- 1Chengdu University of Traditional Chinese Medicine, Chengdu, China
- 2State Key Laboratory of Kidney Diseases, Beijing Key Laboratory of Medical Devices and Integrated Traditional Chinese and Western Drug Development for Severe Kidney Diseases, Beijing Key Laboratory of Digital Intelligent TCM for the Preventionand Treatment of Pan-vascular Diseases, Key Disciplines of National Administration of Traditional Chinese Medicine(zyyzdxk-2023310), Department of Nephrology, First Medical Center of Chinese PLA General Hospital, National Clinical Research Center for Kidney Diseases, Beijing, China
- 3School of Clinical Medicine, Guangdong Pharmaceutical University, Guangzhou, China
- 4Henan University of Traditional Chinese Medicine, Zhengzhou, China
Objective: This study aimed to investigate the effect of dietary acid-base load on patients with chronic kidney disease (CKD) and type 2 diabetes mellitus (T2DM).
Design: A total of 300 patients with CKD were enrolled and divided into three groups according to DAL tertiles. Dietary intake was assessed using a 24-h dietary recall, and diet-based acidity was assessed using net endogenous acid production (NEAP), potential renal acid load (PRAL), and dietary acid load (DAL). Multivariable logistic regression models were used to determine the association between diet-based acid load scores and CKD and T2DM.
Results: Within the food category groupings, the DAL score was positively associated with poultry and eggs and negatively associated with fruits and vegetables. Regarding energy and macronutrients, the DAL score was positively correlated with the intake of protein, animal protein, monounsaturated fatty acids, saturated fatty acids, and fat, while it was negatively correlated with the intake of carbohydrates, plant protein, and dietary fiber. In terms of micronutrients, DAL scores were positively associated with phosphorus intake and negatively associated with potassium, magnesium, and copper intake. After adjusting for age, BMI, energy, and eGFR, and stratifying by sex, logistic regression analysis showed that DAL level (OR = 6.47, 95% CI 1.19–35.18, p = 0.031) was a related factor for CKD and T2DM in females.
Conclusion: DAL score is a related factor for patients with T2DM and CKD.
1 Introduction
Chronic kidney disease (CKD) is a significant global public health concern due to its high morbidity, poor prognosis, and high mortality. In 2024, there were approximately 850 million patients with CKD worldwide, representing a global prevalence of about 9.5% (1). The prevalence of CKD in China is 8.2% (2). CKD is characterized by structural and functional changes in the kidneys lasting more than 3 months (3). Clinically, it involves multiple systems, including the respiratory, circulatory, skeletal, and endocrine systems, and eventually progresses to end-stage renal disease (ESRD). Diabetes and hypertension are the leading contributors to the increased CKD burden. The rising prevalence of diabetes has led to a steady increase in the prevalence of CKD combined with type 2 diabetes mellitus (T2DM). Patients with both CKD and T2DM are at greater risk compared to patients with CKD alone. Early intervention can delay CKD progression and renal failure. Additionally, it has been suggested that diet is an important etiologic and prognostic factor of CKD.
The acid–base status of the body can be influenced by dietary intake, depending on the balance between the intake of acid and alkaline-inducing foods. Diet significantly affects the risk of CKD progression (4). Dietary modifications that reduce acid load may improve prognosis in CKD and T2DM.Potential renal acid load (PRAL), net endogenous acid production (NEAP), and dietary acid load DAL are common metrics used to assess dietary acid–base load (5). Higher PRAL and NEAP scores indicate a greater acidogenic potential of the food. A diet with a high acid load can lead to low-grade metabolic acidosis, which can result in insulin resistance (6), T2DM (7) and metabolic syndrome (7). Furthermore, there are sex-related differences in the prevalence of T2DM (8). Taking sex-related differences into account in the recognition, development, presentation, diagnosis, treatment, and prevention of T2DM can facilitate the development of more personalized diabetes care strategies in the future.
This study aimed to evaluate the association between dietary acid–base load in patients with CKD and T2DM using PRAL, NEAP, and DAL scores, taking into account sex-related differences.
2 Materials and methods
2.1 Study population
This cross-sectional study, conducted between March 2022 and October 2023, involved adult patients with CKD hospitalized at the Department of Nephrology of the First Medical Center of the Chinese People’s Liberation Army General Hospital. The inclusion criteria comprised: (1) diagnosis of CKD according to the 2024 KDIGO Clinical Practice Guidelines (3), (2) age ≥ 18 years. The exclusion criteria were as follows: (1) history of severe infection within the past month; (2) acute and severe diseases in the past 6 months; (3) malignant tumors; (4) pregnancy or lactation; (5) incomplete medical history or clinical examination results; and (6) incomplete dietary intake data or over-reporting (> 4,000 kcal) and under-reporting of energy intake (< 600 kcal). Ultimately, 300 non-dialysis patients with CKD were included in the analysis.
2.2 Dietary intake
Dietary intake was assessed through face-to-face interviews using 24-h dietary recall within 48 h of patient admission to the hospital. During the 24-h dietary review, the researchers directly queried the patients about their food consumption on the preceding day, detailing the variety and quantities of food aided by food pictures or models. Nutrient intake was calculated according to the Chinese Dietary Guidelines (2022 edition) (9) and Chinese Dietary Reference Intakes (2013 edition) (10). Adjustment for food and nutrient intake was performed using the residual energy method (11).
2.3 Dietary acid–base load
Common indicators of dietary acid–base load include NEAP, PRAL, and DAL. NEAP and PRAL were calculated based on the dietary intake of proteins and minerals. DAL was calculated using dietary protein, phosphorus, potassium, calcium, magnesium, height, and weight.
PRAL (mmol/d) = 0.49 × protein (g/day) + 0.037 × phosphorus (mg/day) − 0.021 × potassium (mg/day) − 0.026 × magnesium (mg/day) − 0.013 × calcium (mg/day) (12);
NEAP (mEq/d) = 54.5 × [protein(g/day)/potassium intake (mEq/day)] − 10.2 (13);
DAL (mmol/d) = PRAL + (body surface area [m2] × 41[mEq/d]/1.73 m2) (14);
The body surface area was calculated as follows = 0.007184 × height (cm) ^ 0.725 × weight (kg)^ 0.425 (15, 16).
2.4 Clinical data collection
General information collected included name, sex, height, weight, age, and medical history, including present illness, nephropathy, hypertension, and diabetes. Clinical laboratory parameters measured included white blood cell count (WBC), hemoglobin, total protein, albumin, haptoglobin, prealbumin, urea nitrogen, serum creatinine, estimated glomerular filtration rate (eGFR), serum cystatin C, 24-h urinary protein, serum uric acid, total cholesterol, triglycerides, fasting blood glucose (FBG), serum calcium, potassium, phosphorus, high-density lipoprotein cholesterol (HDL-C) and low-density lipoprotein cholesterol (LDL-C).
