- 1College of Mechanical Electrical Engineering, Shihezi University, Shihezi, China
- 2Key Laboratory of Northwest Agricultural Equipment, Ministry of Agriculture and Rural Affairs, Shihezi, China
- 3Engineering Research Center for Production Mechanization of Oasis Characteristic Economic Crops, Ministry of Education, Shihezi, China
Understanding cottonseeds’ basic physical parameters and compressive mechanical properties is necessary in seed cotton packing and cottonseed processing. In this study, the basic physical parameters of three cottonseed varieties (Huiyuan 720, Xinluzao 61, and Xinluzao 74) under different moisture contents (6%, 9%, 12%, and 15%) were measured. These parameters include 1000-grain weight, triaxial dimension, and sphericity. The effects of different moisture, varieties, and compression orientation (horizontal and vertical) on the compressive mechanical properties of the cottonseed were studied, and the variance analysis of the experimental data showed that the triaxial dimension, 1000-grain weight, arithmetic mean diameter, and geometric mean diameter of cottonseeds of the same variety increased with an increase in moisture content. Extremely significant differences (p < 0.01) were found in the physical parameters among different varieties. The main factors affecting crushing force and apparent elastic modulus are moisture content, variety, and compression orientation. For deformation, the main factors are moisture content, compression orientation, and variety. Moreover, compression orientation, moisture content, and variety are the main factors affecting failure energy. The results obtained that the crushing force and apparent elastic modulus decreased, deformation increased, and failure energy decreased first and then increased with the increase in moisture content under the same cottonseed variety. The results also provided the basic physical parameters for the design parameter optimization of seed cotton harvesting and processing machinery.
1 Introduction
Cottonseed is the seed of the cotton crop. In general, the seed cotton is removed by using the ginning machine to obtain the cashmere cottonseed. Then, the delinted cottonseed can be obtained by chemical treatment. Cottonseed, which can be used to grow cotton, has other functions, such as extracting cottonseed oil; cottonseed hull can be also used as feed raw material and fertilizer (Ouyang, 2012). During cotton harvesting, seed cotton is compressed and molded to facilitate transportation and storage (Bennett et al., 2015). As a part of seed cotton, the cottonseed breaks the compression load in the molding process; the seepage affects the quality of the seed cotton, and the subsequent breeding affects the germination rate, resulting in yield reduction and loss Clark et al., 1969; Columbus and Mangialardi, 1996; Husin, 2016). This scenario affects the income of seed enterprises and the yield of seed cotton farmers. Moreover, the shape and size of the cottonseed are crucial to the design and development of ginning and screening machinery.
At present, research on the compression characteristics of cottonseed is little. Zheng et al. (2007) researched the stress–strain characteristics of cottonseed under different loading speeds. Lalor et al. (1995) showed that compressing seed cotton to a density of 400 kg m−3 causes mechanical damage to the cottonseed. Brashears et al. (1970) studied the relationship between cottonseed mass and pressure density and showed that compressing the seed cotton to 320 kg m−3 does not cause significant damage to the seeds. Kirk and McLeod (1967), the first to study cottonseed, obtained the law of the influence of moisture content on anti-cracking properties. Ozarslan (2002), Asadzadeh (2014), and Anitha (2022) studied the physical properties of cottonseed with different moisture contents by considering the triaxial dimension, bulk density, and static friction coefficient of cottonseeds. Moisture content has a significant effect on the above coefficients. Dowd et al. (2019) studied the influences of different moisture contents, compression orientation, and variety on the crushing force of cottonseed. However, the influence of the apparent elastic modulus and the interaction between the factors have not been proved. However, few direct studies investigated the effects of cottonseed moisture content, variety, and other factors on the compressive mechanical properties, such as crushing force, failure energy, and apparent elastic modulus. These studies can be conducted using the experimental methods of other plant seeds for reference. Many scholars studied the compression characteristics of hazelnut (Cristiana et al., 2015), sorghum grain (Feng et al., 2022), naked oat seed (Zhang et al., 2019; Qiu et al., 2021), almond (Shi et al., 2021), millet (Yang et al., 2015; Sun et al., 2017), and other seeds. The results showed that moisture content, compression direction, and variety affect the destructive force, deformation, and destructive energy of grains.
This study provides a theoretical basis for the research and development of a baling machine adapted to the characteristics of seed cotton in Xinjiang. The basic physical parameters of seed cotton considerably influence the damage of cottonseed in ginning and screening. Thus, the 1000-grain weight, triaxial dimension, sphericity, arithmetic mean diameter, and geometric mean diameter of cottonseed were statistically analyzed under different varieties and moisture contents, thereby providing a reference for the design of cottonseed processing machinery.
2 Materials and methods
2.1 Materials and equipment
The cottonseed used in the experiment was taken from the experimental field of Shihezi University. The test varieties were the three main cotton varieties in the Shihezi area: Huiyuan 720, Xinluzao 61, and Xinluzao 74. During the harvest period in September 2021, they were manually picked and loaded into black sealed bags and sent back to the laboratory. After the harvest, the seed cotton was disheveled completely, and the mature cottonseeds were selected for initial moisture content determination. The initial average moisture contents of the three cottonseeds were 5.56%, 5.10%, and 5.45%. The moisture content of the treated samples was 6%, 9%, 12%, and 15%. The treated cottonseeds were placed into sealed bags and refrigerated at a low temperature. Before each test, the sample was taken out of the sealed bag for testing after standing at room temperature (25°C ± 1°C) for 2 h. The equipment used in the test is shown in Table 1.
