Analysis of Reservoir Seismic Law Based on Three-Dimensional Morphological Characteristics of Joint Surface Related to Listric Fault
- 1College of Electrical Engineering and New Energy, China Three Gorges University, Yichang, China
- 2Key Laboratory of Geological Hazards on Three Gorges Reservoir Area (China Three Gorges University), Ministry of Education, Yichang, China
- 3College of Hydraulic and Environmental Engineering, China Three Gorges University, Yichang, China
Since water storage, earthquakes occurred in Badong County, Hubei Province, accounted for as much as one-third of the strong earthquakes in the Three Gorges Reservoir area. For example, the MS5.1 earthquake occurred in December 2013 near the Gaoqiao fault in Badong County. The earthquake time, magnitude, and location analysis showed that reservoir earthquakes in this area exhibited some characteristics, such as periodicity, migration, and deep extension. Based on the regional stratum lithology and structural characteristics, this paper designed a curved joint on a small scale to simulate the structural morphology of the Gaoqiao fault and carried out triaxial compression tests under different immersion times to analyze the morphological parameters of the joint surface. The results showed that topological parameters such as root mean square height (Sq), arithmetic average height (Sa), reverse load area ratio (Smc), and minimum autocorrelation length (Sal) could effectively characterize the degree of damage and deterioration of curved joints. The test privides a reference for analyzing the evolution law of the seismic characteristics of the reservoir.
Introduction
In the construction of engineering activities, such as reservoirs and energy exploitation, human beings found the induced earthquakes [1] and began to study their temporal and spatial distribution [2–5], regional lithology [6–8], and dynamic response [9].
Reservoir earthquake is a geological phenomenon with complex seismic geology, long duration, and varying damage degrees. The mechanism of the reservoir earthquake has not been fully studied so far. Reservoir earthquake is also one of the special problems in the large-scale development and utilization of water resources. For high dams and large reservoirs, moderate or large earthquakes may cause damage to hydraulic structures and losses of personnel and property. So far, the M6.3 Koyna earthquake that occurred on December 10, 1967, has been the largest and the most destructive reservoir-triggered earthquake [10]. The earthquake caused about 200 deaths, 1,500 injuries and left thousands homeless. More than 300 M ≥ 3 earthquakes occurred in the Koyna Dam reservoir area from 1963 to 1995. In terms of the probability of occurrence, not all reservoirs will necessarily have a reservoir earthquake after establishment. The seismicity includes the increase in seismic activity after water storage [11], weakening [12], periodic changes, changes during an earthquake [13], etc.
Harsh K. Gupta et al. [14] found that some large reservoirs did not trigger earthquakes, while some small reservoirs caused obvious seismic activity. They believe that geology and structure may be important factors in determining the potential earthquake of a reservoir. Talwani [15] used improved positioning parameters to analyze multiple earthquakes in the Koyna area and inferred that seismogenic blocks were under several fluid-filled fracture zones. Seismic activity occurred westward in the Koyna River Fault Zone (KRFZ) and eastward in the northeast-south-south Patan fault. The northwest-southeast rupture that separates the area between the KRFZ and Patan faults may be the channel that extends from the near-surface to the depth of the earthquake source, allowing the penetration of fluid into the depth of the earthquake source. Therefore, the special geological structure is one of the factors that must be considered in the seismic research of reservoirs.
The Three Gorges Project is the largest water conservancy project in China. The earthquake monitoring in the Three Gorges Reservoir area began in 1954. Based on the monitoring data, the largest earthquake in the reservoir area before the dam impoundment was the Ms5.1 earthquake in Longhuiguan, Zigui County in 1979. According to the “Ecology and Environmental Monitoring Bulletin of the Three Gorges Project on the Yangtze River” (1996–2017) issued by the Ministry of Environmental Protection over the years, earthquakes in the reservoir area are mainly distributed in Badong County, Zigui County, Hubei Province, and Wushan County, Chongqing City. Many scholars [16–19] believe that the earthquake that occurred in Badong County, Hubei Province, is inextricably linked to the special geological structure of the area, especially the Gaoqiao fault.
