- 1InnoRenew CoE, Izola, Slovenia
- 2Faculty of Mathematics Natural Sciences and Information Technologies, University of Primorska, Koper, Slovenia
Natural fibers reinforced composites represent a sustainable alternative to synthetic polymer-based materials. However, natural fibers are susceptible to fungal degradation which reduces the lifespan of the composites in use. The objective of this study was to investigate the effect of biochar (BC) on improving the resistance to fungal decay of polylactic acid (PLA) and hemp-PLA composites. The influence of incremental BC loadings (0, 5, 10, and 20 wt%) on physiological and morphological properties of the composites was evaluated. Weight loss, color stability, microscopic observations, and water contact angle measurements were performed during and after 5-months of exposure to white (Trametes Versicolor) and brown (Fomitopsis Betulina) rot fungi. We found that the addition of 10 wt% BC decreased the total weight loss in PLA and hemp-PLA composites by 93% and 34%, respectively in the case of exposure to white rot and by 66% and 83%, respectively in the case of exposure to brown rot as compared to neat PLA. BC addition improved the color stability of the composites. The color change in hemp-PLA samples loaded by 10 wt% of BC decreased by 44% and 37%, in the case of exposure to white and brown rots, respectively as compared to hemp-PLA reference. Moreover, samples containing BC (5 wt% and 10 wt%) had higher gloss after the decay test among all other samples. Micrographs revealed that white and brown rots invaded the surface of the composites at the beginning of the incubation period and then accessed the interior of the materials. Micro-cracks occurred in samples containing hemp fibers which enabled the fungi to invade the internal structure of the composites. At the same time, fungi grew on the surface of samples without hemp fibers except for PLA with 20% BC content which had a porous surface that allowed fungi to access the interior. The hydrophobicity of all samples increased during the first 3 months of the incubation and then decreased again by the end of the incubation as the surface of the composites started to be damaged. Our study provided a novel direction of BC as an organic additive in bio-composites. Uncovering the potential of BC in improving the resistance to fungal decay of natural fibers-reinforced composites paves the way for BC’s utilization as a renewable additive in various applications such as bio-based packaging materials.
1 Introduction
The annual worldwide production of plastics reached 367 Mt in 2020 and is forecasted to reach 445 Mt by 2025 (Ian, 2022). Plastic materials are commonly used in packaging due to their low cost and favorable properties. It was estimated that 66% of the world’s plastic waste is derived from packaging applications. These generated wastes are mainly composed of petroleum-derived polymers such as polyethylene (PE) and polystyrene (PS). Efforts have been made to manage this huge volume of non-degradable plastic waste, however, only a small part is forwarded to recycling because of the limiting financial requirement and difficulties related to polymer sorting, purification, and re-manufacturing (Pivnenko et al., 2015). The remaining part ends up being disposed of in nature, landfills, and oceans. Therefore, the utilization of green and natural composites for packaging can help alleviate the issues associated with synthetic plastic disposal and reduce the negative environmental impact.
Carbonaceous materials have been recognized as high-potential additives for improving the strength of composites, including those used for packaging. For example, biochar (BC) is a renewable, abundant, and carbon-rich residue prepared by the pyrolysis of organic resources. The functionality of BC as an additive in polymeric matrices was attributed to its unique features, namely, its high porosity and rich carbon skeleton which enables the interaction and interfacial bonding with polymeric chains (Aup-Ngoen and Noipitak, 2020). A prior review (Das et al., 2015b) elucidated the essential role of BC in boosting the contribution of wood polymer composites in environmental sustainability given that BC can be obtained from low-added-value biomass. For instance, the investigations on the effect of the incorporation of wood-derived BC in PP-wood flour blends showed that 24 wt% BC improved the mechanical and thermal properties of the blends (Das et al., 2015a) while samples reinforced with 40 wt% of BC exhibited superior water resistance properties (Devallance et al., 2015).
Our previous study (Zouari et al., 2022) found that the incorporation of BC in PLA and hemp-PLA composites formulation was an effective way towards increasing the mechanical and hygroscopic properties of the materials when BC was added at optimized levels. Zhang et al. (Zhang et al., 2017) found that 10 wt% of corn stalk-derived BC was effective in increasing the flexural, tensile, and impact strength properties of wood flour-high-density polyethylene (HDPE) composites. They pointed out that BC can find a pathway to be utilized in extruded materials as a high-potential and renewable additive. In another study (Wang et al., 2019), researchers reported that Douglas-Fir-derived BC could enhance the photostability of HDPE matrices and minimized the color change resulting from accelerated UV weathering tests. In agricultural application, the role of BC in inhibiting pathogens, including fungi, has been verified (Poveda et al., 2021). For this reason, BC has been used for preventing plants diseases through soil amendment. Details about the mode of action of BC on soil pathogens are still rare due to the complexity of the mechanism. The effect of BC for controlling pathogens’ growth in soil was mostly linked to its ability in influencing pathogens motility (Gu et al., 2017), competition with the pathogen for colonization sites (Elmer, 2016), and changing soil properties (water and nutrients retention) (Warnock et al., 2007). The potential of BC in controlling fungal growth in composite materials has not been addressed yet. Our hypothesis suggests that the addition of BC in composites formulations might help to prevent fungal decay by reducing the amount of water and nutrients available for fungal growth.
