- 1Biophotonics Center, Prokhorov General Physics Institute of the Russian Academy of Sciences, Moscow, Russia
- 2Mari State University, Yoshkar-Ola, Russia
- 3Laboratory Physics of Liquids, Prokhorov General Physics Institute of the Russian Academy of Sciences, Moscow, Russia
- 4Bauman Moscow State Technical University, Moscow, Russia
- 5Federal State Budgetary Scientific Institution “Federal Scientific Agroengineering Center VIM” (FSAC VIM), Moscow, Russia
The red and blue ranges of the optical spectrum are most suitable for plant photosynthesis. Moreover, quanta of red light stimulate photosynthesis more than quanta of blue light. In northern latitudes, the average daily intensity of the red and blue parts of the spectrum is usually not sufficient for many plants. To increase the productivity of greenhouses in northern latitudes, a technology has been developed for fluoropolymer films with photoconversion nanoparticles (quantum dots) that convert UV radiation and violet light into red light. The use of photoconversion fluoropolymer films promotes an increase in the biomass of plants grown in greenhouses at high latitudes. The greatest effect is observed when tomato plants are grown under photoconversion fluoropolymer films. The biomass of tomato berry obtained from one bush grown under films is 20% higher than the biomass of berry of control tomato plants.
Introduction
Visible light, corresponding to the wavelength range of the electromagnetic spectrum of 380–780 nm, is the most important environmental factor, primarily because it serves as a source of energy for the processes of photosynthesis, resulting in the formation of organic substances from inorganic components [1]. Visible light plays a large and varied role in the life processes of not only plants, but also animals [2]. The transformation of the energy of light quanta into the energy of chemical bonds occurs through a process called photosynthesis [3]. For this, terrestrial plants need quanta of the red region of the spectrum, since they are mainly absorbed by chlorophyll - the main pigment of plants. The red light within wavelength range of 650–700 nm participates most effectively in photosynthesis. Therefore, to enhance plant growth, it is recommended to use light with a predominance of the red component of the spectrum [4]. It has been shown that changes in the intensity in the UV, visible, and even in the near-IR range of the spectrum can affect the rate of growth and development of plants [5]. For the first time, more than a century ago, the influence of the optical spectrum on the growth and development of plants was shown using absorption filters. It should be noted that in those days the use of modified light was widespread. One of the first three Nobel Prizes in Physiology or Medicine (N. Finzen, 1903) was awarded for the development of optical filters and their use for the treatment of a number of diseases using visible light. Later, monochromators were widely used to study the processes occurring in plants [6]. Later, lasers began to be used in plant physiology, which today are actively replacing LED illuminators [7]. For more than a century, a huge amount of information has been accumulated on the effect of narrow-band light sources on the growth and development of plants [8–10]. The use of narrowband sources is not the only approach. An alternative is an approach based on broad spectrum modulation such as solar radiation. This approach is less widespread and is based on the absorption of light quanta of broadband radiation in one spectral range and their re-emission in another spectral range. The materials used to re-emit light are called photoconversion materials. Today new technologies, methods and procedures have been developed with the help of photoconversion materials. Leaders in the creation, research and implementation of photoconversion materials are theranostics, biophotonics and a number of areas of physics associated with the creation of devices operating on the basis of sunlight [11–14]. The use of such technologies in agriculture is often limited to closed grounds (greenhouses, greenhouses, hotbeds, etc.) [15]. The essence of photoconversion technologies in greenhouses usually boils down to the conversion of UV radiation, green and yellow light, into blue and red light [16]. It is known that for the overwhelming majority of higher plants, red (600–750 nm) rays are of the greatest importance [17]. It is the quanta of this spectral range that are essential for the course of photosynthesis. Also, red quanta affect the processes associated with plant development. Blue and violet quanta (380–490 nm) can participate in the process of photosynthesis. In addition, they stimulate the formation of proteins, affect the processes associated with plant development [18]. Yellow (595–565 nm) and green (565–490 nm) quanta are absorbed by various carotenoids and do not play a key role in photosynthesis. In nature, light in the yellow and green ranges is mainly important for shade-loving plants and some aquatic plants [19]. UV radiation is also not directly involved in photosynthesis. It should be noted that in the solar spectrum of UV radiation no more than 1–2%, even on a clear day [20].
