- 1Department of Pharmaceutical Chemistry, College of Pharmacy, Mustansiriyah University, Baghdad, Iraq
- 2School of Biosciences, College of Health and Life Sciences, Aston University, Birmingham, United Kingdom
- 3Department of Chemistry and Biochemistry, College of Medicine, University of Kerbala, Karbala, Iraq
Nowadays, various factors enhance the resistance of some microbes to antibiotics. Irrational antibiotic use is considered an example of such factors. Therefore, the synthesis and reporting of heterocyclic compounds with multiple biological properties are of considerable value. Ciprofloxacin is an antibiotic used to treat infections. New amidification and esterification derivatives of ciprofloxacin were synthesized. Their structure was identified and confirmed using both proton and carbon-13 nuclear magnetic resonance, mass spectrometry, and elemental analysis. With the employment of MTT (3-(4, 5-dimethylthiazol-2-yl)-2,5-diphenyl-terazoliumbromide) methods, in vitro anticancer activity was evaluated. The utilization of clinical and laboratory standards institute (CLSI) instructions was involved in the in vitro antimicrobial activity evaluation of the newly synthesized derivatives. In terms of anticancer activity, cell proliferation, viability, and IC50, as well as antimicrobial activities, IZD (Inhibition Zone Diameter), MIC (Minimum Inhibitory Concentrations), MBC (Minimum Bactericidal Concentrations), and MFC (Minimum Fungicidal Concentration) were measured and reported. The lowest cell proliferation and viability and IC50 for 2,3-dihydroxypropyl 1-cyclopropyl-6-fluoro-4-oxo-7-(piperazin-1-yl)-1,4-dihydroquinoline-3-carboxylate (compound 5) were observed to be 27.12% and 7.83 μg/mL. In antimicrobial activity, the lowest MIC for 2,3-dihydroxypropyl 7-(4-benzoylpiperazin-1-yl)-1-cyclopropyl-6-fluoro-4-oxo-1,4-dihydroquinoline-3-carboxylate (compound 6c) was observed to be 2 μg/mL (against Proteus mirabilis) and the lowest MIC for 1-cyclopropyl-6-fluoro-7-(4-(4-(methylthio)benzoyl)piperazin-1-yl)-4-oxo-1,4-dihydroquinoline-3-carboxylic acid (compound 3d) was observed to be 32 μg/mL (against Candida albicans, Aspergillus fumigatus). The effects were compared with commercially available drugs, and it was observed that some derivatives have the same efficacy as abemaciclib, which is used to treat breast cancer. Some derivatives were more effective than Cefazolin and Tolnaftate, well-known antibiotics and antifungals, respectively. Finally, a fairly clear relationship between the structure of the derivatives and their biological effectiveness was observed.
1 Introduction
A wide variety of antibiotics are used for various diseases every day worldwide. Unfortunately, the rate of improper utilization of antibiotics is increasing (Terreni et al., 2021) and this has led to the resistance of pathogenic microbial strains to many known antibiotics (Xiao et al., 2022). For this reason, there have been reports of modification to and changing the structure of antibiotics and the synthesis of new compounds (Das et al., 2020; Qu et al., 2023). For example, manganese ferrite–modified biochar from vinasse has been reported for enhanced adsorption of fluoroquinolone antibiotics. The fluoroquinolones kill bacteria by preventing DNA replication. Generally, the fluoroquinolone antibiotic inhibits the ligase activity of type II topoisomerases. With the ligase activity disrupted, DNA with single- and double-strand breaks lead to cell death (Aldred et al., 2014). In another proposed mechanism, fluoroquinolone antibiotics cause the oxidation of guanine nucleotides in the bacterial nucleotide pool, which contributes to their cytotoxicity (Foti et al., 2012). Delafloxacin, gemifloxacin, levofloxacin, moxifloxacin, and norfloxacin (Figure 1) are other examples of fluoroquinolone antibiotics (Hooper, 2001).
Ciprofloxacin is a fluoroquinolone antibiotic. Ciprofloxacin is an antibiotic used to treat infections, including joint and bone infections, intra-abdominal infections, certain types of infectious diarrhea, respiratory tract infections, skin infections, typhoid fever, and urinary tract infections. In the structure of some fluoroquinolone antibiotics such as ciprofloxacin, levofloxacin, and norfloxacin, two heterocyclic compounds of 1,4-dihydroquinoline and piperazine are present (Zhanel et al., 2006; Uncu et al., 2019; Kudiyarova et al., 2021). The bicyclic heterocyclic compound of quinoline consists of a benzene ring connected with a pyridine ring. Derivatives of quinoline are abundantly found in nature, such as Galipea, Cinchona, Papaver, etc. In addition to Ciprofloxacin, commercial drugs such as Tacrine, Mefloquine, Bosutinib, etc., (Figure 2). Have quinoline derivatives in their structure.
Several other biological properties of quinoline-containing heterocyclic compounds have been reported (Matada et al., 2021). Some biological properties, such as anti-bacterial and anti-fungal activity (Dib et al., 2021; Jamshidi et al., 2022), anti-cancer activity (Gao et al., 2019), anti-plasmodial activities (Sharma et al., 2023), etc., have been reported from compounds containing this heteroatom. For example, in 2021, sulfur-containing quinoline derivatives (Figure 3) were reported that were effective against bacterial species such as S. pneumonia, B. subtilis, and E. coli (El-Shershaby et al., 2021).
Benzothiazolyl quinolone derivatives (Figure 4) are another polycyclic heterocyclic compounds that were reported in 2021 as newly synthesized heterocyclic structures effective against E. coli and P. aeruginosia bacterial species (Bolakatti et al., 2021).
Pyrazine is another bioactive compound that (Ferreira and Kaiser, 2012), in addition to Ciprofloxacin, is also present in the structure of Bosutinib. Medicines such as pyrazinamide (Figure 5), paritaprevir, bortezomib, amiloride, etc., have a pyrazine in their structure (Huigens et al., 2022; Tambat et al., 2022). Other pharmacological activities, such as anti-inflammatory activity (Shashikant et al., 2022), antimicrobial activity (Schneider et al., 2022), anti-cancer activity (Tantawy et al., 2020), etc., have been reported from synthetic compounds containing pyrazine.
Considering the biological properties of Ciprofloxacin, as discussed, amidification and esterification of ciprofloxacin can be performed and compounds developed with biological properties. In this study, the derivatives mentioned above were synthesized and studied on various Gram-positive and Gram-negative pathogenic bacterial agents and common pathogenic bacterial strains between aquatic animals and humans. In vitro biological studies of the synthetic compounds were also evaluated on breast cancer cells and bacterial cultures. The results of these in vitro tests showed that the synthesized amidification and esterification derivatives of ciprofloxacin have high antimicrobial and anticancer activities. By performing other supplementary tests in subsequent studies, such as in silico studies, in vivo tests, cytotoxicity texts, etc., and if the results are acceptable, amidification and esterification derivatives of ciprofloxacin can be suitable candidates for developing effective antibiotic and anticancer drugs.
