang, Z., Yang, M., Mao, L., Wang, X., Wang, S., Cui, G., et al. (2021). Efficacy and Safety of Autologous Platelet-Rich Fibrin for the Treatment of Infertility with Intrauterine Adhesions. J. Obstet. Gynaecol. Res. 47 (11), 3883–3894. doi:10.1111/jog.14964
Wang, Z., Kumar, H., Tian, Z., Jin, X., Holzman, J. F., Menard, F., et al. (2018). Visible Light Photoinitiation of Cell-Adhesive Gelatin Methacryloyl Hydrogels for Stereolithography 3D Bioprinting. ACS Appl. Mater Interfaces 10 (32), 26859–26869. doi:10.1021/acsami.8b06607
Wassenaar, J. W., Braden, R. L., Osborn, K. G., and Christman, K. L. (2016). Modulating In Vivo Degradation Rate of Injectable Extracellular Matrix Hydrogels. J. Mater Chem. B 4 (16), 2794–2802. doi:10.1039/C5TB02564H
West, E. R., Shea, L. D., and Woodruff, T. K. (2007a). Engineering the Follicle Microenvironment. Semin. Reprod. Med. 25 (4), 287–299. doi:10.1055/s-2007-980222
West, E. R., Xu, M., Woodruff, T. K., and Shea, L. D. (2007b). Physical Properties of Alginate Hydrogels and Their Effects on In Vitro Follicle Development. Biomaterials 28 (30), 4439–4448. doi:10.1016/j.biomaterials.2007.07.001
Woo, D. H., Kim, S. K., Lim, H. J., Heo, J., Park, H. S., Kang, G. Y., et al. (2012). Direct and Indirect Contribution of Human Embryonic Stem Cell-Derived Hepatocyte-like Cells to Liver Repair in Mice. Gastroenterology 142 (3), 602–611. doi:10.1053/j.gastro.2011.11.030
Wu, X., Cai, H., Kallianpur, A., Li, H., Yang, G., Gao, J., et al. (2014). Impact of Premature Ovarian Failure on Mortality and Morbidity Among Chinese Women. PloS one 9 (3), e89597. doi:10.1371/journal.pone.0089597
Xia, H., Zhao, D., Zhu, H., Hua, Y., Xiao, K., Xu, Y., et al. (2018). Lyophilized Scaffolds Fabricated from 3D-Printed Photocurable Natural Hydrogel for Cartilage Regeneration. ACS Appl. Mater Interfaces 10 (37), 31704–31715. doi:10.1021/acsami.8b10926
Xiao, B., Yang, W., Lei, D., Huang, J., Yin, Y., Zhu, Y., et al. (2019). PGS Scaffolds Promote the In Vivo Survival and Directional Differentiation of Bone Marrow Mesenchymal Stem Cells Restoring the Morphology and Function of Wounded Rat Uterus. Adv. Healthc. Mater 8 (5), e1801455. doi:10.1002/adhm.201801455
Xin, L., Lin, X., Pan, Y., Zheng, X., Shi, L., Zhang, Y., et al. (2019). A Collagen Scaffold Loaded with Human Umbilical Cord-Derived Mesenchymal Stem Cells Facilitates Endometrial Regeneration and Restores Fertility. Acta Biomater. 92, 160–171. doi:10.1016/j.actbio.2019.05.012
Xu H, H., Xu, J., Shen, B., Zhang, S., Jin, B., Zhu, Q., et al. (2017). Dual Regulations of Thermosensitive Heparin-Poloxamer Hydrogel Using ε-Polylysine: Bioadhesivity and Controlled KGF Release for Enhancing Wound Healing of Endometrial Injury. ACS Appl. Mater Interfaces 9 (35), 29580–29594. doi:10.1021/acsami.7b10211
Xu Hl, H. L., Xu, J., Zhang, S. S., Zhu, Q. Y., Jin, B. H., ZhuGe, D. L., et al. (2017). Temperature-sensitive Heparin-Modified Poloxamer Hydrogel with Affinity to KGF Facilitate the Morphologic and Functional Recovery of the Injured Rat Uterus. Drug Deliv. 24 (1), 867–881. doi:10.1080/10717544.2017.1333173
Xu L, L., Ding, L., Wang, L., Cao, Y., Zhu, H., Lu, J., et al. (2017). Umbilical Cord-Derived Mesenchymal Stem Cells on Scaffolds Facilitate Collagen Degradation via Upregulation of MMP-9 in Rat Uterine Scars. Stem Cell Res. Ther. 8 (1), 84. doi:10.1186/s13287-017-0535-0
Xu, M., Banc, A., Woodruff, T. K., and Shea, L. D. (2009). Secondary Follicle Growth and Oocyte Maturation by Culture in Alginate Hydrogel Following Cryopreservation of the Ovary or Individual Follicles. Biotechnol. Bioeng. 103 (2), 378–386. doi:10.1002/bit.22250
Xu, M., West, E., Shea, L. D., and Woodruff, T. K. (2006). Identification of a Stage-specific Permissive In Vitro Culture Environment for Follicle Growth and Oocyte Development. Biol. Reprod. 75 (6), 916–923. doi:10.1095/biolreprod.106.054833
