- 1School of Electrical Engineering and Information Engineering, Lanzhou University of Technology, Lanzhou, China
- 2State Grid Gansu Electric Power Company, Electric Power Research Institute, Lanzhou, China
- 3State Grid Gansu Electric Power Company, Lanzhou, China
- 4State Key Laboratory of Power Transmission Equipment Technology, School of Electrical Engineering, Chongqing University, Chongqing, China
Power transformers are critical equipment responsible for the transmission and transformation of electrical energy, and they are an important component of power system. Under the combined effects of electric field, thermal field, and mechanical stress, insulation degradation is prone to occur in oil-paper insulation structures. Among them, the “liquid-solid” interface formed by oil and oil-immersed pressboard is the weak point in transformer insulation, and extensive operational experience has shown that creeping flashover of insulating pressboard is a common fault in power transformers. Creeping flashover of insulating pressboard can rapidly destroy the insulation structure, leading to irreversible damage to the transformer and posing a serious risk of transformer accidents. In recent years, many scholars have conducted in-depth research on the characteristics and mechanisms of creeping flashover in oil-paper insulation structures using various experimental methods. They have obtained significant findings that have important theoretical and practical implications for optimizing the structure of power transformers and improving their reliability. This paper first introduces the characteristics of the electromagnetic environment inside the power transformer and the common forms of creeping flashover accidents in engineering. Then, classified according to the uneven degree of electric field and creepage distance of test electrodes used in relevant research, and the test methods and flashover characteristics of small size (<5 cm) and large size insulating pressboard under extremely uneven and slightly uneven electric fields are introduced respectively. Secondly, the existing breakdown model and mechanism theory of oil-paper insulation structure creeping flashover are summarized. Finally, the limitations of the existing creeping flashover test method of insulating pressboard are analysed, and the key issues to be solved are condensed. This review aims to provide readers with a comprehensive overview of the test methods, characteristics, and mechanisms of insulating pressboard creeping flashover.
1 Introduction
Power transformers are the core equipment of the power system. Among the four links of generation, transmission and transformation, distribution and consumption, transformers are inseparable from the first three links (Coltman, 2002). With the construction of ultra-high voltage projects, voltage levels continue to increase and operating conditions become increasingly complex. New requirements have been highlighted for the internal insulation structure of transformers based on oil-paper insulation (Muthanna et al., 2006; Metwally, 2011). In the early operation of the UHV project, the overall operating performance of transformers, converter transformers, high-resistance equipment and other equipment was good. However, during the factory testing and operation stages, several insulation material discharge failures occurred, causing large economic losses. Based on China’s Yazhong Nanchang project as an example. Among the eight converter transformer factory test failures, 7 were related to insulation materials, accounting for 87.5%. Inside the power transformer, under the coupling action of multiple fields such as electric field, thermal field and mechanical stress, insulation degradation is prone to occur and space charges accumulate (Cho et al., 2014; Zhang et al., 2022; Meng et al., 2023a). At the same time, the mismatch between the dielectric parameters and conductivity parameters of oil-paper insulation will also cause serious accumulation of interface charges (Zhu et al., 2017). Therefore, the “liquid-solid” interface formed by oil and oil-impregnated pressboard is the insulation weakness of the transformer.
Oil-paper insulation creeping flashover is a transformer fault with a high probability of occurrence and great harm. Therefore, the power industry has been committed to avoiding the occurrence of creeping flashover by improving the performance of insulation materials and optimizing the design of insulation structures (Krause, 2012; Yea et al., 2018). For example, choose insulating pressboard with better creepage performance (Chen et al., 2019), use three-element mixed insulation oil with excellent insulation performance (Hao et al., 2023), improve the electrode shape, increase the tip electrode radius, avoid sharp corners and protrusions on structural parts, reliable insulation coverage of high potential areas (Fleszyński et al., 1982; Beroual and Fleszynski, 2003; Beroual and Fleszynski, 2006), the use of small oil gap structures, and the use of a large number of prefabricated shaped angle rings to replace traditional soft angle rings (Moser, 1979; Giese, 1996), try to avoid wedge-shaped oil gaps and other methods in the structure. Through the above means, the extremely uneven electric field has been eliminated inside the UHV transformer, but the occurrence of creeping flashover accidents of the insulating pressboard has not been eliminated. Therefore, in-depth research on the creeping flashover characteristics and mechanism of insulating pressboard is of great significance to improving the reliability of power transformers.
Currently, pin-plate (Mitchinson et al., 2008; Lv et al., 2014; He, 2020) and rod-plate (Li et al., 2009; Prevost, 2009) are mostly used in the research on creeping flashover of insulating pressboard. Equi-electrode structure, conduct research on creeping flashover characteristics and mechanism of small size (<50 mm). The creeping flashover of the small-size model starts from the partial discharge in the high electric field intensity area, that is, the tip of the needle in the extremely uneven electric field formed by the needle-plate electrode, and the wedge-shaped oil gap tip of the slightly uneven electric field formed by the rod-plate electrode. Due to the complex electrode structure design and difficulty in testing, there are relatively few reports on large size (>50 mm) creeping flashover tests (Okubo et al., 1987; Prevost, 2009). Through a large number of tests of creeping flashover at different scales (1∼330 mm), Weidmann co. provided a curve of electric field strength of insulating pressboard materials, which has great reference value for the optimization design of transformer structures (Moser, 1979). It is of great significance to obtain the creeping flashover scale effect of insulating pressboard through large-scale creeping flashover tests. However, there are still relatively few related studies at present. Comprehensive articles on the test methods, characteristics, and mechanisms of creeping flashover of insulating pressboard are extremely rare.
This paper will give a detailed overview from four aspects: the internal electromagnetic environment of the power transformer and the creeping flashover accident form, the creeping flashover test method and characteristics of the oil-paper insulation structure, the scale effect of the insulation pressboard flashover, and the creeping flashover mechanism of the oil-paper insulation structure. At the same time, the current problems faced in the research on creeping flashover of oil-paper insulation structures are summarized, and the possible future research directions in the research on creeping flashover of insulating pressboard are briefly described. This paper has significant innovative and practical value in deepening the understanding of creeping flashover phenomena in insulating paperboard and improving the design and operation of power transformers.
2 Insulating pressboard operating conditions and creeping flashover accidents
2.1 Electromagnetic environment inside the UHV transformer
The electromagnetic environment in UHV transformers is very complex, and the distribution of electrical stress in various parts is significantly different. The equipotential lines inside the transformer are shown in Figure 1 (Moser, 1979). Common forms include flat plate uniform electric field, cylindrical slightly uneven electric field, coaxial cylindrical slightly uneven electric field, mutually perpendicular cylindrical slightly uneven electric field, tip-to-tip uneven electric field, tip-to-plate uneven electric field (Xie, 2014). Classified according to the degree of unevenness of the electric field, it can be divided into slightly uneven fields and extremely uneven fields. For example, the electric field is designed to be slightly uneven at the angle rings and spacers. In the current structural optimization design of UHV transformers, engineers have achieved the goal of reducing and eliminating extremely uneven fields as much as possible by adopting a thin paper tube-small oil gap structure, high potential parts coated with pulp, use prefabricated insulation and other measures. However, there are still great difficulties in realizing an ideal uniform field because there are extremely uneven electric fields caused by defects such as burrs, impurities, and bubbles (Elborki et al., 2002; Ribeiro et al., 2009).
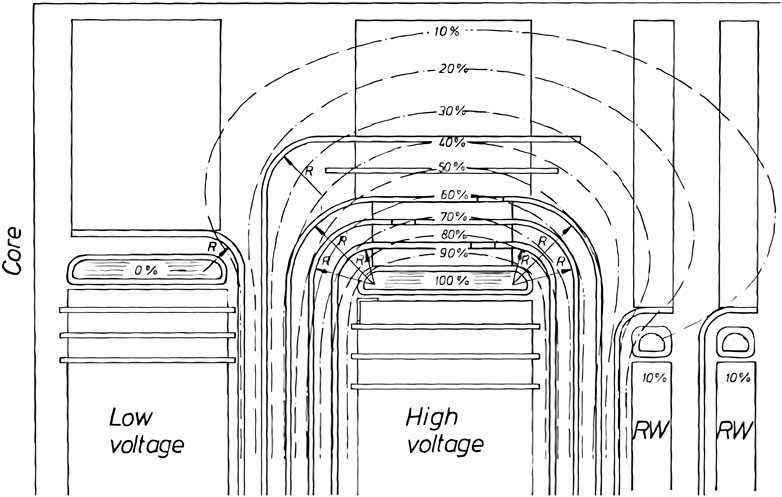
FIGURE 1. Equipotential lines inside the transformer (Moser, 1979), R is the radius of the angle rings, RW is regulating winding.