2 Experimental
2.1 Chemicals and reagents
All solvents, chemicals, and consumables utilized in this study were obtained from Merck Millipore and Sigma-Aldrich.
2.2 Amidification of ciprofloxacin
An accurately weighed amount of 10 mmol Acyl chloride derivatives was added to a stirring mixture of 1.84 g of ciprofloxacin hydrochloride (5 mmol) and 2.65 g of Na2CO3 in 20 mL of tetrahydrofuran at 0°C (Table 1). The resultant mixture was continuously stirred for 1 h at 0°C. Thereafter, a yellow precipitate was observed. The column chromatography method (silica gel 60–120 mesh, a gradient solvent system of 0–20%methanol/DCM) was used for the purification of the synthesized derivatives.
7-(4-acetylpiperazin-1-yl)-1-cyclopropyl-6-fluoro-4-oxo-1,4-dihydroquinoline-3-carboxylic acid (3a).
MS m/z 373 (M+). Element analysis. Calcd for C19H20FN3O4: C, 61.12. H, 5.40. N, 11.25. O, 17.14. Found: C, 61.15. H, 5.38. N, 11.24. O, 17.17. 1H NMR (DMSO-d6) δ = 1.05-4.07 (m, 2H), 1.38-1.42 (m, 2H), 2.18 (s, 3H), 3.36 (t, J = 8.0 Hz, 4H), 3.62 (t, J = 8.0 Hz, 4H), 4.21-4.25 (m, 1H), 6.17 (s, 1H), 7.86 (s, 1H), 8.25 (s, 1H), 12.37 (s, 1H). 13C NMR (DMSO-d6) δ = 7.8, 21.9, 36.4, 44.7, 52.8, 103.2, 109.5, 111.3, 116.1, 135.8, 146.1, 147.8, 152.4, 165.4, 168.7, 177.3.
1-cyclopropyl-6-fluoro-4-oxo-7-(4-pivaloylpiperazin-1-yl)-1,4-dihydroquinoline-3-carboxylic acid (3b).
MS m/z 415 (M+). Element analysis. Calcd for C22H26FN3O4: C, 63.60. H. 6.31. N, 10.11. O, 15.40. Found: C, 63.62. H, 6.38. N, 10.10. O, 15.46. 1H NMR (DMSO-d6) δ = 1.04, 1.08 (m, 2H), 1.25 (s, 9H), 1.35-1.39 (m, 2H), 3.31 (t, J = 7.5 Hz, 4H), 3.57 (t, J = 8.0 Hz, 4H), 4.15-4120 (m, 1H), 6.09 (s, 1H), 7.82 (s, 1H), 8.17 (s, 1H), 12.32 (s, 1H). 13C NMR (DMSO-d6) δ = 7.5, 28.3, 35.8, 38.3, 45.1, 53.2, 102.6, 109.7, 112.4, 115.8, 134.9, 147.3, 148.4, 152.7, 165.7, 176.3, 177.5.
7-(4-benzoylpiperazin-1-yl)-1-cyclopropyl-6-fluoro-4-oxo-1,4-dihydroquinoline-3-carboxylic acid (3c).
MS m/z 435 (M+). Element analysis. Calcd for C24H22FN3O4: C, 66.20. H, 5.09. N, 9.65. O, 14.70. Found: C, 66.18. H, 5.07. N, 9.69. O, 14.69. 1H NMR (DMSO-d6) δ = 1.01-1.05 (m, 2H), 1.32-1.40 (m, 2H), 3.28 (t, J = 8 Hz, 4H), 3.47 (t, J = 8.4 Hz, 4H), 4.12-4.17 (m, 1H), 6.04 (s, 1H), 7.52-7.58 (m, 3H), 7.96-8.05 (m, 3H), 8.24 (s, 1H), 12.42 (s, 1H). 13C NMR (DMSO-d6) δ = 7.1, 35.4, 49.6, 52.9, 101.9, 109.3, 112.7, 115.3, 126.7, 128.1, 129.5, 134.3, 135.2, 146.9, 148.1, 152.3, 166.3, 168.6, 177.9.
1-cyclopropyl-6-fluoro-7-(4-(4-(methylthio)benzoyl)piperazin-1-yl)-4-oxo-1,4-dihydroquinoline-3-carboxylic acid (3d).
MS m/z 481 (M+). Element analysis. Calcd for C25H24FN3O4S: C, 62.36. H, 5.02. N, 8.73. S, 6.66. Found: C, 62.35. H, 5.05. N, 8.70. S, 6.69. 1H NMR (DMSO-d6) δ = 1.02-1.06 (m, 2H), 1.27-1.35 (m, 2H), 2.46 (s, 1H), 3.24 (t, J = 8.5 Hz, 4H), 3.41 (t, J = 8.5 Hz, 4H), 4.14-4.21 (m, 1H), 6.10 (s, 1H), 7.55 (d, J = 8.4 Hz, 2H), 7.91-7.99 (m, 3H), 8.41 (s, 1H), 12.36 (s, 1H). 13C NMR (DMSO-d6) δ = 7.7, 14.9, 34.8, 50.1, 53.4, 101.5, 109.1, 112.4, 115.6, 126.1, 128.4, 132.9, 134.8, 140.2, 146.4, 149.9, 153.1, 165.9, 167.8, 178.1.
2.3 Esterification and amidification of ciprofloxacin
A total of 14.7 g of ciprofloxacin hydrochloride (40 mmol) was dissolved in 40 mL of tetrahydrofuran and then 11.1 g of glycerol (120 mmol) was added to the solution (Table 2). The mixture stirred and concentrated H2SO4 (2 mL) was added dropwise. Thereafter, the mixture was refluxed for 4 h at 110°C. The column chromatography method (silica gel 60–120 mesh, a gradient solvent system of 0%–20% methanol/DCM) was used for the purification of the synthesized compounds.
2,3-dihydroxypropyl 1-cyclopropyl-6-fluoro-4-oxo-7-(piperazin-1-yl)-1,4-dihydroquinoline-3-carboxylate (5).
MS m/z 405 (M+). Element analysis. Calcd for C20H24FN3O5: C, 59.25. H, 5.97. N, 10.36. O, 19.73. Found: C, 21.18. H, 5.96. N, 10.33. O, 19.68. 1H NMR (DMSO-d6) δ = 1.03-1.09 (m, 3H), 1.29-1.34 (m, 2H), 2.75-2.79 (m, 4H), 3.44-3.52 (m, 6H), 3.98 (s, 1H), 4.12-4.31 (m, 4H), 5.73 (s, 1H), 6.08 (s, 1H), 7.99 (s, 1H), 8.57 (s, 1H). 13C NMR (DMSO-d6) δ = 7.1, 35.5, 45.4, 51.2, 63.0, 67.6, 71.1, 102.7, 110.2, 119.9, 115.3, 134.6, 144. 147.5, 152.2, 165.8, 171.4.