Xue, B., Liu, D., Song, M., Zhao, G., Cao, Y., Yan, G., et al. (2019). Leukemia Inhibitory Factor Promotes the Regeneration of Rat Uterine Horns with Full-Thickness Injury. Wound Repair Regen. 27 (5), 477–487. doi:10.1111/wrr.12729
Yamaguchi, N., Zhang, L., Chae, B. S., Palla, C. S., Furst, E. M., and Kiick, K. L. (2007). Growth Factor Mediated Assembly of Cell Receptor-Responsive Hydrogels. J. Am. Chem. Soc. 129 (11), 3040–3041. doi:10.1021/ja0680358
Yang, J., Bai, R., Chen, B., and Suo, Z. (2020). Hydrogel Adhesion: A Supramolecular Synergy of Chemistry, Topology, and Mechanics. Adv. Funct. Mater 30 (2), 1901693. doi:10.1002/adfm.201901693
Yang, K., Xie, D., Lin, W., Xiang, P., and Peng, C. (2021). Adipose Mesenchymal Stem Cells and Gingival Mesenchymal Stem Cells Have a Comparable Effect in Endothelium Repair. Exp. Ther. Med. 22 (6), 1415. doi:10.3892/etm.2021.10851
Yao, Q., Zheng, Y. W., Lan, Q. H., Wang, L. F., Huang, Z. W., Chen, R., et al. (2020). Aloe/poloxamer Hydrogel as an Injectable β-estradiol Delivery Scaffold with Multi-Therapeutic Effects to Promote Endometrial Regeneration for Intrauterine Adhesion Treatment. Eur. J. Pharm. Sci. 148, 105316. doi:10.1016/j.ejps.2020.105316
Yin, H., Kristensen, S. G., Jiang, H., Rasmussen, A., and Andersen, C. Y. (2016). Survival and Growth of Isolated Pre-antral Follicles from Human Ovarian Medulla Tissue during Long-Term 3D Culture. Hum. Reprod. 31 (7), 1531–1539. doi:10.1093/humrep/dew049
Yoon, H. J., Lee, Y. J., Baek, S., Chung, Y. S., Kim, D. H., Lee, J. H., et al. (2021). Hormone Autocrination by Vascularized Hydrogel Delivery of Ovary Spheroids to Rescue Ovarian Dysfunctions. Sci. Adv. 7 (18), eabe8873. doi:10.1126/sciadv.abe8873
Yu, D., Wong, Y. M., Cheong, Y., Xia, E., and Li, T. C. (2008). Asherman Syndrome-Oone Century Later. Fertil. Steril. 89 (4), 759–779. doi:10.1016/j.fertnstert.2008.02.096
Yu, L., Zhang, Z., Zhang, H., and Ding, J. (2010). Biodegradability and Biocompatibility of Thermoreversible Hydrogels Formed from Mixing a Sol and a Precipitate of Block Copolymers in Water. Biomacromolecules 11 (8), 2169–2178. doi:10.1021/bm100549q
Yuan, T., Li, K., Guo, L., Fan, H., and Zhang, X. (2011). Modulation of Immunological Properties of Allogeneic Mesenchymal Stem Cells by Collagen Scaffolds in Cartilage Tissue Engineering. J. Biomed. Mater Res. A 98 (3), 332–341. doi:10.1002/jbm.a.33121
Yuan, T., Zhang, L., Feng, L., Fan, H., and Zhang, X. (2010). Chondrogenic Differentiation and Immunological Properties of Mesenchymal Stem Cells in Collagen Type I Hydrogel. Biotechnol. Prog. 26 (6), 1749–1758. doi:10.1002/btpr.484
Zhang C, C., Hsieh, M. H., Wu, S. Y., Li, S. H., Wu, J., Liu, S. M., et al. (2020). A Self-Doping Conductive Polymer Hydrogel that Can Restore Electrical Impulse Propagation at Myocardial Infarct to Prevent Cardiac Arrhythmia and Preserve Ventricular Function. Biomaterials 231, 119672. doi:10.1016/j.biomaterials.2019.119672
Zhang, L., Yin, H., Lei, X., Lau, J., Yuan, M., Wang, X., et al. (2019). A Systematic Review and Meta-Analysis of Clinical Effectiveness and Safety of Hydrogel Dressings in the Management of Skin Wounds. Front. Bioeng. Biotechnol. 7, 342. doi:10.3389/fbioe.2019.00342
Zhang Q, Q., Bu, S., Sun, J., Xu, M., Yao, X., He, K., et al. (2017). Paracrine Effects of Human Amniotic Epithelial Cells Protect against Chemotherapy-Induced Ovarian Damage. Stem Cell Res. Ther. 8 (1), 270. doi:10.1186/s13287-017-0721-0
Zhang, S., Xia, W., Xu, J., Xu, H., Lu, C., Zhao, Y., et al. (2017). Three-dimensional Structure Micelles of Heparin-Poloxamer Improve the Therapeutic Effect of 17β-Estradiol on Endometrial Regeneration for Intrauterine Adhesions in a Rat Model. Int. J. Nanomedicine 12, 5643–5657. doi:10.2147/IJN.S137237