According to the distribution characteristics of electrical stress on insulating oil and insulating pressboard, it can be divided into normal and tangential components. The superposition of normal and tangential components can express various types of electrical stress (Hekmati, 2017). The schematic diagram of the tangential component and the normal component is shown in Figure 2A. The normal component corresponds to the breakdown, while the tangential component corresponds to the creeping flashover. Based on this, the main structures that cause creeping discharge of insulating pressboard inside the transformer can be divided into two types: one is that the electric field line is basically along the “liquid-solid” interface, that is, there is only the tangential component and the normal component is very small, the existence of solid dielectric does not change the discharge path between electrodes, as shown in Figure 2B mainly tangential; second, the electric field line passes through the interface of the solid and liquid, and the tangential component and the normal component exist at the same time. At this time, the discharge path between the two electrodes is changed due to the presence of the solid dielectric, as shown in Figure 2B mainly normal. Research has shown that a structure with both tangential and normal components easily forms a slide discharge structure, resulting in a creeping flashover voltage of oil-paper insulation that is much lower than that of pure tangential components (Xie, 2014). Therefore, in transformer design, the tangential component of the surface of the insulator should be avoided as much as possible, that is, the shape of the solid dielectric should be matched to the equipotential lines (Nelson, 1989).
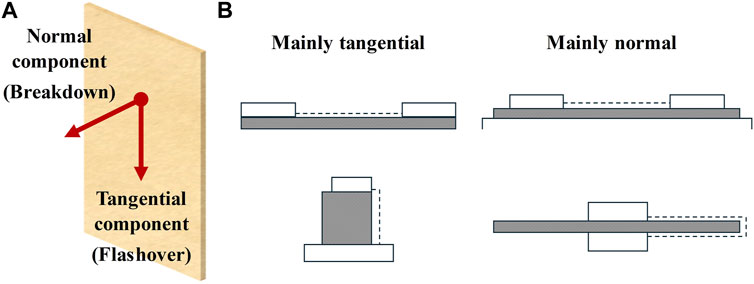
FIGURE 2. Schematic diagram of the electric field characteristics of insulating pressboard in power transformer. (A) Normal and tangential components on insulating pressboard. (B) Distribution characteristics of electrical stress on insulating pressboard. The dotted lines are electric field lines and the rectangle filled with diagonal lines is the solid dielectric.
It can be seen that the current electric field distribution in UHV transformers is mainly a slightly uneven field, and the normal component and the tangential component can be used to analyse the form of electrical stress at the “liquid-solid” interface of the oil-paper insulation structure.
2.2 Common forms of creeping flashover of insulating pressboard
Based on literature research and engineering experience, this paper summarizes some common forms of creeping flashover of oil-paper insulation structures during operation and testing. Among them, the creeping flashover form that starts from the pad and develops on the screen is shown in Figure 3A. Creeping discharge first occurs on the insulating spacer between winding layers, then develops on the surrounding screen, and finally complete flashover (Qi et al., 2016). Another form is creeping flashover that occurs at top of screen, as shown in Figure 3B. First, it breaks down a layer of screen, and then creeps around the angle ring and along the top of screen until the low-voltage electrode completely flashover (Xie, 2014). In addition, the creeping flashover developed from the screen to the tensile belt of clamping is shown in Figure 3C. In this case, the screen is first broken down, then develops to the tensile belt of clamping, and creeps up to the lower yoke to complete the flashover (Xie, 2014) By analysing the electric field distribution characteristics of these common creeping flashover forms, it is found that the flashover starting area is a creeping discharge caused by a strong tangential component, or a local breakdown caused by partial discharge. The flashover development area is in areas with strong tangential components and weak normal components, such as screens, etc. This is the insulation structure that easily forms a slide discharge structure. Understanding the common accident forms of creeping flashover of insulating press board plays an important role in guiding the design of test simulation electrodes.
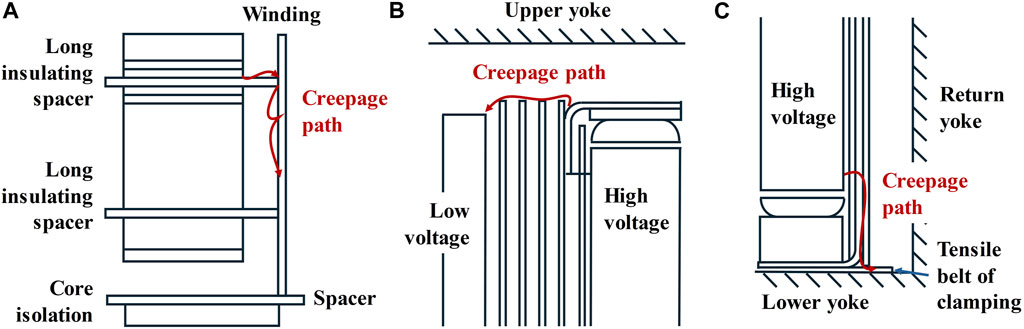
FIGURE 3. Creeping flashover of insulating pressboard is a typical accident form. (A) Creeping flashover occurring between the spacer and the screen (Qi et al., 2016). (B) Creeping flashover at the top of screen (Xie, 2014). (C) Creeping flashover on screen and tensile belt of clamping (Xie, 2014).
3 Small size creeping flashover test method for insulating pressboard
This paper defines the test of oil-paper insulation with a creepage distance of less than 50 mm as a small size creeping flashover test. Based on the electric field non-uniformity coefficient of the electrode structure used in the test, the following describes the flashover test methods of insulating pressboard under extremely uneven fields and slightly uneven fields.
3.1 Small size creeping flashover test method of insulating pressboard under extremely uneven conditions
Although the optimized design of the transformer has eliminated the extremely uneven electric field as much as possible, due to the existence of defects, the extremely uneven electric field still exists in the transformer. Scholars have carried out a large number of studies on small size creeping flashover under extremely uneven fields and obtained many useful conclusions, so they are reviewed first.
For creeping discharge under extremely uneven fields, scholars most often use needle-plate electrodes for simulation. The standard needle-plate creeping discharge model is given in IEC 61294. The tangential components of the needle-plate electrode is very strong. Creeping flashover can occur at lower voltages, and the requirements for high voltage source are not high, so needle-plate electrodes are used in many studies. It is worth noting that different needle curvature radii will lead to differences in the uneven coefficient of the electric field, thereby affecting the test results. The standard states that the needle electrode should be a tip with a tip curvature radius of 3 μm.
Dai et al. (2010) used a needle electrode pressed on insulating pressboard and a plate electrode close to the edge of the board as electrode structures to explore the effect of moisture and other aging by-products in pressboard on creeping flashover. Study have shown that an increase in moisture content in pressboard significantly reduces PD initial voltage. When the moisture reaches about 3%, the partial discharge initial voltage (PDIV) drops by about 30%. Mitchinson et al. (2008), Mitchinson et al. (2010), Zainuddin et al. (2011) designed a needle-bar electrode, and the electrode structure is shown in Figure 4A. The study found that white marks due to partial discharge will appear in the early stage of creeping flashover, as shown in Figure 4B. Then the white marks disappear and carbonization channels are formed, causing damage to the pressboard. At the same time, an oil-paper insulation interface model was proposed. The surface morphology shown shows that the surface of the insulating pressboard is uneven and there are cellulose chains extending outward, as shown in Figures 5A, B. Therefore, there is a transition zone between transformer oil and insulating pressboard that is not very clear. There is a transition zone, a no-slip layer (equivalent to the Stern layer in the electric double layer theory) and a boundary layer between the oil-impregnated paper and the oil, as shown in Figure 5C. Research has found that the creeping discharge and tracking processes at lower energies are related to the drift of space charge in the EDL and oil/pressboard transition zone. For the creeping flashover process at higher energy, it is related to the charge transport in the oil boundary layer. Lv et al. (2014) used the needle-plate model to study the effect of TiO2 on creeping flashover of insulating pressboard, and at the same time, the space charge was measured. Test results show that TiO2-doped insulating oil can increase the flashover voltage of insulating pressboard by 14.8%. TiO2 can reduce the shallow trap density and shallow trap energy level, thereby accelerating the charge dissipation rate at the interface between oil and pressboard. Qi et al. (2015) used needle-plate electrodes to study the impact of space charge effect on flashover voltage when polarity reversal occurs. The change density is measured at the oil-paper interface before and after DC polarity reversal, and found that the induced potential generated by the interface charge can reach 40% of the polarization voltage. The electric field generated by the interface charge can enhance the maximum field strength of the needle electrode after polarity reversal, thereby greatly reducing the flashover voltage. Li et al. (2018) used needle-bar electrode to explore the creeping discharge characteristics under combined AC and DC voltages. The test results show that creeping flashover is significantly affected by the DC component, because there will be a large number of positive space charges near the needle tip, which promotes the occurrence of flashover. At the same time, creeping flashover develops in the free oil boundary above the oil-immersed pressboard, so quality control of the oil is very important. Chongqing University, (2020) used needle-plate electrodes to study the streamer characteristics of creeping flashover of 5∼50 mm vegetable oil and oil paper insulation structures under power frequency voltage. The electrode structure is shown in Figure 4C. Research has found that different types of insulating oils have differences in streamer characteristics due to differences in molecular ionization energy and viscosity. Insulating oil with higher viscosity can slow down the electron migration rate and restrict the development of streamers, resulting in the streamer development speed and streamer stop length are both lower at the same voltage. Regarding the space charge effect, the impact of the space charge on the head of the streamer on the spatial electric field distribution during the streamer development process leads to differences in the shape of the positive and negative half-cycle streamers, and the development degree of the positive half-cycle streamer is significantly stronger than that of the negative half-cycle streamer. Under the same voltage polarity, the space electric field formed by the space charge generated by the previous discharge has a certain inhibitory effect on the development of subsequent discharges, but when the voltage develops to the polarity conversion, the space charge has a promoting effect on the development of subsequent discharges.