2.5 Statistical analysis
Measurement data with a normal distribution were presented as mean ± standard deviation, while non-normally distributed data were expressed as medians with interquartile ranges. Differences between different DAL tertiles were compared, and measurement data with normal distribution and homogeneity of variance were compared between groups using a one-way analysis of variance test. A non-parametric test was used for comparison between groups if the homogeneity of variance was not satisfied. Count variables were expressed as frequencies and percentages and analyzed using chi-square or Fisher’s exact tests. Logistic regression analysis was used to analyze the relationship between the DAL score and CKD combined with T2DM after controlling for the confounding effects of age, body mass index (BMI), energy intake, and eGFR. Statistical analyses were performed using SPSS version 26.0 for Mac software (SPSS Inc., Chicago, IL, United States). Statistical significance was set at p (or P-trend) < 0.05.
3 Results
3.1 Screening of the selected cases
A total of 321 patients with CKD hospitalized at the Department of Nephrology of the First Medical Center of the Chinese People’s Liberation Army General Hospital between March 2022 and October 2023 were initially selected. After excluding seven patients due to incomplete dietary information, 13 patients with extreme energy intake (< 600 kcal/day or > 4,000 kcal/day), and one patient undergoing dialysis, 300 participants remained eligible for inclusion. Figure 1 provides a visual representation of the participant screening process.
3.2 General information on the study population
This study enrolled a total of 300 patients with CKD; approximately 63.7% were male. PRAL, NEAP, DAL, and BMI were significantly different in DAL tertiles, with an increasing trend (p < 0.001). Out of the total, 148 (49.3%) had T2DM, and significant differences appeared primarily between Q3 and Q1/Q2. No significant differences were observed in age, CKD course, or hypertension between the different DAL categories. Table 1 presents the characteristics of the 300 patients. Patients with T2DM were analyzed separately, Supplementary Table 1 shows baseline characteristics of patients combine CKD with T2DM by categories of DAL median.
3.3 Comparison of clinical characteristics of study participants
Blood urea nitrogen (BUN), cystatin C, uric acid, and FBG levels were significantly different in the DAL tertiles, with an increasing trend (p < 0.05). The participants assigned to the highest DAL category had significantly higher Serum creatinine levels, and significant differences appeared primarily between Q3 and Q1/Q2 (p < 0.05). The participants assigned to the highest DAL category had significantly lower HDL-C levels. In HDL-C levels, significant differences were observed particularly between the highest tertile and lower tertiles (p < 0.05).There were no significant differences in WBC count, hemoglobin, total protein, haptoglobin, prealbumin, eGFR, 24-h urinary protein, total cholesterol, triglyceride, serum calcium, serum potassium, serum phosphorus, or LDL-C levels among the different DAL categories. Table 2 presents a comparison of the clinical characteristics of the participants based on the DAL category.
3.4 Distribution of dietary intake in the study population
Among the food groups, DAL was positively associated with meat and eggs (p < 0.001) but negatively associated with fruits and vegetables (p < 0.001). Regarding energy and macronutrients, DAL was positively associated with protein, animal protein, monounsaturated fatty acids (MUFA), saturated fatty acids, and fat intake (p < 0.05) while displaying a negative association with carbohydrate, plant protein, and fiber intake (p < 0.001). No significant association was found in polyunsaturated fatty acid (PUFA) intakes across the DAL tertiles.
Concerning dietary micronutrient intake, DAL was negatively associated with sodium, potassium, calcium, magnesium, and iron while being positively associated with phosphorus and zinc intake. Specifically, DAL was negatively associated with potassium, magnesium, and copper intakes (p < 0.05), but it was positively associated with phosphorus intake (p < 0.05). Table 3 presents the dietary intakes of the participants in the DAL tertiles.
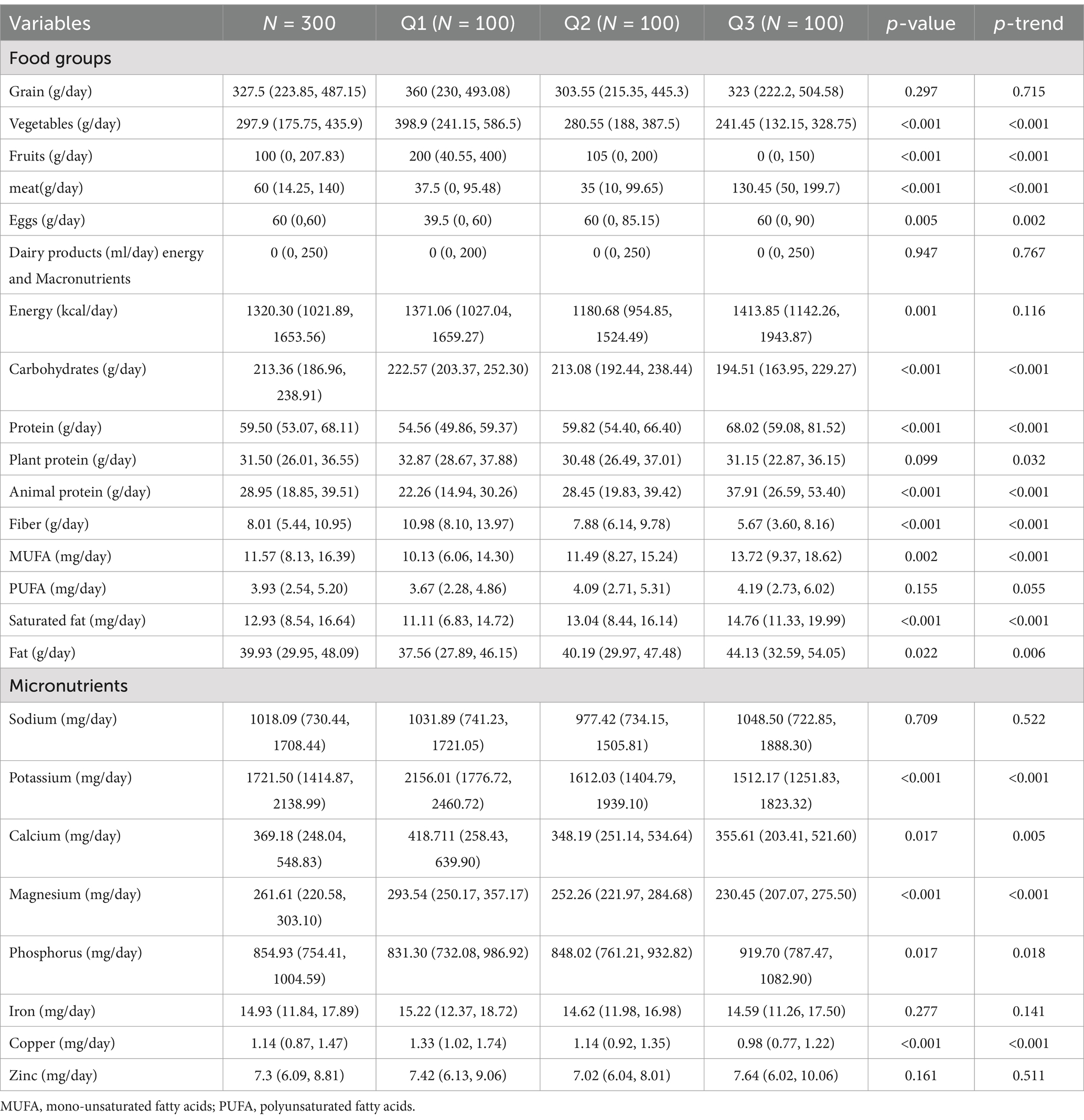
Table 3. Food groups, macronutrient, and micronutrient intake of study participants by categories of DAL.