An accurately weighed amount of 60 mmol Acyl chloride derivatives was added to a stirring mixture of 8.1g of compound 2,3-dihydroxypropyl 1-cyclopropyl-6-fluoro-4-oxo-7-(piperazin-1-yl)-1,4-dihydroquinoline-3-carboxylate (20 mmol) and 10.5g of Na2CO3 in 40 mL of tetrahydrofuran at 0°C. The resultant solution was continuously stirred for 1 h at 0°C. The column chromatography method (silica gel 60–120 mesh, isocratic solvent system of 1:4 methanol/DCM) was used for the purification of the synthesized derivatives.
2,3-dihydroxypropyl 7-(4-acetylpiperazin-1-yl)-1-cyclopropyl-6-fluoro-4-oxo-1,4-dihydroquinoline-3-carboxylate (6a).
MS m/z 447 (M+). Element analysis. Calcd for C22H26FN3O6: C, 59.05. H, 5.86. N, 9.39. O, 21.45. Found: C, 59.04. H, 5.85. N, 9.41. O, 21.44. 1H NMR (DMSO-d6) δ = 1.01-1.05 (m, 2H), 1.30-1.33 (m, 2H), 2.14 (s, 1H), 3.31 (t, J = 8Hz, 4H), 3.53-3.57 (m, 6H), 3.65 (s, 1H), 4.15-4.35 (m, 4H), 5.79 (s, 1H), 6.03 (s, 1H), 8.02 (s, 1H), 8.62 (s, 1H). 13C NMR (DMSO-d6) δ = 7.4, 21.9, 35.2, 46.7, 51.6, 63.5, 67.1, 71.9, 102.1, 110.3, 112.6, 115.0, 134.1, 144.6, 146.9, 152.5, 165.5, 167.4, 171.9.
2,3-dihydroxypropyl 1-cyclopropyl-6-fluoro-4-oxo-7-(4-pivaloylpiperazin-1-yl)-1,4-dihydroquinoline-3-carboxylate (6b).
MS m/z 489 (M+). Element analysis. Calcd for C25H32FN3O6: C, 61.34. H, 6.59. N, 8.58. O, 19.61. Found: C, 61.35. H, 6.62. N, 8.59. O, 19.62. 1H NMR (DMSO-d6) δ = 1.03-1.09 (m, 2H), 1.23-1.35 (m, 3H), 3.28 (t, J = 7.5 Hz, 4H), 3.52-3.59 (m, 6H), 3.77 (s, 1H), 4.12-4.36 (m, 4H), 5.75 (s, 1H), 6.10 (s, 1H), 8.12 (s, 1H), 8.76 (s, 1H). 13C NMR (DMSO-d6) δ = 7.1, 27.3, 35.7, 38.2, 46.9, 53.5, 63.2, 67.7, 71.9, 101.9, 110.8, 112.1, 115.6, 134.6, 145.2, 147.6, 152.1, 165.1, 169.8, 172.9.
2,3-dihydroxypropyl 7-(4-benzoylpiperazin-1-yl)-1-cyclopropyl-6-fluoro-4-oxo-1,4-dihydroquinoline-3-carboxylate (6c).
MS m/z 509 (M+). Element analysis. Calcd for C27H28FN3O6: C, 63.65. H, 5.54. N, 8.25. O, 18.84. Found: C, 63.70. H, 5.43. N, 8.28. O, 18.86. 1H NMR (DMSO-d6) δ = 1.0-1.10 (m, 2H), 1.21-1.29 (m, 32), 3.33 (t, J = 8.4 Hz, 4H), 3.55-3.62 (m, 6H), 3.84 (s, 1H), 4.11-4.31 (m, 4H), 5.79 (s, 1H), 6.05 (s, 1H), 7.63-7.84 (m, 5H), 8.70 (s, 1H). 13C NMR (DMSO-d6) δ = 7.3, 34.9, 50.8, 53.1, 63.6, 67.1, 71.4, 10.2.3, 111.2, 116.4, 115.9, 127.2, 128.5, 129.9, 134.7, 135.1, 144.6, 147.8, 152.6, 165.4, 68.5, 171.3.
2.4 Anticancer activity tests
In the anticancer activity tests, the culture medium was Roswell Park Memorial Institute 1640 (RPMI), 10% Fetal Bovine Serum (FBS), and 200 μL of antibiotic (G/streptomycin and penicillin). The test steps are as follows (Moghaddam-manesh et al., 2021; Moghaddam-Manesh and Hosseinzadegan, 2021):
First, breast cancer cells (MCF-7) were cultured in the culture medium. 200 μL of MCF-7 with a density of 1.2 × 104 cells per well was seeded in a microplate and incubated in suitable conditions. It should be noted that the suitable conditions were 5% CO2, 37°C, and 24 h. Then, concentrations of 6.25, 12.5, 25, and 50 μg/mL of the derivatives were added to the wells and incubated for 24 and 48 h under the above conditions. After the tested times (24 and 48 h), the contents of the wells, including derivatives and culture medium, were emptied and 50 μL of MTT solution and 150 μL of culture medium were added to the wells and incubated in 5% CO2, 37°C for 4 h. Then, the culture medium and MTT were emptied and 200 μL of dimethyl sulfoxide (DMSO) was added to the wells. Finally, the absorbance of the contents of the wells at 570 nm was read using an ELISA reader.
In the test stages, at the same time as studying the derivatives, a culture medium without derivatives was used as a control. The test steps were carried out on abemaciclib, a well-known commercial MCF-7 breast cancer cells drug, to compare the properties of the compounds. It should be noted that the MTT solution contained 2 μg/mL of MTT in phosphate-buffered saline (PBS).
2.5 Antimicrobial activity tests
In antimicrobial activity tests of the synthesized derivatives for antibacterial activity, IZD, MIC, and MBC were evaluated, whereas for the antifungal activity, IZD, MIC, and MFC were evaluated. The guidelines of CLSI (the Clinical and laboratory standards institute, guidelines M07-A9, M26-A, M27-A2) were used in all the tests (Etemadi et al., 2016; Beyzaei et al., 2017). Mueller Hinton Broth and Mueller Hinton Agar were used for the antibacterial tests and Dextrose Tryptone Broth and Dextrose Tryptone Agar were used as culture media for the antifungal tests. It should be noted that the final results are the average of the results of three repetitions of the test. The test steps are as follows (Beyzaei et al., 2017; Moghaddam-Manesh et al., 2020):
First, using a spectrophotometer, a concentration of 1 × 105 colony-forming unit/mL (CFU/mL) of bacterial and fungal species was prepared in a broth culture medium. Then, concentrations of 1–2,048 μg/mL (1, 2, 4, 8, 16, and 2,048 μg/mL) of the derivatives were prepared in DMSO.