2.2 Experimental methods
2.2.1 Cottonseed moisture content adjustment
The moisture content of cottonseeds with moisture content lower than the target moisture content level was increased through humidification to prevent the mechanical properties of cottonseeds from being affected (Yang et al., 2015). The samples were placed in an environment with a temperature of 50°C and a humidity of 20% for prehumidification for 2 h. Then, they were dried in the 101-1BS electric blast drying oven for 48 h. The moisture content was adjusted, and the sample was sealed for 2 h at a constant temperature to make the moisture content uniform. The mass of the deionized water added was calculated according to the following equation:
where m1 is the mass of deionized water, g; m0 is the initial mass of cottonseed, g; W0 is the initial moisture content of cottonseed, %; and W1 is the target moisture content of cottonseed, %.
2.2.2 Determination of basic physical parameters
The shape and size of cottonseed directly affect the smooth depilation of seed cotton in the ginning machine. This principle is the basis for the design of the ginning machine’s structure and critical dimensions. The seed size is usually expressed by the triaxial dimensions of the grain and the 1000-grain weight. The former is crucial in seed sorting, whereas the latter is the main index for seed quality measurement.
For the measurement of cottonseed’s 1000-grain weight, 1,000 cottonseeds were randomly selected, and the mass was weighed on an electronic balance thrice to obtain the average, that is,
where m is the 1000-grain weight, g, and m1, m2, and m3 are three measurements, g.
For the triaxial dimension measurement of cottonseed, a plump seed without mildew, pests, or damages was chosen each time. The length (L), width (W), and height (H) were measured with a digital Vernier caliper (Figure 1). A total of 30 cottonseeds were taken, and the average value was obtained after repeated measurements. The shape of cottonseeds is irregular and varies greatly. Thus, sphericity and particle size are usually used to represent the shape and size of cottonseeds. The calculation equation of sphericity Sp is as follows:
where Sp is the sphericity of the dewoolled cottonseed, %; L is the length of dewoolled cottonseed, mm; W is the width of dewoolled cottonseed, mm; and H is the height of dewoolled cottonseed, mm.
The particle size of dewoolled cottonseed can be expressed by its triaxial arithmetic mean Da and geometric mean Dg:
where Da is the arithmetic mean of cottonseed, mm, and Dg is the geometric mean of cottonseed, mm.
2.2.3 Compression test
In the present study, the TA.XTplus Texture Analyzer was used for the compression test of cottonseed, with moisture content, compression orientation, and variety as the factors and the crushing force, failure energy, deformation, and apparent elastic modulus as the indexes. The test mode of the TA.XTplus Texture Analyzer was set as the compression mode, and a ϕ100 mm × 90 mm self-made cottonseed auxiliary compression mounting seat and an SMS P/36R cylindrical indenter were used as compression devices. The pretest speed, compression speed, posttest speed, and trigger force were set to 0.5 mm s−1, 0.01 mm s−1, 1.0 mm s−1, and 0.049 N, respectively (Yang et al., 2015). Before the test, the physical property analyzer was preheated for 20 min, and the digital Vernier caliper was used to measure the triaxial dimensions of cottonseeds. Then, the cottonseeds were placed on the self-made cottonseed auxiliary compression mounting seat for the compression test. Finally, the compression force–displacement curve was recorded. The test device and cottonseed compression diagram are shown in Figure 2.
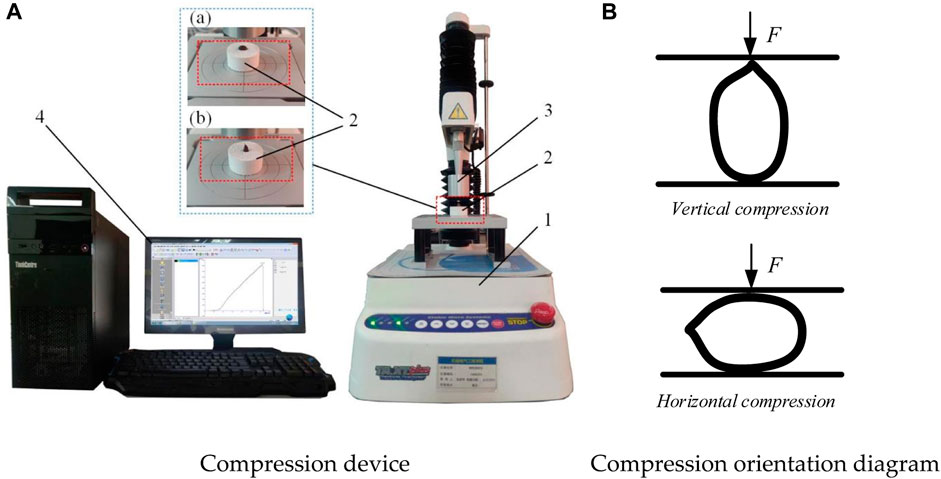
FIGURE 2. Compression device and compression schematic. Note: 1. TA.XTplus Texture Analyzer. 2. Auxiliary compression mounting seat. 3. P/36R cylindrical indenter. 4. Computer. (A) Horizontal; (B) vertical. F is the compression force, N.