At present, seismic research on special geological structures still focuses on seismic parameter research [20], with little consideration of the special shape of the structure [21]. The existing literature [22–24] on jointed rock masses mainly studies the influence of geometric parameters, including the inclination angle [25], length, and connectivity rate [26], on the mechanical properties of rock masses. There are few joint studies on the inclination angle changes. In this paper, the listric fault structure of the Gaoqiao fault was simplified as a curved joint in the rock sample. Long-term soaking was carried out for the limestone in the seismic area of the reservoir, and triaxial compression tests considering the water pressure of the fissure were carried out to study the morphological characteristics of the joint surface. Combined with the temporal and spatial laws of reservoir earthquakes, the mechanism of triggering earthquakes was analyzed. The results indicated that the changes in the morphological characteristics of the joint surfaces could explain the seismic laws of the reservoir in the listric fault structure area.
Seismic Law of Badong Section in Three Gorges Reservoir Area
According to the statistics of the “Operation Record of the Three Gorges Project on the Yangtze River” released by the Yangtze Three Gorges Group, from the beginning of water storage in 2003 to the maximum water level in 2009, the number of earthquakes in the Three Gorges reservoir area has reached tens of thousands. Earthquakes in the reservoir area are mainly manifested in weak earthquakes, slight earthquakes, and extremely small earthquakes, and individual earthquakes have reached the level of moderate to strong earthquakes. Due to artificial earthquakes and the loss of some seismic data before 2009, this article mainly analyzed earthquakes with a magnitude greater than 1 in Badong County from 2009 to 2020.
Periodic
The number of earthquakes from 2009 to 2020 is listed in Figure 1. At the initial stage of the 175 m testing impoundment from 2009 to 2010, the number of earthquakes in the reservoir increased rapidly, forming the first peak after impoundment. From 2011 to 2013, the number of earthquakes in the reservoir declined, entering a period of relative calm. From the end of 2013 to the end of 2014, the number of earthquakes in the reservoir increased again, and the intensity was weaker than that from 2009 to 2010. From 2015 to mid-2017, the reservoir earthquake entered a relatively quiet period once again. From mid-2017 to 2018, the number of reservoir earthquakes rebounded again, entering a relatively active period. The average time of the relative active period is 2 years, and the average time of the relatively quiet period is 3 years.
Migration
In order to clearly illustrate the movement of the epicenter, Figure 1 only shows the epicenter location of the reservoir earthquake from 2009 to 2011. Most earthquakes are located on both sides of the Gaoqiao fault north of the Yangtze River. Although the earthquake distribution has been concentrated and mostly near the Gaoqiao fault during the relatively active period of reservoir earthquakes from 2009 to 2010, the trend of the epicenter moving to the periphery is obvious.
Extension Downward
The focal depth of the reservoir earthquake is mainly concentrated in 3–10 km, indicating that the rupture distribution area in the formation is relatively concentrated. Since 2016, the maximum depth of the seismic source has shown an increasing trend (Figure 1). From 2016 to 2020, many earthquakes with a depth of about 20 km have occurred in the reservoir area, indicating that the scope of influence of the reservoir water is extending to the deep crust.
Experiment Method
Since the Three Gorges reservoir area began to store water in 2003, the number of earthquakes in the Badong Reservoir of the Three Gorges Reservoir area has increased significantly. The occurrence of earthquakes is closely related to the water level, the water storage period [27], and the special geological structure of the Gaoqiao fault. This article generalized the Gaoqiao fault and strata into limestone samples with variable dip angle joints and soaked them for different lengths of time in advance to simulate the immersion effect of reservoir water on the strata in the reservoir area. According to the characteristics of water level change, the water level is divided into four periods: high water level period (from December to February of the next year), water level descending period (from March to May), low water level period (from June to August) and water level rising period (from September to November). The average time of each period is 90 days, the maximum immersion time is set to 90 days. After the immersion, fracture water pressure was applied to the joint surface. A triaxial compression test was carried out to simulate the mechanical response of the fault under the long-term action of reservoir water, and the correlation between the fault and the macro-seismic law was analyzed through the variation of topographic parameters on the joint surface. The characteristics of the sample and the test process are shown in Figure 2. Because different positions of the joint surface have different forces during the test, the root mean square height (Sq), arithmetic average height (Sa), and reverse load has been selected for the joints in this article. The main analysis parameters are the area ratio (Smc) and the minimum autocorrelation length (Sal). The Sq represents The standard deviation of the height distribution, or RMS surface roughness for computing the standard deviation for the amplitudes of the surface. The Sa represents the mean surface roughness. The Smc represents the height c at which a given areal material ratio p is satisfied. The height is calculated from the mean surface. The Sal represents the horizontal distance of the autocorrelation function (tx, ty) has the fastest decay to a specified value s (0 < s < 1). The default value for s in the software is 0.2. This parameter indicates the content of the wavelength on the surface. A high value indicates that the surface has mainly high wavelengths (low frequencies). The change rates of the topography parameters of the concave and convex surfaces in the joint were considered. Among them, the Sq, Sa, Smc, and Sal characterize the degree of discrete change in the height of each point on the joint surface before and after the test, the change in the average height of each point in the defined area, the smoothness change of the joint surface before and after the test, and the offset degree of the upper and lower part of the joint surface before and after the test, respectively.