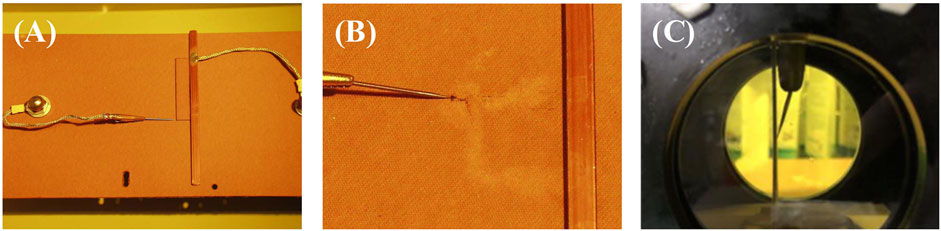
FIGURE 4. Some typical creeping flashover models with extremely uneven electric fields. (A) Picture of needle-bare structure (Mitchinson et al., 2008). (B) Pressboard with 30 kV at 35 mm at 9 h (Mitchinson et al., 2008). (C) Picture of the needle plate structure (He, 2020).
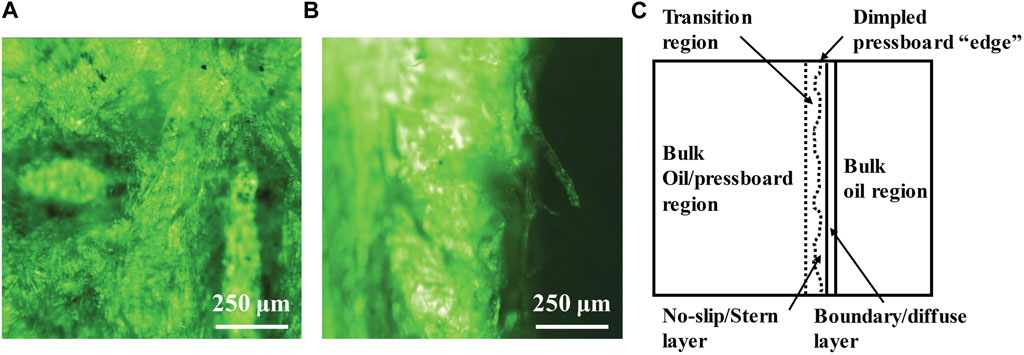
FIGURE 5. Oil-paper insulation structure interface model (Mitchinson et al., 2010). (A) Morphology of dry pressboard surface. (B) Microtome of pressboard edge. (C) Model diagram.
It is worth noting that in many studies, the back electrode structure is introduced to regulate the size of the normal component of the insulating pressboard to better simulate the real conditions of the transformer. Zhou et al. (2013) designed a rod-rod electrode structure with a back electrode based on the unique operating characteristics of the valve side winding of the converter transformer. The test results show that under AC/DC composite stress, the existence of the tangential component causes the AC flashover voltage to decrease, but has no effect on the DC flashover voltage. Murdiya et al. (2014) used needle electrodes and fixed back electrodes as test electrodes, and the schematic diagram of the model is shown in Figure 6A. The paper explored the difference in creeping discharge characteristics of vegetable oil and mineral oil under AC. The streamer rate in rapeseed oil and mineral oil is faster and has more branches than PF AE oil. At the same time, it was observed that obvious white marks appeared on the surface of the pressboard during the creeping discharge process, which was due to the drying process caused by the Joule heating effect. Li et al. (2021) used a needle-plate electrode structure with a back electrode. By adjusting the back electrode, the creeping discharge under the combined action of tangential and normal electric fields can be simulated, as shown in Figure 6B. The test results show that the development process of creeping discharge can be divided into three stages based on the apparent charge amplitude and partial discharge repetition rate. The time-resolved PD modes and equivalent time-frequency modes of the strong and weak vertical component models are different. Most discharge pulses are single peaks with underdamped oscillations, but double peak pulses may occur in weak vertical component models.
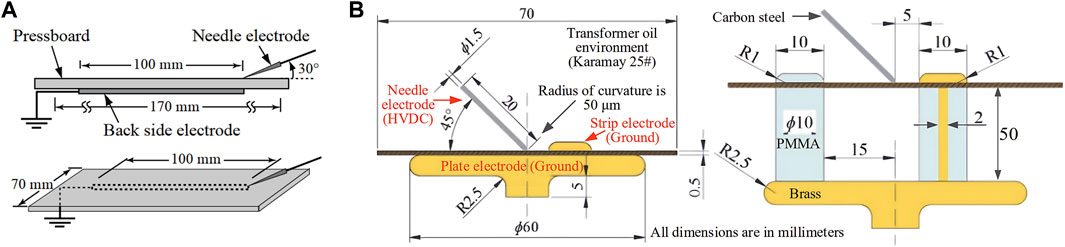
FIGURE 6. (A) Creeping flashover model with back electrode structure (Murdiya et al., 2014). (B) Electrode structure with adjustable normal component (Li et al., 2021).
It can be seen that the small size creeping flashover test method under extremely uneven electric fields based on the needle-plate electrode structure has been very mature. Scholars have carried out a lot of research and obtained rich creeping flashover characteristics of oil-paper insulation structure. However, it is worth noting that the needle-plate electrode is essentially used to study partial discharge. The IEC 61294 that everyone refers to is itself the standard for partial discharge. The research conclusions also mostly focus on partial discharge during creeping discharge and creeping flashover. There are few studies on the flashover characteristics along the surface under different creepage distances. Creeping flashover under extremely uneven fields starts from partial discharge in the high electric field intensity region (Hussain et al., 2021), that is, at the tip of the needle-plate electrode. Therefore, the test results depend more on the electric field intensity at the “three-junction point” formed by the electrode, oil, and pressboard, and have a weak connection with the intrinsic properties of the pressboard. Therefore, the use of needle-plate electrodes to carry out research on creeping flashover of insulating pressboard is suitable for partial discharge research during creeping discharge, but is not suitable for performance assessment of oil and pressboard.
3.2 Small size creeping flashover test method of insulating pressboard under slightly uneven electric field
IEC 60234-1 recommends using column-plate electrodes to conduct creeping discharge tests under slightly uneven conditions, simulating creeping discharge under the combined action of tangential and normal electric fields. At the same time, some scholars have realized the limitations of using needle-plate electrode structures to study creeping flashover of oil-paper insulation structures, and have designed more electrode structures with different electric field components.
Li et al. (2009) set up a triple-junction model with a column-plate electrode structure to explore the characteristics from partial discharge to complete flashover. During the flashover process, corona discharge first occurs at the electric field enhancement point, and then streamer is generated and developed at the interface between oil and pressboard, and finally develops to complete flashover. The study pointed out that the obvious difference in the partial discharge characteristic quantity under different applied voltages can be used to detect the creeping discharge form at the interface between oil and pressboard. Weidmann co., (2009) published a “Weidmann oil-paper insulation creeping flashover strength design curve,” which guides the design of transformers based on mineral oil. Weidmann co. realized the potential of natural esters and modified the curve to suit the design of transformers based on natural esters. In order to compare the different dielectric properties of mineral oil and natural ester in oil-paper insulation structure, a “U”-shaped electrode structure with a creepage of 35 mm was designed. The high-voltage electrode is composed of a “U”-shaped copper tube. Kraft paper is wrapped around the high voltage electrode, the ground electrode will be a metal disc with machined radius edges, and a pressboard disc is placed on top of the ground electrode as shown in Figure 7A. This electrode structure has a strong tangential interface electric field. At the same time, by optimizing electrode parameters and insulation coating, it eliminates sharp points in the electrode structure and any wedge-shaped oil gaps that may produce partial discharge, so that the occurrence of creeping flashover is minimized. It may be related to the size of the tangential interface electric field and the performance of the oil-paper insulation structure. The test results show that the creeping flashover strength (1% breakdown probability) of natural ester is very close to that of mineral oil. Considering that the relative dielectric constant of natural ester is higher and closer to cellulose, which can improve the electric field distribution inside the transformer, natural ester has good application potential. Qi et al. (2016) used column-plate electrodes to simulate creeping flashover that occurs on the insulating spacer of the converter transformer winding shielding layer. The electrode structure is shown in Figure 7B. Voltages are applied in series during the test, with a DC voltage on the column electrode and an AC voltage on the plate electrode. The average amount of gas from the start of creeping discharge to surface flashover under the AC/DC composite field is smaller than that under the pure AC electric field. In the development process from creeping discharge to flashover, C2H6 and C2H2 appear in the AC/DC composite electric field, while CH4 and C2H2 dominate in the AC electric field. The test results show that the gas composition is related to the voltage phase, so the characteristics of the gas dissolved in the oil can be used to provide a basis for the initial diagnosis of creeping flashover of the converter. Li, (2018) used needle-plate electrodes and column-plate electrodes to simulate the creeping flashover phenomenon of oil-paper insulation under extremely uneven electric fields and slightly uneven electric fields under AC/DC combined conditions. The results show that the DC component under an extremely uneven electric field causes discharge to only appear in the positive half cycle of the AC component, making it difficult to produce white marks. The DC component under a slightly uneven electric field has little effect on the discharge phase distribution, statistical characteristics and the generation of white marks on the pressboard surface. The white marks that appear on the pressboard are essentially gas channels, and significantly increase the discharge intensity and development speed. Their occurrence is affected by the electrode electric field distribution, geometric structure, and moisture in the pressboard. Qin, (2020) used a ball-ball electrode structure to explore the creeping flashover characteristics of oil-paper insulation under a DC/AC combined voltage of 30 mm size, as shown in Figure 7C. The test results show that the AC/DC composite flashover voltage is the largest, followed by the flashover voltage under DC voltage, and the AC flashover voltage is the smallest; the AC discharge current starts from the “three-junction point” at both ends of the electrode at the same time, and the DC discharge starts at the same time. The current injection starts from the “three junction points on the high-voltage side,” and the AC/DC discharge current injection starts from the “three junction points on the high-voltage side”.