Plant fiber-reinforced thermoplastic composites like those using wood or hemp, are renewable, cost-efficient, and have good mechanical properties. They have successfully emerged as suitable products for applications in large industries such as automotive (Huda and Widiastuti, 2021) and construction (Zhang, 2014). Here they are mainly for non-structural construction uses like decking, fencing, and siding. Plant fibers derived from agricultural side streams are a good candidate for polymer reinforcement given their availability and cost efficiency. For instance, hemp fibers that are obtained from hemp stems, a major by-product of the cannabidiol industry, were investigated as potential fillers for polymeric materials (Zouari et al., 2022). Hemp fibers were widely investigated as reinforcement in composite materials. Hemp fibers were reported to enhance Young modulus (Marrot et al., 2021) and other features of PLA composites such as resistance to moisture and fire (Alao et al., 2021). Prior research (Thiagamani et al., 2019) reported that combined hemp and sisal fibers reinforcements were effective in increasing tensile and compressive strength of epoxy-based hybrid composites when the stacking sequences were well-optimized. Likewise, hemp-sisal fibers fillers had a positive effect in term of maintaining the impact resistance (Senthilkumar et al., 2021) and improving the creep behaviors (Senthilkumar et al., 2022) of bio-epoxy composites after exposure to accelerated UV-weathering. However, the use of plant fibers in polymer composites is still limited due to the susceptibility to biological degradation by living organisms such as termites and wood fungi which damage the material and cause early decay leading to loss of mechanical, morphological, and physical properties (Ding et al., 2018). We believe that the addition of BC can help improve the composite’s resistance to biological degradation. To date, most studies have focused on the effect of carbon-based additives on the mechanical, thermal, and physical properties of fiber-polymer composites while their effect on the fungal decay and biodegradation of plant fiber-polymer composites has not been extensively studied.
The objective of this study is to investigate the potential effect of different BC loadings on the fungal decay resistance of PLA and hemp-PLA composites.
2 Materials and methods
2.1 Materials
PLA composites reinforced with short hemp fibers (0 and 30 wt%) and wood-derived BC (0, 5, 10, and 20 wt%) were compounded and injection molded into test specimens. Details about the preparation and characterization of the composites are reported in our previous work (Zouari et al., 2022).
Potato dextrose purchased from Liofilchem (Teramo, Italy) was utilized as a medium culture for inoculation of the rots and incubation of the samples. According to manufacturer instructions, 42 g of potato dextrose agar powder was mixed with 1 L of distilled water and sterilized for 15 min at 121°C.
2.2 Isolation and preparation of the fungi inoculum
The inoculum was taken from seven-day-old cultures of white rot: Trametes Versicolor (TV 20-10) and brown rot: Fomitopsis betulina, growing at 25°C and 92% relative humidity. A mycelium of cultures (6 mm × 6 mm) was placed in the center of Petri dishes coated with 50 mm thick potato dextrose agar preparation. The inoculums were then incubated in dark conditions for 7 days at 25°C and 92% relative humidity until complete colonization of the culture media surface.
2.3 Fungal decay test
Specimens of PLA and reinforced PLA composites (1 × 1 × 0.5 cm3) were sterilized in the oven at 105 °C before being put into the prepared Petri dishes. For each type of sample, ten Petri dishes were prepared (five inoculated with white rot and five inoculated with brown rot) resulting in a total of 70 Petri dishes for the 5-month test period. The inoculation process was conducted under sterile conditions in a UV chamber (Medico Tehnika d. o.o, Ljubljana, Slovenia). The cultures were then covered and incubated in a climatic chamber (Kambič KK-8000 CH-2, Semič, Slovenia) at 25°C and 90% relative humidity for 5 months. For each sample, the Petri dishes were marked by month number: M1, M2, M3, M4, and M5. At the end of each month, specimens corresponding to the relevant month were taken for characterization while the other specimens were kept under incubation to continue the degradation test. For example, after the first degradation month, specimens marked with M1 were taken, cleaned with distilled water, and oven dried at 105°C overnight until constant weight, then, samples were characterized. The same strategy was followed for the next months.
2.4 Characterization of the decayed composites
2.4.1 Weight loss measurements
The degradation of composites under fungal activity was evaluated by determination of the weight loss at the end of every month during 5 months of incubation.
The percentage of weight loss was calculated using Eq. 1.
Where Wi is the initial dry weight of the sample before exposure to fungi and Wf is the weight of the cleaned and dried sample after exposure to fungi.
2.4.2 Morphological and optical characterization
A digital microscope (VHX-6000, Keyence, Osaka, Japan) was used to observe the apparent morphology of the decayed composites and track the growth of the mycelium on the surface and inside the materials. Microscopic images were taken monthly, however, in the results section, only images taken at the end of the biodegradation test period were represented.
2.4.3 Color measurements
The color of the composites was evaluated before and after the biodegradation test using a spectrophotometer (Spektromaster 565-45, Erichsen, Hemer, Germany) based on the CIE L*a*b* system. “L” is the lightness coordinate with a value ranging between 0 for perfect black and 100 for perfect white; “a” and “b” refer to chromaticity coordinates on the green-red and blue-yellow axes. D65 illuminant, 10° viewer angle, and a spectral range of 400–700 nm were used for the measurements. The values reported in this study are the result of the average of three repetitions performed at different spots on the surface of each sample. The total color change ∆E caused by 5 months of exposure to fungi was calculated according to Eq. 2 to assess color stability.
where ∆L, ∆a, and ∆b correspond to the differences between color coordinate values measured before and after exposure of the composites to fungi.
2.4.4 Water contact angle measurements
Water resistance was assessed by measuring the dynamic contact angle at the surface of the samples following the sessile drop test method. Tests were performed at 23°C using an optical tensiometer (Attension Theta Flex Auto 4 system, Biolin Scientific, Gothenburg, Sweden) equipped with a 3D topography module. Five drops of distilled water (4 µL each) were placed on each specimen and the average values of water contact angles were determined.
2.4.5 Statistical analysis
For statistical evaluation of the data, one-way analysis of variance (ANOVA) was conducted using IBM SPSS Statistics program (version: 29). Significant differences among the mean values of the different parameters (i.e., total weight loss, color change, gloss unit, and water contact angle) measured before and after 5 months of the decay test were determined using Tukey post-hoc test at 5% significance level.
3 Results and discussion
3.1 Weight loss during the fungal decay test
Weight loss occurred during incubation of the composites is plotted in Figures 1A, B for white and brown rot, respectively.
