- 1Materiales y Tecnologias para Energia, Instituto Politecnico Nacional, Ciudad de Mexico, Mexico
- 2Department of Aircraft Maintenance and Repair, School of Civil Aviation, Fırat University, Elazığ, Turkey
- 3UNINDETEC, Polígono Naval, Antón Lizardo, Mexico
- 4Universidad Marista de Mérida, Mérida, Mexico
- 5Department of Materials, The University of Manchester, Manchester, United Kingdom
Graphene oxide, as a nanoscopic platform for functional materials, has been extensively studied for several applications. The present Mini Review stresses the collaborative research in graphene-oxide materials pivoted from the Group of Materials and Technologies for Energy, Health, and Environment at an Instituto Politecnico Nacional unit in Tamaulipas, in Northeastern Mexico, with Mexican, Turkish, and British collaborators. This review covers the recent works on photovoltaic and photocatalytic materials, coatings for thermonuclear reactors, and composites and metamaterials for military and aeronautic applications.
1 Introduction: International collaborations in graphene oxide materials from Northeastern Mexico
The chemical versatility of carbon allotropes allows macroscopic forms such as activated carbons, graphite, or diamond, as well as nano-sized ones such as fullerene, nanotubes, and graphene, to be applied in energy, health, environment, electronics, communications, and so on. Within this wealth of forms and applications, graphene materials were placed in the spotlight after the seminal work of Geim and Novoselov, who first isolated and tested graphene and their related materials, paving the way for a myriad of applications which nowadays, are transiting into commercial technologies in coatings, energy storage, polymer composites and many more. While Europe supported the greatest institutionalized effort in developing the whole graphene value chain, from basic research to the creation of novel industries, with a budget of ca. 1 billion euro (Graphene research, 2023), in America, several research groups and startups across the continent are developing novel applications as well as new products based on graphene and its derivatives, which comprise a market around 270 million of dollars (Graphene Market Size, 2023). Our group at Instituto Politecnico Nacional unit in the Northeastern state of Tamaulipas, Mexico (Figure 1A), develops graphene oxide-based materials for photovoltaics, photocatalytic hydrogen generation, coatings for fusion plasma-facing materials, and composites and metamaterials for aeronautics and for electromagnetic interference, in collaboration with the Mexican Navy, Mexican universities, as well as with partners in Türkiye and United Kingdom. This initiative and broad network provided the present research consortium with the expertise in practical applications and accelerated testing of the graphene-engineered materials under realistic environmental conditions. These applications are summarized in the present MiniReview, to stress the potential of carbon-related collaborative work led from America and the perspectives for further development in the field.
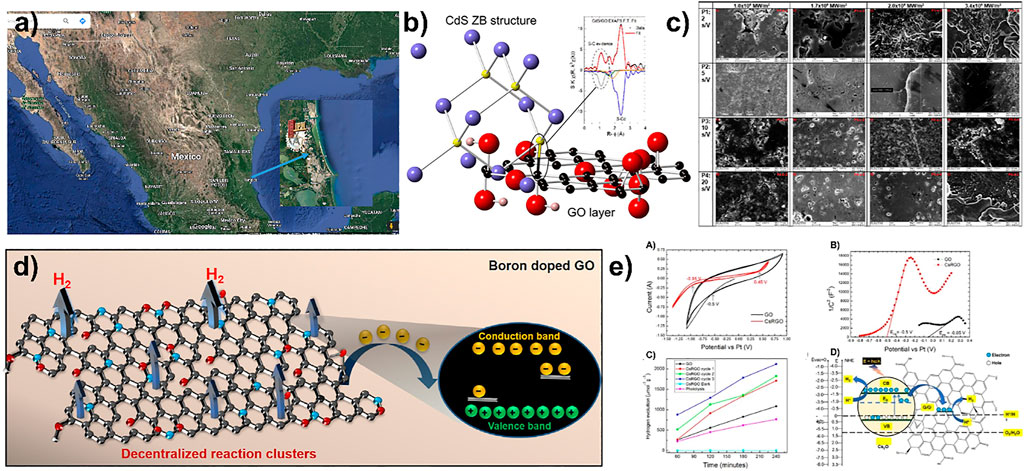
FIGURE 1. (A) Location of CICATA Altamira in northeastern Mexico; (B) Scheme of the bonding formation between S and C, derived from EXAFS data. Reprinted with permission from (Colina-Ruiz et al., 2019), Copyright (2019) American Chemical Society; (C) SEM micrographs of GO/Nb samples irradiated at increasing laser fluences from left to right, and increasing deposition times from top to bottom. Reproduced from (Tolentino-Hernandez et al., 2022), Copyright (2022), with permission from Elsevier; (D) Formation of decentralized reaction clusters in GB for photocatalytic hydrogen evolution, Copyright (2020), with permission from Elsevier (Gnanaseelan et al., 2022a). (E) Cyclic voltammogram (A), impedance spectra (B), accumulative hydrogen evolution under UV light (C) and electronic band diagram (D) of Cs-decorated GO, Copyright (2022), with permission from Elsevier (Gnanaseelan et al., 2022b).