To obtain MIC, 100 μL of the derivatives, 100 μL of the broth culture medium, and 10 μL of the studied bacterial or fungal species were added to a microplate (96 well plates). Then, it was placed in a shaker incubator for a suitable time and at a suitable temperature and was slowly stirred. After the necessary time, turbidity in the well indicated growth and transparency a lack of growth. Here, we define the MIC as the first concentration after which turbidity was observed. It should be noted that the proper time for the MIC test is 48 h and the proper temperature for bacterial species is 37°C and is 27°C for fungal species.
To obtain MBC and MFC, the contents of the MIC concentration and three more concentrated concentrations were cultured on the agar culture medium and placed inside the incubator at the proper time and temperature. Then, the concentration at which bacterial or fungal species did not grow was reported as MBC or MFC. It should be noted that the appropriate time for the MBC and MFC tests is 72 h and the proper temperature for bacterial species is 37°C and is 27°C for fungal species.
To obtain IZD, the studied bacterial or fungal species were first cultured on an agar medium. Then, a disc blank was placed on them. A total of 10 μL of the concentration obtained for the MIC of the derivatives was injected on the disk blanks and was placed inside the incubator at the right time and temperature. Finally, the diameter of the halo created by the caliper was measured and reported. It should be noted that the appropriate time for the IZD test is 24 h and the proper temperature for bacterial species is 37°C and is 27°C for fungal species.
In the test stages, at the same time as studying the derivatives, the solvent (DMSO) without derivatives was used as a control. The test steps were carried out on several well-known commercial antibacterial and antifungal drugs to compare the properties of the compounds.
3 Results and discussion
3.1 Amidification and esterification of ciprofloxacin
As mentioned in the introduction, in this study, considering the bioactivity of ciprofloxacin, its amidification derivatives and esterification/amidification derivatives were synthesized and their biological properties were tested. As shown in Figure 6, from the reaction of ciprofloxacin with acyl chloride derivatives, ciprofloxacin/ciprofloxacin/amidification derivatives were synthesized. The reaction was carried out in the presence of Na2CO3 as a Brønsted–Lowry base. The structure of the derivatives was confirmed using proton nuclear magnetic resonance (1H NMR), carbon-13 nuclear magnetic resonance (13C NMR), mass spectrometry (MS), and elemental analysis (EA), the results of which are given in Sections 2, 3.
The advantages of the synthesis of the ciprofloxacin/amidification derivatives studied in this research include the synthesis of new derivatives, the use of Na2CO3 as a green catalyst, and the high efficiency of the derivatives, as shown in Figure 6.
Esterification/amidification derivatives of ciprofloxacin were synthesized (Figure 7). For this purpose, first, an esterification reaction using ciprofloxacin and glycerol in the presence of sulfuric acid as Brønsted–Lowry acid was carried out. Then, the esterified ciprofloxacin was amidified under the aforementioned conditions and using Na2CO3.
The high efficiency of the derivatives and the greenness of Na2CO3 can also be expressed here. By using 1H NMR, 13C NMR, MS, and EA, the synthesized esterification/amidification derivatives of ciprofloxacin were identified and confirmed.
3.2 Examination of anticancer activities
The anticancer activity of the synthesized derivatives and abemaciclib was tested on breast cancer cells (MCF-7). Abemaciclib, a known breast cancer drug, was used to compare the anticancer properties of the compounds. As mentioned in paragraphs 2–5, concentrations of 6.25, 12.5, 25, and 50 μg/mL of abemaciclib and compounds were investigated for 24 and 48 h. In the evaluations, the cell proliferation and viability were obtained at different concentrations for 24 and 48 h, and, finally, the IC50 value was reported (Figure 8).
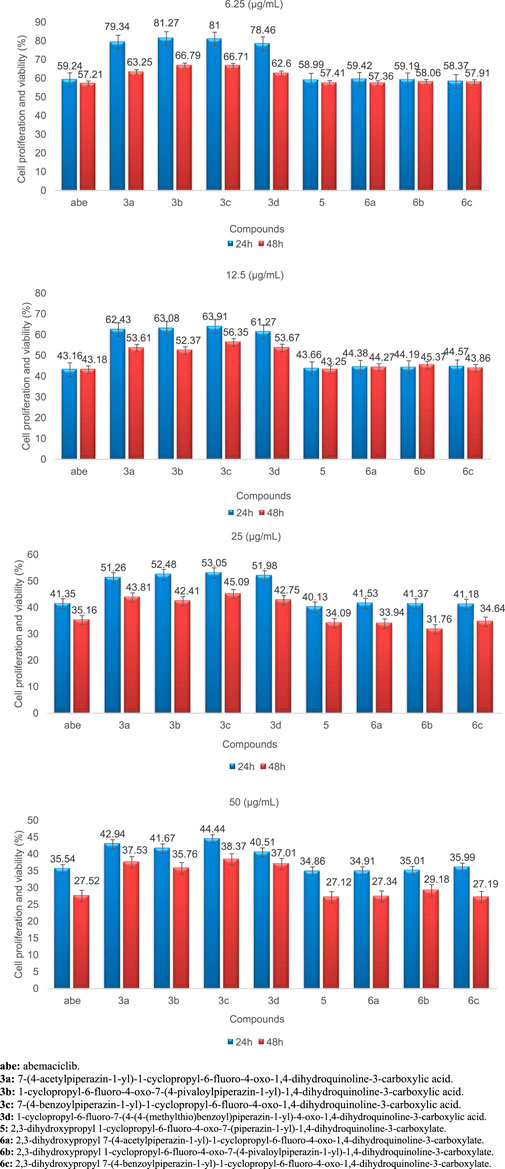
FIGURE 8. Cell proliferation and viability of abemaciclib, 3a, 3b, 3c, 3d, 5, 6a, 6b, and 6c at different concentrations and after 24 and 48 h (n = 3), ±SD.
After 24 h and at a concentration of 50 μg/mL, the cell proliferation and viability for abemaciclib, 3a, 3b, 3c, 3d, 5, 6a, 6b, and 6c were determined to be 35.54%, 42.94%, 41.67%, 44.44%, 40.51%, 34.86%, 34.91%, 35.01%, and 35.99%, respectively, compared to the control. After 48 h and at a concentration of 50 μg/mL, the cell proliferation and viability for abemaciclib and 3a, 3b, 3c, 3d, 5, 6a, 6b, and 6c were determined to be 27.52%, 37.53%, 35.76%, 38.37%, 37.01%, 27.12%, 27.34%, 29.18%, and 27.19%, respectively, compared to the control. The results showed that in both times (24 and 48 h), in the abemaciclib and synthesized derivatives, the highest cell proliferation and viability is at the concentration of 50 μg/mL. Moreover, the effectiveness of abemaciclib and the derivatives, based on cell proliferation and viability, was higher after 48 h than 24 h.