This article proposes a method of photoconversion of a part of UV radiation not used by plants and radiation in the violet part of the spectrum into radiation in the red part of the spectrum. Nanoparticles with exciton emission are used as photoconversion centers. Cd0.6Zn0.4Se quantum dots are one of the most technologically advanced materials for the production of photoconversion nanoparticles. By varying the size of such nanoparticles, one can shift the maximum of their fluorescence within the entire visible range of the spectrum. A low-temperature technology for incorporating such nanoparticles into fluoropolymer films is presented. The developed film can significantly increase the productivity of closed soils located in high latitudes.
Methods
Obtaining quantum dots (QD) and studying their properties. QD of Cd0.6Zn0.4Se were synthesized by injecting trioctylphosphine–Se into a metal solution at 320°C. The solution contained a constant precursor ratio of Cd/Zn = 1/9 and oleic acid and oleylamine surfactants in octadecene. Details of the technology for manufacturing QD Cd0.6Zn0.4Se were described earlier [13]. The advantage of this technology is the ability to obtain QD with a controlled ratio of metals such as cadmium and zinc. To prepare photoconversion polymer films, a QD solution in acetone (7%) was used. The QD solution was mixed with the liquid fluoropolymer component in a ratio of 1/100. Experimental details related to the low-temperature introduction of nanoparticles into polymer matrices were published earlier [21]. The resulting mixture was stirred until homogeneous. From the obtained colloidal solution, photoconversion fluoropolymer films were obtained. Zetasizer Nano (Malvern, United States) was used to determine the QD sizes [22]. The morphology of the generated nanoparticles was investigated using a Libra 200 FE HR transmission electron microscope (Carl Zeiss). Details of the sample preparation of nanoparticles for microscopy were published earlier [23]. Optical absorption and photoluminescence were measured using high-speed spectrometers (OceanOptics, United States) on optical devices described earlier [23]. A laboratory illumination system based on a 150 W XBO150W/4 xenon lamp (OSRAM, Germany) was used as a light source. Ultra violet 3W High Power Led (375 nm) was used as an additional source of excitation. In the spectral study of films, light from both sources was projected in one place on the film, passed through the film, and collected on the other side using a light-collecting device. When using such an optical scheme, the relative position of lighting devices is of little importance. The main thing is that the relative position does not change during the experiment. Dosimetry was performed using a PM200 power and radiation energy meter (Thorlabs, United States).
Plant cultivation and morphometry. The effect of photoconversion fluoropolymer films on the morphometric parameters of plants was carried out in a climatic room. Work on the cultivation of fruiting plants was carried out in greenhouses. In this case, the plants were grown in soil. Details on plant cultivation have been published earlier [24]. Pepper (Capsicum annuum L.), eggplant (Solanum melongena), common cucumber (Cucumis sativus), tomato (Solanum lycopersicum) were used as objects of research. The main morphometric parameter was the area of leaves. The area of leaves was determined using the GreenImage software developed by our team [25]. The software can be downloaded here (https://drive.google.com/open?id=1gcDcz1A9iGdd48YaXWO7duWMG263sotS).
Results
The characteristics of photoconversion nanoparticles and fluoropolymer films based on them, which convert UV radiation and violet radiation into the red region of the visible spectrum, have been investigated. Figure 1A shows the QD size distribution. It was found that the maximum distribution is made up of nanoparticles with a size of about 7.5 nm. Distribution half-width 2 nm (from 6.5 to 8.5 nm). The size distribution contains a small amount of QDs with sizes greater than 20 nm (less than 0.1% of the total). The dynamic light scattering data was confirmed by electron microscopy (Figure 1B).