2 Graphene oxide materials for clean energy applications
2.1 Photovoltaic and photoelectrochemical cells
The development of more efficient solar and photoelectrochemical cells lead to hybrid materials based on cadmium chalcogenides such as CdS, CdSSe, and CdTe, in graphene oxide (GO) or reduced graphene oxide (rGO) matrix (Kagkoura et al., 2017). Hybrid CdS1-xSex/GO multilayers prepared by facile and low-cost methods present an intriguing optoelectronic behavior, such as the variation in the density of states measured by X-ray absorption near edge structure (XANES) and the formation of new interband states (IS) related to the Se/S-GO skeleton interaction (Colina-Ruiz et al., 2019). The materials present a high but damped photocurrent vs. potential response for CdS/GO and CdS0.5Se0.5-GO multilayers, which is attributed to the electronic structure modification of the multilayers due to the S-deficiency or Cd-excess depending on the x-value. As shown in Figure 1B), the direct bond of nanoparticles and GO matrix benefits the interfacial charge transfer from the conduction band and IS to graphene sheets, promoting the electron-hole pairs separation and enhancing the photocurrent activity (Colina-Ruiz et al., 2019; Wei et al., 2019). Our current work on this topic includes the study of other systems such as ZnS1-xSex/GO and possible application to photoelectrochemical cells that will exploit quasi-static photocurrent under applied potential for sustained water splitting.
2.2 Plasma-facing components for fusion tokamaks
The research in structural materials for nuclear fusion reactor technology is attracting wider attention due to the near commissioning of the International Thermonuclear Reactor (ITER), and other fusion initiatives worldwide such as the recently-launched STEP (Spherical Tokamak for Energy Production) programme in the United Kingdom. Materials for the fuel vessel of a fusion nuclear reactor must withstand a very aggressive and extreme environment. Tungsten, the element with the highest melting point, is the frontrunner candidate to be used as the material facing the hot fusion plasma, although its innate embrittlement, exhacerbated by intense radiation fluxes from the plasma, and surface erosion with the formation of surface nano-structure and potential plasma contamination over time, are key challenges to be overcome before moving into higher technology readiness levels (Pitts et al., 2011). Graphene-based materials have been proposed as W-based thermal/radiation barrier coatings for plasma facing components (PFCs), due to their reduced pore size, good radiation tolerance and high thermal conductivity, which would serve as a heat-dissipating and damage barrier against He ions from the deuterium-tritium plasma reaction, therefore potentially extending the safe lifetime of those PFC under service local conditions (Bolt et al., 2004). GO is an alternative for coatings in nuclear metals and alloys, due to the improved adherence on the metallic substrate facilitiated by the oxygen groups present in the GO structure. GO/metal films can be prepared by electrophoretic deposition; during the electrophoresis, GO is reduced to rGO, which improves electrical and thermal conductivity properties (Tolentino-Hernandez et al., 2022). In (8) rGO films were tested against high-power laser irradiation, as a simulant to high heat fluxes expected in (Figure 1C) service PFCs. Figure 1B shows the rGO protection imparted to Nb substrates against laser irradiation with different fluences and exposure times; rGO presented very good heat dissipation properties and is evident that the thermal conductivity relies on the electrophoretic deposition conditions during manufacture (Tolentino-Hernandez et al., 2022). Future activities within this research framework will include the assessment of the coating behaviour under high heat and particles fluxes simulant of predicted tokamak reactor conditions, as well as the deposition and testing of GO coatings onto novel multilayered metallic materials.