All composites showed low weight variation after the first month (M1) of incubation with white and brown rots. The slow weight loss at the beginning of the degradation test was expected because the growth cycle of micro-organisms starts with a stationary phase which is required to adapt to the culture media and produce the necessary enzymes to utilize the present nutrients, proliferate, and initiate the degradation. Similarly, Ding et al. (Ding et al., 2018) did not record any weight loss in PLA-10 wt% jute fiber composites after incubation with five different fungi (Aspergillus niger, Gliocladium virens, Chaetomium globosum, Aureobasidium pullulans, and Penicillium pinophilum) for 28 days.
For neat PLA, the weight loss caused by Trametes Versicolor (white rot) was constant and lower than 1% from M1 to M3 then, it increased to 2.5% and 3.2% in M4 and M5, respectively. However, the weight loss caused by Fomitopsis betulina (brown rot) was near 1% and remained steady during the entire decay test period, thus we could assume that the white rot fungi had a higher ability to degrade PLA’s molecular chains which was likely caused by the difference in degradation mechanism adopted by the different fungi, namely, the type of enzymes produced and used for the degradation. Few studies have focused on the degradation of PLA using fungi (Xu et al., 2022). For instance, (Janczak et al., 2020), reported the efficiency of fungi in PLA degradation, in their study however, they investigated selected fungal strains other than white and brown rots. In general, results obtained for neat PLA degradation showed that the polymeric matrix had limited biodegradability under the effect of wood basidiomycetes which was supported by the low weight loss during the fungal exposure period. Indeed, PLA is composed of long molecular chains and its degradation requires the presence of enzymes mixture produced by fungi to break down the complex polymers into simple molecules (i.e., oligomer, dimer, and monomer) (Urbanek et al., 2020). The fungi can then utilize the degradation products as source of carbon and energy. The total weight loss determined at the end of the degradation test (M5), decreased with the increase of the BC loading up to 10 wt% BC signifying that BC reduced the intensity of decay in both fungal strains. The BC addition significantly affected the weight loss of the composites. ANOVA test results showed a statistically significant difference between composites (p = 0.0009) regardless of the BC loading.
The weight loss caused by white rot was reduced by 76% and 93% for 5 wt% and 10 wt% BC loadings, respectively compared to the neat PLA sample. Tukey’s test showed statistically significant differences between the average weight loss of the PLA-BC and hemp-PLA-BC composites as compared to the neat PLA reference.
Brown rot caused a total weight loss that was 74% and 66% lower compared to the neat PLA reference for 5 wt% and 10 wt% BC loadings, respectively. ANOVA test results showed a statistically significant difference between all the composites (p = 0.0009). Tukey’s test showed that there was no statistically significant difference in average weight losses between PLA-5% BC and PLA-10% BC (p = 1.000).
The decrease in weight loss after the incorporation of BC particles can be attributed to its hygroscopic property. The BC utilized in this current study has a relatively high porosity with 230.8 m2/g specific and 480.0 m2/g microporous surface areas, respectively (Zouari et al., 2022). This high porosity and surface area of the BC particles enabled water molecules retention from the culture media which reduced the availability of water necessary for fungal growth. In a similar context, Kord et al. (Kord et al., 2021) investigated the fungal degradation (under the effect of white-rot fungus: Coriolus Versicolor) of PP-40 wt% rice straw composites loaded with different loads of carbon nanotubes (CNTs) over 4 months. They found that the susceptibility of composites degradation upon CNTs addition decreased and that the weight loss was reduced by 17%, 33%, and 44% after the addition of 2 wt%, 4 wt%, and 6 wt% of CNTs as compared to the pure PP sample. They reported that water molecules were saturated around the CNTs which prohibited fungi from using the water for their proliferation. They also reported that, based on scanning electron microscopy images, CNTs filled the cavities on the surface of the composites which helped to limit the extent of fungal attack. Compared to the study that used CNTs, BC showed a great potential in limiting weight loss and preventing fungal growth which makes it a suitable cost-efficient and renewable alternative to engineered carbonaceous products (e.g., CNTs).
Further addition of BC up to 20 wt% was not favorable in terms of reducing the weight loss and the extent of damage caused by fungi. For the specimens containing 20 wt% of BC, the weight loss increased gradually with the increasing exposure time reaching 2% by M5 in the case of white rot fungi. In the case of brown rot, the weight loss was almost constant from M1 to M4 with an average of 1.2%. Then, by M5, the weight loss increased to reach 2.6%. It is worth noting that, for PLA-20% BC, the total weight loss was close to 64% and 89%, and 89% and 91% higher compared to PLA-5% BC and PLA-10% BC in case of exposure to white and brown rots, respectively. These findings can be attributed to the less homogeneous dispersion of BC particles within the polymeric matrix when high BC content was added. The poor dispersion likely favored the occurrence of aggregates which were responsible for lowering the strength and the structural integrity of the composites. Thus, the fungi could initiate biodegradation and invade the material through the weakened spots.
Hence, it is crucial to optimize the BC levels to achieve optimal resistance to biological decay. The difference between weight loss percentages caused by white and brown rots can be the result of different degradation mechanisms that vary from one species to another depending on the type of enzymes produced by the microorganism.
For the hemp-PLA sample (PLA- 30% HF), the weight loss increased gradually during the exposure period in the case of both fungal strains. The total weight loss (i.e., at M5) caused by white and brown rots was 58% and 75% higher as compared to neat PLA, respectively. Hemp fibers are composed of natural polymers (i.e., hemicellulose, cellulose, and lignin) which are susceptible to biodegradation. These natural polymers can be hydrolyzed and utilized as a source of nutrients, supporting the growth of microorganisms. It is important to mention that the weight loss was more apparent in the case of exposure to white rot which can be attributed to the ability of white rot to decompose the three major components of plant cell wall via the hydrolytic and oxidative reactions of hemicellulases (glycoside hydrolases and carbohydrate esterases), cellulases (endoglucanases, cellobiohydrolases, and β-glucosidases), and ligninolytic enzymes (Lynd et al., 2002; Zabel and Morrell, 2020) compared to brown rot that selectively degrades cellulosic polymers (Zabel and Morrell, 2020). When BC was added to hemp-PLA, the total weight loss decreased by 23% and 34% for 5 wt% and 10 wt% loadings, respectively in the case of white rot exposure and by 41% and 83% for 5 wt% and 10 wt% loadings, respectively in the case of brown rot exposure. These findings confirm the efficiency of BC as an additive to minimize the total weight loss resulting from fungal degradation and therefore retarding the decay of the composites.