3.5 Logistic regression analysis was used to analyze the relationship between dietary acid–base load and CKD combined with T2DM
Regarding the association between dietary acid–base load and CKD combined with T2DM, odds ratios (ORs) and 95% confidence intervals (CI) for the association between dietary acid and base are shown in Table 4. In the crude model, the DAL score was significantly associated with higher odds in CKD combined with T2DM. Specifically, the risk of CKD combined with T2DM in the highest tertile of DAL was 91% greater than that in the lowest tertile (OR = 1.91, 95%CI 1.09–3.36, p = 0.024). After adjusting for age, BMI, the DAL score was significantly associated with higher odds of patients with CKD and T2DM. The risk of CKD combined with T2DM in the highest tertile of DAL was 2.07 times greater than that in the lowest tertile (OR = 2.07, 95%CI 1.06–4.03, p = 0.033). Similarly, In Model 2, the odds of CKD combined with T2DM in the highest DAL tertile were 2.06 times greater than those in the lowest tertile (OR = 2.06, 95%CI 1.05–4.01, p = 0.035). Subsequently, in Model 3, when further adjusted for eGFR, no significant association was observed between DAL in CKD combined with T2DM. No significant association was observed between PRAL or NEAP levels in CKD combined with T2DM.
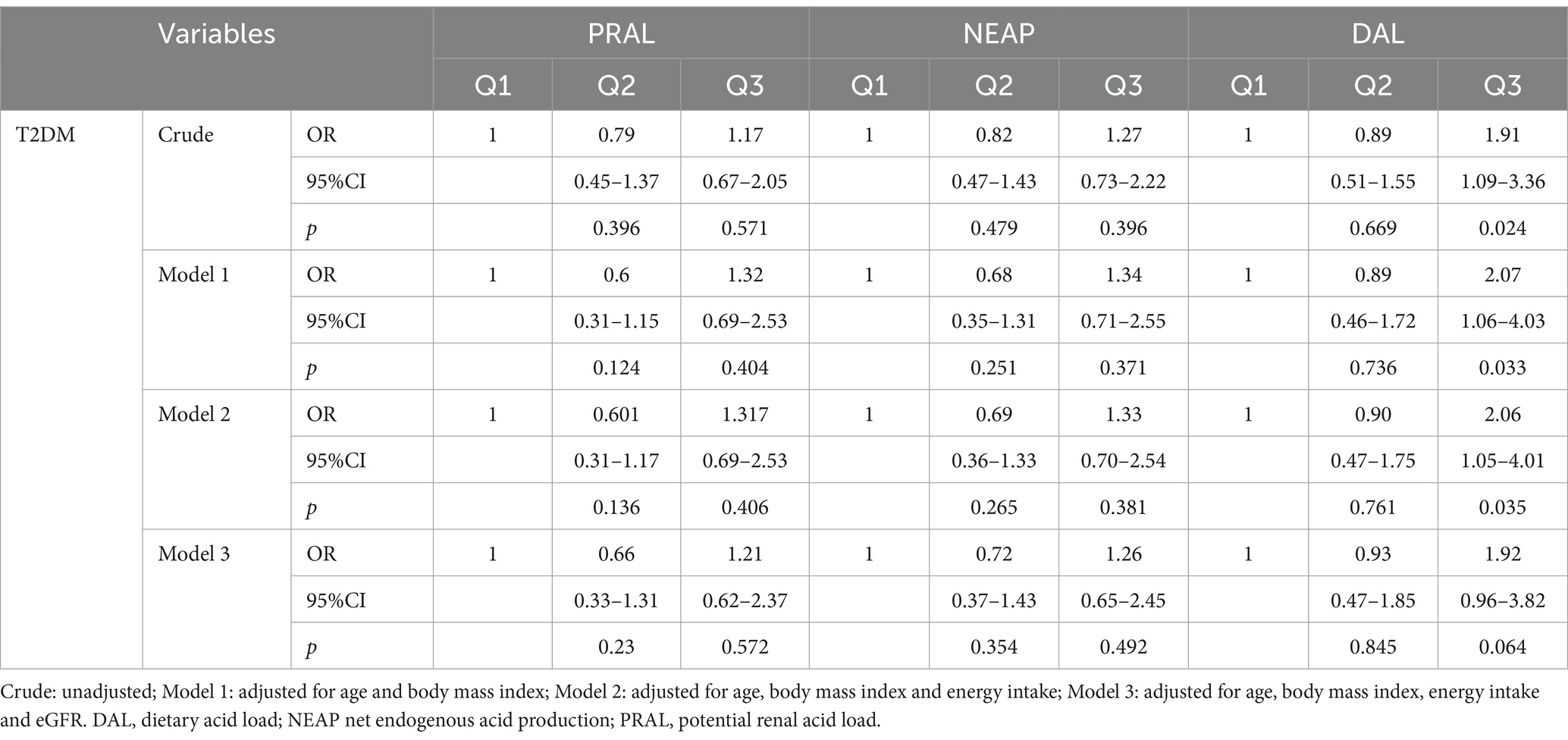
Table 4. Logistic regression analysis models for the association between CKD combined with T2DM and tertiles of PRAL, NEAP, and DAL.
3.6 Logistic regression analysis models for the association between CKD combined with T2DM and tertiles of PRAL, NEAP, and DAL categorized by sex
The results of crude and multivariable adjusted ORs and 95% CI for the association between CKD combined with T2DM and tertiles of PRAL, NEAP, and DAL scores stratified by sex are presented in Table 5. In males, no significant association was observed between any indices of dietary acid–base load and CKD combined with T2DM. However, in females, higher DAL scores were significantly associated with higher odds of CKD combined with T2DM. In Models 1, 2, and 3, the risk of CKD combined with T2DM in the highest DAL tertile was 6.61, 6.57, and 6.47 times higher, respectively, than that in the lowest DAL tertile. 1. No significant modifications were observed between PRAL, NEAP, and DAL and CKD combined with T2DM (p > 0.05; Supplementary Table 2).