The test procedure was as follows. The rock sample in the saturated state was selected to implement different soaking conditions, including non-soaking, soaking for 10 days, soaking for 30 days, soaking for 60 days, and soaking for 90 days. According to the self-weight stress of the rock masses in the study area, the initial axial pressure and confining pressure in the triaxial test was selected to be 100 and 50 MPa, respectively. After the initial stress was applied, different water pressures (0 MPa, 20 MPa) were applied to the fracture surface through the permeable holes at the bottom of the rock sample. The water pressure remained unchanged after a period of time, and the axial pressure continued until the specimen was broken. In the test, the axial pressure loading rate was 2 MPa/min, the confining pressure loading rate was 1 MPa/min, and the hydraulic loading rate was 1 MPa/min. When the water pressure reached the predetermined value, the water pressure was maintained for 30 min to ensure that the water pressure was evenly distributed on the fracture surface.
Analysis of Test Results
The influence of soaking time on the strength of jointed rock samples under different pore water pressures is shown in Figure 3. In the rock samples with different pore water pressures and different immersion time, the triaxial compressive strength fluctuates to different degrees. The comparison of different working conditions (not applying fracture water pressure after immersion and applying 20 MPa fracture water pressure) showed a similar variation of the compressive strength of the sample. From 0 to 10 days of soaking, the compressive strength increases first, then decreases. Among them, the strength of the rock sample is the highest at 10-day soaking. From 10 to 30 days of soaking, the compressive strength decreases. From soaking for 30–90 days, the triaxial compressive strength increases again.
When the immersion time is 10 days, the tiny pores on the surface of the rock sample are saturated with water due to adsorption. When the rock sample is compressed, the bearing capacity of the pores is greatly enhanced. Due to the short immersion time, there is no significant connection between the pores due to water-rock chemistry, and the strength of the rock sample is increased. When the immersion time is 30 days, micro-cracks that expand inward due to water-rock chemistry between the pores will promote the interconnection of the micro-pores in the rock sample, increase the stress concentration point, and ultimately reduce the strength. After immersion for 60 days or 90 days, the softening effect of water on the rock sample becomes prominent. During the loading process, the passage between the pores will be closed so that the saturated pores can bear the load, which increases strength. Figure 3 shows that the failure form on the joint surface is related to the triaxial compressive strength. When the strength is lower, the number of cracks on the joint surface is more and cracks are distributed closer to the center of the sample. When the strength is higher, the number of cracks on the joint surface is less, and cracks are distributed closer to the edge of the specimen. The results shows that the increase of the immersion time has a non-uniform influence on the strength and failure morphology of the sample.
Correlation Between Test Results and Earthquake Laws
The number of earthquakes in the reservoir in Figure 1 shows a trend of periodic change and decreasing volatility with time, which is highly similar to the trend of the triaxial compressive strength of the sample with the immersion time. This phenomenon shows that the bearing capacity of the stratum within the influence of the reservoir does not change monotonously under immersion. When the bearing capacity is strong, the possibility of earthquakes is reduced. When the bearing capacity of the stratum is small, the possibility of earthquakes increases.
The distribution of cracks of the sample after immersion for 90 days (Figure 3) and the phenomenon that the concave surface is more damaged than the convex surface in the analysis of the morphological parameter change rate show that the footwall of the fault is more likely to produce horizontal and downward cracks when an earthquake occurs. A new reservoir underwater seepage channel has been formed, which macroscopically manifested as the migration of earthquakes.