3.2 Morphology and optical properties of the decayed PLA-based composites
3.2.1 Visual and microscopic morphology assessment
The appearance of the specimens before and after 5 months of exposure to white rot and brown rot fungi is shown in Figures 2A, B for white and brown rot, respectively.
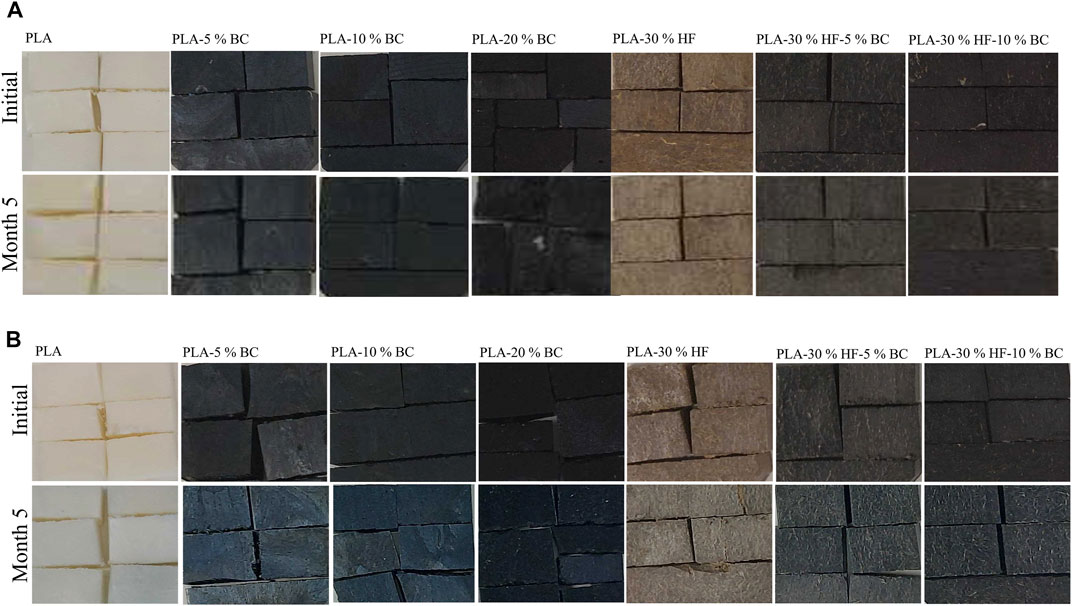
FIGURE 2. Photographs of the specimens before and after 5 months of incubation with (A) white rot and (B) brown rot.
Visual assessment of the sample’s surface shows that the original color of all the composites was altered and that all samples, except pure PLA, became brighter after exposure to fungi. Thin white marks could be occasionally observed on PLA-BC composites; however, they were more frequent in the case of hemp-containing composites (with and without BC). These marks are attributed to the damage caused by the fungal decay and the possible remaining grown fungal mycelium after cleaning the samples. The visual change in morphology was more noticeable in the case of hemp-PLA samples with and without BC which can be attributed to the surface damage and occurrence of visible cracks favored by the presence of hemp fibers as discussed previously in Section 3.1.
Changes on the composites’ exposed surface were also observed under the microscope after the fifth month of exposure to white and brown rots, shown in Figures 3A, B, respectively.
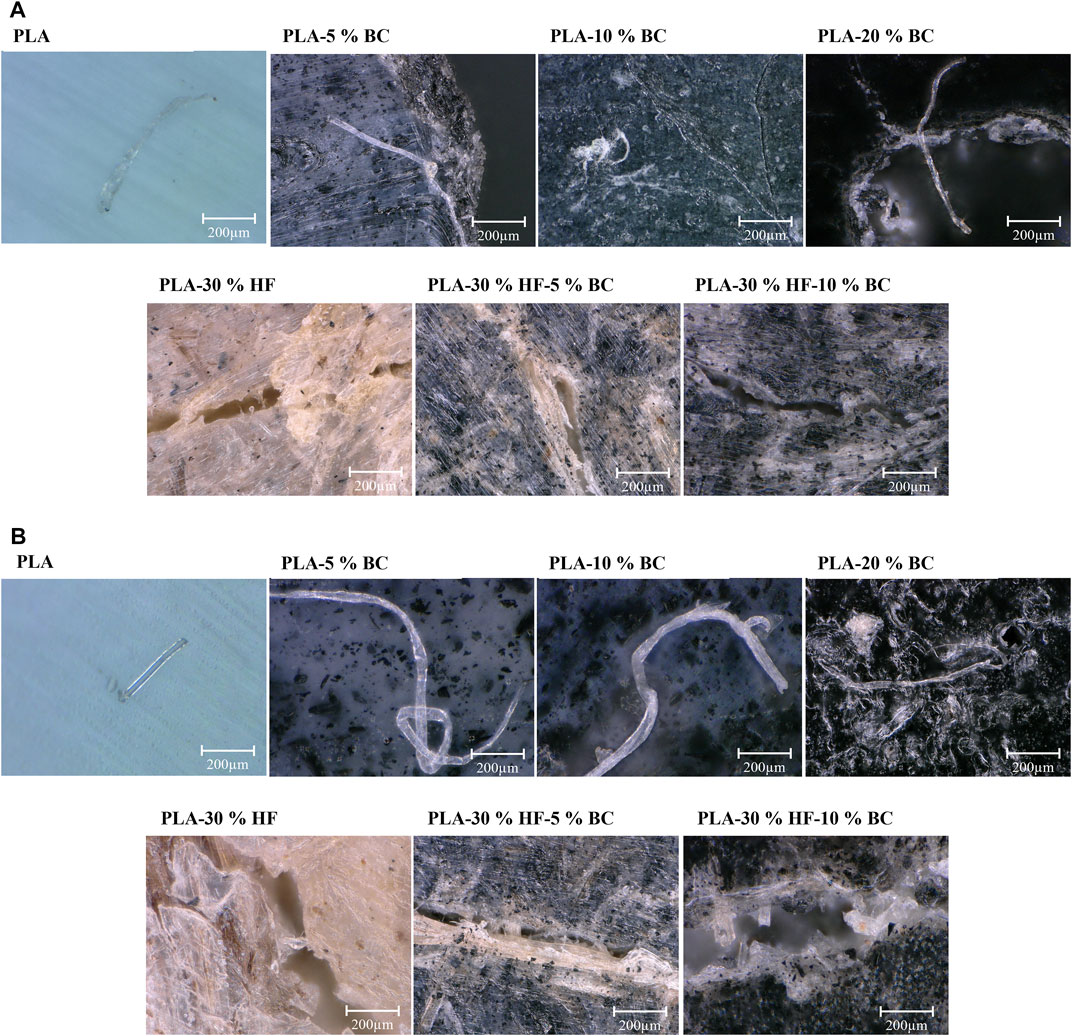
FIGURE 3. Microscopic images of the surface of specimens incubated with (A) white rot and (B) brown rot for 5 months.