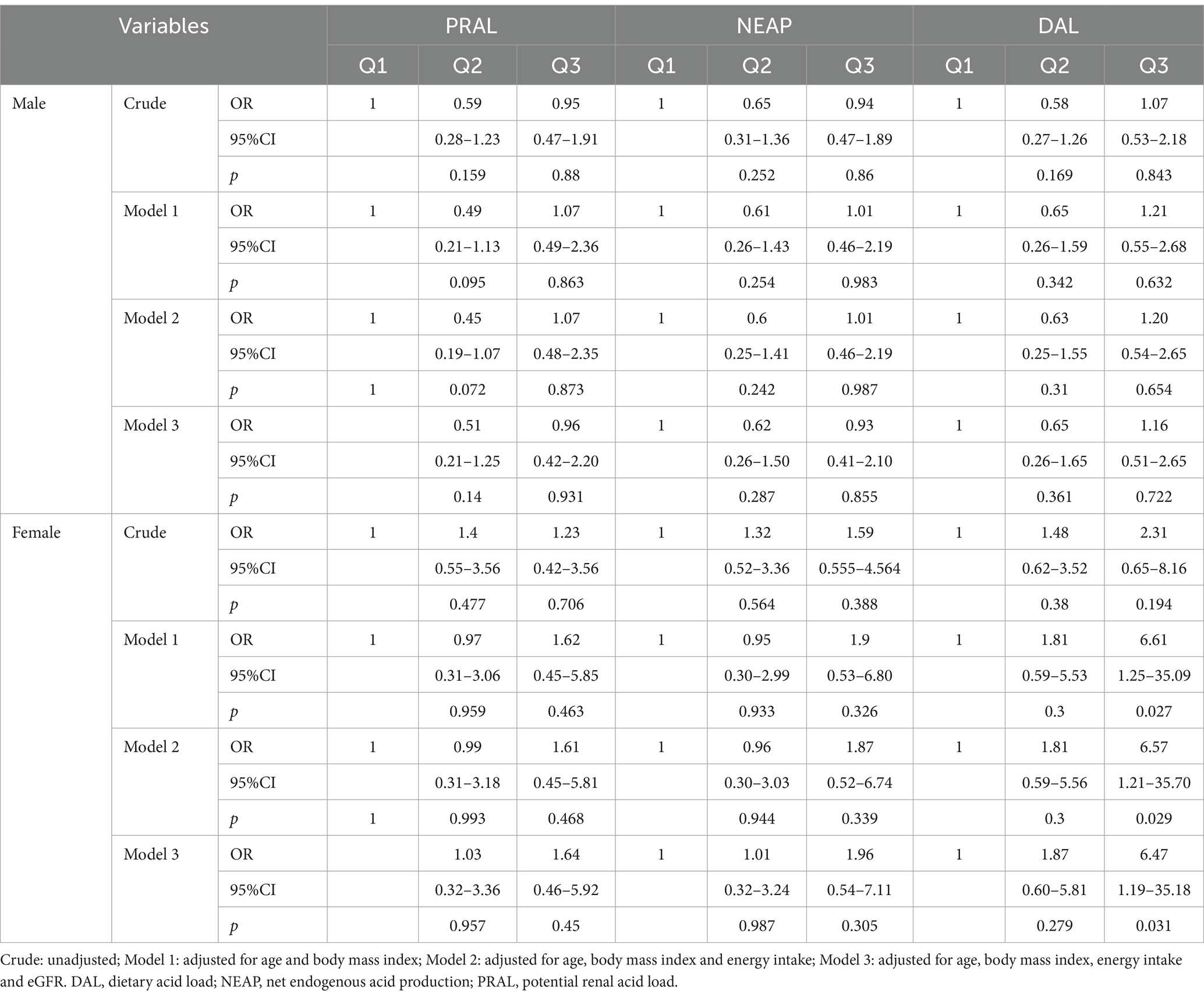
Table 5. Logistic regression analysis models for the association between CKD combined with T2DM and tertiles of PRAL, NEAP, and DAL categorized by sex.
4 Discussion
4.1 Association between dietary acid–base load and CKD with T2DM
Metabolic acidosis occurs when the kidneys do not fully excrete the acid load generated by metabolism. Metabolic acidosis is a common complication in patients with CKD. The potentially deleterious effects of metabolic acidosis in CKD include muscle wasting, bone demineralization, hyperkalemia, and accelerated disease progression (17). One study showed that metabolic acidosis caused proteinuria and decreased eGFR, associated with the intrarenal renin-angiotensin system activation (18). Elevated dietary acid intake can also provoke tubular toxicity, triggering the complement pathway and boosting renal medullary ammonia levels (19). Diet plays a crucial role in generating nonvolatile acid loads. In an elderly Spanish population study targeting metabolic syndrome, higher PRAL and NEAP scores were associated with decreased eGFR and increased urine albumin creatine ratio (UACR) after 1 year of follow-up (20). A meta-analysis also confirmed the association between dietary acid–base load and renal function, with a high dietary acid load leading to decreased renal function (21). After adjusting for multiple confounders, a higher dietary acid load was associated with a higher prevalence of CKD or impaired renal function, as indicated by low eGFR (22–24). A large body of evidence links dietary acid load to renal outcomes.
Recent research has focused on the relationship between diet-induced acid–base load, T2DM, and insulin resistance (6, 25–29). A pooled study of three prospective cohorts showed that a higher diet-dependent acid load (including NEAP, PRAL, and animal protein-to-potassium ratio) was associated with an increased risk of T2DM (25). Similarly, a prospective study in a middle-aged and elderly Korean population found that a higher PRAL score was associated with an increased risk of future insulin resistance (6). Additionally, a meta-analysis of 14 studies demonstrated that participants with the highest PRAL and NEAP scores had a 19 and 22% increased risk of diabetes mellitus (DM), respectively, compared to those in the lowest category (30). Similarly, the DAL score was positively associated with CKD and T2DM in our study. The mechanisms by which DAL specifically affects the progression of T2DM pathophysiology may be as follows. Firstly, high DAL results in low interstitial fluid PH. Insulin receptors are found on the plasma membrane, particularly in insulin-targeted cells. When insulin hooks onto its receptor, it does so on the side that faces the surrounding interstitial fluid. Therefore, alterations in the microenvironments of interstitial fluids, particularly pH levels, influence the insulin’s binding strength with its receptor (31, 32). Secondly, High DAL enhance adrenal cortex cortisol production (33). Chronically elevated cortisol levels can lead to insulin resistance (34). DAL modification could be a feasible intervention target. The Mediterranean diet is mainly a plant-based diet and belongs to a low diet-based acidity. The Mediterranean diet is recommended by the National Kidney Foundation’s Kidney Disease Outcomes Quality Initiative (35). The study has shown that the Mediterranean diet can reduce FBG, glycated hemoglobin and LDL-C (36). There is evidence that adhering to a Mediterranean diet lowers the likelihood of developing T2DM (37, 38).