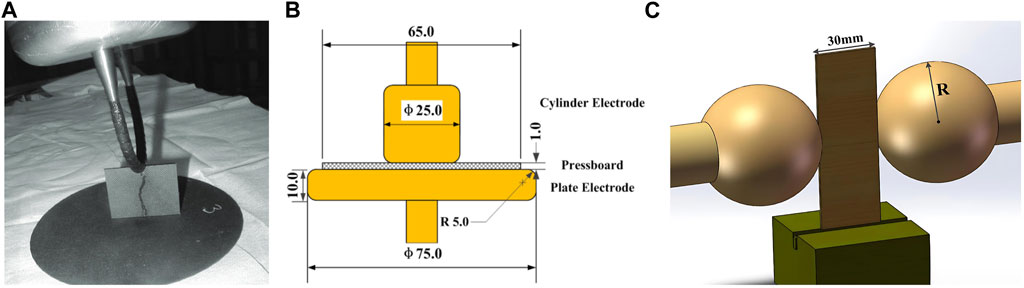
FIGURE 7. Some slightly uneven electric field creeping flashover models along the surface. (A) Photo of “U" shaped electrode structure (Prevost, 2009). (B) Schematic diagram of column-plate model (CIGRE Method Ⅱ) (Qi et al., 2016). (C) Photo of ball-ball electrode structure (Qin, 2020).
In general, small size test models with electrode structures such as needle-plate, column-plate, ball-ball, etc., with a creepage distance within 50 mm are used to conduct creeping flashover. Most research focuses on different voltage stresses, different physical and chemical insulating materials, and Effects of dielectric properties and different test conditions on creeping flashover performance. Many useful conclusions were obtained, which may have certain guiding significance for transformer insulation material selection, operation and maintenance, and structural design.
4 Large size creeping flashover test of insulating pressboard and scale effect
Due to the high requirements of the test platform, difficulty in model processing, large test dispersion, and long test cycle, there are very few studies on using large size (>50 mm) models to carry out creeping flashover tests of insulating pressboard. It is worth noting that by changing the creepage distance and large scale creeping flashover test, the scale effect can be obtained, and the allowable surface field strength curve of the insulating pressboard can be obtained, thereby guiding the design of the insulation structure of the power transformer. Therefore, this section will review the large size creeping flashover test method and scale effect of insulating pressboard.
4.1 Large size creeping flashover test method of insulating pressboard
Okubo et al. (1987) used a double-circular electrode structure with a back electrode to study the scale effect of creeping flashover of an oil-paper insulation structure under impulse voltage. By testing oil-impregnated paper cylinders with a larger size of 25–960 mm, the electrode structure is shown in Figure 8A. The designed model adjusts the normal component of the electric field of the “three junction points” by adjusting the length of the back electrode, and simulates the screen area with strong back electrode effect (strong normal, weak tangential), as shown in Figure 8B and the insulation surface of a lead support with weak back electrode effect (weak normal direction, strong tangential direction), as shown in Figure 8C. The test results show that the stronger the normal phase component, the smaller the flashover voltage, as shown in Figures 8D, E. At the same time, this paper used crepe paper to cover high-voltage electrodes to simulate the paper pulp coating measures for live locations in power transformers. Research has found that the initial discharge voltage of partial discharge increases significantly after the electrode is insulated and coated. At the same time, once creeping discharge occurs, it will quickly lead to flashover. Zhang et al. (2022) designed double-ball electrodes with a creepage distance of 50∼150 mm to simulate creeping flashover on the outer angle ring of the converter transformer where the tangential component dominates. Experimental results show that as the distance increases, the AC creeping flashover voltage increases, but the average field strength decreases significantly, and the approximate exponential law decreases. For insulation structures where the tangential component dominates, the insulation margin needs to increase as the creepage distance increases.
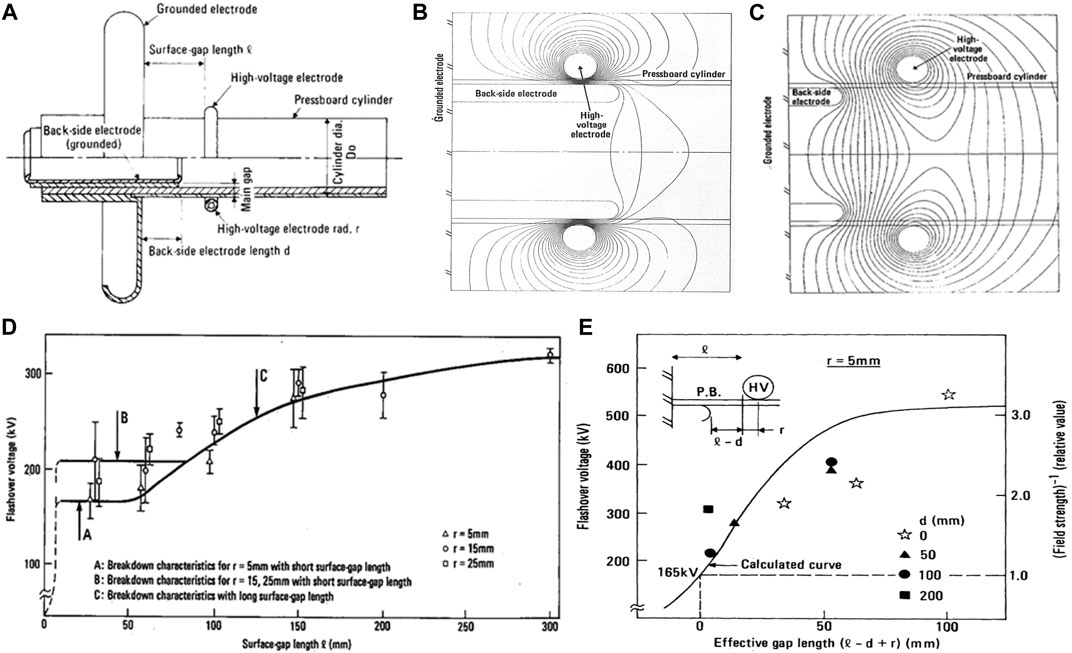
FIGURE 8. The electrode structure used and the obtained creeping flashover characteristic curve Okubo et al. (1987). (A) Schematic diagram of the double-ring electrode structure with an adjustable back electrode. (B) Equipotential lines with strong back electrode effect. (C) Equipotential lines with weak back electrode effect. (D) The relationship between creeping flashover impulse voltage and creepage distance under strong back electrode effect. (E) The relationship between creeping flashover impulse voltage and creepage distance under weak back electrode effect.
According to a small number of studies of large size creeping flashover of insulating pressboard, the electric field form of the double-circular and double-ball electrodes is slightly uneven. The required impact and AC flashover voltage are quite high, and the capacity requirements of the test power supply are very high.
4.2 Scale effect of creeping flashover of insulating pressboard
The volume effect can be used to explain the scale effect of creeping flashover of insulating pressboard (Nelson, 1989) Due to the inhomogeneity of materials and processes, from a probabilistic perspective, the larger the volume, the higher the possibility of defects in the insulator. For example, a large amount of insulating oil can serve as a reservoir for aging products such as gas, moisture, and impurities. Under the action of an electric field, the particles will move to areas where electrical stress is concentrated, playing a positive role in breakdown. According to the literature search results, only a few studies have revealed the creeping flashover scale effect of insulating pressboard.