Microscopic observations show that for neat PLA and PLA loaded with 5 wt% and 10 wt% of BC samples, the mycelium cells are present on the surface which appears to have a robust condition. However, for the sample containing 20 wt% of BC, a porous structure was observed, and fungal cells were detected around the pores from where they could likely invade the interior of the material and facilitate the decay. At high BC levels, the dispersion of the particles within the polymeric matrix was not uniform which made the material more fragile and susceptible to attack by fungi. These findings agree with the weight loss results (Section 3.1) that were higher in the case of PLA-20% BC compared to PLA, PLA-5% BC, and PLA-10% BC.
In the case of specimens containing hemp fibers, narrow micro-cracks could be observed (Figure 2). The fungi were growing around the cracks which enabled the fungal mycelia to access the interior of the composites. It is worth mentioning that the cracks were specifically observed around the hemp fibers. Hemp fibers are composed of hemicellulose, cellulose, and lignin, specifically the presence of hemicelluloses gives a hydrophilic character to the fibers due to the ability of hydroxyl groups to absorb and retain water molecules. The fibers likely swell after trapping the moisture from the surrounding media which favored the occurrence of micro-cracks and allowed micro-organisms to grow near the humid area. For hemp-PLA composites loaded with BC, microcracks also occurred around the fibers, however, fewer fungal hyphae were observed which confirms the potential effect of BC on improving the resistance of the composites against fungal attack.
3.2.2 Color change
In terms of color change and gloss units, ANOVA test results showed a statistically significant difference between all the composites (p = 0.001) regardless of the BC loading and the utilized fungal strain. Tukey’s test showed statistically significant differences between the average color change of all composites as compared to the neat PLA reference.
Figures 4A, B represent the color change (∆E) of the composites after exposure to white and brown fungi, respectively. Figures 5A, B represent the gloss unit (GU) values of the composites before (control) and after (decayed) the 5 months of exposure to white and brown fungi, respectively.
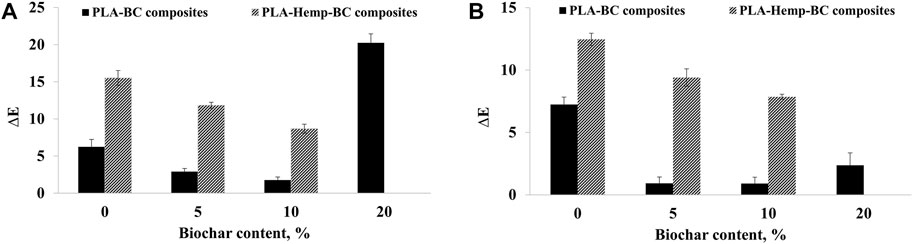
FIGURE 4. Color change (∆E) of PLA-based composites after exposure to (A) white rot and (B) brown rot fungi in the function of biochar content.
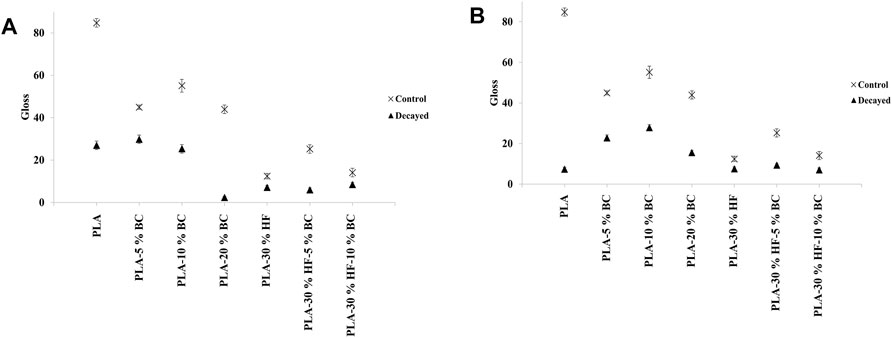
FIGURE 5. Gloss unit (GU) of PLA-based composites before and after exposure to (A) white rot and (B) brown rot fungi.
For PLA-BC composites, the intensity of color change (∆E) after fungal decay of the composites decreased gradually with the addition of 5 wt% and 10 wt% of BC in the case of exposure to white and brown rots (Figure 5). The composites with 10 wt% BC content exhibited the least color change among all the composites after 5 months of incubation with fungi. However, further addition of BC up to 20 wt% was correlated with an increase in ∆E.