4.2 Association between dietary factors and risks of CKD and T2DM
In our study, the food groups in the highest tertile of DAL scores were characterized by low consumption of vegetables and fruits but high consumption of meat and eggs. Food groups with high DAL scores are characterized by low consumption of vegetables and fruits and high consumption of dairy products, cereals, and eggs (39). Vegetables and fruits have excellent antioxidant properties that help eliminate free radicals and mitigate oxidative stress, thus protecting cells and structures from oxidative damage (40). A high intake of fruits and vegetables has long been associated with health benefits such as protecting against cancer, diabetes, neurodegenerative diseases, and cardiovascular diseases (41, 42). On the other hand, increased meat intake, particularly synthetic and processed meat, has been positively associated with proinflammatory substances (43). Animal proteins, rich in sulfur-containing amino acids such as methionine, homocysteine, and cysteine, contribute to the production of sulfate with an acidification effect during the metabolic process. This can lead to metabolic acidosis (44, 45).
In this study, in terms of energy and macronutrients, patients with CKD and T2DM in the highest DAL tertiles consumed more protein, including animal protein, as well as MUFA, saturated fatty acids, fat, and phosphorus. Conversely, their intake of carbohydrates, plant protein, dietary fiber, potassium, magnesium, and copper was lower. Some studies suggest that total and animal proteins can increase the risk of T2DM, while plant proteins can reduce the risk of T2DM (46, 47). Hence, it is essential to consider both the type and food source of dietary proteins for the prevention of diabetes.
Several studies have provided evidence supporting an association between dietary fatty acids, especially saturated fatty acids, and the development of insulin resistance and T2DM. However, recent cohort studies have shown no association between saturated fatty acids and the incidence of T2DM (48, 49). Conversely, several prospective studies have demonstrated an inverse association between total MUFA intake and the risk of T2DM (50, 51). There may be the following reasons the negative association between DAL and MUFA in this study. Firstly, Traditional Chinese cooking, such as stir frying, pan frying, and deep frying, can lead to isomerization of unsaturated fatty acids (UFA), which in turn produces harmful substances such as trans fatty acids (52, 53). Secondly, 24-h dietary review do not represent long-term dietary intake habits. Higher concentrations of marine-derived omega-3 PUFA biomarkers have been linked to greater risks of T2DM, coronary heart disease, and total mortality (54). Conversely, another study reported that higher levels of omega-3 PUFA in seafood sources were associated with a lower risk of CKD (55). Whether fatty acids are risk factors or protective factors for T2DM remains controversial, potentially influenced by differences in food sources and carbon chain lengths (48, 56).
4.3 Sex disparities in the dietary acid–base load and CKD with T2DM
Three distinct dietary acid–base load measures were employed in this study, each with its unique formula, limitations, and advantages. Consequently, utilizing these three indicators enhances the reliability of the results. In a cross-sectional study of 1945 Iranian adults, participants in the highest quartile of PRAL, NEAP, and DAL had 42, 48, and 44% higher ORs for metabolic syndrome compared to those in the lowest quartile, respectively. Subsequent analysis of the population by sex showed that higher PRAL, NEAP, and DAL scores were significantly associated with increased odds of metabolic syndrome in the male population but not in the female population (7). Notably, no previous study has investigated the association between these three dietary acid–base load measures (PRAL, NEAP, and DAL) in patients with CKD and T2DM while considering sex differences. Further analysis by sex showed that in women in Models 1, 2, and 3, the risk of CKD with T2DM in the highest DAL tertile was 6.61, 6.57, and 6.47 times higher than that in the lowest DAL tertile. However, no significant association was observed among males.
Evidence suggests that sex differences play an important role in the pathophysiology, epidemiology, treatment, and prognosis of metabolic syndrome and DM. There are sex-related differences in the prevalence of T2DM. Globally, the prevalence of DM is higher in men, but more women are affected by T2DM than men (57). The sex difference in DM prevalence is reversed according to the reproductive life stage: prepubertal males exhibit higher rates of DM, whereas postmenopausal and older women have more DM. Gestational diabetes mellitus (GDM) is the most important risk factor for the development of T2DM in women (58). Additionally, premature menopause is associated with an increased risk of T2DM (59–61). This reason is caused by hormonal differences in metabolic responses between genders. Estrogen protects against insulin resistance by activating the ERα pathway in insulin-sensitive tissues (62). Estrogen decreases in women after menopause. Low estrogen levels increase inflammation and enhances fat accumulation in the body. Excess fat produces excess triglycerides and free fatty acids, which in turn impair insulin signaling and β-cell regulation, leading to T2DM (63). Estrogen influences microbiota in the gut, the gut microbiota regulates glucose homeostasis (64).Endogenous estrogens play a protective role in preventing females from developing type 2 diabetes. Men have small fluctuations in hormone levels throughout life compared with women. Androgen is converted into estrogen through the process of aromatization. Men with an androgen/estrogen ratio imbalance are at metabolic risk.
Taking gender differences into account in the recognition, development, presentation, diagnosis, treatment, and prevention of T2DM will facilitate the development of more personalized diabetes care in the future.
4.4 Strengths and limitations
This study is the first to use three measures (PRAL, NEAP, and DAL) to assess the association between dietary acid–base load and the risk of combined CKD and T2DM while considering disparities in sex. However, we excluded all subjects with extreme values of total energy intake and adjusted the intake of food and nutrients according to the energy residual method to control for reporting bias.
Nonetheless, this study has some limitations. Firstly, the dietary data of the patients did not encompass the use of supplements. Secondly, this was a cross-sectional, single-center study, and it was not able to determine cause and effect. Longitudinal design or interventional studies are recommended to further verify causality. The diversity and representativeness of the samples may be limited. Future studies be validated in a broader population, including patients with different regions, ethnicities, and medical backgrounds. Thirdly, the 24-h dietary review was used to assess dietary intake, which may be subject to recall bias. It is recommended that future studies use more accurate dietary assessment methods (such as food diaries or multiple 24-h reviews). Fourthly, despite accounting for potential confounding elements, other potential confounders may still exist, such as physical activity levels, medication use, or socioeconomic status.
5 Conclusion
In conclusion, our study revealed a positive association between the DAL scores and CKD with T2DM, particularly among women. An alkaline - rich diet, which is abundant in fruits and vegetables and lower in excessive meat, might be a modifiable factor in reducing DAL. Additional studies are required to ascertain the specific foods’ acidogenic potential to establishing specific dietary recommendations.
Data availability statement
The original contributions presented in the study are included in the article/Supplementary material, further inquiries can be directed to the corresponding authors.
Ethics statement
The study was approved by the Ethics Committee of the Chinese People’s Liberation Army General Hospital (approval number: S2022-324-01). The studies were conducted in accordance with the local legislation and institutional requirements. The participants provided their written informed consent to participate in this study. Written informed consent was obtained from the individual(s) for the publication of any potentially identifiable images or data included in this article.