The photoluminescence spectra of QD in acetone and in a fluoropolymer are studied. Excitation was carried out using a diode at a wavelength of 375 nm (Figure 3). As can be seen from the graph (Figure 2A), with photoluminescence, photons have an average energy of about 1.9 eV (half-width 1.83–1.95 eV), which corresponds to about 650 nm. The determined quantum yield was 17%. The photoluminescence spectrum of a fluoropolymer film containing photoconversion nanoparticles was investigated (Figure 2B). Photoluminescence was excited with a xenon lamp and an additional diode source (375 nm) to isolate the luminescence peak. When light passes through a fluoropolymer that does not containing QD, the base spectrum of the lamp does not undergo significant changes. When light passes through a fluoropolymer containing QD, a luminescence peak with an emission maximum of 650 nm is added to the lamp spectrum. In general, it can be argued that a composite photoconversion film makes it possible to obtain base light with a superposition of a “red” component on it.
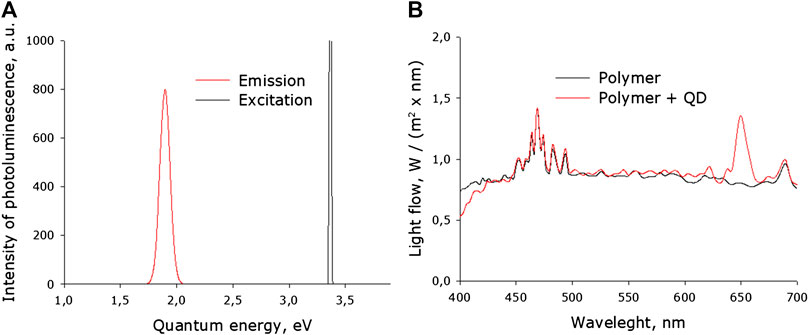
FIGURE 2. The photoluminescence spectrum of QD. (A)Photoluminescence spectrum of photoconversion nanoparticles in acetone. (B) The photoluminescence spectrum of a fluoropolymer containing and not containing photoconversion nanoparticles. Luminescence was excited with a xenon lamp and a diode source (375 nm).
The effect of photoconversion fluoropolymer films on the growth rate of Capsicum annuum L., Solanum melongena, Cucumis sativus, and Solanum lycopersicum has been studied. It was shown that photoconversion polymer films increase the growth rate of all studied plants (Figure 3A). The results in Figure 3A are expressed in relative units. The control values for each plant culture are taken as 100%. For ease of perception of the results, we have combined the control values obtained from the cultivation of all types of plants. Statistical studies were carried out only among plants of one species. The median leaf area of Cucumis sativus grown under photoconversion film is about 15% larger than the leaf area of control plants. However, from a statistical point of view, such differences are not significant. The leaf area of pumpkin, pepper and tomato grown under photopolymer films is 25, 30, and 50% higher, respectively, compared to control plants. The greatest influence on the growth rate is observed in tomato plants. In Figure 3B shows the dynamics of changes in the leaf area of tomatoes grown under control film and photoconversion film. It was found that the leaf area of tomato plants grown under a photoconversion coating by the end of 4 weeks of growing season is significantly larger than in the control group. By the end of the fifth week of the growing season, the difference reaches on average almost 50%.