For hemp-PLA composites, ∆E was higher by 149% and 72% compared to neat PLA samples in the case of exposure to white and brown rots, respectively. When BC was added to hemp-PLA composites by up to 10 wt%, the ∆E decreased by 44% and 37%, in the case of exposure to white and brown rots respectively.
Irrespective of the BC and hemp fibers contents, the gloss values of all the composites before the biodegradation test ranged from 12.4 to 84.8. After 5 months of exposure to white and brown rots, the gloss values dropped to the range of 2.3–29.8 and 7.3–27.8, respectively.
The fluctuation of gloss values followed a similar trend as ∆E. Samples containing up to 10 wt% of BC had higher gloss compared to neat PLA, PLA-20% BC, and PLA-30% HF samples.
These results correlated with the weight loss measurements presented in Section 3.1. The addition of BC (up to 10 wt%) limited the fungal growth and extent of damage which contributed to the color stability and gloss of the decayed composites. Moreover, the positive effect of BC on the optical properties of the composites can be attributed to its natural black color which likely helped to cover the defects on the surface of the samples after incubation with the fungi. Therefore, the BC addition could preserve the aesthetic properties of the PLA and hemp-PLA materials.
3.3 Surface hydrophobicity of the PLA-based composites
The surface hydrophobicity of the PLA -based composites was evaluated by water contact angle (WCA) measurements recorded for 30 s. Mean values are obtained from the result of five drops per specimen and the corresponding standard deviations are represented in Table 1.
In terms of WCA, ANOVA test results showed a statistically significant difference between all the composites (p = 0.0009) regardless of the BC loading and the utilized fungal strain. Tukey’s test showed statistically significant differences between the average color change of all composites as compared to the neat PLA reference.
The WCA of all samples followed the same trend. In the case of exposure to white and brown rots, the WCA values increased gradually (compared to the undecayed samples) from M1 to M3 then decreased during M4 and M5.
The increase in the surface hydrophobicity of the composites can be attributed to the water-resilient character of the fungal mycelia covering the surface. Another reason can be the increased surface roughness of the composites caused by the grown hyphae. Prior research (Haneef et al., 2017) investigated the hydrodynamic properties of mycelium fibrous films produced by two types of white rot fungi (Ganoderma lucidum and Pleurotus ostreatus) grown on pure cellulose and cellulose-potato dextrose broth media. The research findings showed that the films were resistant to water which was reflected by low moisture uptake values (<4% at 50% RH) and hydrophobic WCA higher than 120° for all the samples. The results were assigned to the rough topology of the films’ surfaces and to the presence of hydrophobic proteins that are specific to the cell wall of fungi such as mannoproteins and hydrophobins. Prior literature (Wessels, 1996; Alexandre et al., 2000) reported the presence of water-repellent proteins with the ability to self-assemble and form a hydrophobic layer on the outer part of the fungal cell wall. Further research should be implemented to confirm the presence of such types of hydrophobic proteins on the surface of the decayed specimens.
From M1 to M3, PLA- 30% HF sample had a high hydrophobicity (WCA ranged between 121 ± 15° and 138 ± 7° and between 130 ± 7° and 138 ± 5° in the case of incubation with white and brown rots, respectively) which can be explained by rapid fungal growth of the surface and a greater concentration of hydrophobic components released by microorganisms. However, during the same period, hemp-PLA samples loaded with 5 wt% and 10 wt% of BC had lower hydrophobicity which can be attributed to the effect of BC in limiting fungal growth. After M3, the WCA of the PLA- 30% HF sample decreased to reach 97 ± 2° and 115 ± 5° by M5 for composites exposed to white and brown rots, respectively which can be correlated with the occurrence of micro-cracks on the surface of the composites at the same period and the invasion of the interior. Micro-cracks likely interrupted the hydrophobic layer produced by the rots that were covering the surface which likely resulted in moisture uptake and led to decreasing the surface hydrophobicity. Non-etheless, PLA-30% HF-5% BC and PLA-30% HF-10% BC had higher WCA at M5 compared to the non-reinforced hemp-PLA (The increase in WCA was about 6% and 9%, and 10% and 12% in the case of white and brown fungi exposure, respectively). The higher WCA at the end of the biodegradation test compared to the PLA-30% HF sample can be attributed to the favorable effect of BC that reduced the occurrence of microcracks, thus, the mycelium layer stayed on the surface and less moisture was absorbed. These results agree with the microscopic observations (Figure 3).
With the progress of the exposure period, the surface of the composites started to be damaged and microcracks appeared. Moreover, hyphae started to access the interior, therefore, the WCA decreased again. However, it stayed higher than the initial values for most of the samples likely because of the presence of hydrophobic hyphae.
4 Conclusion
Fungal decay resistance of plant fiber-reinforced polymeric composites is an important factor contributing to the performance and durability of the material in service. The effect of biochar (BC) additives on the weight loss, color stability, and water resistance of PLA and hemp-PLA composites exposed to fungal decay was investigated. The main outcomes of the study were.
• The increase in BC levels to up to 10 wt% decreased the total weight loss of PLA by 93% and 66% after 5 months of exposure to white and brown rots, respectively. 10 wt% of BC also reduced the total weight loss of hemp-PLA by 34% and 83% after 5 months of exposure to white and brown rots, respectively.
• Further increase in BC loading (20 wt%) was not beneficial in terms of minimizing weight loss.
• The color change was more apparent in samples containing hemp fibers. Composites with 10 wt% BC exhibited the highest color stability after the decay test.
• Microscopic observation showed that for PLA and PLA-BC samples, the growth of fungi occurred mainly at the surface except for PLA-20 wt% BC where hyphae accessed the interior of the material through the porous structure. For hemp-PLA samples with and without BC, the rots grew mainly around the fibers which favored the occurrence of micro-cracks and the invasion of the composites’ interior.