Given the small size, light weight, and irregular shape of the cottonseed, compression via conventional methods during testing is challenging. An auxiliary compression mounting seat was adopted to fix the cottonseed without affecting the results. The test instrument and placement form of the cottonseed are shown below in Figure 2A. In harvesting, transportation, and processing, cottonseed is subjected to different compression loads in different directions, and the damage degree is different. Therefore, compression tests were performed on the cottonseed in the X- and Y-axis (Figure 2B).
Making a complete compression–deformation curve is necessary to describe the compression characteristics of cottonseed. The curve of the relationship between compression force and displacement exhibited a yield inflexion point (Figure 3). Before reaching the yield point, the compression force and displacement increased approximately linearly. The cottonseed was observed using a stereomicroscope and showed no cracks. After reaching the yield point, the compression force reached the first peak; that is, the surface of the cottonseed was broken. Therefore, the yield point of the cottonseed was the significant demarcation point of failure. Moreover, the corresponding compression force was taken as the crushing force of the cottonseed under compression, and the corresponding displacement was the deformation amount of the cottonseed when it yields. The area enclosed by the curve before the yield point and the abscissa was the failure energy.
The Hertz equation for contact stress in solid mechanics can be used to estimate the apparent elastic modulus E of relatively strong materials. Under X-axis compression, the Hertz equation can be used to calculate the apparent contact modulus of cottonseed as follows (ASAE, 2008):
where E is the cottonseed’s apparent contact elastic modulus, MPa; F is the crushing force, N; D is the cottonseed deformation, mm; μ is the cottonseed Poisson’s ratio, 0.3;
where
Three seed cotton varieties (Huiyuan 720, Xinluzao 61, and Xinluzao 74), four moisture content levels (6%, 9%, 12%, and 15%), and two compression orientations (horizontal and vertical) were selected to conduct a ‘2 × 3 × 4’ experiment with 24 groups, with seed cotton variety, moisture content, and compression orientation as the experimental factors. Each test was repeated five times, and the crushing force, cottonseed deformation, failure energy, and apparent elastic modulus were used as response variables for statistical analysis.
3 Results and discussion
3.1 Analyzing the basic physical parameters of different cottonseeds under different moisture contents
The triaxial dimensions and 1000-grain weights of dewoolled cottonseeds with different moisture contents and varieties were measured at normal temperature (25°C ± 1°C). The sphericity, arithmetic mean diameter, and geometric mean diameter of cottonseeds were calculated through the triaxial dimensions. The results are shown in Table 2. The influences of seed cotton variety and moisture content on the basic physical parameters of cottonseeds are shown in Table 3.
Table 3 shows that moisture content had an extremely significant effect on the length, width, height, 1000-grain weight, sphericity, arithmetic mean diameter, and geometric mean diameter of cottonseeds (p < 0.001). The length, width, 1000-seed weight, arithmetic mean diameter, and geometric mean diameter of cottonseeds were significantly affected by the variety of seed cotton (p < 0.01). They also had significant effects on cottonseed height (p < 0.05). The interaction of seed cotton variety and moisture content had extremely significant effects on the length, arithmetic mean diameter, and geometric mean diameter of cottonseeds (p < 0.01). However, the interaction of variety, moisture content, and variety and moisture content had no significant effects on the residual response index.
Table 2 shows that when the moisture content of cottonseeds increased from 6% to 15%, the triaxial dimension, 1000-grain weight, arithmetic average diameter, and geometric mean diameter of cottonseeds presented an increasing trend because cottonseeds mainly consist of solid-phase material, water, and gas-filled pores (Qiu et al., 2021). The internal voids in cottonseeds continued to decrease with water loss. The cottonseed also showed tiny folds with the decrease in moisture content, and the cottonseed’s triaxial dimension gradually decreased with the decrease in moisture content under the dual action.
The sphericity of cottonseed was above 60% at all moisture content levels. Moreover, the sphericity of the cottonseed of the same variety showed a fluctuating decreasing trend with the increase in moisture content. This increase might be caused by the increase in the ratio of length, width, and height. The arithmetic mean diameter of the cottonseed was between 6.314 and 6.904 mm, and the geometric mean diameter was between 5.957 and 6.469 mm. In addition, the aspect ratio of the cottonseed was close to 1. When simulation modeling was needed, the shape of the cottonseed could be approximately regarded as an ellipsoid.
Under the same moisture content, the order of the 1000-grain weight of each variety was Huiyuan 720, Xinluzao 74, and Xinluzao 61, indicating that the Huiyuan 720 cottonseed was plump and could store many nutrients. According to the existing literature and field measurement, the lint percentages of Huiyuan 720, Xinluzao 61, and Xinluzao 74 are 40.9%, 42%, and 41.4%, respectively. This finding shows that Huiyuan 720 had a large proportion of cottonseeds, with the seed cotton having the same quality. Moreover, the number of cottonseeds in a single petal of seed cotton was 7 or 8. The larger the triaxial dimension of the Huiyuan 720 cottonseed is, the larger the 1000-grain weight is.
3.2 Test results of cottonseeds’ compressive mechanical properties
The cottonseeds were compressed at different moisture contents and compression orientations. The crushing force and deformation of different cottonseed varieties are shown in Table 4. Their failure energies and apparent elastic modulus are shown in Table 5. The results of analysis of variance (ANOVA) are shown in Table 6.