Figure 4 shows the change rate of joint surface morphology parameters under different immersion time and fracture water pressures (0 and 20 MPa). The data of Figure 4 have been added in the Supplementary Material. When the immersion time is 0 and 90 days, the change rate of the parameters on the joint surface of the sample is low. When the immersion time is 10, 30, and 60 days, the change rate of the morphology parameters is large, indicating that the local deterioration of the joint surface is more obvious. Among them, the change rate of morphology parameters under the condition of fracture water pressure of 20 MPa is lower than that under the condition of fracture water pressure of 0 MPa. In the initial stage of water storage, the fault that occurred during the earthquake has the strongest degree of non-uniformity and the largest number of earthquakes. In the mid-term of water storage, local degradation occurs on the fault plane of the earthquake, and the number of earthquakes decreases slightly. In the late stage of water storage, the degree of degradation of the rock mass on the seismogenic fault plane is relatively uniform, and the number of earthquakes is correspondingly reduced.
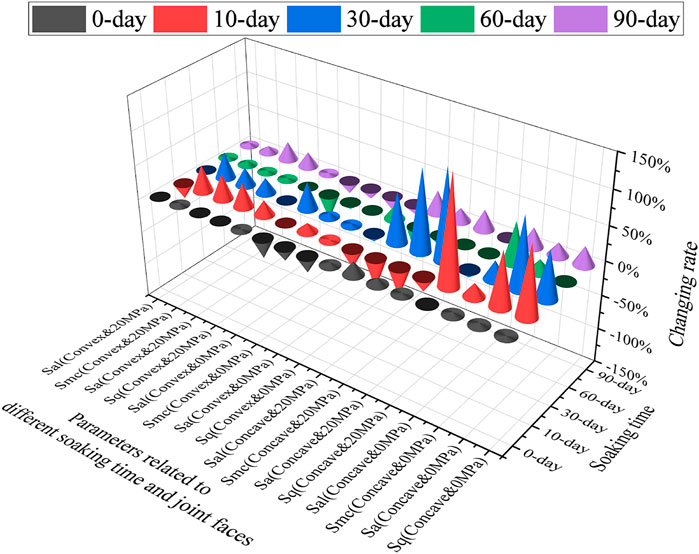
FIGURE 4. The change rate of joint surface morphology parameters under different immersion time and fracture water pressure conditions.
Conclusion
Based on the seismic data of the reservoir since the impoundment of the Three Gorges Reservoir, the periodicity, horizontality, and downward migration of earthquakes are analyzed. Rock samples with curved joints are used to simulate special geological structures. By applying different fracture water pressures and different immersion times, The time-effect of water storage on the earthquake-generating fault is simulated. The test results can better explain the earthquake occurrence law of the reservoir earthquake. The conclusions are as follows:
1) Earthquakes in the Badong section of the Three Gorges Reservoir area are periodic and horizontal and tend to migrate to the depths. It is feasible to simulate special geological structures in rock samples and study the influence of reservoir water on seismogenic faults through indoor triaxial compression tests.
2) The study of morphological parameters on curved joints shows that the influence of immersion time on rock strength is non-linear, and the strength changes periodically, but the fluctuations weaken in a short period of time. Large fracture water pressure can reduce the anisotropy on the joint surface, which shows that the change rate of morphology parameters is relatively low.
3) Seismic faults have the largest anisotropy in the initial stage of reservoir immersion, and the number of earthquakes is the largest. The local degradation of the seismogenic fault is constantly changing over the immersion time, and the number of seismogenesis presents a certain periodicity. When the reservoir water is immersed for a certain period of time, the degree of degradation of the earthquake-producing fault will be more uniform, and the number of earthquakes will be decreased.
Data Availability Statement
The original contributions presented in the study are included in the article/Supplementary Material, further inquiries can be directed to the corresponding author.
Author Contributions
GD contributed to the conception of the study and performed the experiment; YW contributed significantly to analysis and manuscript preparation; JC performed the data analyses and wrote the manuscript; JZ helped perform the analysis with constructive discussions.