• An increase in surface hydrophobicity was observed during the first 3 months of incubation which was assumed to have resulted from the ability of the utilized rots in releasing hydrophobic proteins during their proliferation besides the increase in surface roughness. Then, surface hydrophobicity decreased because of the occurrence of micro-cracks and fragilization of the materials.
We attributed the potential of BC in improving the composites’ resistance to fungal decay to its hygroscopic property which limited the availability of water molecules necessary for fungal proliferation. To sum up, the addition of optimized BC content to natural fibers reinforced polymeric composites could enhance the resistance to fungal decay and improve the durability of the material.
Further research will be implemented in the future to study the effect of different BC properties (surface area, pores distribution, and particle size) on the biodegradability of bio-composites. Another aspect that will be considered in future research is the utilization of a compatibilizer to ensure better bonding and interaction between PLA and hemp fibers. Then investigate the potential synergetic effect of compatibilizer and BC on the occurrence of micro-cracks.
Data availability statement
The original contributions presented in the study are included in the article/Supplementary material, further inquiries can be directed to the corresponding author.
Author contributions
The manuscript was prepared through the contributions of all authors. Material preparation was performed by MZ and MM. Testing, data collection, and first draft writing were performed by MZ. Validation and manuscript reviews were performed by MM and MS. All authors have approved the final version of the manuscript.
Acknowledgments
The authors gratefully thank the European Commission for funding the InnoRenew project (Grant agreement #739574 under the Horizon 2020 WIDESPREAD-2-Teaming program) and the Republic of Slovenia (investment funding from the Republic of Slovenia and the European Regional Development Fund). The authors would also like to thank the Slovenian Research Agency and Grant Agency of the Czech Republic for funding the WEAVE project (22-14942K) which is complementary and has enhanced this study.
Conflict of interest
The authors declare that the research was conducted in the absence of any commercial or financial relationships that could be construed as a potential conflict of interest.
Publisher’s note
All claims expressed in this article are solely those of the authors and do not necessarily represent those of their affiliated organizations, or those of the publisher, the editors and the reviewers. Any product that may be evaluated in this article, or claim that may be made by its manufacturer, is not guaranteed or endorsed by the publisher.
References
Alao, P. F., Marrot, L., Kallakas, H., Just, A., Poltimäe, T., and Kers, J. (2021). Effect of hemp fiber surface treatment on the moisture/water resistance and reaction to fire of reinforced PLA composites. Materials 14, 4332. doi:10.3390/ma14154332
Alexandre, H., Blanchet, S., and Charpentier, C. (2000). Identification of a 49-kDa hydrophobic cell wall mannoprotein present in velum yeast which may be implicated in velum formation. FEMS Microbiol. Lett. 185, 147–150. doi:10.1111/j.1574-6968.2000.tb09053.x
Aup-Ngoen, K., and Noipitak, M. (2020). Effect of carbon-rich biochar on mechanical properties of PLA-biochar composites. Sustain. Chem. Pharm. 15, 100204–100212. doi:10.1016/j.scp.2019.100204
Das, O., Sarmah, A. K., and Bhattacharyya, D. (2015a). A novel approach in organic waste utilization through biochar addition in wood/polypropylene composites. Waste Manag. 38, 132–140. doi:10.1016/j.wasman.2015.01.015
Das, O., Sarmah, A. K., and Bhattacharyya, D. (2015b). A sustainable and resilient approach through biochar addition in wood polymer composites. Sci. Total Environ. 512–513, 326–336. doi:10.1016/j.scitotenv.2015.01.063
Devallance, D., Oporto, G., and Quigley, P. (2015). Investigation of hardwood biochar as a replacement for wood flour in wood-polypropylene composites. J. Elastomers Plast. 48, 510–522. doi:10.1177/0095244315589655
Ding, D., Yu, T., and Li, Y. (2018). Biodegradation of jute/poly(lactic acid) composites by fungi. Sci. China Technol. Sci. 61, 1705–1712. doi:10.1007/s11431-017-9215-7
Elmer, W. H. (2016). Effect of leaf mold mulch, biochar, and earthworms on mycorrhizal colonization and yield of Asparagus affected by Fusarium crown and root rot. Plant Dis. 100, 2507–2512. doi:10.1094/PDIS-10-15-1196-RE
Gu, Y., Hou, Y., Huang, D., Hao, Z., Wang, X., Wei, Z., et al. (2017). Application of biochar reduces Ralstonia solanacearum infection via effects on pathogen chemotaxis, swarming motility, and root exudate adsorption. Plant Soil 415, 269–281. doi:10.1007/s11104-016-3159-8
Haneef, M., Ceseracciu, L., Canale, C., Bayer, I. S., Heredia-Guerrero, J. A., and Athanassiou, A. (2017). Advanced materials from fungal mycelium: Fabrication and tuning of physical properties. Sci. Rep. 7, 41292–41303. doi:10.1038/srep41292
Huda, K., and Widiastuti, I. (2021). Natural fiber reinforced polymer in automotive application: A systematic literature review. J. Phys. Conf. Ser. 1808, 012015–012031. doi:10.1088/1742-6596/1808/1/012015
Ian, T. (2022). Global plastics production forecast 2025-2050. Statista. Available at: https://www.statista.com/statistics/664906/plastics-production-volume-forecast-worldwide/ (Accessed September 4, 2022).