Okubo et al. (1987) conducted experiments on oil-impregnated paper tubes with a larger size of 25–960 mm. A flashover model with model proportions S of 0.5, 1, 3, and 6 is designed. The test results show that the creeping flashover impulse voltage Vfo of the oil-paper insulation increases nonlinearly by Vfo∝S0.73 as the size increases. Weidmann co. has carried out a large number of relevant basic research on many key issues of oil-paper insulation structure. Over the years, it has formed complete integrated research from insulation structure design, basic material property research, to material production and processing. However, its related research results are regarded as its core technology is confidential and rarely disclosed to the outside world. Weidmann co., (1979) gave a curve about the 50 Hz AC electric field strength along the surface of insulating pressboard materials, as shown in Figure 9A, but the curve was not explained in detail. The “Weidmann curve” is a partial discharge-free curve with a withstand voltage of 1 min, which is obtained through a large number of experiments. Nelson, (1989) details the design guidelines for insulation within transformers. The “Weidmann curve” is an empirical curve in which different electric field distributions caused by geometric characteristics have been fully considered, so the characteristics are not uniform in a set of curves. Plotting the “Weidmann curve” on the log-log coordinate system, it can be found that the curve satisfies the negative power rate, as shown in Figure 9B.
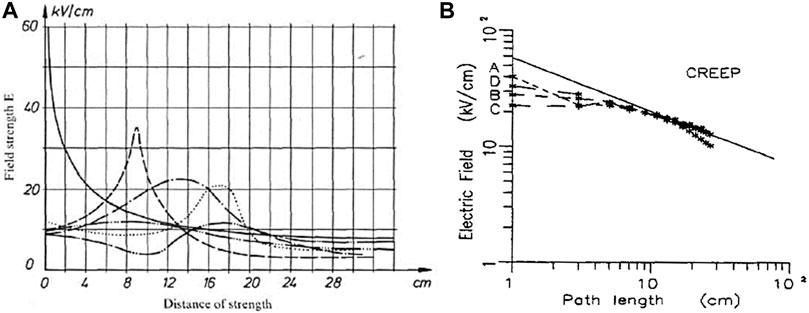
FIGURE 9. Scale effect of creeping flashover of insulating pressboard. (A) “Weidmann curve”, Allowable surface field strengths of transformerboard. Dry, oil-impregnated, between insulated electrodes. Test frequency 50 Hz. 1 min, PD free (Moser, 1979). (B) The “Weidmann Curve” plotted on a log-log coordinate system (Nelson, 1989).
Although there are relatively few studies on creeping flashover of insulating pressboard, the conclusion that “the relationship between failure field strength and creepage distance satisfies the negative power ratio” has become a consensus, which has guiding significance for future research.
5 Creeping flashover mechanism of insulating pressboard
For liquid dielectric breakdown and liquid/solid interface, common breakdown or flashover mechanisms include: streamer theory, electron theory, bubble discharge theory based on current thermal effect and electrostatic repulsion between ions, and small bridge based on impurity breakdown theory, etc. (Huang, 2013). Compared with solid and gas dielectric that already have relatively mature breakdown models and mechanisms, there are currently no complete breakdown mechanisms for liquid dielectric and flashover mechanisms along the solid/liquid interface that can explain all test phenomena. Among the above mechanisms, streamer theory is a widely accepted theory. The high conductivity channel before arc breakdown is named “streamer”, and the formation and development process of streamer is named “pre-breakdown” process.
5.1 Streamer theory
The streamer characteristics of the flashover process at the interface of solid/liquid composite insulation systems are more complex than those of the liquid dielectric breakdown process, but they have many levels of commonality (Huang, 2013). The development of streamer in liquid dielectric includes electronic processes and gas phase processes (Zhao, 2017) In the 1950s, the theory of electron process streamer in liquid dielectric was established (Anderson and Liao, 1955). Subsequently, scholars used advanced optical measurement equipment to discover the existence of a large number of free carriers before the penetrating arc (Lesaint et al., 1991; Meng et al., 2023b; Lin et al., 2023), confirmed the existence of electronic processes during the development of streamer. The existence of electronic processes indicates that the streamer is a plasma channel. In addition, many studies have shown that the use of nano-modified insulating oil that can adsorb carriers can significantly change the streamer characteristics (Lei, 2019), which also confirms the existence of electronic processes. Krasucki and Bowden, (1966) established the streamer theory cantered on the observation of bubbles. The existence of gas phase processes indicates that the streamer is a low-density gas channel. Many studies have shown that oil hydrostatic pressure has an impact on the streamer development process (Kattan et al., 1989; Hu et al., 2019), and also confirmed the existence of bubbles during the discharge process, which later caused pre-breakdown and breakdown of the liquid dielectric. The streamer theory of gas phase processes is widely accepted in research.
Lundgaard et al. (1998) proposed that the creeping discharge process of oil-impregnated pressboard includes four stages. In the first stage, only partial discharge occurs at the needle electrode. At this time, the partial discharge cannot be observed by the naked eye and can only be detected by a partial discharge detector. In the second stage, when the voltage continues to increase, a slightly visible corona discharge occurs on the needle electrode side. In the third stage, when the applied voltage continues to increase, significant streamer discharge will occur on the needle electrode side. In the fourth stage, when the voltage rises above a certain value, a bright arc will be generated along the surface and penetrate the needle-plate electrode instantly. At the same time, the polarity effect of streamers was studied. Under twice the creeping flashover voltage, the average velocity of positive streamers is 2∼4 mm/μs. When the voltage continues to increase, rapid streamers >100 mm/μs will appear; The breakdown voltage of negative streamers is twice that of positive streamers, which is related to the slower development speed of negative streamers. Zhang et al. (2021) explored the effect of dielectric constant matching of oil-paper insulation structure on streamer propagation. The closer the ratio of the dielectric constants of insulating oil and oil-immersed pressboard to 1, the weaker the space charge accumulation at the solid-liquid interface, the more uniform the electric field distribution, and the shorter the streamer propagation length. He et al. (2022) simulated the wedge-shaped oil gap in the rod-plate electrode structure using hydrodynamic drift diffusion theory and bipolar charge transfer theory, as shown in Figure 10. The results show that the creeping discharge process of the wedge-shaped oil gap at the insulating oil-paper interface under the rod electrode can be divided into four stages: corona in the oil, surface discharge at the oil-paper interface, longitudinal development inside the pressboard, and creeping flashover. The flashover voltage shows a polar effect, and the negative flashover voltage is higher than the negative flashover voltage. This polar effect is related to the adsorption of negative charges by cellulose in the insulating pressboard. The adsorbed negative charges weaken the electric field between the electrodes when the voltage polarity is negative, and enhance the electric field when the voltage polarity is positive. Wang et al. (2023) constructed a hydrodynamic drift-diffusion model in oil, a bipolar charge transport model in insulating pressboard, and an Ohmic model of interface charge transport, and explored the convection changes in the surface morphology of the insulating pressboard caused by partial discharge. Pay attention to the impact of development. Compared with smooth surfaces, convex and concave surfaces will lead to more charge accumulation and accelerate the streamer development process. In contrast, convex surfaces have a greater impact than concave surfaces.
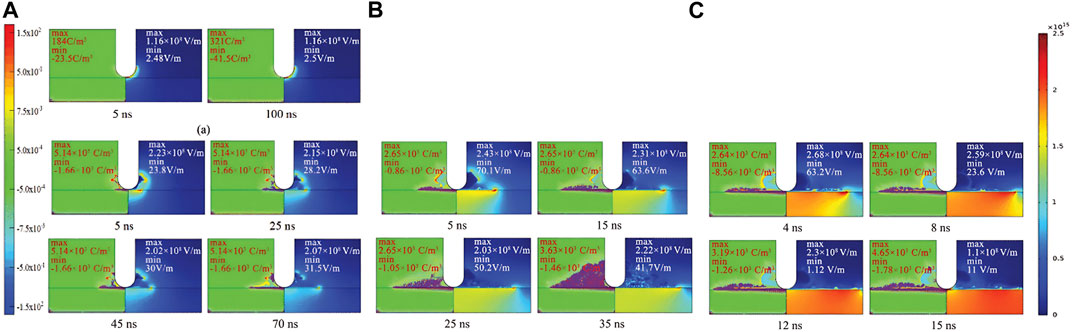
FIGURE 10. Simulation results of the creeping discharge process of wedge-shaped oil droplets under positive polarity voltage. The left is the charge distribution and the right is the electric field distribution (He et al., 2022). (A) 5 kV, discharge process within 0–100 ns. (B) 40 kV, discharge process within 0–70 ns. (C) 70 kV, discharge process within 0–35 ns. (C) 90 kV, discharge process within 0–15 ns.
Since Hwang, (2010) proposed a numerical simulation method for streamer development, many scholars have improved on this basis and explored the characteristics of streamer discharge through numerical simulation methods, which has greatly enriched the streamer theory of streamer discharge of insulating pressboard.