The IC50 values after 48 h for abemaciclib, 3a, 3b, 3c, 3d, 5, 6a, 6b, and 6c were determined to be 7.95 μg/mL, 22.61 μg/mL, 22.37 μg/mL, 26.15 μg/mL, 21.62 μg/mL, 7.83 μg/mL, 8.36 μg/mL, 8.49 μg/mL, and 8.96 μg/mL, respectively.
The results show that the highest anticancer property was found in 5, and that the anticancer properties of ciprofloxacin esterification/amidification derivatives (6a, 6b, and 6c) are higher than ciprofloxacin amidation derivatives (3a, 3b, 3c, and 3d). A review of the literature shows that glycerin can increase anticancer properties (Pruchnik et al., 2016; Wang et al., 2018). Therefore, based on the structure of 5, ciprofloxacin esterification/amidification derivatives, and ciprofloxacin amidation derivatives, it can be concluded that the esterification of ciprofloxacin due to the addition of glycerol increases the anticancer property. Regarding the obtained results, it was found that the anticancer properties of compound 5 and ciprofloxacin derivatization (esterification/amidation) were close to each other. Moreover, the anticancer property of ciprofloxacin amidation is almost the same. Thus, it can be concluded that the groups binding to the pyrazole ring do not play an essential role in the anticancer properties of the products.
Based on the values of cell proliferation and viability, as well as IC50 related to 5 and abemaciclib, it was found that the anticancer property of 5 is slightly higher than abemaciclib, which is a known anti-breast cancer drug.
Statistical studies were performed for abemaciclib, 3a, 3b, 3c, 3d, 5, 6a, 6b, and 6c at 24 h and 48 h and IC50 values; p-values were obtained, and the results are shown in Table 3.
Based on the results shown in the Table, it can be concluded that concentration is a critical parameter at 24 and 48 h. Therefore, the IC50 values and the effectiveness of the compounds depend on the exposure time.
As a final result, it can be said that 5 has the ability to be used as an effective anticancer drug against breast cancer, and, after other supplementary tests, it can be developed as a potent drug (Figure 9).
3.3 Examination of antimicrobial activities
The antimicrobial properties of the synthesized derivatives along with drugs 1 and 2 were investigated on four Gram-positive strains (ATCC11778/Bacillus cereus, ATCC15352/Streptococcus equinus, ATCC25729/Rhodococcus equi, and ATCC29213/Staphylococcus aureus), four Gram-negative strains (ATCC7002/Proteus mirabilis, ATCC19606/Acinetobacter baumannii, ATCC9610/Yersinia enterocolitica, and ATCC25922/Escherichia coli) and two fungal species (ATCC10231/Candida albicans and ATCC1022/Aspergillus fumigatus Fresenius). The results of the antimicrobial activity of the synthesized compounds, Gentamicin, Cefazolin, Terbinafine, and Tolnaftate, including the MIC value, MBC/MFC value, and IZD value, are shown in Tables 4–6.
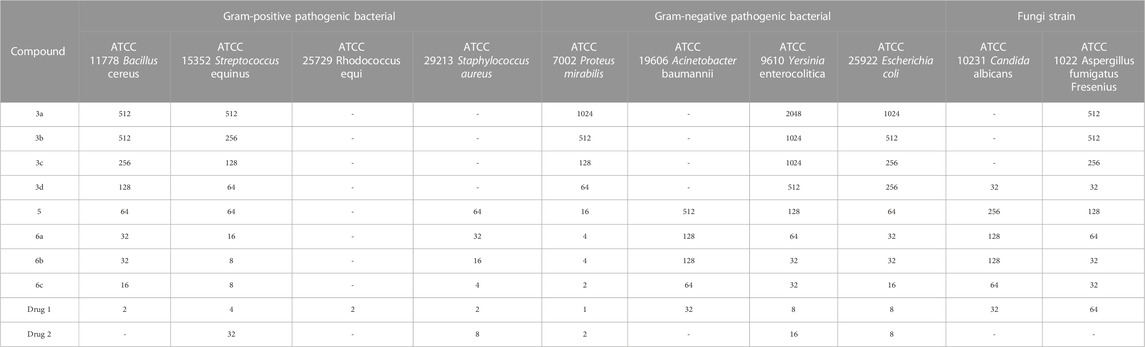
TABLE 4. MIC value (μg/mL) results in investigating the antimicrobial properties of the synthesized derivatives.
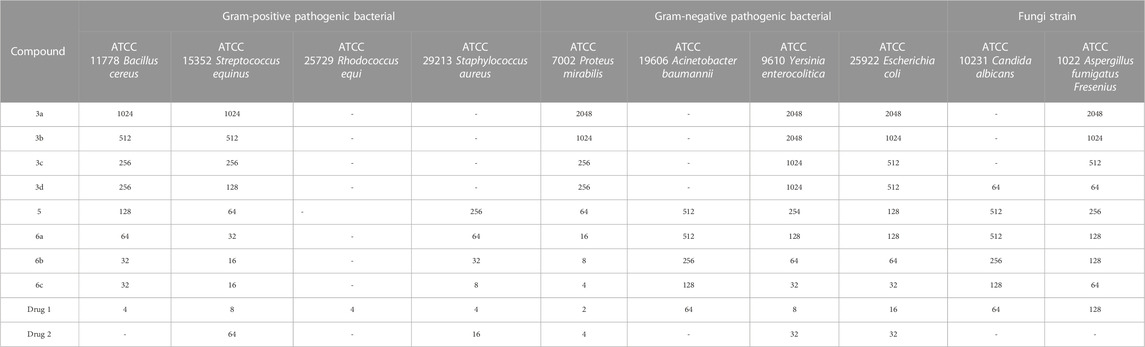
TABLE 5. MBC (μg/mL) value for bacterial strains and MFC (μg/mL) value for fungi strains results in investigating the antimicrobial properties of the synthesized derivatives.
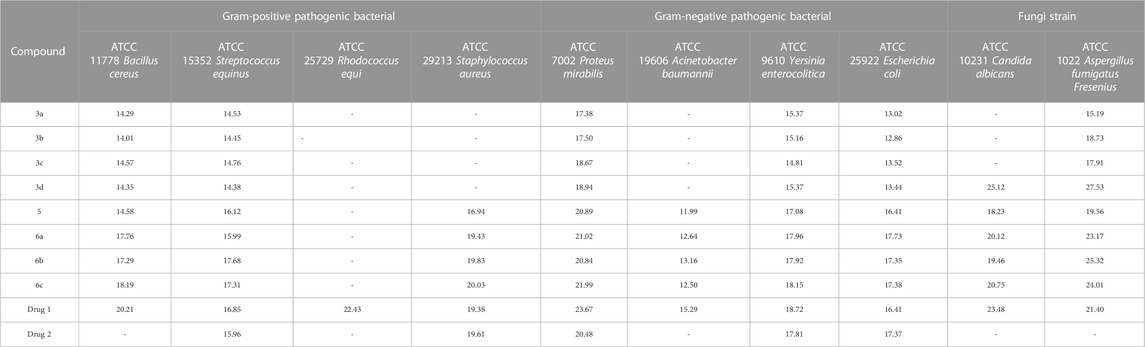
TABLE 6. IZD (mm) value results in investigating the antimicrobial properties of the synthesized derivatives.