Author contributions
HH: Investigation, Software, Validation, Writing – original draft. QW: Methodology, Writing – original draft. RZ: Validation, Writing – original draft. FL: Data curation, Writing – original draft. YN: Methodology, Writing – original draft. YL: Investigation, Writing – original draft. SLi: Data curation, Writing – review & editing. TL: Conceptualization, Writing – original draft. ZT: Data curation, Writing – original draft. XW: Software, Writing – review & editing. JY: Visualization, Writing – review & editing. YW: Writing – review & editing. LZ: Writing – review & editing, Resources. SLu: Writing – review & editing, Formal analysis. WZ: Writing – review & editing, Project administration. YZ: Visualization, Writing – review & editing. WD: Visualization, Writing – original draft. GC: Writing – review & editing, Data curation. XC: Funding acquisition, Project administration, Resources, Writing – review & editing. ZD: Funding acquisition, Project administration, Resources, Supervision, Writing – review & editing.
Funding
The author(s) declare that financial support was received for the research and/or publication of this article. This research was supported by Science & Technology Project of Beijing, China [No. Z221100007422121], Capital’s Funds for Health Improvement and Research [No. CFH 2024-1-5021], Beijing Natural Science Foundation [Nos. 7252018, L222133, and L232122], and National Natural Science Foundation of China [No. 62450131].
Acknowledgments
We would like to thank the Nephrology Team of the Chinese People’s Liberation Army General Hospital for supporting our work and the subjects for the provision of the data. We would like to thank Fresenius Kabi and BYHEALTH Institute of Nutrition & Health for technical support.
Conflict of interest
The authors declare that the research was conducted in the absence of any commercial or financial relationships that could be construed as a potential conflict of interest.
Generative AI statement
The author(s) declare that no Gen AI was used in the creation of this manuscript.
Publisher’s note
All claims expressed in this article are solely those of the authors and do not necessarily represent those of their affiliated organizations, or those of the publisher, the editors and the reviewers. Any product that may be evaluated in this article, or claim that may be made by its manufacturer, is not guaranteed or endorsed by the publisher.
Supplementary material
The Supplementary material for this article can be found online at: https://www.frontiersin.org/articles/10.3389/fnut.2025.1581009/full#supplementary-material
References
1. Bello, AK, Okpechi, IG, Levin, A, Ye, F, Damster, S, Arruebo, S, et al. An update on the global disparities in kidney disease burden and care across world countries and regions. Lancet Glob Health. (2024) 12:e382–95. doi: 10.1016/s2214-109x(23)00570-3
2. Wang, L, Xu, X, Zhang, M, Hu, C, Zhang, X, Li, C, et al. Prevalence of chronic kidney disease in China: results from the sixth China chronic disease and risk factor surveillance. JAMA Intern Med. (2023) 183:298–310. doi: 10.1001/jamainternmed.2022.6817
3. Kdigo. Clinical practice guideline for the evaluation and Management of Chronic Kidney Disease. Kidney Int (2024). (2024) 105:S117–s314. doi: 10.1016/j.kint.2023.10.018
4. Wesson, DE, and Simoni, J. Acid retention during kidney failure induces endothelin and aldosterone production which Lead to progressive Gfr decline, a situation ameliorated by alkali diet. Kidney Int. (2010) 78:1128–35. doi: 10.1038/ki.2010.348
5. Han, E, Kim, G, Hong, N, Lee, YH, Kim, DW, Shin, HJ, et al. Association between dietary acid load and the risk of cardiovascular disease: Nationwide surveys (Knhanes 2008-2011). Cardiovasc Diabetol. (2016) 15:122. doi: 10.1186/s12933-016-0436-z
6. Lee, KW, and Shin, D. Positive association between dietary acid load and future insulin resistance risk: findings from the Korean genome and epidemiology study. Nutr J. (2020) 19:137. doi: 10.1186/s12937-020-00653-6
7. Seifi, N, Rahimi, H, Koochakpoor, G, Zarei, A, Assaran Darban, R, Ferns, GA, et al. Higher dietary acid load is associated with an increased risk of metabolic syndrome. Sci Rep. (2023) 13:22154. doi: 10.1038/s41598-023-48429-2
8. Kautzky-Willer, A, Leutner, M, and Harreiter, J. Sex differences in type 2 diabetes. Diabetologia. (2023) 66:986–1002. doi: 10.1007/s00125-023-05891-x
9. Society CN. Dietary guidelines for Chinese residents (2022). Beijing,China: People's Medical Publishing House (2022).
10. Society, CN. Dietary reference intakes for Chinese. 2013th ed. China: Beijing: Sciences Press (2014). 660 p.
11. Willett, WC, Howe, GR, and Kushi, LH. Adjustment for Total energy intake in epidemiologic studies. Am J Clin Nutr. (1997) 65:1220S–8S.
12. Remer, T, Dimitriou, T, and Manz, F. Dietary potential renal acid load and renal net acid excretion in healthy, free-living children and adolescents. Am J Clin Nutr. (2003) 77:1255–60. doi: 10.1093/ajcn/77.5.1255
13. Frassetto, LA, Todd, KM, Morris, RC Jr, and Sebastian, A. Estimation of net endogenous noncarbonic acid production in humans from diet potassium and protein contents. Am J Clin Nutr. (1998) 68:576–83.
14. Remer, T, and Manz, F. Estimation of the renal net acid excretion by adults consuming diets containing variable amounts of protein. Am J Clin Nutr. (1994) 59:1356–61.
15. Du Bois, D, and Du Bois, EF. A formula to estimate the approximate surface area if height and weight be known. 1916. Nutrition. (1989) 5:303–11.
16. Verbraecken, J, Van de Heyning, P, De Backer, W, and Van Gaal, L. Body surface area in Normal-weight, overweight, and obese adults. Comparison Study Metabolism. (2006) 55:515–24. doi: 10.1016/j.metabol.2005.11.004
17. Vincent-Johnson, A, and Scialla, JJ. Importance of metabolic acidosis as a health risk in chronic kidney disease. Adv Chronic Kidney Dis. (2022) 29:329–36. doi: 10.1053/j.ackd.2022.05.002
18. Ng, HY, Chen, HC, Tsai, YC, Yang, YK, and Lee, CT. Activation of intrarenal renin-angiotensin system during metabolic acidosis. Am J Nephrol. (2011) 34:55–63. doi: 10.1159/000328742
19. Nagami, GT, and Hamm, LL. Regulation of Acid-Base balance in chronic kidney disease. Adv Chronic Kidney Dis. (2017) 24:274–9. doi: 10.1053/j.ackd.2017.07.004
20. Valle-Hita, C, Becerra-Tomás, N, Díaz-López, A, Vázquez-Ruiz, Z, Megías, I, Corella, D, et al. Longitudinal Association of Dietary Acid Load with kidney function decline in an older adult population with metabolic syndrome. Front Nutr. (2022) 9:986190. doi: 10.3389/fnut.2022.986190
21. Silva, L, Moço, SA, Antunes, ML, Ferreira, AS, and Moreira, AC. Dietary acid load and relationship with albuminuria and glomerular filtration rate in individuals with chronic kidney disease at Predialysis state. Nutrients. (2021) 14:170. doi: 10.3390/nu14010170
22. Mirmiran, P, Yuzbashian, E, Bahadoran, Z, Asghari, G, and Azizi, F. Dietary Acid-Base load and risk of chronic kidney disease in adults: Tehran lipid and glucose study. Iran J Kidney Dis. (2016) 10:119–25.