Zhang S, S., Xu, X., Xiang, W., Zhang, H., Lin, H., Shen, L., et al. (2020). Using 17β-Estradiol Heparin-Poloxamer Thermosensitive Hydrogel to Enhance the Endometrial Regeneration and Functional Recovery of Intrauterine Adhesions in a Rat Model. FASEB J. 34 (1), 446–457. doi:10.1096/fj.201901603RR
Zhang W, W., Wang, R., Sun, Z., Zhu, X., Zhao, Q., Zhang, T., et al. (2020). Catechol-functionalized Hydrogels: Biomimetic Design, Adhesion Mechanism, and Biomedical Applications. Chem. Soc. Rev. 49 (2), 433–464. doi:10.1039/c9cs00285e
Zhang, X., Liu, W., Zhou, Y., Qiu, J., Sun, Y., Li, M., et al. (2021a). Comparison of Therapeutic Efficacy of Three Methods to Prevent Re-adhesion after Hysteroscopic Intrauterine Adhesion Separation: a Parallel, Randomized and Single-Center Trial. Ann. Palliat. Med. 10 (6), 6804–6823. doi:10.21037/apm-21-1296
Zhang, X., Zhang, L., Li, Y., Yin, Z., Feng, Y., and Ji, Y. (2021b). Human Umbilical Cord Mesenchymal Stem Cells (hUCMSCs) Promotes the Recovery of Ovarian Function in a Rat Model of Premature Ovarian Failure (POF). Gynecol. Endocrinol. 37 (4), 353–357. doi:10.1080/09513590.2021.1878133
Zhao G, G., Cao, Y., Zhu, X., Tang, X., Ding, L., Sun, H., et al. (2017). Transplantation of Collagen Scaffold with Autologous Bone Marrow Mononuclear Cells Promotes Functional Endometrium Reconstruction via Downregulating ΔNp63 Expression in Asherman's Syndrome. Sci. China Life Sci. 60 (4), 404–416. doi:10.1007/s11427-016-0328-y
Zhao X, X., Wu, H., Guo, B., Dong, R., Qiu, Y., and Ma, P. X. (2017). Antibacterial Anti-oxidant Electroactive Injectable Hydrogel as Self-Healing Wound Dressing with Hemostasis and Adhesiveness for Cutaneous Wound Healing. Biomaterials 122, 34–47. doi:10.1016/j.biomaterials.2017.01.011
Zheng, J., Zhang, J., Kong, D., Song, Y., Zhao, S., Qi, W., et al. (2020). Quantification of the CM-Dil-Labeled Human Umbilical Cord Mesenchymal Stem Cells Migrated to the Dual Injured Uterus in SD Rat. Stem Cell Res. Ther. 11 (1), 280. doi:10.1186/s13287-020-01806-4
Zheng, W., Hao, Y., Wang, D., Huang, H., Guo, F., Sun, Z., et al. (2021). Preparation of Triamcinolone Acetonide-Loaded Chitosan/fucoidan Hydrogel and its Potential Application as an Oral Mucosa Patch. Carbohydr. Polym. 272, 118493. doi:10.1016/j.carbpol.2021.118493
Zhou, Y., Wen, L. L., Li, Y. F., Wu, K. M., Duan, R. R., Yao, Y. B., et al. (2022). Exosomes Derived from Bone Marrow Mesenchymal Stem Cells Protect the Injured Spinal Cord by Inhibiting Pericyte Pyroptosis. Neural Regen. Res. 17 (1), 194–202. doi:10.4103/1673-5374.314323
Keywords: hydrogel, premature ovarian failure, intrauterine adhesion, mesenchymal stem cells, artificial ovary
Citation: Zhang D, Ding C, Duan T and Zhou Q (2022) Applications of Hydrogels in Premature Ovarian Failure and Intrauterine Adhesion. Front. Mater. 9:942957. doi: 10.3389/fmats.2022.942957
Received: 13 May 2022; Accepted: 31 May 2022;
Published: 04 July 2022.
Edited by:
Boguang Yang, The Chinese University of Hong Kong, ChinaReviewed by:
Sayan Ganguly, Bar-Ilan University, IsraelWei Kang, Dalian University of Technology, China
Copyright © 2022 Zhang, Ding, Duan and Zhou. This is an open-access article distributed under the terms of the Creative Commons Attribution License (CC BY). The use, distribution or reproduction in other forums is permitted, provided the original author(s) and the copyright owner(s) are credited and that the original publication in this journal is cited, in accordance with accepted academic practice. No use, distribution or reproduction is permitted which does not comply with these terms.
*Correspondence: Tao Duan, dGR1YW5AeWFob28uY29t; Qian Zhou, c2h6aG91cWlhbkAxMjYuY29t