2.3 Photocatalytic hydrogen generation
GO has a unique graphene-like quasi-two-dimensional structure with covalently attached oxygen functional groups (carbonyl, epoxy, hydroxyl). These groups were modified by doping and co-doping with nitrogen and/or boron using hydrazine and NaBH4, respectively (Gnanaseelan et al., 2022a), which changed the optical, electrical, and photocatalytic properties of GO. Boron-doped GO (GB) is more resistive than GO, whereas optical and photocatalytic properties were significantly improved, because the formation of decentralized reaction clusters on the graphene plane of GB, which improves the light absorption coefficient, leads to the formation of inter-gap states, and reduced charge recombination. These clusters spread across the graphene plane and produced (Figure 1D) hydrogen independently (Figure 1C). The hydrogen evolution rate of GB was 1,704 µmolg−1h−1 at the end of 4 h (Gnanaseelan et al., 2022a).
A novel material, Cs-decorated reduced GO, was reported (Gnanaseelan et al., 2022b) for the enhancement of photocatalytic hydrogen generation. The incorporation of Cs in graphene led to the formation of a Cs2O-like structure due to its replacement of hydrogen in hydroxyl and carboxyl groups. The material exhibited an electrical conductivity of 10−4 S cm−1, and a direct band gap of 2.85 eV. The hydrogen evolution rate was 2,000 μmol g−1 with improved activity after three cycles of H2 generation, (Figure 1E) which could make this material a game-changer for this application (Gnanaseelan et al., 2022b).
Other studied composites were MoS2-TiO2-rGO (MTG) and CeO2-Ce2Ti3O8.7-TiO2-rGO (CTG) (Gnanaseelan et al., 2020), synthesized after a KOH pre-treatment on TiO2 nanoparticles to form K-titanate which transformed to Ce-titanate upon hydrothermal treatment. The synergy of improved optical absorption, reduced charge recombination and effective charge separation positively impacted the performance of photocatalysts. The hydrogen evolution rate of MTG and CTG were 363.83 µmolg−1h−1 and 355.9 µmolg−1h−1 respectively (Gnanaseelan et al., 2020).
Synchrotron studies of CTG and Cs-GO are programmed to understand the relation between the photocatalytic activity and the material structure variations during operation.
3 Graphene oxide materials for military and aeronautic applications
3.1 Electromagnetic interference shielding
Electromagnetic waves such as radio, telephone, Wi-Fi, radar system, etc. (Liang et al., 2021) could adversely affect electric-electronic devices, producing corrupt signals, sudden power cuts, power fluctuations, and damaged or unusable electronic devices (Geetha et al., 2009). Therefore, electromagnetic interference (EMI) shielding is required in commercial and scientific electronic instruments as well as in aerospace and military systems. Reflection and absorption are two key factors in EMI shielding, and the interaction between the conductive parts of the material and the impinging electromagnetic field lead to the reflection mechanism, while the presence of materials with high electrical conductivity and high magnetic permeability increase the absorption efficiency (Xu et al., 2016; Ruiz-Perez et al., 2022a). Metal-based EMI shielding has the disadvantage of high density, bulky, costly, and narrow absorption band (Kim et al., 2010), but also, due to its reflection-based shielding of EM waves, they increase the possibility of self-disturbing the device by secondary electromagnetic wave pollution (Ji et al., 2018). Therefore, electromagnetic wave absorbers (EWA’s) are currently among the most studied materials as they can be lightweight, thin, flexible, efficient, with electrical and magnetic properties, with a broadband attenuation response and exceptional absorption ability. Magnetic GO, obtained by the incorporation of magnetic nanoparticles (Fe3O4, Ni, Co) has been used to create wearable devices such as cotton fibers for protection in the X-band (8.2–12.4 GHz) (Gupta et al., 2020). EWA’s potential applications include, in addition, the development of coatings in the military field to absorb radar waves of equipment or vehicles and reduce their detectability (Sahoo et al., 2023). A recent publication presents an in silico study of a metamaterial coating with modulable absorption in the X-band frequency range. The unit of this metamaterial consists of a chessboard structure with squares of different heights, which are made of an rGO/Fe3O4/Ppy composite (Ruiz-Perez et al., 2022b). A new concept to evaluate the attenuation response of materials, the effective loss reflection (RL), which considers the area under the curve of the attenuation response graph greater than −10 dB in the full evaluated frequency range is introduced. A maximum reflection loss value of −35.83 dB @ 10 GHz with effective RL of −37.45 dB/GHz was obtained, in contrast to the effective RL of −28.77 dB/GHz of the 3 mm layer coating (Ruiz-Perez et al., 2022b). In addition, the metamaterial allowed the modulation of the reflection loss frequency throughout the range of interest covering an interesting area in the military detection field of the Mexican Navy. The near perspective is to manufacture the reported structures to test in the military environment.