23. Banerjee, T, Crews, DC, Wesson, DE, Tilea, AM, Saran, R, Ríos-Burrows, N, et al. High dietary acid load predicts Esrd among adults with Ckd. J Am Soc Nephrol. (2015) 26:1693–700. doi: 10.1681/asn.2014040332
24. Machado, AD, Marchioni, DM, Lotufo, PA, Benseñor, IM, and Titan, SM. Dietary acid load and the risk of events of mortality and kidney replacement therapy in people with chronic kidney disease: the Progredir cohort study. Eur J Clin Nutr. (2024) 78:128–34. doi: 10.1038/s41430-023-01361-0
25. Kiefte-de Jong, JC, Li, Y, Chen, M, Curhan, GC, Mattei, J, Malik, VS, et al. Diet-dependent acid load and type 2 diabetes: pooled results from three prospective cohort studies. Diabetologia. (2017) 60:270–9. doi: 10.1007/s00125-016-4153-7
26. Abshirini, M, Bagheri, F, Mahaki, B, Siassi, F, Koohdani, F, Safabakhsh, M, et al. The dietary acid load is higher in subjects with prediabetes who are at greater risk of diabetes: A case-control study. Diabetol Metab Syndr. (2019) 11:52. doi: 10.1186/s13098-019-0447-5
27. Zhao, R, Zhou, L, Lei, G, Wang, S, Li, Y, Yang, X, et al. Dietary acid load is positively associated with risk of gestational diabetes mellitus in a prospective cohort of Chinese pregnant women. Front Nutr. (2022) 9:892698. doi: 10.3389/fnut.2022.892698
28. Hatami, E, Abbasi, K, Salehi-Sahlabadi, A, Beigrezaei, S, Bahrami, A, Ghiasvand, R, et al. Dietary acid load and risk of type 2 diabetes mellitus: A case-control study. Clin Nutr ESPEN. (2022) 48:308–12. doi: 10.1016/j.clnesp.2022.01.029
29. Ozturk, EE, and Yildiz, H. Examining dietary acid load in individuals with type 2 diabetes: A case-control study. Eur Rev Med Pharmacol Sci. (2023) 27:2443–52. doi: 10.26355/eurrev_202303_31778
30. Dehghan, P, and Abbasalizad, FM. Dietary acid load, blood pressure, fasting blood sugar and biomarkers of insulin resistance among adults: findings from an updated systematic review and Meta-analysis. Int J Clin Pract. (2020) 74:e13471. doi: 10.1111/ijcp.13471
31. Marunaka, Y. Roles of interstitial fluid Ph and weak organic acids in development and amelioration of insulin resistance. Biochem Soc Trans. (2021) 49:715–26. doi: 10.1042/bst20200667
32. Marunaka, Y. Molecular mechanisms of obesity-induced development of insulin resistance and promotion of amyloid-Β accumulation: dietary therapy using weak organic acids via improvement of lowered interstitial fluid Ph. Biomol Ther. (2023) 13:779. doi: 10.3390/biom13050779
33. Esche, J, Shi, L, Sánchez-Guijo, A, Hartmann, MF, Wudy, SA, and Remer, T. Higher diet-dependent renal acid load associates with higher glucocorticoid secretion and potentially bioactive free glucocorticoids in healthy children. Kidney Int. (2016) 90:325–33. doi: 10.1016/j.kint.2016.02.033
34. Karimi, R, Yanovich, A, Elbarbry, F, and Cleven, A. Adaptive effects of endocrine hormones on metabolism of macronutrients during fasting and starvation: A scoping review. Meta. (2024) 14:336. doi: 10.3390/metabo14060336
35. Ikizler, TA, Burrowes, JD, Byham-Gray, LD, Campbell, KL, Carrero, JJ, Chan, W, et al. Kdoqi clinical practice guideline for nutrition in Ckd: 2020 update. Am J Kidney Dis. (2020) 76:S1–s107. doi: 10.1053/j.ajkd.2020.05.006
36. Zahedi, M, Akhlagh, SA, Aboomardani, M, Alipoor, R, Hosseini, SA, and Shahmirzadi, AR. Efficacy of Mediterranean diet on blood biochemical factors in type ii diabetic patients: A randomized controlled trial. Gazi Med J. (2020) 31, 714–718. doi: 10.12996/gmj.2020.166
37. Whiteley, C, Benton, F, Matwiejczyk, L, and Luscombe-Marsh, N. Determining dietary patterns to recommend for type 2 diabetes: an umbrella review. Nutrients. (2023) 15:861. doi: 10.3390/nu15040861
38. Jing, T, Zhang, S, Bai, M, Chen, Z, Gao, S, Li, S, et al. Effect of dietary approaches on glycemic control in patients with type 2 diabetes: A systematic review with network Meta-analysis of randomized trials. Nutrients. (2023) 15:3156. doi: 10.3390/nu15143156
39. Remer, T, and Manz, F. Potential renal acid load of foods and its influence on urine Ph. J Am Diet Assoc. (1995) 95:791–7.