Funding
This work was supported by the financial support by Open Fund of Key Laboratory of Geological Hazards on Three Gorges Reservoir Area (China Three Gorges University), Ministry of Education (Grant No. 2020KDZ12), the National Nature Science Foundation of China (Grant No. 52009067), China National Natural Science Foundation Joint Funded Project (No. U1965109), Hubei Provincial Natural Science Foundation Innovation Group Project (No.2020CFA049), the National Nature Science Foundation of China (Grant No.U2034203).
Conflict of Interest
The authors declare that the research was conducted in the absence of any commercial or financial relationships that could be construed as a potential conflict of interest.
Publisher’s Note
All claims expressed in this article are solely those of the authors and do not necessarily represent those of their affiliated organizations, or those of the publisher, the editors and the reviewers. Any product that may be evaluated in this article, or claim that may be made by its manufacturer, is not guaranteed or endorsed by the publisher.
Acknowledgments
The authors gratefully acknowledge the financial support by Open Fund of Key Laboratory of Geological Hazards on Three Gorges Reservoir Area (China Three Gorges University), Ministry of Education (Grant No. 2020KDZ12), the National Nature Science Foundation of China (Grant No. 52009067), China National Natural Science Foundation Joint Funded Project (No. U1965109), Hubei Provincial Natural Science Foundation Innovation Group Project (No.2020CFA049), the National Nature Science Foundation of China (Grant No.U2034203).
Supplementary Material
The Supplementary Material for this article can be found online at: https://www.frontiersin.org/articles/10.3389/fphy.2021.811609/full#supplementary-material
References
1. Li L, Tan J, Wood DA, Zhao Z, Becker D, Lyu Q, et al. A Review of the Current Status of Induced Seismicity Monitoring for Hydraulic Fracturing in Unconventional Tight Oil and Gas Reservoirs. FUEL (2019) 242:195–210. doi:10.1016/j.fuel.2019.01.026
2. Ferreira PAR, Ferreira JM, Do Nascimento AF, Bezerra FHR, Lima Neto HC, Menezes EAS. Seismicity Migration Induced by the Açu Reservoir, Northeast Brazil, and Implications for Fault Hydraulic Variability. Bulletin. Seismol. Soc. Am. (2018) 108(5B):3107–18. doi:10.1785/0120170372
3. Meng L, Mcgarr A, Zhou L, Zang Y. An Investigation of Seismicity Induced by Hydraulic Fracturing in the Sichuan Basin of China Based on Data from a Temporary Seismic Network. Bulletin. Seismol. Soc. Am. (2019) 109(1):348–57. doi:10.1785/0120180310
4. Lellouch A, Reshef M. Velocity Analysis and Subsurface Source Location Improvement Using Moveout-Corrected Gathers[J]. GEOPHYSICS (2019) 84(3):S119–S131. doi:10.1190/geo2018-0517.1
5. Willacy C, van Dedem E, Minisini S, Li J, Blokland J-W, Das I, et al. Full-waveform Event Location and Moment Tensor Inversion for Induced Seismicity[J]. Geophysics (2019) 84(2):S39–S57. doi:10.1190/geo2018-0212.1
6. Santos JE, Savioli GB. Long‐wave Equivalent Viscoelastic Solids for Porous Rocks Saturated by Two‐phase Fluids. Geophys. J. Int. (2018) 214(1):302–14. doi:10.1093/gji/ggy136
7. He Y-X, Wu X-Y, Fu K, Zhou D, Wang S-X. Modeling the Effect of Microscopic and Mesoscopic Heterogeneities on Frequency-dependent Attenuation and Seismic Signatures. IEEE Geosci Remote Sensing Lett (2018) 15(8):1174–8. doi:10.1109/lgrs.2018.2829837
8. Guo J, Rubino JG, Glubokovskikh S, Gurevich B. Dynamic Seismic Signatures of Saturated Porous Rocks Containing Two Orthogonal Sets of Fractures: Theory versus Numerical Simulations. Geophys. J. Int (2018) 213(2):1244–62. doi:10.1093/gji/ggy040