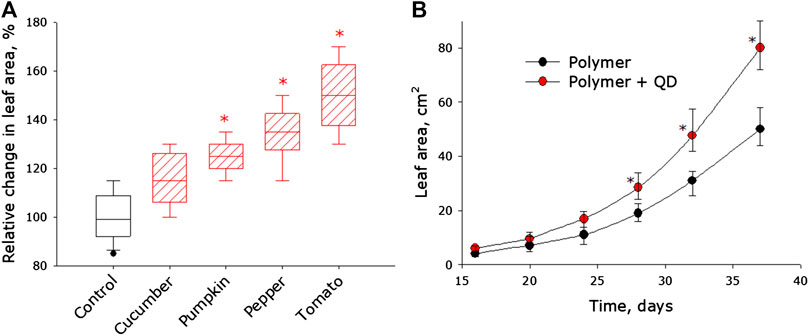
FIGURE 3. Effect of photoconversion fluoropolymer film on the growth and development of plants of various species. (A) Effect of photoconversion polymer film on the size of leaves of cucumber (Cucumis sativus), pumpkin (Cucurbita pepo), pepper (Capsicum annuum L), tomato (Solanum lycopersicum). • - control values are presented for all plants, the differences were calculated by groups. Other reference values are normalized. Data are presented as median with 95% confidence interval (box). The range is shown in the form of a whiskers. Statistically significant differences between сontrol group and QD groups (Mann-Whitney U test, p < 0.05) are marked by asterisks. (B) Dynamics of changes in the leaf area of control tomato and tomato plants grown under a photoconversion polymer film. Statistically significant differences between control group and QD groups (Mann-Whitney U test, p < 0.05) are marked by asterisks.
For most crops, not only the amount of biomass is important, but also the weight of the fruit. Since the photoconversion polymer films had the greatest effect on tomato plants, further research was carried out on these plants. In photoconversion coated greenhouses, we were able to grow tomato fruit (Figure 4A). In control greenhouses, about 7.5 kg of tomato berry were collected from one bush (Figure 4B). When growing plants under photoconversion polymer films, the median weight of tomato berry is about 9 kg per bush. Thus, in conditions of high latitudes, the use of photoconversion films makes it possible to obtain 20% more tomato berry.
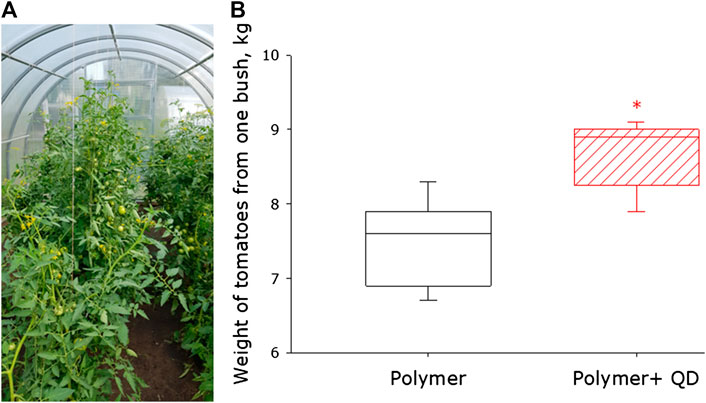
FIGURE 4. Influence of photoconversion fluoropolymer film on tomato yield. (A) Photo of tomatoes grown inside a greenhouse made from photoconverted polymer film. (B) Weight of tomato berry harvested from one tomato bush grown under a control polymer film and photoconversion plastic film. Data are presented as median with 95% confidence interval (box). The range is shown in the form of a whiskers. Statistically significant differences between control group and QD groups (Mann-Whitney U test, p < 0.05) are marked by asterisks.
Discussion
The properties of a composite fluoropolymer film containing photoconversion nanoparticles with fluorescence in the red spectral region have been investigated (Figures 1, 2). The recorded quantum yield of nanoparticles is comparable to the previously recorded quantum yield of nanoparticles of this type [26]. The proposed technology for manufacturing quantum dots is extremely convenient, since it allows one to obtain QD with photoluminescence in the entire optical range. The developed technology of low-temperature incorporation of QD into the polymer also makes it possible to work in the future with various types of nanoparticles. A possible unsolved problem is the vapor permeability of fluoropolymers, especially in the surface layer [27], although this index of fluoropolymers is often much lower compared to other classes of polymers [28].