40. Harasym, J, and Oledzki, R. Effect of fruit and vegetable antioxidants on Total antioxidant capacity of blood plasma. Nutrition. (2014) 30:511–7. doi: 10.1016/j.nut.2013.08.019
41. Wang, DD, Li, Y, Bhupathiraju, SN, Rosner, BA, Sun, Q, Giovannucci, EL, et al. Fruit and vegetable intake and mortality: results from 2 prospective cohort studies of us men and women and a Meta-analysis of 26 cohort studies. Circulation. (2021) 143:1642–54. doi: 10.1161/circulationaha.120.048996
42. Aune, D, Giovannucci, E, Boffetta, P, Fadnes, LT, Keum, N, Norat, T, et al. Fruit and vegetable intake and the risk of cardiovascular disease, Total Cancer and all-cause mortality-a systematic review and dose-response Meta-analysis of prospective studies. Int J Epidemiol. (2017) 46:1029–56. doi: 10.1093/ije/dyw319
43. Papier, K, Hartman, L, Tong, TYN, Key, TJ, and Knuppel, A. Higher meat intake is associated with higher inflammatory markers, mostly due to adiposity: results from Uk biobank. J Nutr. (2022) 152:183–9. doi: 10.1093/jn/nxab314
44. Adeva, MM, and Souto, G. Diet-induced metabolic acidosis. Clin Nutr. (2011) 30:416–21. doi: 10.1016/j.clnu.2011.03.008
45. Rehman, T, Shabbir, MA, Inam-Ur-Raheem, M, Manzoor, MF, Ahmad, N, Liu, ZW, et al. Cysteine and homocysteine as biomarker of various diseases. Food Sci Nutr. (2020) 8:4696–707. doi: 10.1002/fsn3.1818
46. Tian, S, Xu, Q, Jiang, R, Han, T, Sun, C, and Na, L. Dietary protein consumption and the risk of type 2 diabetes: A systematic review and Meta-analysis of cohort studies. Nutrients. (2017) 9:982. doi: 10.3390/nu9090982
47. Schulze, MB, Haardt, J, Amini, AM, Kalotai, N, Lehmann, A, Schmidt, A, et al. Protein intake and type 2 diabetes mellitus: an umbrella review of systematic reviews for the evidence-based guideline for protein intake of the German nutrition Society. Eur J Nutr. (2024) 63:33–50. doi: 10.1007/s00394-023-03234-5
48. Ericson, U, Hellstrand, S, Brunkwall, L, Schulz, CA, Sonestedt, E, Wallström, P, et al. Food sources of fat may clarify the inconsistent role of dietary fat intake for incidence of type 2 diabetes. Am J Clin Nutr. (2015) 101:1065–80. doi: 10.3945/ajcn.114.103010
49. Liu, S, van der Schouw, YT, Soedamah-Muthu, SS, Spijkerman, AMW, and Sluijs, I. Intake of dietary saturated fatty acids and risk of type 2 diabetes in the European prospective investigation into Cancer and nutrition-Netherlands cohort: associations by types, sources of fatty acids and substitution by macronutrients. Eur J Nutr. (2019) 58:1125–36. doi: 10.1007/s00394-018-1630-4
50. Krishnan, S, Steffen, LM, Paton, CM, and Cooper, JA. Impact of dietary fat composition on prediabetes: A 12-year follow-up study. Public Health Nutr. (2017) 20:1617–26. doi: 10.1017/s1368980016003669
51. Mirmiran, P, Esfandyari, S, Moghadam, SK, Bahadoran, Z, and Azizi, F. Fatty acid quality and quantity of diet and risk of type 2 diabetes in adults: Tehran lipid and glucose study. J Diabetes Complicat. (2018) 32:655–9. doi: 10.1016/j.jdiacomp.2018.05.003
52. Cui, Y, Hao, P, Liu, B, and Meng, X. Effect of traditional Chinese cooking methods on fatty acid profiles of vegetable oils. Food Chem. (2017) 233:77–84. doi: 10.1016/j.foodchem.2017.04.084
53. Yildiz, AY, Echegaray, N, Öztekin, S, and Lorenzo, JM. Quality and stability of frying oils and fried foods in ultrasound and microwave-assisted frying processes and hybrid technologies. Compr Rev Food Sci Food Saf. (2024) 23:e13405. doi: 10.1111/1541-4337.13405
54. Jiang, H, Wang, L, Wang, D, Yan, N, Li, C, Wu, M, et al. Omega-3 polyunsaturated fatty acid biomarkers and risk of type 2 diabetes, cardiovascular disease, Cancer, and mortality. Clin Nutr. (2022) 41:1798–807. doi: 10.1016/j.clnu.2022.06.034
55. Ong, KL, Marklund, M, Huang, L, Rye, KA, Hui, N, Pan, XF, et al. Association of Omega 3 polyunsaturated fatty acids with incident chronic kidney disease: pooled analysis of 19 cohorts. BMJ. (2023) 380:e072909. doi: 10.1136/bmj-2022-072909
56. Forouhi, NG, Koulman, A, Sharp, SJ, Imamura, F, Kröger, J, Schulze, MB, et al. Differences in the prospective association between individual plasma phospholipid saturated fatty acids and incident type 2 diabetes: the epic-interact case-cohort study. Lancet Diabetes Endocrinol. (2014) 2:810–8. doi: 10.1016/s2213-8587(14)70146-9
57. Campesi, I, Franconi, F, Seghieri, G, and Meloni, M. Sex-gender-related therapeutic approaches for cardiovascular complications associated with diabetes. Pharmacol Res. (2017) 119:195–207. doi: 10.1016/j.phrs.2017.01.023
58. Bellamy, L, Casas, JP, Hingorani, AD, and Williams, D. Type 2 diabetes mellitus after gestational diabetes: A systematic review and Meta-analysis. Lancet. (2009) 373:1773–9. doi: 10.1016/s0140-6736(09)60731-5
59. Mauvais-Jarvis, F, Clegg, DJ, and Hevener, AL. The role of estrogens in control of energy balance and glucose homeostasis. Endocr Rev. (2013) 34:309–38. doi: 10.1210/er.2012-1055
60. Anagnostis, P, Christou, K, Artzouchaltzi, AM, Gkekas, NK, Kosmidou, N, Siolos, P, et al. Early menopause and premature ovarian insufficiency are associated with increased risk of type 2 diabetes: A systematic review and Meta-analysis. Eur J Endocrinol. (2019) 180:41–50. doi: 10.1530/eje-18-0602
61. Lambrinoudaki, I, Paschou, SA, Armeni, E, and Goulis, DG. The interplay between diabetes mellitus and menopause: clinical implications. Nat Rev Endocrinol. (2022) 18:608–22. doi: 10.1038/s41574-022-00708-0
62. Tramunt, B, Smati, S, Grandgeorge, N, Lenfant, F, Arnal, JF, Montagner, A, et al. Sex differences in metabolic regulation and diabetes susceptibility. Diabetologia. (2020) 63:453–61. doi: 10.1007/s00125-019-05040-3
63. Ko, SH, and Jung, Y. Energy metabolism changes and dysregulated lipid metabolism in postmenopausal women. Nutrients. (2021) 13:4556. doi: 10.3390/nu13124556
Keywords: chronic kidney disease, type 2 diabetes mellitus, dietary acid load, potential renal acid load, net endogenous acid
Citation: Huang H, Wang Q, Zhang R, Liu F, Niu Y, Luo Y, Li S, Li T, Tang Z, Wang X, Yang J, Wang Y, Zhang L, Luo S, Zhang W, Zheng Y, Deng W, Cai G, Chen X and Dong Z (2025) Correlation between dietary acid–base load and chronic kidney disease patients with type 2 diabetes mellitus. Front. Nutr. 12:1581009. doi: 10.3389/fnut.2025.1581009
Edited by:
Ruixia Dong, Jinling Institute of Technology, ChinaReviewed by:
Ximing Wu, Hefei Normal University, ChinaFangyuan Yang, Capital Medical University, China
Copyright © 2025 Huang, Wang, Zhang, Liu, Niu, Luo, Li, Li, Tang, Wang, Yang, Wang, Zhang, Luo, Zhang, Zheng, Deng, Cai, Chen and Dong. This is an open-access article distributed under the terms of the Creative Commons Attribution License (CC BY). The use, distribution or reproduction in other forums is permitted, provided the original author(s) and the copyright owner(s) are credited and that the original publication in this journal is cited, in accordance with accepted academic practice. No use, distribution or reproduction is permitted which does not comply with these terms.
*Correspondence: Xiangmei Chen, eG1jaGVuMzAxQDEyNi5jb20=; Zheyi Dong, c2hlbmdkYWkyNkAxNjMuY29t
†These authors have contributed equally to this work