5.2 Streamer photography method
Different from the severe photothermal effect that occurs when creeping flashover of oil-paper insulation, the discharge phenomenon is weak and short in duration during the pre-discharge process of streamer development. In addition, insulating oil also has certain light-absorbing properties, and light will produce certain refraction phenomena when passing through different materials inside the tank, which increases the difficulty of streamer discharge observation (He, 2020). There are two types of oil-paper interface streamer photography: schlieren method and shadow method. The schlieren method can only shoot the side, which can reflect the streamer development trend of the oil-paper interface. The shadow method can shoot both the front and the side, and the front is more likely to be photographed. It reflects the number of all morphological branches of the streamer, so the shadow method has the advantage of showing comprehensive information on the development of the streamer (Settles, 2001).
Liu and Wang, (2011) photographed the front and side views of streamer discharge of oil-paper insulation, as shown in Figure 11. Due to the opaque characteristics of the insulating pressboard, it is difficult to take pictures of the front side of the streamer discharge along the surface of the oil-paper insulation. Ariza et al. (2017) built a high-speed photography shadow imaging platform and compared the streamer propagation characteristics of mineral oil-kraft paper and mineral oil-kraft fibril paper. The test results show that the surface roughness of the solid dielectric will affect the streamer branching. For mineral oil-kraft fibril paper with high dielectric constant and low surface roughness, the streamer develops close to the liquid-solid interface. For mineral oil-kraft paper with a high degree of coarseness, the streamer will propagate into the oil due to the presence of oil-filled pores on the solid surface. Ge et al. (2018) studied the effect of doping TiO2 nanoparticles in insulating oil on the streamer propagation process on the surface of insulating pressboard under lightning impulse voltage. Using the schlieren method, the development process of the streamer was photographed from the direction parallel to the pressboard using an intensified charge coupled device camera at a voltage of 60 kV. Research has found that doping TiO2 in insulating oil will cause the streamers to tend to float in the insulating oil instead of sticking to the surface of the insulating pressboard. The streamer images of pure oil-impregnated and nanofluid oil-impregnated are shown in Figures 12A, B respectively. This is related to the dielectric constant difference between the insulating oil and pressboard. Chongqing University, (2020) used the shadow imaging method to complete the acquisition of the dynamic process of streamer discharge with the help of a high-speed CMOS camera and a high-speed trigger recording system, and the streamer characteristics of creeping flashover of 5∼50 mm vegetable oil and oil-paper insulation structure are studied under power frequency voltage. The schematic diagram and physical diagram of the platform are shown in Figures 12C, D respectively. It can be seen from the figure that when the applied voltage is low, the streamer morphology generated by the creeping discharge is filaments that diverge and develop near the needle tip. As the applied voltage increases, the streamer will extend along the pressboard surface. Ariza et al. (2020) built a shadow imaging platform and used a non-intensified high-speed camera to obtain streamer propagation images on three types of kraft paper. The chemical composition of kraft paper will affect streamer propagation by affecting charge injection. Lignin-free paper has a lower dielectric constant and has a greater average charge injection than k107 kraft paper (high lignin content), streamers tend to propagate in the oil.
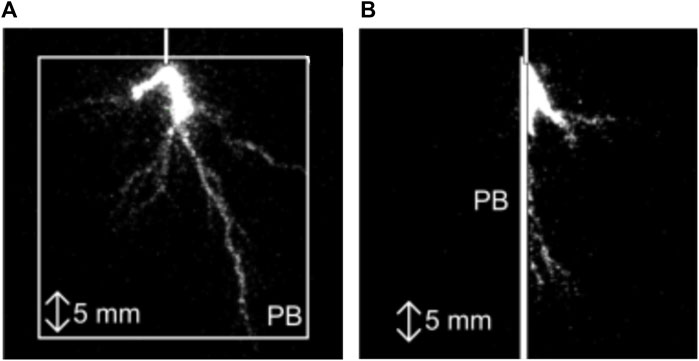
FIGURE 11. High-speed photography of negative streamer at −110 kV, 50 mm. The (A) shows the front of the streamer, and the (B) shows the side of the streamer (Liu and Wang, 2011).
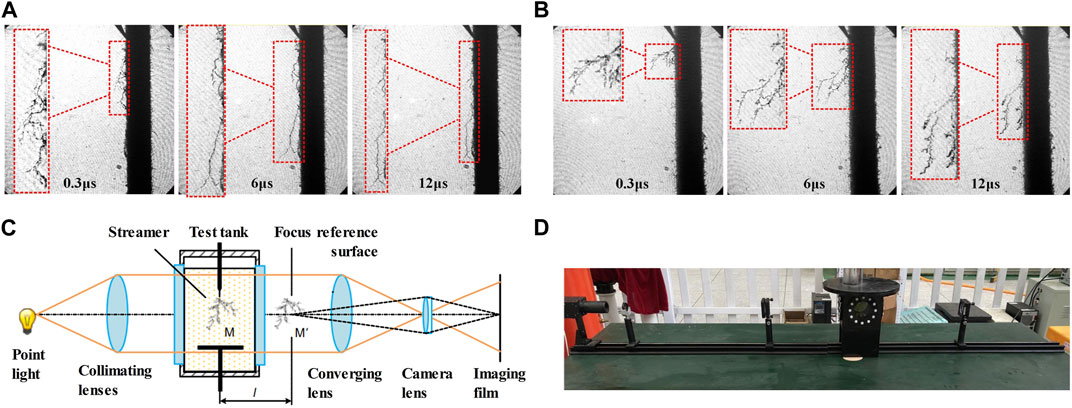
FIGURE 12. (A) Streamer propagation images for pure oil-impregnated image, 60 kV (Ge et al., 2018). (B) Streamer propagation images for nanofluid-impregnated images, 60 kV (Ge et al., 2018). (C) Schematic diagram of the streamer shadow imaging system (He, 2020). (D) Physical picture of the streamer observation system (He, 2020).
It can be seen that a large number of studies have used the shadow method to shoot side views of streamer development, and the photography method has become mature. Through the method of combining numerical simulation and experimental observation, the influence of streamer propagation mode, streamer propagation speed and material properties on streamer development were studied in depth, and gained a deeper understanding of the causes of creeping discharge of insulating pressboard. Since the insulating pressboard is opaque, it is difficult to obtain the front view of the streamer, and it is impossible to obtain the full view of the streamer.
6 Conclusion and outlook
In terms of creeping flashover test methods, small size (<50 mm) creeping flashover model standards have been completed. Researchers have used needle-plate and column-plate electrode structure models to carry out a large number tests The development process of creeping flashover of insulating pressboard under different voltage stresses was revealed, and the effects of temperature, water content, steam content, impurities, polarity of applied voltage, additives of different properties and other factors on the creeping flashover characteristics of the transformer were studied. The selection of insulation materials and structural optimization are of reference significance. Due to high voltage source requirements and high test complexity, there are very few studies on creeping flashover tests of large size (>50 mm) insulating pressboard. However, the existence of scale effect has been found, that is, the failure field strength and creepage along the surface show obvious negative power ratio. In terms of flashover mechanism, streamer theory is widely accepted, and numerical simulation methods are mature. Researchers use advanced optical photography technology to obtain high-speed photography images of streamers and conduct research on streamer initiation and development processes, discharge characteristics, average speed, etc. conducted in-depth research.
However, there are still some limitations in the current research on creeping flashover of oil-paper insulation structures, and the following research work still needs to be further carried out:
1. Thanks to the optimized design of the insulation structure, the extremely uneven electric field area inside the transformer has been effectively suppressed. The test was carried out using needle-plate and column-plate electrodes and a small size model with a creepage distance within 50 mm. The assessment results were local breakdown under extremely uneven fields, which was inconsistent with the electromagnetic environment inside the real transformer. Therefore, it is necessary to establish an insulating pressboard creeping flashover test model that can simulate real conditions, and simulate solid insulation parts in different areas by regulating the tangential and normal components.
2. The method for creeping flashover of insulating pressboard with a creepage distance greater than 50 mm is not yet mature. Therefore, it is difficult to obtain the scale effect. The only “Weidmann curve” is a curve without partial discharge stress and cannot be directly converted into a flashover voltage. It can be seen that the scale effect of creeping flashover of insulating pressboard is not clearly revealed. Therefore, it is necessary to build an insulating pressboard creeping flashover test platform with a wide-range adjustable creepage distance, and design a gradient creepage distance test to clarify the scale effect.
3. Due to the limitations of optical photography technology, capturing clear frontal images of streamer propagation is challenging, which restricts the study of the initiation and development process of surface discharges. Additionally, there is limited research on surface streamer discharge characteristics in slightly non-uniform fields or uniform fields, making it difficult to confirm their conformity to streamer theory. Therefore, it is necessary to overcome the challenge of capturing high voltage, large creeping distance surface flashover streamer discharges in insulating paperboard and to reveal the mechanisms of surface streamer discharge in uniform fields.