Table 6 shows that seed cotton variety had no significant effect on deformation. Similarly, compression orientation had no significant effect on apparent elastic modulus. However, moisture content had an extremely significant effect on the apparent elastic modulus (p < 0.01). Variety, moisture content, and compression orientation had extremely significant effects on crushing force and failure energy (p < 0.01). Moreover, moisture content and compression orientation had extremely significant effects on deformation (p < 0.01). From the interaction perspective, variety × moisture content, variety × compression orientation, and variety × moisture content × compression orientation had extremely significant effects on crushing force (p < 0.01). Moisture content × compression orientation and variety × moisture content × compression orientation had significant effects on deformation (p < 0.05). The apparent modulus of elasticity was significantly affected by variety × moisture content (p < 0.01). However, other interactions had no significant effect on it. Table 6 shows that all interactions had a very significant effect on failure energy (p < 0.01).
The main factors affecting the crushing force and the apparent elastic modulus are moisture content, variety, and compression orientation. For deformation, the main factors are moisture content, compression orientation, and variety. Moreover, compression orientation, moisture content, and variety are the main factors affecting failure energy.
3.3 Effect of moisture content on the compressive mechanical properties of cottonseeds
All test data were imported into Origin 2019 for processing.
3.3.1 Characteristics of moisture content and its effect on crushing force
The relationship between crushing force and moisture content was fitted by an exponential equation (Figure 4). Moreover, the coefficient of determination is R2 ≥ 0.91, indicating that the correlation was high. For the same cottonseed, the crushing force in horizontal and vertical compression directions decreased with the increase in moisture content when the moisture content was in the 6%–15% range. Moreover, the crushing force decreased rapidly when the moisture content was in the 6%–12% range and decreased slowly when the moisture content was in the 12%–15% range. These results are in accordance with the exponential law. They are also similar to those obtained by Saiedirad et al. (2008) on cumin seeds, where the crushing force decreases with the increase in the seeds’ moisture content. According to the analysis, water molecules would cause a certain gap and lubrication effect inside the cottonseed, which would weaken the interaction force between molecules and reduce the compressive strength of them. The higher the moisture content is, the lower the strength and hardness of cottonseed are; the worse the compressive resistance is, the smaller the crushing force is.
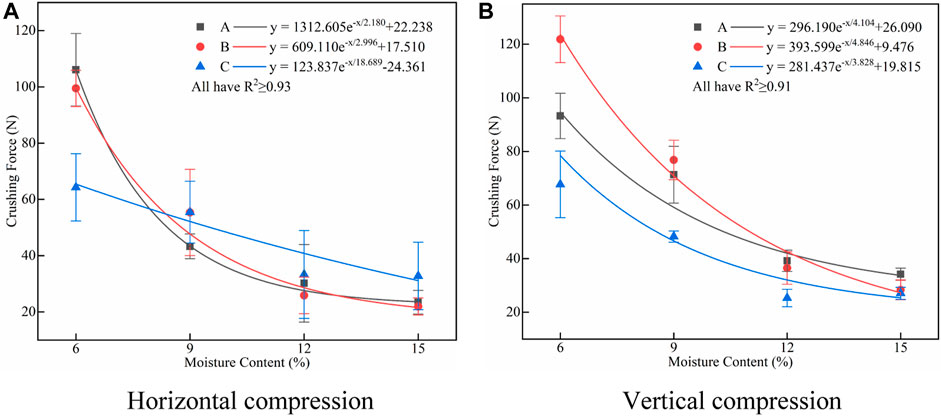
FIGURE 4. Influence curve of cotton seed moisture content on crushing force. Two different compressive orientations: (A) Horizontal compression. (B) Vetical compression. Note: A, B, C represent Huiyuan 720, Xinluzao 64, Xinluzao 71 respectively, the same below.
3.3.2 Relationship between moisture content and deformation
The relationship between deformation and moisture content was fitted by a quadratic polynomial (Figure 5). Moreover, the coefficient of determination is R2 ≥ 0.95, indicating that the correlation was high. For the same cottonseed, the deformation needed to destroy cottonseeds showed an upward trend with the increase in moisture content. The analysis showed that with the increase in moisture content, the triaxial dimension of cottonseeds increased, the fiber tissue of the shell softened, the flexibility increased, the deformation resistance weakened, and the deformation amount increased when damaged. Managing seed moisture content is critical in agriculture and seed treatment. The quality and storage life of seeds can be ensured by controlling their moisture content. Seeds are stacked together and squeezed against one another during storage. Thus, very high or very low moisture content may cause the seed to rot, deteriorate, or lose the ability to germinate.