3.2 Aeronautics
Many complex factors have an influential role to achieve the desired EMI-shielding efficiency for carbon nanoparticle-filled conductive polymer composites (CPCs). First, the type, morphology, size, amount, and distribution of carbon nanoparticles such as graphene, rGO, CNT, and MWCNT highly affect the conductivity properties of CPCs. In polymer composites containing carbon nanoparticles, conductive, inorganic filler materials tend to be irregularly dispersed and agglomerated in the organic phase polymer matrix. This will cause interfacial interactions between the carbon filler and the matrix in the composites which results in poor reinforcement effect and shielding efficiency. The uniform dispersion of graphene nanoparticles in the epoxy matrix without aggregation and an optimized dispersion can be achieved by overcoming the Van der Waals forces that hinder the dispersion of graphene by covalent and non-covalent modifications (Kaftelen-Odabaşı et al., 2022; Kaftelen-Odabaşı et al., 2023). Significant improvements were achieved in the electrical conductivity and flexural strength of CFRP containing reduced graphene oxide dispersed with polyvinylpyrrolidone. Maximum strength (550 ± 43 MPa) and ductility (2.65% ± 0.21%) were obtained for 0.15 wt% rGO/epoxy CF composites with PVP modification (Kaftelen-Odabaşı et al., 2022). Moreover, PVP-modified rGO composites showed approximately 27 and 36-times improvement in surface and T-T-T electrical conductivity, respectively, compared to those without graphene filler (Kaftelen-Odabaşı et al., 2022). The next studies to be performed are the deicing properties and lighting protection of these composites in real aeronautic structures. Figure 2 presents a summary of the applications discussed in this section.
4 Concluding remarks
Recent advances and perspectives in carbon materials for photovoltaics, photocatalytic H2 production, coatings for thermonuclear materials, nano and metamaterials for EMI insulation, and aeronautics for civil and military applications done under a collaborative scheme, were described. This MiniReview highlights our overseas collaborations by leveraging the materials synthesis capabilities of our Mexico-based Group, with other teams in the United Kingdom and Türkiye who have access to advanced materials characterization capabilities and practical testing facilities, together with relevant expertise for the intended applications, and the formation of Ph.D. students, which act as co-authors of the present work. The collaboration has already produced some potential game-changing materials such as CTG, Cs-GO, rGO/coatings, chessboard-like nanostructured metamaterials. The rGO-based composites will be systematically studied in real-life environments in the near future. Nevertheless, for such applications, the mass production of good quality, repeatable graphene materials is a challenge yet to be overcome with a strong interaction between academics and industry with government support.
Author contributions
FC-B and HK-O conceptualized the work. FC-B wrote the introduction and concluding remarks and got financial support. HK-O wrote the aeronautics section and revised the references. RVT-H, FR-P, and NG wrote the energy, hydrogen, and military sections, prepare the figures and got reproduction permissions. SKK, SMLE, EJ-M, and FJE-F revised the partial and final drafts. All authors agreed with the final version. All authors have read and agreed to the published version of the manuscript. All authors contributed to the article and approved the submitted version.
Funding
This work was financed by Instituto Politecnico Nacional, through SIP-IPN aid.
Conflict of interest
The authors declare that the research was conducted in the absence of any commercial or financial relationships that could be construed as a potential conflict of interest.
Publisher’s note
All claims expressed in this article are solely those of the authors and do not necessarily represent those of their affiliated organizations, or those of the publisher, the editors and the reviewers. Any product that may be evaluated in this article, or claim that may be made by its manufacturer, is not guaranteed or endorsed by the publisher.