Janczak, K., Dąbrowska, G. B., Raszkowska-Kaczor, A., Kaczor, D., Hrynkiewicz, K., and Richert, A. (2020). Biodegradation of the plastics PLA and PET in cultivated soil with the participation of microorganisms and plants. Int. Biodeterior. Biodegr. 155, 105087. doi:10.1016/j.ibiod.2020.105087
Kord, B., Ayrilmis, N., and Ghalehno, M. D. (2021). Effect of fungal degradation on technological properties of carbon nanotubes reinforced polypropylene/rice straw composites. Polym. Polym. Compos. 29, 303–310. doi:10.1177/0967391120915347
Lynd, L. R., Weimer, P. J., van Zyl, W. H., and Pretorius, I. S. (2002). Microbial cellulose utilization: Fundamentals and biotechnology. Microbiol. Mol. Biol. Rev. 66, 506–577. doi:10.1128/MMBR.66.3.506-577.2002
Marrot, L., Alao, P. F., Mikli, V., and Kers, J. (2021). Properties of frost-retted hemp fibers for the reinforcement of composites. J. Nat. Fibers 10, 16017–16028. doi:10.1080/15440478.2021.1904474
Pivnenko, K., Jakobsen, L., Eriksen, M., Damgaard, A., and Astrup, T. (2015). “Challenges in plastics recycling,” in Sardinia 2015-15th international waste management and landfill symposium (Padova, Italy: CISA Publisher).
Poveda, J., Martínez-Gómez, Á., Fenoll, C., and Escobar, C. (2021). The use of biochar for plant pathogen control. Phytopathology® 111, 1490–1499. doi:10.1094/PHYTO-06-20-0248-RVW
Senthilkumar, K., Subramaniam, S., Ungtrakul, T., Kumar, T. S. M., Chandrasekar, M., Rajini, N., et al. (2022). Dual cantilever creep and recovery behavior of sisal/hemp fibre reinforced hybrid biocomposites: Effects of layering sequence, accelerated weathering and temperature. J. Ind. Text. 51, 2372S–2390S. doi:10.1177/1528083720961416
Senthilkumar, K., Ungtrakul, T., Chandrasekar, M., Senthil Muthu Kumar, T., Rajini, N., Siengchin, S., et al. (2021). Performance of sisal/hemp bio-based epoxy composites under accelerated weathering. J. Polym. Environ. 29, 624–636. doi:10.1007/s10924-020-01904-7
Thiagamani, S. M. K., Krishnasamy, S., Muthukumar, C., Tengsuthiwat, J., Nagarajan, R., Siengchin, S., et al. (2019). Investigation into mechanical, absorption and swelling behaviour of hemp/sisal fibre reinforced bioepoxy hybrid composites: Effects of stacking sequences. Int. J. Biol. Macromol. 140, 637–646. doi:10.1016/j.ijbiomac.2019.08.166
Urbanek, A. K., Mirończuk, A. M., García-Martín, A., Saborido, A., de la Mata, I., and Arroyo, M. (2020). Biochemical properties and biotechnological applications of microbial enzymes involved in the degradation of polyester-type plastics. Biochim. Biophys. Acta Proteins Proteomics 1868, 140315. doi:10.1016/j.bbapap.2019.140315
Wang, X., Yu, Z., and McDonald, A. G. (2019). Effect of different reinforcing fillers on properties, interfacial compatibility and weatherability of wood-plastic composites. J. Bionic Eng. 16, 337–353. doi:10.1007/s42235-019-0029-0
Warnock, D. D., Lehmann, J., Kuyper, T. W., and Rillig, M. C. (2007). Mycorrhizal responses to biochar in soil – concepts and mechanisms. Plant Soil 300, 9–20. doi:10.1007/s11104-007-9391-5
Wessels, J. G. H. (1996). “Hydrophobins: Proteins that change the nature of the fungal surface,” in Advances in microbial physiology (Elsevier), 1–45. doi:10.1016/S0065-2911(08)60154-X
Xu, B., Chen, Y., He, J., Cao, S., Liu, J., Xue, R., et al. (2022). New insights into the biodegradation of polylactic acid: From degradation to upcycling. Environ. Rev. 30, 30–38. doi:10.1139/er-2020-0117
Zabel, R. A., and Morrell, J. J. (2020). “Chapter Eight - chemical changes in wood caused by decay fungi,” in Wood microbiology. Editors R. A. Zabel, and J. J. Morrell Second Edition (San Diego: Academic Press), 215–244. doi:10.1016/B978-0-12-819465-2.00008-5
Zhang, C. (2014). “23 - life cycle assessment (LCA) of fibre reinforced polymer (FRP) composites in civil applications,” in Eco-efficient construction and building materials. Editors F. Pacheco-Torgal, L. F. Cabeza, J. Labrincha, and A. de Magalhães (Sawston, United Kingdom: Woodhead Publishing), 565–591. doi:10.1533/9780857097729.3.565
Zhang, Q., Cai, H., Yang, K., and Yi, W. (2017). Effect of biochar on mechanical and flame retardant properties of wood – plastic composites. Results Phys. 7, 2391–2395. doi:10.1016/j.rinp.2017.04.025
Keywords: biodegradation, reinforced bio-composites, biochar, weight loss, color stability, hydrophobicity
Citation: Zouari M, Mikuljan M and Schwarzkopf M (2023) Impact of biochar on the fungal decay resistance of hemp-polylactic acid composites against wood basidiomycetes. Front. Mater. 10:1129303. doi: 10.3389/fmats.2023.1129303
Received: 21 December 2022; Accepted: 16 February 2023;
Published: 27 February 2023.
Edited by:
Zhongwei Zhang, Beijing Institute of Technology, ChinaReviewed by:
Senthil Muthu Kumar Thiagamani, Kalasalingam University, IndiaApri Heri Iswanto, University of North Sumatra, Indonesia
Copyright © 2023 Zouari, Mikuljan and Schwarzkopf. This is an open-access article distributed under the terms of the Creative Commons Attribution License (CC BY). The use, distribution or reproduction in other forums is permitted, provided the original author(s) and the copyright owner(s) are credited and that the original publication in this journal is cited, in accordance with accepted academic practice. No use, distribution or reproduction is permitted which does not comply with these terms.
*Correspondence: Mariem Zouari, bWFyaWVtLnpvdWFyaUBpbm5vcmVuZXcuZXU=