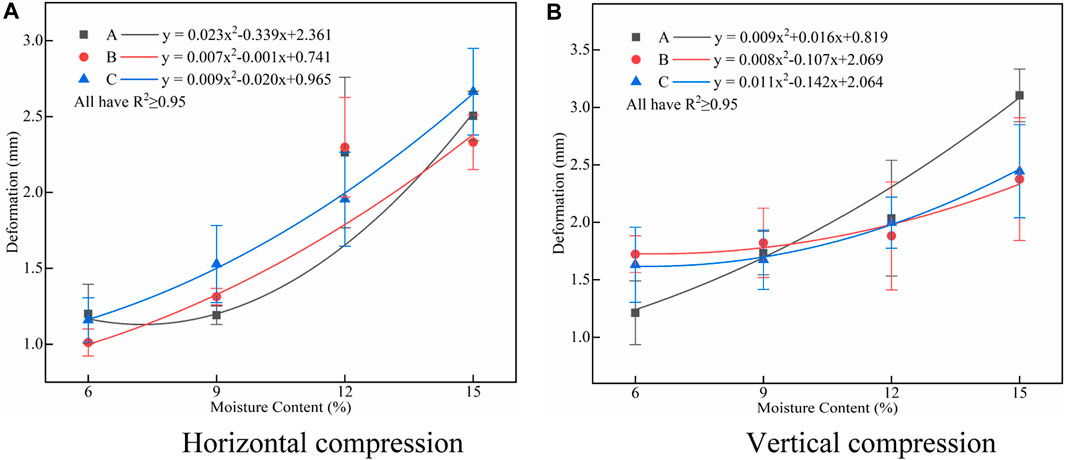
FIGURE 5. Influence curve of cotton seed moisture content on deformation. Two different compressive orientations: (A) Horizontal compression. (B) Vetical compression.
3.3.3 Influence of moisture content on failure energy
Failure energy is the minimum energy required for the damage of cottonseeds, which is related to the crushing force and deformation. The failure energy at the yield point is numerically equal to the area enclosed by the curve and abscissa before the yield point (Figure 3). Except for Xinluzao 71, the failure energy of the two other cottonseeds decreased first and then increased with the increase in moisture content at the same compression orientation (Figure 6). The analysis showed that with the increase in moisture content, the internal organization became soft, the crushing force decreased, and the deformation increased. The crushing force would decrease rapidly when the moisture content was 6%–12%. Moreover, the failure energy, considerably affected by the crushing force, would greatly decrease. However, when the moisture content was 12%–15%, the crushing force of vertical compression decreased slowly, slightly affecting the failure energy. The failure energy increased mainly with the increase in deformation. Further increase in moisture content may form a more compact structure, improve the ability to resist damage, and be more prominent in vertical compression.
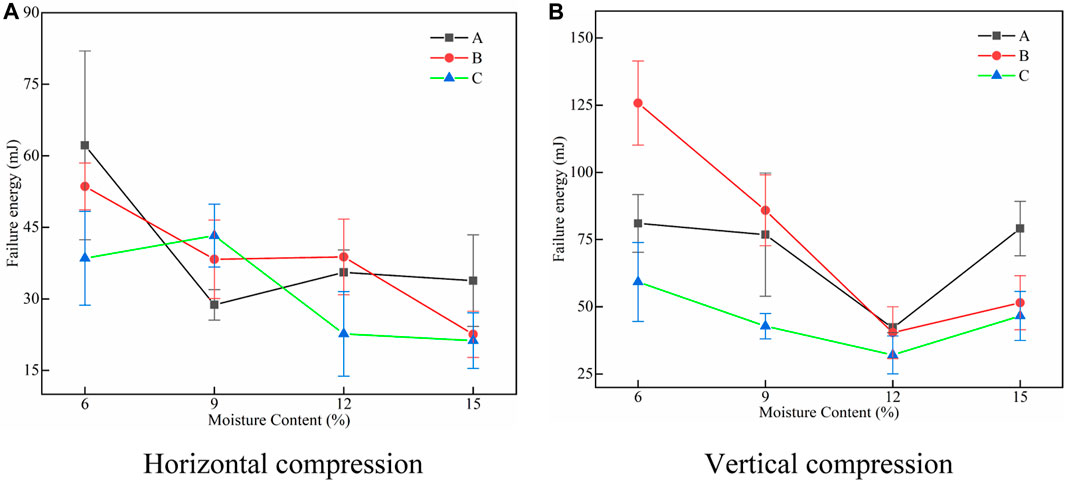
FIGURE 6. Influence curve of cotton seed moisture content on failure energy. Two different compressive orientations: (A) Horizontal compression. (B) Vetical compression.
3.3.4 Analyzing the influence of moisture content on apparent elastic modulus
The relationship between deformation and moisture content was fitted by a quadratic polynomial (Figure 7). Moreover, the coefficient of determination is R2 ≥ 0.93, indicating that the correlation was high. Apparent elastic modulus reflects the ability of the grain material to produce elastic deformation; that is, it reflects the plasticity and brittleness of the material. The apparent elastic modulus was related to the triaxial dimension, deformation, and crushing force of the cottonseed. For the same cottonseed, the apparent elastic modulus decreased with the increase in moisture content in the same compression orientation. This observation shows that elastic deformation occurred easily when the moisture content of the cottonseed was high; that is, the force required for the same deformation was small. This finding is consistent with the experimental results of crushing force and Hooke’s law. The following are possible reasons, which may be the increase in moisture content caused by internal chemical reactions (such as oxidation or corrosion reactions) or caused by changes in chemical bonds, changing the structure and relative position between molecules, thereby affecting the apparent elastic modulus of the cottonseed.