Gentamicin, Cefazolin, Terbinafine, and Tolnaftate are known antibiotics, and, in this study, they were used to compare the antibacterial properties of the synthetic compounds. Gentamicin (antibacterial activity Tables, drug 1) and Cefazolin (antibacterial activity Tables, drug 2) are recognized potent antibacterial drugs, while Terbinafine (antifungal activity Tables, drug 1) and Tolnaftate (antifungal activity Tables, drug 2) are recognized potent antifungal drugs.
In terms of antimicrobial activity, the highest effectiveness was observed for 6c. The order of antimicrobial activity is described as 6b > 6a>5>3d> 3c > 3b > 3a. From the comparison of the structures, it can be concluded that the effectiveness of the compounds against bacterial species depends first on the hydroxyl and ester groups of the compounds, then on having an electron-rich benzene ring, and finally on substitutions with more methyl. The review of the literature showed that compounds containing oxygen and esters have high antibacterial properties (Shafiee et al., 1973; Dongamanti et al., 2015; Xie et al., 2015; Qin et al., 2020; Shi et al., 2021); here, it was also determined that the esterification of ciprofloxacin by glycerol led to the synthesis of compounds with significant antimicrobial properties.
In terms of antifungal activity, the highest effectiveness was observed for 3d. The MIC values for this combination were 32 μg/mL for both Candida albicans and Aspergillus fumigatus Fresenius. The presence of sulfur and the electron-rich benzene ring in the structure of this compound is the reason for the effectiveness of this compound in terms of antifungal activity. The order of antifungal activity is described as 6c > 6b > 6a>5>3c > 3b > 3a. By examining the structures of the derivatives, the order of effectiveness can be attributed to compounds with hydroxyl and ester groups, followed by the benzene ring, and, finally, substitutions with more methyl, just like the case for the antibacterial activity. Therefore, it was proved here that the presence of sulfur in the structure of 3d (methylthio) plays a significant role in antifungal activity (Pathania et al., 2019; Hua et al., 2020; Asif and Imran, 2022).
Comparing the results with known drugs such as Gentamicin, Cefazolin, Terbinafine, and Tolnaftate proved that the synthesized compounds are more active than them. For example, Cefazolin was ineffective against Bacillus cereus and Acinetobacter baumannii, but the MIC values for 6c were observed to be 16 μg/mL and 64 μg/mL, respectively. In terms of antifungal activity, Tolnaftate was not effective against Candida albicans and Aspergillus fumigatus Fresenius, but an MIC value of 32 μg/mL was observed for 3d.
As a final result, 6c and 3d can be reported as effective agents with antimicrobial and antifungal activities, respectively (Figure 10). After being proven to be safe and passing additional supplementary pharmacology tests, including in vivo, cytotoxicity, etc., they can be suggested as strong effective drugs.
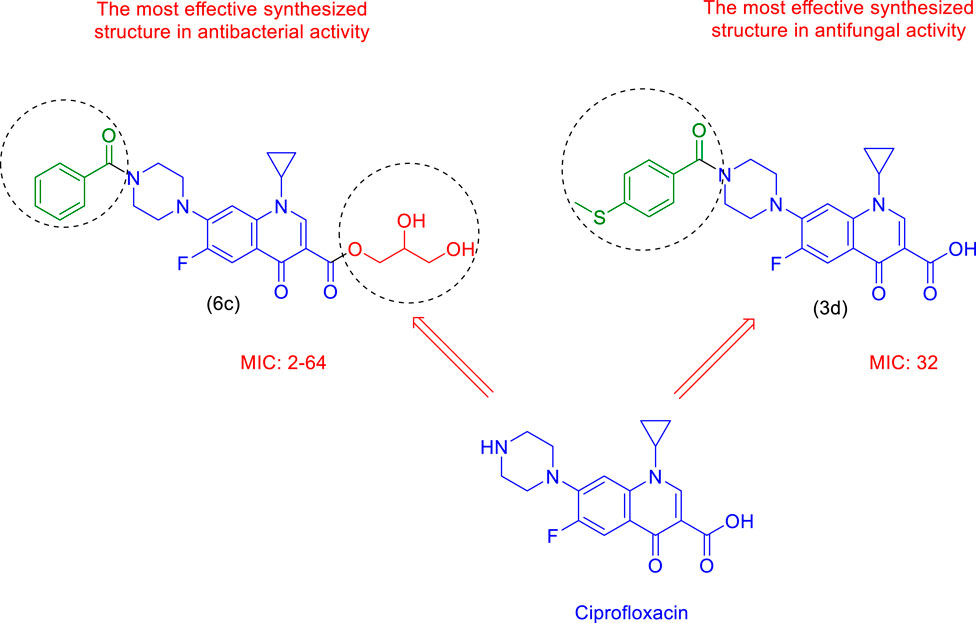
FIGURE 10. The most effective synthesized structures in terms of antibacterial activity (6c) and antifungal activity (3d).
4 Conclusion
In summary, in this research, ciprofloxacin was used as a starting material for synthesizing new amidification and esterification derivatives of ciprofloxacin. The synthetic compounds were confirmed by using proton nuclear magnetic resonance (1H NMR), carbon-13 nuclear magnetic resonance (13C NMR), mass spectrometry (MS), and elemental analysis (EA). IZD, MIC, MBC, and MFC were measured for antimicrobial properties, including Gram-positive antibacterial, Gram-negative antibacterial, and antifungal based on clinical and laboratory standards. In some derivatives, more effective antibacterial and antifungal properties were observed than for some commercial drugs. In continuation of the biological investigations, the in vitro anticancer activity of the derivatives against breast cancer cells was evaluated by the MTT method. In terms of anticancer properties, the effectiveness of some derivatives was similar to abemaciclib, a well-known anti-breast cancer drug. In biological evaluations, a clear relationship between the structure of the derivatives and biological properties such as antibacterial, antifungal, and anticancer was observed. As the results of biological activities have proven, some derivatives have higher antimicrobial and anticancer properties than commercial drugs used in the market. Therefore, the synthesized compounds in this study are important in this regard; it is suggested that other supplementary pharmacological and biological assays, such as in silico studies on the mechanism of action, in vivo tests, cytotoxicity tests, etc., be performed and if positive findings can be seen, then these compounds can be reported as effective leads for further preclinical and clinical studies.