Photoconversion fluoropolymer films have the greatest effect on the development of pepper and tomato plants, and the least on cucumber and pumpkin plants (Figure 3A). Pepper and tomato belong to the Solanaceae family, cucumber and pumpkin belong to the Cucurbitaceae family. It is known that the structure of the light-harvesting complex may differ in different plant families [29]. It is likely that by changing the QD photoluminescence wavelength, an increase in the rate of biomass accumulation can be achieved not only in plants of the Solanaceous family, but also in plants of other families. It is known that the structure of the light-harvesting complex undergoes changes in the process of plant development [30]. It has been shown that the leaf area of tomato plants grown under a photoconversion coating is stably larger than that of control plants from the first weeks of life (Figure 3B). Thirty days after germination, the difference in the area of the leaf blades reaches almost 50%. The weight of tomatoes berry per bush is only 20% higher for plants grown under a photoconversion film than for control plants (Figure 4). Probably, the spectrum of the photoconversion fluoropolymer film is optimal for the growth of tomato plants at the stage of germination, but at later stages of ontogenesis it requires correction.
Conclusion
The manuscript presents a technology for obtaining photoconversion fluoropolymer films based on QD for greenhouses. Films are capable of converting UV radiation and violet radiation into the red region of the visible spectrum. A slight increase in the intensity of radiation in the red region of the spectrum promotes an increase in the biomass of plants grown in greenhouses located in high latitudes. The greatest effect is observed when tomato plants are grown under photoconversion fluoropolymer films. The use of films allows you to get 20% more biomass of fruits from one bush.
Data Availability Statement
The original contributions presented in the study are included in the article/supplementary material, further inquiries can be directed to the corresponding author.
Author Contributions
VI, VK, and AS conducted experiments. KB, NB, and MD participated in the processing of the results and their discussion. AD and SG participated in writing the text of the manuscript.
Funding
This work was supported by a grant of the Ministry of Science and Higher Education of the Russian Federation for large scientific projects in priority areas of scientific and technological development (grant number 075-15-2020-774).
Conflict of Interest
The authors declare that the research was conducted in the absence of any commercial or financial relationships that could be construed as a potential conflict of interest.
Acknowledgments
The authors are grateful to the Center for Collective Use of the GPI RAS for the equipment provided.
References
1. Sæbø A, Krekling T, Appelgren M. Light quality affects photosynthesis and leaf anatomy of birch plantlets in vitro. Plant Cell Tissue Organ Cult (1995) 41:177–85. doi:10.1007/BF00051588
2. Gudkov SV, Andreev SN, Barmina EV, Bunkin NF, Kartabaeva BB, Nesvat AP, et al. . Effect of visible light on biological objects: physiological and pathophysiological aspects. Phys Wave Phenom (2017) 25:207–13. doi:10.3103/S1541308X17030074
3. Arnon DI. Conversion of light into chemical energy in photosynthesis. Nature (1959) 184:10–21. doi:10.1038/184010a0