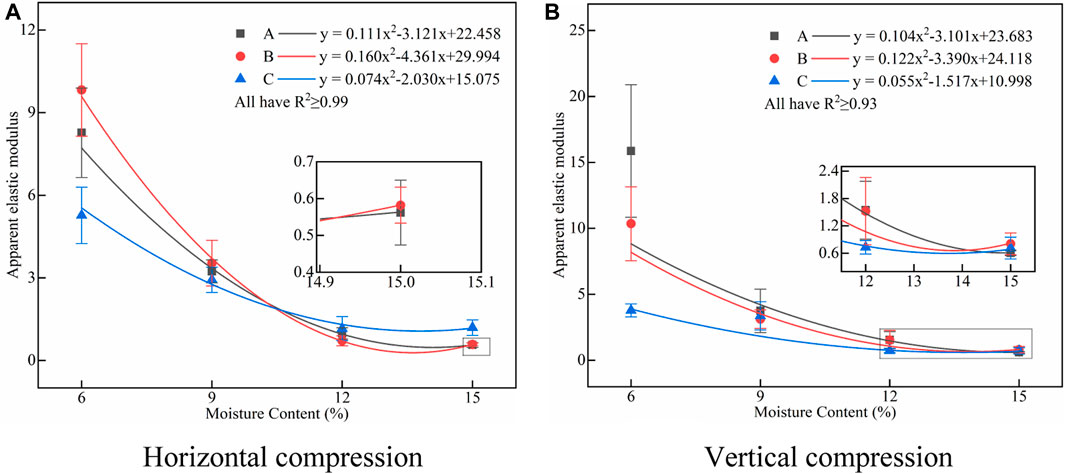
FIGURE 7. Influence curve of cotton seed moisture content on apparent elastic modules. Two different compressive orientations: (A) Horizontal compression. (B) Vetical compression.
3.4 Influence of compressive orientation on the compressive mechanical properties of cottonseed
Compressive orientations could not be analyzed by the regression equation. Table 6 shows that the compression orientation has an extremely significant effect on the crushing force, deformation, and failure energy. However, it has no significant effect on the apparent elastic modulus. The cracking pattern of the cottonseed at different compression orientations and the uncompressed cottonseed is shown in Figure 8.
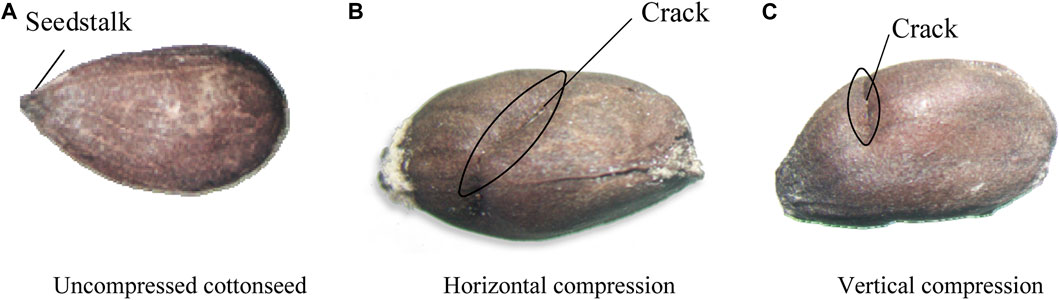
FIGURE 8. Uncompressed cottonseed and cracks under different compression directions. (A) Uncompressed cottonseed. (B) Horizontal compression. (C) Vetical compression.
When the cottonseed was compressed horizontally, the crack was a curve passing through the middle and running through half of the cottonseed. When it was compressed vertically, the initial broken position was at the seedstalk. Then, it expanded and extended along the direction of the navel, forming a curved crack on the back of the cottonseed. The contact area between the inner hilum and seedstalk was small, and stress concentration occurred easily. Thus, it was first broken near the contact point. The external forces of vertical compression and horizontal compression were applied to the cottonseed, and the stress transfer paths might be different, resulting in different directions of cracks.
Seeds usually have a specific growth direction (stems always grow upward, and roots always grow downward). The cells and fibers in this direction are arranged more compactly, resulting in higher stiffness and strength, which means that when the external stress is perpendicular to the growth direction, the seeds may exhibit higher resistance and require more energy to destroy. When the cottonseed was placed vertically, the fiber in this state was also in the height direction, and the damage required more energy. It is also clear from Table 1 that the height of vertical compression was roughly twice the height of horizontal compression, which might also be the reason for more energy required for vertical compression. Under 6%–9% moisture content, the vertical deformation of the same varieties was greater than the horizontal deformation. At 12%–15% moisture content, the horizontal and vertical deformations had no obvious difference. The possible reason is that the apparent elastic modulus was greatly affected by the moisture content. When the moisture content was very high (12%–15%), the apparent elastic modulus was small regardless of whether the compression was horizontal or vertical. Thus, the deformations generated by the two compressions had no difference. The impact of compression orientation on the apparent elastic modulus was not significant because the apparent elastic modulus was the physical property of the cottonseed itself and was not affected by the way of force. At a low moisture content level (12%–15%), the vertical compression was likely to press the most vulnerable part of the cottonseed (seedstalk). Thus, the destruction occurred rapidly and the deformation increased. For the horizontal compression, the cotton shell was hard and had excellent resistance. The contact area was large because of the low moisture content. Moreover, the pressure per unit area was smaller, so the deformation was small.
Under different compression orientations, the crushing force of horizontal compression was generally smaller than that of vertical compression. For the same cottonseed with the same moisture content, the failure energy of vertical compression was greater than that of horizontal compression. However, in the existing ginning machine, the roller is mostly applied, generally considering the horizontal situation of the cottonseed, so the damage of the cottonseed is more serious.