Data availability statement
The original contributions presented in the study are available upon request, further inquiries can be directed to the corresponding author.
Author contributions
GA: Writing–original draft. ZA-O: Conceptualization, Supervision, Writing–original draft, Writing–review and editing.
Funding
The authors declare that no financial support was received for the research, authorship, and/or publication of this article.
Conflict of interest
The authors declare that the research was conducted in the absence of any commercial or financial relationships that could be construed as a potential conflict of interest.
Publisher’s note
All claims expressed in this article are solely those of the authors and do not necessarily represent those of their affiliated organizations, or those of the publisher, the editors and the reviewers. Any product that may be evaluated in this article, or claim that may be made by its manufacturer, is not guaranteed or endorsed by the publisher.
References
Aldred, K. J., Kerns, R. J., and Osheroff, N. (2014). Mechanism of quinolone action and resistance. Biochemistry 53 (10), 1565–1574. doi:10.1021/bi5000564
Asif, M., Imran, M., and Abida, (2022). Antimicrobial activities of various thiazine based heterocyclic compounds: A mini-review. Mini-Reviews Org. Chem. 19 (2), 166–172. doi:10.2174/1570193x18666210629102447
Beyzaei, H., Aryan, R., Moghaddam-Manesh, M., Ghasemi, B., Karimi, P., Delarami, H. S., et al. (2017). Evaluation and structure-activity relationship analysis of a new series of 4-imino-5H-pyrazolo [3, 4-d] pyrimidin-5-amines as potential antibacterial agents. J. Mol. Struct. 1144, 273–279. doi:10.1016/j.molstruc.2017.05.050
Bolakatti, G., Palkar, M., Katagi, M., Hampannavar, G., Karpoormath, R. V., Ninganagouda, S., et al. (2021). Novel series of benzo [d] thiazolyl substituted-2-quinolone hybrids: design, synthesis, biological evaluation and in-silico insights. J. Mol. Struct. 1227, 129413. doi:10.1016/j.molstruc.2020.129413
Das, B., Verma, J., Kumar, P., Ghosh, A., and Ramamurthy, T. (2020). Antibiotic resistance in Vibrio cholerae: understanding the ecology of resistance genes and mechanisms. Vaccine 38, A83–A92. doi:10.1016/j.vaccine.2019.06.031
Dib, M., Ouchetto, H., Ouchetto, K., Hafid, A., and Khouili, M. (2021). Recent developments of quinoline derivatives and their potential biological activities. Curr. Org. Synth. 18 (3), 248–269. doi:10.2174/1570179417666201216162055
Dongamanti, A., Aamate, V. K., Devulapally, M. G., Gundu, S., Kotni, M. K., Manga, V., et al. (2015). Synthesis, antimicrobial activity and molecular docking of novel tetracyclic scaffolds incorporating a flavonoid framework with medium sized oxygen heterocycles. Bioorg. Med. Chem. Lett. 25 (4), 898–903. doi:10.1016/j.bmcl.2014.12.066
El-Shershaby, M. H., El-Gamal, K. M., Bayoumi, A. H., El-Adl, K., Alswah, M., Ahmed, H. E., et al. (2021). The antimicrobial potential and pharmacokinetic profiles of novel quinoline-based scaffolds: synthesis and in silico mechanistic studies as dual dna gyrase and dhfr inhibitors. New J. Chem. 45 (31), 13986–14004. doi:10.1039/d1nj02838c
Etemadi, Y., Shiri, A., Eshghi, H., Akbarzadeh, M., Saadat, K., Mozafari, S., et al. (2016). Synthesis, characterisation, and in vitro antibacterial evaluation of a new class of 2-substituted-4-methyl-7, 8-dihydro-5H-pyrimido [4, 5-d] thiazolo [3, 2-a] pyrimidines. J. Chem. Res. 40 (10), 600–603. doi:10.3184/174751916x14737838285904
Ferreira, S. B., and Kaiser, C. R. (2012). Pyrazine derivatives: A patent review (2008–present). Expert Opin. Ther. Pat. 22 (9), 1033–1051. doi:10.1517/13543776.2012.714370
Foti, J. J., Devadoss, B., Winkler, J. A., Collins, J. J., and Walker, G. C. (2012). Oxidation of the guanine nucleotide pool underlies cell death by bactericidal antibiotics. Science 336 (6079), 315–319. doi:10.1126/science.1219192
Gao, F., Zhang, X., Wang, T., and Xiao, J. (2019). Quinolone hybrids and their anti-cancer activities: an overview. Eur. J. Med. Chem. 165, 59–79. doi:10.1016/j.ejmech.2019.01.017
Hooper, D. C. (2001). Emerging mechanisms of fluoroquinolone resistance. Emerg. Infect. Dis. 7 (2), 337–341. doi:10.3201/eid0702.010239
Hua, X., Liu, W., Su, Y., Liu, X., Liu, J., Liu, N., et al. (2020). Studies on the novel pyridine sulfide containing SDH based heterocyclic amide fungicide. Pest Manag. Sci. 76 (7), 2368–2378. doi:10.1002/ps.5773
Huigens, R. W., Brummel, B. R., Tenneti, S., Garrison, A. T., and Xiao, T. (2022). Pyrazine and phenazine heterocycles: platforms for total synthesis and drug discovery. Molecules 27 (3), 1112. doi:10.3390/molecules27031112
Jamshidi, H., Naimi-Jamal, M. R., Safavi, M., RayatSanati, K., Azerang, P., and Tahghighi, A. (2022). Synthesis and biological activity profile of novel triazole/quinoline hybrids. Chem. Biol. Drug Des. 100 (6), 935–946. doi:10.1111/cbdd.14031
Kudiyarova, A. D., Ashurov, J. M., Ibragimov, A. B., Sabirov, V. K., and Ibragimov, B. T. (2021). Synthesis, сrystal structure and Hirshfeld surface analysis of ciprofloxacinium tetrachlorocadmium dihydrate. Chem. Data Collect. 31, 100633. doi:10.1016/j.cdc.2020.100633
Matada, B. S., Pattanashettar, R., and Yernale, N. G. (2021). A comprehensive review on the biological interest of quinoline and its derivatives. Bioorg. Med. Chem. 32, 115973. doi:10.1016/j.bmc.2020.115973
Moghaddam-Manesh, M., Ghazanfari, D., Sheikhhosseini, E., and Akhgar, M. (2020). Synthesis, characterization and antimicrobial evaluation of novel 6'-Amino-spiro [indeno [1, 2-b] quinoxaline [1, 3] dithiine]-5'-carbonitrile derivatives. Acta Chim. Slov. 67 (1), 276–282. doi:10.17344/acsi.2019.5437
Moghaddam-manesh, M., Beyzaei, H., Heidari Majd, M., Hosseinzadegan, S., and Ghazvini, K. (2021). Investigation and comparison of biological effects of regioselectively synthesized thiazole derivatives. J. Heterocycl. Chem. 58 (7), 1525–1530. doi:10.1002/jhet.4278