4. The existing standards only specify testing methods for dielectric strength of insulating pressboard, and there are no testing methods for creeping flashover. Large-scale insulating pressboards lack effective means for assessment, evaluation, and selection. Therefore, there is an urgent need to establish assessment standards under laboratory conditions, simulate the operating conditions of insulation materials inside UHV equipment, study the creeping flashover characteristics and mechanism of large-size insulation materials under relatively uniform field strengths, and comprehensively enhance the reliability of power equipment.
Author contributions
YB: Conceptualization, Investigation, Methodology, Writing–original draft. FW: Funding acquisition, Supervision, Writing–original draft. KL: Investigation, Project administration, Writing–review and editing. BT: Investigation, Supervision, Writing–original draft.
Funding
The author(s) declare financial support was received for the research, authorship, and/or publication of this article. This work was supported by the Science and Technology Project of State Grid Corporation of China (Grant No. 5108-202218280A-2-356-XG). The funder was not involved in the study design, collection, analysis, interpretation of data, the writing of this article, or the decision to submit it for publication.
Acknowledgments
Thanks for the strong guidance given by BT in the writing of the paper, the help of FW and KL, and the financial support of Professor State Grid Corporation of China.
Conflict of interest
Authors YB, FW, and KL were employed by State Grid Gansu Electric Power Company. Author FW was employed by State Grid Gansu Electric Power Company.
The remaining author declares that the research was conducted in the absence of any commercial or financial relationships that could be construed as a potential conflict of interest.
Publisher’s note
All claims expressed in this article are solely those of the authors and do not necessarily represent those of their affiliated organizations, or those of the publisher, the editors and the reviewers. Any product that may be evaluated in this article, or claim that may be made by its manufacturer, is not guaranteed or endorsed by the publisher.
References
Anderson, J. G., and Liao, T. W. (1955). The propasation mechanism of impulse creepage discharges over oil-immersed surfaces. Trans. Am. Inst. Electr. Eng. Part I Commun. Electron. 74, 218–226. doi:10.1109/TCE.1955.6372276
Ariza, D., Becerra, M., Methling, R., Gortschakow, S., Hollertz, R., and Wågberg, L. (2017). “Influence of paper properties on streamers creeping in mineral oil,” in 2017 IEEE 19th International Conference on Dielectric Liquids (ICDL), Manchester, UK, 25-29 June 2017 (IEEE), 1–7. doi:10.1109/ICDL.2017.8124659
Ariza, D., Hollertz, R., Methling, R., and Gortschakow, S. (2020). Positive streamers: inception and propagation along mineral-oil/solid interfaces. J. Phys. Commun. 4, 025008. doi:10.1088/2399-6528/ab7358
Beroual, A., and Fleszynski, J. (2003). “Influence of electrodes coating on the initiation and development of electroconvection in transformer oils,” in 2003 Annual Report Conference on Electrical Insulation and Dielectric Phenomena, Albuquerque, NM, USA, 19-22 October 2003 (IEEE), 686–689. doi:10.1109/CEIDP.2003.1254947
Beroual, A., and Fleszynski, J. (2006). “Influence of the electrodes coating with thin layers on the dielectric strength of transformer oils,” in Conference Record of the 2006 IEEE International Symposium on Electrical Insulation, Toronto, ON, Canada, 11-14 June 2006 (IEEE), 210–213. doi:10.1109/ELINSL.2006.1665294
Chen, Q., Yang, H., Wang, X., Liu, H., Zhou, K., and Ning, X. (2019). Dielectric properties of epoxy resin impregnated nano-SiO2 modified insulating paper. Polymers 11, 393. doi:10.3390/polym11030393
Cho, S. J., Lee, Y. J., Kim, Y. H., and Koo, J. Y. (2014). DC field distribution in HVDC transformer considering the effects of space charge and temperature due to presence of oil immersed pressboard. IEEE Trans. Dielectr. Electr. Insulation 21, 866–872. doi:10.1109/TDEI.2013.003957
Coltman, J. W. (2002). The transformer [historical overview]. IEEE Ind. Appl. Mag. 8, 8–15. doi:10.1109/2943.974352
Dai, J., Wang, Z. D., and Jarman, P. (2010). Creepage discharge on insulation barriers in aged power transformers. IEEE Trans. Dielectr. Electr. Insulation 17, 1327–1335. doi:10.1109/TDEI.2010.5539705
Elborki, M. A., Crossley, P. A., Wang, Z. D., Darwin, A., and Edwards, G. (2002). Detection and characterisation of partial discharges in transformer defect models. IEEE Power Eng. Soc. Summer Meet. 1, 405–410. doi:10.1109/PESS.2002.1043266
Fleszyński, J., Lutyński, B., and Zelek, A. (1982). Effect of paper electrode coatings on the electric strength of transformer oil. J. Electrost. 12, 629–635. doi:10.1016/0304-3886(82)90131-0
Ge, Y., Lv, Y., Han, Q., Sun, Q., Huang, M., Li, C., et al. (2018). Effects of TiO2 nanoparticles on streamer propagation at the surface of oil-impregnated insulation paper. IEEE Trans. Plasma Sci. 46, 2491–2496. doi:10.1109/TPS.2018.2838068
Giese, K. (1996). Electrical strength of pressboard components for transformer insulations. IEEE Electr. Insul. Mag. 12, 29–33. doi:10.1109/57.484106
Hao, J., Ye, W., Li, H., Zhang, H., Zhang, J., and Liao, R. (2023). Lightning impulse breakdown improvement properties of cellulose insulation pressboard impregnated with three-element mixed insulation oil. IEEE Trans. Dielectr. Electr. Insulation 2023, 1. doi:10.1109/TDEI.2023.3324284
He, D., Gong, W., Xu, Z., Geng, P., Liu, H., and Li, Q. (2022). Effect of the DC electric field polarity and amplitude on partial discharge of oil–paper insulated under wedge-shaped electrodes. IEEE Trans. Dielectr. Electr. Insulation 29, 599–606. doi:10.1109/TDEI.2022.3157887
He, J. (2020). Study on streamer characteristics of partial discharge of vegetable oil paper insulation under power frequency voltage.
Hekmati, A. (2017). Calculation of creepage discharge safety factors against the tangential component of electric fields in the insulation structure of power transformers. Turkish J. Electr. Eng. Comput. Sci. 25, 3487–3500. doi:10.3906/elk-1604-240
Hu, G., Wu, G., Yu, R., Zhou, P., Gao, B., Yang, Y., et al. (2019). The influence of pressure on the discharge along oil-paper interface under AC stress. Energies 12, 1846. doi:10.3390/en12101846
Huang, L. (2013). Creeping discharge under fast pulse voltage. Beijing: Intellectual Property Publishing House.
Hussain, M. R., Refaat, S. S., and Abu-Rub, H. (2021). Overview and partial discharge analysis of power transformers: a literature review. IEEE Access 9, 64587–64605. doi:10.1109/ACCESS.2021.3075288
Hwang, J.-W. G. (2010). Elucidating the mechanisms behind pre-breakdown phenomena in transformer oil systems. Available at: https://dspace.mit.edu/handle/1721.1/60145 (Accessed November 10, 2022).
Kattan, R., Denat, A., and Lesaint, O. (1989). Generation, growth, and collapse of vapor bubbles in hydrocarbon liquids under a high divergent electric field. J. Appl. Phys. 66, 4062–4066. doi:10.1063/1.343990
Krasucki, Z., and Bowden, F. P. (1966). Breakdown of liquid dielectrics. Proc. R. Soc. Lond. Ser. A. Math. Phys. Sci. 294, 393–404. doi:10.1098/rspa.1966.0214
Krause, C. (2012). Power transformer insulation – history, technology and design. IEEE Trans. Dielectr. Electr. Insulation 19, 1941–1947. doi:10.1109/TDEI.2012.6396951
Lei, E. (2019). Study on the influence of TiO_2 nanoparticles on surface flashover of oil-paper insulation system. doi:10.27140/d.cnki.ghbbu.2019.000299
Lesaint, O., Gournay, P., and Tobazeon, R. (1991). Investigations on transient currents associated with streamer propagation in dielectric liquids. IEEE Trans. Electr. Insulation 26, 699–707. doi:10.1109/14.83692
Li, J., Si, W., Yao, X., and Li, Y. (2009). Partial discharge characteristics over differently aged oil/pressboard interfaces. IEEE Trans. Dielectr. Electr. Insulation 16, 1640–1647. doi:10.1109/TDEI.2009.5361584
Li, S., Liu, Z., and Ji, S. (2021). Characteristics of creeping discharge caused by a needle electrode in oil-pressboard insulation under +DC voltage. IEEE Trans. Dielectr. Electr. Insulation 28, 215–222. doi:10.1109/TDEI.2020.009157
Li, X. (2018). Research on partial discharge characteristics and electrical aging failure model of oil-paper insulation along the surface under combined AC and DC voltages.