3.5 Analyzing the effects of varieties on the compressive mechanical properties of cottonseed
Cottonseed varieties could not be analyzed by the regression equation. The main components of cottonseed include cellulose, fatty acids, proteins, and carbohydrates. Different varieties of cottonseed have different triaxial dimensions. The contents of various components are also different. Thus, the mechanical properties of compression also differ. Table 6 shows that the effect of cottonseed variety on crushing force, failure energy, and apparent elastic modulus was extremely significant (p < 0.01). The influences of moisture content on the crushing force, failure energy, and apparent elastic modulus of different varieties were similar, but the degree of influence differed. For example, the following rules are presented when different varieties were compressed vertically: the order of crushing force and failure energy at low moisture content is Xinluzao 61, Huiyuan 720, and Xinluzao 74 in descending order, whereas that of the crushing force and failure energy at a high moisture content is Huiyuan 720, Xinluzao 61, and Xinluzao 74. Under the same compression conditions, Xinluzao 74 required the least crushing force and the least failure energy. Therefore, attention should be paid to reduce damage during the storage and transportation of this cottonseed variety. In the design of related mechanical products, different mechanical properties of cottonseed varieties should be considered, and the minimum mechanical properties should be taken as the design index.
4 Conclusion
In this study, the compressive mechanical properties of different varieties were studied at different moisture contents and compression orientations. The main conclusions are as follows:
1. The length, width, height, 1000-grain weight, sphericity, arithmetic mean diameter, and geometric mean diameter of cottonseeds were extremely affected by moisture content (P < 0.001). They increased with the increase in moisture content. Cottonseed varieties had significant effects on all indexes except sphericity (P < 0.01). At the 0.01 level, variety, moisture content, and compression orientation significantly affected the crushing force. Moisture content and compression orientation had significant effects on deformation and failure energy (P < 0.01).
2. For the same cottonseed, the crushing force and the apparent elastic modulus of the cottonseed at two compression orientations decreased with the increase in moisture content. Moreover, crushing force and apparent elastic modulus decreased slowly at the moisture content of 12%–15%. The deformation of the two compression orientations increased with the increase in moisture content, possibly because of the increase in moisture content, softening of the shell’s fibrous tissue and weakening of the anti-deformation ability. This phenomenon resulted in an increase in deformation. At the moisture content of 6%–12%, the failure energy showed a decreasing trend with the increase in moisture content.
3. The crack direction differed in the cottonseed with a different compression orientation. When the cottonseed was compressed horizontally, the crack was a curve passing through the middle and running through half of the cottonseed. However, when the cottonseed was compressed vertically, the initial broken position was at the seedstalk. Then, it expanded and extended along the navel direction, forming a curved crack on the back of the cottonseed.
4. Under different compression orientations, the crushing force of horizontal compression was generally smaller than that of vertical compression.
5. When different varieties were compressed vertically, the order of crushing force and failure energy at low moisture content was Xinluzao 61, Huiyuan 720, and Xinluzao 74 in descending order. The order of crushing force and failure energy at high moisture content was Huiyuan 720, Xinluzao 61, and Xinluzao 74. Therefore, the pressure resistance of Xinluzao 74 was poor, and it needed considerable attention in storage and transportation.
Data availability statement
The original contributions presented in the study are included in the article/Supplementary Material; further inquiries can be directed to the corresponding author.
Author contributions
YZ: data curation, formal analysis, investigation, software, validation, visualization, writing–original draft, writing–review and editing, and methodology. MW: conceptualization, data curation, formal analysis, funding acquisition, methodology, resources, validation, and writing–review and editing. XD: data curation, formal analysis, investigation, validation, and writing–original draft. HZ: conceptualization, funding acquisition, methodology, project administration, resources, supervision, and writing–review and editing. LW: conceptualization, resources, and writing–review and editing. XW: software, visualization, and writing–review and editing. XF: writing–review and editing. JW: investigation and writing–review and editing.
Funding
The authors declare that financial support was received for the research, authorship, and/or publication of this article. This study was funded by the National Natural Science Foundation (Grant Number 32260435), the Shihezi 514 University Youth Innovation Cultivation Talent Project (Grant Number CXPY202120), the Corps Major Scientific and Technological Projects (Grant Number 2018AA008), and the 513 Corps Key Field Innovative Team Building Project (Grant Number 2019CB006).
Conflict of interest
The authors declare that the research was conducted in the absence of any commercial or financial relationships that could be construed as a potential conflict of interest.
Publisher’s note
All claims expressed in this article are solely those of the authors and do not necessarily represent those of their affiliated organizations, or those of the publisher, the editors, and the reviewers. Any product that may be evaluated in this article, or claim that may be made by its manufacturer, is not guaranteed or endorsed by the publisher.
References
Anitha, G. (2022). Physical and mechanical properties of cotton seeds. Pharma Innovation J. SP- 11 (10), 1331–1333.
Asadzadeh, A. H. (2014). Some physical properties of cotton seed (‘Varamin’ cultivar) at different moisture contents. Acta Hortic. 1054, 205–213. doi:10.17660/ActaHortic.2014.1054.24
ASAE (2008). Compression test of food materials of convex shape. ASAE S368.4 DEC2000 (R2008). St. Joseph: American Society of Agricultural and Biological Engineers.
Bennett, J. M., Woodhouse, N. P., Keller, T., Jensen, T. A., and Antille, D. L. (2015). Advances in cotton harvesting technology: a review and implications for the John Deere round baler cotton picker. J. Cotton Sci. 19 (2), 225–249.