4. Kuleshova TE, Likhachev AI, Pavlova ES, Kuleshov DO, Nashchekin AV, Gall NR. Interrelation of absorption spectra of plant pigments and LED lighting with different spectral compositions. Tech Phys (2018) 63:1243–47. doi:10.1134/S1063784218090104
5. Warrington IJ, Mitchell KJ. The influence of blue- and red-biased light spectra on the growth and development of plants. Agric Meteorol (1976) 16:247–62. doi:10.1016/0002-1571(76)90045-5
6. Haxo FT, Blinks LR. Photosynthetic action spectra of marine algae. J Gen Physiol (1950) 33:389–422. doi:10.1085/jgp.33.4.389
7. Alrifai O, Hao X, Marcone MF, Tsao R. Current review of the modulatory effects of LED lights on photosynthesis of secondary metabolites and future perspectives of microgreen vegetables. J Agric Food Chem (2019) 67:6075–90. doi:10.1021/acs.jafc.9b00819
8. Alvar-Beltrán J, Fabbri C, Verdi L, Truschi S, Dalla Marta A, Orlandini S. Testing proximal optical sensors on quinoa growth and development. Remote Sens (2020) 12:1958. doi:10.3390/rs12121958
9. Landi M, Zivcak M, Sytar O, Brestic M, Allakhverdiev SI. Plasticity of photosynthetic processes and the accumulation of secondary metabolites in plants in response to monochromatic light environments: a review. Biochim Biophys Acta Bioenerg (2020) 1861:148131. doi:10.1016/j.bbabio.2019.148131
10. Giraldo JP, Wu H, Newkirk GM, Kruss S. Nanobiotechnology approaches for engineering smart plant sensors. Nat Nanotechnol (2019) 14:541–53. doi:10.1038/s41565-019-0470-6
11. Edmonds AM, Sobhan MA, Sreenivasan VKA, Grebenik EA, Rabeau JR, Goldys EM, et al. . Nano‐ruby: a promising fluorescent probe for background‐free cellular imaging. Part Part Syst Char (2013) 30:506–13. doi:10.1002/ppsc.201200112
12. Guryev EL, Volodina NO, Shilyagina NY, Gudkov SV, Balalaeva IV, Volovetskiy AB, et al. . Radioactive (90Y) upconversion nanoparticles conjugated with recombinant targeted toxin for synergistic nanotheranostics of cancer. Proc Natl Acad Sci USA (2018) 115:9690–95. doi:10.1073/pnas.1809258115
13. Pu YC, Hsu YJ. Multicolored Cd(1-x)Zn(x)Se quantum dots with type-I core/shell structure: single-step synthesis and their use as light emitting diodes. Nanoscale (2014) 6:3881–8. doi:10.1039/C3NR06158B
14. Guryev EL, Shilyagina NY, Kostyuk AB, Sencha LM, Balalaeva IV, Vodeneev VA, et al. . Preclinical study of biofunctional polymer-coated upconversion nanoparticles. Toxicol Sci (2019) 170:123–32. doi:10.1093/toxsci/kfz086
15. Khramov RN, Kreslavski VD, Svidchenko EA, Surin NM, Kosobryukhov AA. Influence of photoluminophore-modified agro textile spunbond on growth and photosynthesis of cabbage and lettuce plants. Optic Express (2019) 27:31967–77. doi:10.1364/OE.27.031967
16. Abdel-Ghany AM, Al-Helal IM, Alzahrani SM, Alsadon AA, Ali IM, Elleithy RM. Covering materials incorporating radiation-preventing techniques to meet greenhouse cooling challenges in arid regions: a review. Sci World J (2012) 2012:906360. doi:10.1100/2012/906360
17. Wolf BM, Blankenship RE. Far-red light acclimation in diverse oxygenic photosynthetic organisms. Photosynth Res (2019) 142:349–59. doi:10.1007/s11120-019-00653-6
18. Petroutsos D, Tokutsu R, Maruyama S, Flori S, Greiner A, Magneschi L, et al. . A blue-light photoreceptor mediates the feedback regulation of photosynthesis. Nature (2016) 537:563–6. doi:10.1038/nature19358
19. Smith HL, McAusland L, Murchie EH. Don’t ignore the green light: exploring diverse roles in plant processes. J Exp Bot (2017) 68:2099–110. doi:10.1093/jxb/erx098