References
Bolt, H., Barabash, V., Krauss, W., Linke, J., Neu, R., Suzuki, S., et al. (2004). Materials for the plasma-facing components of fusion reactors. J. Nucl. Mater [Internet] 329, 66–73. –333:66–73. doi:10.1016/j.jnucmat.2004.04.005
Colina-Ruiz, R. A., Tolentino-Hernández, R. V., Guarneros-Aguilar, C., Mustre de León, J., Espinosa-Faller, F. J., and Caballero-Briones, F. (2019). Chemical bonding and electronic structure in CdS/GO and CdSSe/GO multilayer films. J. Phys. Chem. C 123, 13918–13924. doi:10.1021/acs.jpcc.9b03328
Geetha, S., Satheesh Kumar, K. K., Rao, C. R. K., Vijayan, M., and Trivedi, D. C. (2009). EMI shielding: Methods and materials-A review. J. Appl. Polym. Sci. [Internet] 112 (4), 2073–2086. doi:10.1002/app.29812
Gnanaseelan, N., Latha, M., Mantilla, A., Sathish-Kumar, K., and Caballero-Briones, F. (2020). The role of redox states and junctions in photocatalytic hydrogen generation of MoS2-TiO2-rGO and CeO2-Ce2Ti3O8.7-TiO2-rGO composites. Mat. Sci. Semicond. process. 118, 105185. doi:10.1016/j.mssp.2020.105185
Gnanaseelan, N., Marasamy, L., Mantilla, A., Kamaraj, S. K., Espinosa-Faller, F. J., and Caballero-Briones, F. (2022). Cesium-decorated reduced graphene oxide for photocatalytic hydrogen generation. Mater Lett. 314, 131864. January. doi:10.1016/j.matlet.2022.131864
Gnanaseelan, N., Marasamy, L., Mantilla, A., Kamaraj, S. K., Espinosa-Faller, F. J., and Caballero-Briones, F. (2022). Exploring the impact of doping and co-doping with B and N on the properties of graphene oxide and its photocatalytic generation of hydrogen. Int. J. Hydrogen Energy 47 (97), 40905–40919. doi:10.1016/j.ijhydene.2022.08.234
Graphene Market Size, (2023). Growth, share | research report [internet]. Available from: https://www.fortunebusinessinsights.com/graphene-market-102930.
Graphene research, (2023). Innovation and collaboration | graphene flagship [internet]. Available from: https://graphene-flagship.eu/.
Gupta, S., Chang, C., Anbalagan, A. K., Lee, C.-H., and Tai, N.-H. (2020). Reduced graphene oxide/zinc oxide coated wearable electrically conductive cotton textile for high microwave absorption. Compos Sci. Technol. 188, 107994. [Internet]. doi:10.1016/j.compscitech.2020.107994
Ji, H., Zhao, R., Zhang, N., Jin, C. X., Lu, X. F., and Wang, C. (2018). Lightweight and flexible electrospun polymer nanofiber/metal nanoparticle hybrid membrane for high-performance electromagnetic interference shieldingflexible electrospun polymer nano fiber/metal nanoparticle hybrid membrane for high-performance electromagnetic interference shielding. NPG Asia Mater [Internet] 10, 749–760. doi:10.1038/s41427-018-0070-1
Kaftelen-Odabaşı, H., Odabaşı, A., Caballero-Briones, F., Arvizu-Rodriguez, L. E., Özdemir, M., and Baydoğan, M. (2022). Effects of polyvinylpyrrolidone as a dispersant agent of reduced graphene oxide on the properties of carbon fiber-reinforced polymer composites. J. Reinf. Plast. Compos [Internet], 073168442211455. doi:10.1177/07316844221145560
Kaftelen-Odabaşı, H., Odabaşı, A., Özdemir, M., and Baydoğan, M. (2023). A study on graphene reinforced carbon fiber epoxy composites: Investigation of electrical, flexural, and dynamic mechanical properties. Polym. Compos [Internet] 44, 121–135. doi:10.1002/pc.27031
Kagkoura, A., Skaltsas, T., and Tagmatarchis, N. (2017). Transition-metal chalcogenide/graphene ensembles for light-induced energy applications. Chem-A Eur. J. 23, 12967–12979. doi:10.1002/chem.201700242