Brashears, A. D., Kirk, I. W., and Hudspeth, E. B. (1970). “Pressure-density relationship of seed cotton and its effects on seed quality,” in ASAE southwest region meeting (St. Joseph: Mich. ASAE).
Clark, R. L., Welch, G. B., and Anderson, J. H. (1969). Effect of high-velocity impact on germination and crackage of cottonseed. Amer. Soc. Agric. Eng. Trans. Asae. 12 (6), 0748–0751. doi:10.13031/2013.38943
Columbus, E. P., and Mangialardi, G. J. (1996). Cottonseed moisture and seed damage at gins. Trans. ASAE 39 (5), 1617–1621. doi:10.13031/2013.27677
Cristiana, D., Simone, G., Emanuele, R., Rolle, L., and Sesana, R. (2015). Experimental characterization and numerical modeling of the compressive mechanical behavior of hazelnut kernels. J. Food Engi 166, 364–369. doi:10.1016/j.jfoodeng.2015.06.037
Dowd, M. K., Manandhar, R., and Delhom, C. D. (2019). Effect of seed orientation, acid delinting, moisture level, and sample type on cottonseed fracture resistance. Trans. ASABE 62 (4), 1045–1053. doi:10.13031/trans.13109
Feng, Y., Qiu, S., Yuan, X., Cui, Q., and Ji, Z. (2022). Study on compression mechanical properties of sorghum grain. China Agric. Sci. Tech. Bul. 24 (05), 102–110. doi:10.13304/j.nykjdb.2021.0615
Husin, N. A. B. (2016). Impact of seed cotton compression on cottonseed quality. United States: Texas A&M University.
Kirk, I. W., and McLeod, H. E. (1967). Cottonseed rupture from static energy and impact velocity. Trans. ASAE 10 (2), 217–219. doi:10.13031/2013.39638
Lalor, W. F., Willcutt, M. H., and Curley, R. G. (1995). Seed cotton storage and handling. Cotton Ginners’ Handbook. Agric. Handb. 503, 16–25.
Ouyang, L. (2012). Development status and prospect of cottonseed processing industry in China. Hunan Feed 05, 11–13. doi:10.3969/j.issn.1673-7539.2012.05.005
Ozarslan, C. (2002). PH—postharvest technology. Biosyst. Eng. 83 (2), 169–174. doi:10.1006/bioe.2002.0105
Qiu, S., Li, L., Cui, Q., Yang, Z., Feng, Y., Guo, Y., et al. (2021). Study on shearing characteristics of naked oat grain. Chin. Jour. Agric. Mach. Chem. 42 (06), 67–71. doi:10.13733/j.jcam.issn.2095-5553.2021.06.11
Saiedirad, M. H., Tabatabaeefar, A., Borghei, A., Mirsalehi, M., Badii, F., and Varnamkhasti, M. G. (2008). Effects of moisture content, seed size, loading rate and seed orientation on force and energy required for fracturing cumin seed (Cuminum cyminum Linn.) under quasi-static loading. J. Food Eng. 86, 565–572. doi:10.1016/j.jfoodeng.2007.11.021
Shi, X., Niu, C., Tu, T., and Zhang, L. (2021). Study on the material characteristics of Amygdalus communis. Xinjiang Agri. Mech. 2021 (02), 33–34. +44. doi:10.13620/j.cnki.issn1007-7782.2021.02.010
Sun, Y., Yang, Z., Guo, Y., Cui, Q., Wu, X., Zhang, Y., et al. (2017). Compressive mechanical properties and damage crack formation mechanism of millet grain. J. Agri. Engi. 33 (18), 306–314. doi:10.11975/j.issn.1002-6819.2017.18.040
Yang, Z., Sun, J., and Guo, Y. (2015). Effects of different moisture content on compression mechanical properties and friction characteristics of millet grain. J. Agri. Engi. 31 (23), 253–260. doi:10.11975/j.issn.1002-6819.2015.23.034
Zhang, Z., Tao, G., Yi, S., and Mao, X. (2019). Experimental study on compression mechanical properties of naked oat kernels and analysis of cracking rules. J. Shenyang Agri. Uni. 50 (03), 371–377. doi:10.3969/j.issn.1000-1700.2019.03.016
Keywords: cottonseed, compressive mechanical properties, moisture content, compression orientation, failure energy
Citation: Zheng Y, Wang M, Du X, Zhang H, Wang L, Wei X, Fu X and Wang J (2023) Compressive mechanical properties of cottonseed. Front. Mater. 10:1322301. doi: 10.3389/fmats.2023.1322301
Received: 17 October 2023; Accepted: 30 November 2023;
Published: 22 December 2023.
Edited by:
Domenico De Tommasi, Politecnico di Bari, ItalyReviewed by:
Kailu Xiao, Texas A&M University, United StatesPaulo Carteri Coradi, Federal University of Santa Maria, Brazil
Copyright © 2023 Zheng, Wang, Du, Zhang, Wang, Wei, Fu and Wang. This is an open-access article distributed under the terms of the Creative Commons Attribution License (CC BY). The use, distribution or reproduction in other forums is permitted, provided the original author(s) and the copyright owner(s) are credited and that the original publication in this journal is cited, in accordance with accepted academic practice. No use, distribution or reproduction is permitted which does not comply with these terms.
*Correspondence: Hongwen Zhang, emh3X21hY0BzaHp1LmVkdS5jbg==
†These authors have contributed equally to this work and share first authorship