Li, X., Li, J., Jiang, T., Wang, Y., and Huang, Z. (2018). Analysis of creeping discharges on oil-impregnated pressboard under combined AC and DC voltages. IEEE Trans. Dielectr. Electr. Insulation 25, 2380–2388. doi:10.1109/TDEI.2018.007233
Lin, L., Meng, X., Mei, H., and Wang, L. (2023). Influence of AC and DC composite voltage on positive streamer discharge. IEEE Trans. Dielectr. Electr. Insulation 7, 1. doi:10.1109/TDEI.2023.3325421
Liu, Q., and Wang, Z. D. (2011). Streamer characteristic and breakdown in synthetic and natural ester transformer liquids with pressboard interface under lightning impulse voltage. IEEE Trans. Dielectr. Electr. Insulation 18, 1908–1917. doi:10.1109/TDEI.2011.6118629
Lundgaard, L., Linhjell, D., Berg, G., and Sigmond, S. (1998). Propagation of positive and negative streamers in oil with and without pressboard interfaces. IEEE Trans. Dielectr. Electr. Insulation 5, 388–395. doi:10.1109/94.689428
Lv, Y. Z., Zhou, Y., Li, C. R., Ma, K. B., Wang, Q., Wang, W., et al. (2014). Nanoparticle effects on creeping flashover characteristics of oil/pressboard interface. IEEE Trans. Dielectr. Electr. Insulation 21, 556–562. doi:10.1109/TDEI.2013.004151
Meng, X., Chen, W., Mei, H., and Wang, L. (2023a). Corrosion mechanism of UHV transmission line tower foot in southern China. IEEE Trans. Power Deliv. 2023, 1–10. doi:10.1109/TPWRD.2023.3329140
Meng, X., Mei, H., Yin, F., and Wang, L. (2023b). The development of the streamer discharge to flashover along the dielectric surfaces. IEEE Trans. Dielectr. Electr. Insulation 30, 1733–1742. doi:10.1109/TDEI.2023.3250615
Metwally, I. A. (2011). Failures, monitoring and new trends of power transformers. IEEE Potentials 30, 36–43. doi:10.1109/MPOT.2011.940233
Mitchinson, P. M., Lewin, P. L., Chen, G., and Jarman, P. N. (2008). “A new approach to the study of surface discharge on the oil-pressboard interface,” in 2008 IEEE International Conference on Dielectric Liquids), 1–4. doi:10.1109/ICDL.2008.4622473
Mitchinson, P. M., Lewin, P. L., Strawbridge, B. D., and Jarman, P. (2010). Tracking and surface discharge at the oil pressboard interface. IEEE Electr. Insul. Mag.) 26, 35–41. doi:10.1109/MEI.2010.5482553
Murdiya, F., Hanaoka, R., Akiyama, H., Miyagi, K., Takamoto, K., and Kano, T. (2014). Creeping discharge developing on vegetable-based oil/pressboard interface under AC voltage. IEEE Trans. Dielectr. Electr. Insulation 21, 2102–2110. doi:10.1109/TDEI.2014.004569
Muthanna, K. T., Sarkar, A., Das, K., and Waldner, K. (2006). Transformer insulation life assessment. IEEE Trans. Power Deliv. 21, 150–156. doi:10.1109/TPWRD.2005.855474
Nelson, J. K. (1989). An assessment of the physical basis for the application of design criteria for dielectric structures. IEEE Trans. Electr. Insulation 24, 835–847. doi:10.1109/14.42161
Okubo, H., Okamura, K., Ikeda, M., and Yanabu, S. (1987). Creepage flashover characteristics of oil/pressboard interfaces and their scale effects. IEEE Trans. Power Deliv. 2, 126–132. doi:10.1109/TPWRD.1987.4308082
Prevost, T. A. (2009). “Dielectric properties of natural esters and their influence on transformer insulation system design and performance — an update,” in 2009 IEEE Power and Energy Society General Meeting, Calgary, AB, Canada, 26-30 July 2009 (IEEE), 1–7. doi:10.1109/PES.2009.5275167
Qi, B., Gao, C., Li, C., Zhao, L., and Sun, X. (2015). “Characteristics of interface charges on oil-pressboard insulation and their influence on flashover under polarity reversal voltage,” in 2015 IEEE Conference on Electrical Insulation and Dielectric Phenomena (CEIDP), Ann Arbor, MI, USA, 18-21 October 2015 (IEEE), 844–847. doi:10.1109/CEIDP.2015.7352093
Qi, B., Wei, Z., and Li, C. (2016). Creepage discharge of oil-pressboard Insulation in AC-DC composite field: phenomenon and characteristics. IEEE Trans. Dielectr. Electr. Insulation 23, 237–245. doi:10.1109/TDEI.2015.005404
Qin, W. (2020). Study on the differences in the effects of AC, DC and AC-DC composite voltages on flashover and partial discharge along the surface of oil-paper insulation. doi:10.27670/d.cnki.gcqdu.2020.004211
Ribeiro, C. D. J., Marques, A. P., Azevedo, C. H. B., Souza, D. C. P., Alvarenga, B. P., and Nogueira, R. G. (2009). “Faults and defects in power transformers - a case study,” in 2009 IEEE Electrical Insulation Conference, Montreal, QC, Canada, 31 May 2009 - 03 June 2009 (IEEE), 142–145. doi:10.1109/EIC.2009.5166333
Settles, G. S. (2001). Schlieren and shadowgraph techniques: visualizing phenomena in transparent media. Springer Science and Business Media.
Wang, F., Liang, K., Zhong, L., Chen, S., Sun, Q., Wang, L., et al. (2023). Influence of paper surface morphology on streamer propagation at oil-paper interface. IEEE Trans. Dielectr. Electr. Insulation 30, 2305–2314. doi:10.1109/TDEI.2023.3289475
Yea, M., Han, K. J., Park, J., Lee, S., and Choi, J. (2018). Design optimization for the insulation of HVDC converter transformers under composite electric stresses. IEEE Trans. Dielectr. Electr. Insulation 25, 253–262. doi:10.1109/TDEI.2018.006629
Zainuddin, H., Mitchinson, P. M., and Lewin, P. L. (2011). “Investigation on the surface discharge phenomenon at the oil-pressboard interface,” in 2011 IEEE International Conference on Dielectric Liquids, Trondheim, Norway, 26-30 June 2011 (IEEE), 1–4. doi:10.1109/ICDL.2011.6015439
Zhang, L., Liu, R., and Huang, M. (2021). “Simulation of the effect of dielectric match on surface flashover along oil-paper insulation,” in 2021 International Conference on Advanced Electrical Equipment and Reliable Operation (AEERO), Beijing, China, 15-17 October 2021 (IEEE), 1–4. doi:10.1109/AEERO52475.2021.9708213
Zhang, S., Yu, X., Wu, C., Yang, L., Cheng, H., and Deng, J. (2022a). Study on surface discharge characteristics and design method of large size oil-paper insulation of electrical transformer. J. Phys. Conf. Ser. 2355, 012065. doi:10.1088/1742-6596/2355/1/012065
Zhang, Y., Wang, G., Zhang, X., Zhong, W., Su, Z., Xu, C., et al. (2022b). Study on space charge characteristics of transformer insulating paper under different working conditions. IEEE Trans. Plasma Sci. 50, 731–739. doi:10.1109/TPS.2022.3147506
Zhao, L. (2017). Partial discharge and streamer characteristics of transformer liquids under AC stress. United Kingdom: The University of Manchester.
Zhou, Y., Jin, F., Sun, Q., Sha, Y., and Huang, M. (2013). “Surface flashover characteristics of oil-paper insulation under combined AC-DC voltage,” in 2013 IEEE International Conference on Solid Dielectrics (ICSD), Bologna, Italy, 30 June 2013 - 04 July 2013 (IEEE), 1005–1008. doi:10.1109/ICSD.2013.6619696
Keywords: oil-paper insulation structure, insulation pressboard, creeping flashover, scale effect, streamer theory
Citation: Bao Y, Wang F, Liu K and Tao B (2024) Creeping flashover test methods, characteristics, and mechanisms of oil-paper insulation structures of power transformers. Front. Mater. 10:1345676. doi: 10.3389/fmats.2023.1345676
Received: 28 November 2023; Accepted: 27 December 2023;
Published: 16 January 2024.
Edited by:
Xiaobo Meng, Guangzhou University, ChinaReviewed by:
Yong Liu, Tianjin University, ChinaZhong Wang, Sichuan University, China
Hongwei Mei, Tsinghua University, China
Copyright © 2024 Bao, Wang, Liu and Tao. This is an open-access article distributed under the terms of the Creative Commons Attribution License (CC BY). The use, distribution or reproduction in other forums is permitted, provided the original author(s) and the copyright owner(s) are credited and that the original publication in this journal is cited, in accordance with accepted academic practice. No use, distribution or reproduction is permitted which does not comply with these terms.
*Correspondence: Yanyan Bao, MTA3NjQ2MzYyMUBxcS5jb20=