20. Verdaguer D, Jansen MA, Llorens L, Morales LO, Neugart S. UV-A radiation effects on higher plants: exploring the known unknown. Plant Sci (2017) 255:72–81. doi:10.1016/j.plantsci.2016.11.014
21. Barmina EV, Mel’nik NN, Rakov II, Shafeev GA. Optical properties of nanocomposites based on polymers and metal nanoparticles. Phys Wave Phenom (2017) 5:165–9. doi:10.3103/S1541308X17030013
22. Gudkov SV, Penkov NV, Baimler IV, Lyakhov GA, Pustovoy VI, Simakin AV, et al. . Effect of mechanical shaking on the physicochemical properties of aqueous solutions. Int J Mol Sci. (2020) 21:E8033. doi:10.3390/ijms21218033
23. Chernov AS, Reshetnikov DA, Kovalitskaya YuA, Manokhin AA, Gudkov SV. Influence of wideband visible light with an padding red component on the functional state of mice embryos and embryonic stem cells. J Photochem Photobiol B (2018) 188:77–86. doi:10.1016/j.jphotobiol.2018.09.007
24. Gudkov SV, Simakin AV, Bunkin NF, Shafeev GA, Astashev ME, Glinushkin AP, et al. . Development and application of photoconversion fluoropolymer films for greenhouses located at high or polar latitudes. J Photochem Photobiol B (2020) 213:112056. doi:10.1016/j.jphotobiol.2020.112056
25. Gudkov SV, Shafeev GA, Glinushkin AP, Shkirin AV, Barmina EV, Rakov II, et al. . Production and use of selenium nanoparticles as fertilizers. ACS Omega (2020) 5:17767–74. doi:10.1021/acsomega.0c02448
26. Fitzmorris BC, Pu YC, Cooper JK, Lin Y, Hsu YJ, Li Y, et al. . Optical properties and exciton dynamics of alloyed core/shell/shell Cd(1-x)Zn(x)Se/ZnSe/ ZnS quantum dots. ACS Appl Mater Interfaces (2013) 5:2893–900. doi:10.1021/am303149r
27. Bunkin NF, Kozlov VA, Shkirin AV, Ninham BW, Balashov AA, Gudkov SV. Dynamics of Nafion membrane swelling in H2O/D2O mixtures as studied using FTIR technique. J Chem Phys (2018) 148:124901. doi:10.1063/1.5022264
28. Graunke T, Schmitt K, Raible S, Wöllenstein J. Towards enhanced gas sensor performance with fluoropolymer membranes. Sensors (2016) 16:1605. doi:10.3390/s16101605
29. Rogl H, Schödel R, Lokstein H, Kühlbrandt W, Schubert A. Assignment of spectral substructures to pigment-binding sites in higher plant light-harvesting complex LHC-II. Biochemistry (2002) 41:2281–7. doi:10.1021/bi015875k
Keywords: nanoscale photoluminophor, quantum dots, photoconversion fluoropolymer films, growth and development of plants, closed ground
Citation: Ivanyuk VV, Shkirin AV, Belosludtsev KN, Dubinin MV, Kozlov VA, Bunkin NF, Dorokhov AS and Gudkov SV (2020) Influence of Fluoropolymer Film Modified With Nanoscale Photoluminophor on Growth and Development of Plants. Front. Phys. 8:616040. doi: 10.3389/fphy.2020.616040
Received: 10 October 2020; Accepted: 11 November 2020;
Published: 03 December 2020.
Edited by:
Alexander Vladimirovich Simakin, Prokhorov General Physics Institute (RAS), RussiaReviewed by:
Andrei Zvyagin, Macquarie University, AustraliaOksana Sherstneva, Lobachevsky State University of Nizhny Novgorod, Russia
Copyright © 2020 Ivanyuk, Shkirin, Belosludtsev, Dubinin, Kozlov, Bunkin, Dorokhov and Gudkov. This is an open-access article distributed under the terms of the Creative Commons Attribution License (CC BY). The use, distribution or reproduction in other forums is permitted, provided the original author(s) and the copyright owner(s) are credited and that the original publication in this journal is cited, in accordance with accepted academic practice. No use, distribution or reproduction is permitted which does not comply with these terms.
*Correspondence: Sergey V. Gudkov, c19tYWthcml5QHJhbWJsZXIucnU=