Moghaddam-Manesh, M., and Hosseinzadegan, S. (2021). Introducing new method for the synthesis of polycyclic compounds containing [1, 3] dithiine derivatives, with anticancer and antibacterial activities against common bacterial strains between aquatic and human. J. Heterocycl. Chem. 58 (11), 2174–2180. doi:10.1002/jhet.4345
Pathania, S., Narang, R. K., and Rawal, R. K. (2019). Role of sulphur-heterocycles in medicinal chemistry: an update. Eur. J. Med. Chem. 180, 486–508. doi:10.1016/j.ejmech.2019.07.043
Pruchnik, H., Kral, T., Poradowski, D., Pawlak, A., Drynda, A., Obmińska-Mrukowicz, B., et al. (2016). New cytotoxic butyltin complexes with 2-sulfobenzoic acid: molecular interaction with lipid bilayers and dna as well as in vitro anticancer activity. Chemico-Biological Interact. 243, 107–118. doi:10.1016/j.cbi.2015.11.007
Qin, H.-L., Zhang, Z.-W., Ravindar, L., and Rakesh, K. (2020). Antibacterial activities with the structure-activity relationship of coumarin derivatives. Eur. J. Med. Chem. 207, 112832. doi:10.1016/j.ejmech.2020.112832
Qu, L., Li, X., Li, Z., Zhou, J., Zheng, M., Shang, Y., et al. (2023). A new approach to replace antibiotics with natural pigment derivatives: surface modification on the titanium implants. Appl. Surf. Sci. 608, 155122. doi:10.1016/j.apsusc.2022.155122
Schneider, H., Merbouh, N., Keerthisinghe, S., Antonietti, M., and Filonenko, S. (2022). Microwave-irradiated rapid synthesis of antimicrobial pyrazine derivatives in reactive eutectic media. Green Chem. 24 (24), 9745–9754. doi:10.1039/d2gc03122a
Shafiee, A., Lalezari, I., Yazdany, S., and Pournorouz, A. (1973). Selenium heterocycles VIH: synthesis and antibacterial activity of selenosemicarbazide and 1,3,4-selenadiazolylcarbamic acid esters. J. Pharm. Sci. 62 (5), 839–840. doi:10.1002/jps.2600620538
Sharma, B., Chowdhary, S., Legac, J., Rosenthal, P. J., and Kumar, V. (2023). Quinoline-based heterocyclic hydrazones: design, synthesis, anti-plasmodial assessment, and mechanistic insights. Chem. Biol. Drug Des. 101 (4), 829–836. doi:10.1111/cbdd.14185
Shashikant, M., Bains, A., Chawla, P., Sharma, M., Kaushik, R., Kandi, S., et al. (2022). In-vitro antimicrobial and anti-inflammatory activity of modified solvent evaporated ethanolic extract of calocybe indica: GCMS and HPLC characterization. Int. J. Food Microbiol. 376, 109741. doi:10.1016/j.ijfoodmicro.2022.109741
Shi, Y.-g., Zhang, R.-r., Zhu, C.-m., Liang, X.-r., Ettelaie, R., Jiang, L., et al. (2021). On the mechanism behind enhanced antibacterial activity of alkyl gallate esters against foodborne pathogens and its application in Chinese icefish preservation. Food Microbiol. 99, 103817. doi:10.1016/j.fm.2021.103817
Tambat, N., Mulani, S., Ahmad, A., Shaikh, S., and Ahmed, K. (2022). Pyrazine derivatives—versatile scaffold. Russ. J. Bioorg. Chem. 48 (5), 865–895. doi:10.1134/s1068162022050259
Tantawy, E. S., Amer, A. M., Mohamed, E. K., Abd Alla, M. M., and Nafie, M. S. (2020). Synthesis, characterization of some pyrazine derivatives as anti-cancer agents: in vitro and in silico approaches. J. Mol. Struct. 1210, 128013. doi:10.1016/j.molstruc.2020.128013
Terreni, M., Taccani, M., and Pregnolato, M. (2021). New antibiotics for multidrug-resistant bacterial strains: latest research developments and future perspectives. Molecules 26 (9), 2671. doi:10.3390/molecules26092671
Uncu, L., Donici, E., Valica, V., Vislouh, O., Gonciar, V., and Parii, S. (2019). Development and validation of an assay method for ciprofloxacin hydrochloride determination in combination ear drops. Chem. J. Moldova 14 (2), 56–61. doi:10.19261/cjm.2019.607
Wang, Z., Chen, P., Tao, N., Zhang, H., Li, R., Zhan, X., et al. (2018). Anticancer activity of polysaccharides produced from glycerol and crude glycerol by an endophytic fungus Chaetomium globosum CGMCC 6882 on human lung cancer A549 cells. Biomolecules 8 (4), 171. doi:10.3390/biom8040171
Xiao, Y., Liu, S., Gao, Y., Zhang, Y., Zhang, Q., and Li, X. (2022). Determination of antibiotic residues in aquaculture products by liquid chromatography tandem mass spectrometry: recent trends and developments from 2010 to 2020. Separations 9 (2), 35. doi:10.3390/separations9020035
Xie, Y., Yang, W., Tang, F., Chen, X., and Ren, L. (2015). Antibacterial activities of flavonoids: structure-activity relationship and mechanism. Curr. Med. Chem. 22 (1), 132–149. doi:10.2174/0929867321666140916113443
Keywords: ciprofloxacin, amidification, esterification, anticancer, antimicrobial
Citation: Alasadi GM and Al-Obaidi Z (2023) Synthesis of novel acylated and esterified ciprofloxacin derivatives as efficient anticancer and antimicrobial agents. Front. Mater. 10:1255955. doi: 10.3389/fmats.2023.1255955
Received: 10 July 2023; Accepted: 21 August 2023;
Published: 07 September 2023.
Edited by:
Farooq Sher, Nottingham Trent University, United KingdomReviewed by:
Lizie Daniela Tentler Prola, Independent Researcher, Greenville, NC, United StatesIvana Aleksic, University of Belgrade, Serbia
Hadia Almahli, University of Cambridge, United Kingdom
Copyright © 2023 Alasadi and Al-Obaidi. This is an open-access article distributed under the terms of the Creative Commons Attribution License (CC BY). The use, distribution or reproduction in other forums is permitted, provided the original author(s) and the copyright owner(s) are credited and that the original publication in this journal is cited, in accordance with accepted academic practice. No use, distribution or reproduction is permitted which does not comply with these terms.
*Correspondence: Zaid Al-Obaidi, ei5hbG9iYWlkaTFAYXN0b24uYWMudWs=
†ORCID: Zaid Al-Obaidi, https://orcid.org/0000-0003-3488-4572