Kim, B. R., Lee, H. K., Kim, E., and Lee, S.-H. (2010). Intrinsic electromagnetic radiation shielding/absorbing characteristics of polyaniline-coated transparent thin films. Synth. Met. [Internet] 160 (17–18), 1838–1842. –42. doi:10.1016/j.synthmet.2010.06.027
Liang, C., Gu, Z., Zhang, Y., Ma, Z., Qiu, H., and Gu, J. (2021). Structural design strategies of polymer matrix composites for electromagnetic interference shielding: A review. Nano-Micro Lett. [Internet] 13 (1), 181. doi:10.1007/s40820-021-00707-2
Pitts, R. A., Carpentier, S., Escourbiac, F., Hirai, T., Komarov, V., Kukushkin, A. S., et al. (2011). Physics basis and design of the ITER plasma-facing components. J. Nucl. Mater [Internet] 415 (1), S957–S964. doi:10.1016/j.jnucmat.2011.01.114
Ruiz-Perez, F., Estrada, S. M. L., and Briones, F. C. (2022). Tunable, wideband X-band microwave absorbers using variable chessboard surfaces. IEEE Lett. Electromagn. Compat. Pract. Appl. 4, 44–46. doi:10.1109/lemcpa.2022.3141775
Ruiz-Perez, F., López-Estrada, S. M., Tolentino-Hernández, R. V., and Caballero-Briones, F. (2022). Carbon-based radar absorbing materials: A critical review. J. Sci. Adv. Mater Devices [Internet] 7 (3), 100454. Disponible en. doi:10.1016/j.jsamd.2022.100454
Sahoo, P., Saini, L., and Dixit, A. (2023). Microwave-absorbing materials for stealth application: A holistic overview. Oxf. Open Mater Sci. [Internet] 3 (1). doi:10.1093/oxfmat/itac012
Tolentino-Hernandez, R. V., Garcia-Pastor, F. A., Baez-Medina, H., Jimenez-Melero, E., and Caballero-Briones, F. (2022). Structural damage in graphene oxide coatings onto Nb substrates upon laser irradiation. Surf. Coatings Technol. [Internet] 431, 128013. doi:10.1016/j.surfcoat.2021.128013
Wei, X.-N., Ou, C.-L., Guan, X.-X., Peng, Z.-K., and Zheng, X.-C. (2019). Facile assembly of CdS-reduced graphene oxide heterojunction with enhanced elimination performance for organic pollutants in wastewater. Appl. Surf. Sci. 469, 666–673. PII: S0169433218331805. doi:10.1016/j.apsusc.2018.11.102
Keywords: graphene oxide, supercapacitors, photocatalytic hydrogen production, aeronautics, military
Citation: Caballero-Briones F, Kaftelen-Odabaşı H, Gnanaseelan N, Ruiz-Perez F, Tolentino-Hernandez RV, Kamaraj SK, Lopez Estrada SM, Espinosa-Faller FJ and Jimenez-Melero E (2023) International research in graphene-oxide based materials for net-zero energy, military and aeronautic applications catalysed by Tamaulipas, Mexico: a mini review. Front. Mater. 10:1192724. doi: 10.3389/fmats.2023.1192724
Received: 23 March 2023; Accepted: 03 May 2023;
Published: 15 May 2023.
Edited by:
Ismael Alejandro Aguayo-Villarreal, University of Colima, MexicoReviewed by:
Pallab Bhattacharya, National Metallurgical Laboratory (CSIR), IndiaSatish Teotia, NIMS University, India
Copyright © 2023 Caballero-Briones, Kaftelen-Odabaşı, Gnanaseelan, Ruiz-Perez, Tolentino-Hernandez, Kamaraj, Lopez Estrada, Espinosa-Faller and Jimenez-Melero. This is an open-access article distributed under the terms of the Creative Commons Attribution License (CC BY). The use, distribution or reproduction in other forums is permitted, provided the original author(s) and the copyright owner(s) are credited and that the original publication in this journal is cited, in accordance with accepted academic practice. No use, distribution or reproduction is permitted which does not comply with these terms.
*Correspondence: F. Caballero-Briones, ZmNhYmFsbGVyb0BpcG4ubXg=; H. Kaftelen-Odabaşı, aGtvZGFiYXNpQGZpcmF0LmVkdS50cg==