9. Telesca L, Fat-Elbary R, Stabile TA, Haggag M, Elgabry M. Dynamical Characterization of the 1982-2015 Seismicity of Aswan Region (Egypt). Tectonophysics (2017) 712-713:132–44. doi:10.1016/j.tecto.2017.05.009
10. Gupta HK, Arora K, Purnachandra Rao N, Roy S, Tiwari VM, Patro PK, et al. Investigations of Continued Reservoir Triggered Seismicity at Koyna, India. Geol Soc Lond Spec Publications (2017) 445(1):151–88. doi:10.1144/sp445.11
11. Tuan TA, Purnachandra Rao N, Gahalaut K, Trong C D, Van D L, Chien C, et al. Evidence that Earthquakes Have Been Triggered by Reservoir in the Song Tranh 2 Region, Vietnam[J]. J Seismology (2017) 21(5):1131–43. doi:10.1007/s10950-017-9656-2
12. Gahalaut K, Hassoup A, Hamed H, Kundu B, Gahalaut V. Long-Term and Annual Influence of Aswan Reservoir (Egypt) on the Local Seismicity: A Spatio-Temporal Statistical Analysis. Pure Appl Geophys (2017) 174(1):133–50. doi:10.1007/s00024-016-1397-6
13. Gahalaut K, Tuan TA, Purnachandra Rao N. Rapid and Delayed Earthquake Triggering by the Song Tranh 2 Reservoir, Vietnam. Bull Seismological Soc America (2016) 106(5):2389–94. doi:10.1785/0120160106
14. Harsh K, Gupta A Review of Recent Studies of Triggered Earthquakes by Artificial Water Reservoirs with Special Emphasis on Earthquakes in Koyna, India[J]. Amsterdam, Netherlands: Earth-Science Reviews (2002).
15. Talwani P. On the Nature of Reservoir-Induced Seismicity. Pure Appl Geophys (1997) 150(3-4):473–92. doi:10.1007/978-3-0348-8814-1_8
16. Dong J. Analysis of Seismic Activity in Gaoqiao Fault. Crustal Deformation and Earthquake (2004).
17. Zhang LF, Liao WL, Li JG, Wei G-C, Shen X-L. Analysis On The 2013 Badong M5.1 Earthquake Sequence And The Seismogenic Structure[J]. Seismology and Geology (2016) 38(3):747–59. doi:10.3969/j.issn.0253-4967.2016.03.019
18. Dai M, Yao Y, Chen J. Study on Relationship Between Earthquake Activity in Three Gorges Reservoir Area and Water Level in Front of dam[J]. Yangtze River: China International Book Trading Corporation (2010).
19. Junhua C, Lingyun Z, Haibo W. Study on the Focal Mechanism of the M5.1 Badong Earthquake in Hubei. Geodesy and Geodynamics (2014) 5(1):47–54. doi:10.3724/sp.j.1246.2014.01047
20. Zhang L, Lei X, Liao W, Li J, Yao Y. Statistical Parameters of Seismicity Induced by the Impoundment of the Three Gorges Reservoir, Central China. Tectonophysics (2019) 751:13–22. doi:10.1016/j.tecto.2018.12.022
21. Duan G, Lehua W, Huafeng D, Jingyu Z, Zuosen L, Qiao J, et al. Mechanical Response of Listric Faults in the Three Gorges Reservoir Area Based on Three-Dimensional Morphological Characteristics. Front Phys (2021) 9:604. doi:10.3389/fphy.2021.766920
22. Assefa E, Feng DH, Deng H. Degradation Mechanism of Intermittent Jointed sandstone under Water-Rock Interaction[J]. Yantu Gongcheng Xuebao/Chinese J Geotechnical Eng (2021) 43(4):634–43. doi:10.11779/CJGE202104005
23. Shu PY, Li HH, Wang TT. Dynamic Strength of Rock with Single Planar Joint under Various Loading Rates at Various Angles of Loads Applied[J]. J Rock Mech Geotechnical Eng (2018) 10(3), 5. doi:10.1016/j.jrmge.2018.01.005
24. Yin DW, Chen SJ, Chen B. Strength and Failure Characteristics of the Rock-Coal Combined Body with Single Joint in Coal[J]. Geomechanics Eng (2018) 15(5):1113–24. doi:10.12989/gae.2018.15.5.1113
25. Duan G, Li J, Zhang J. Mechanical Properties and Failure Modes of Rock Specimens with Specific Joint Geometries in Triaxial Unloading Compressive Test[J]. Adv Mater Sci Eng (2019) 14. doi:10.1155/2019/1340934
26. Guoyong D, Jianlin L, Jingyu Z. The Mechanical Behaviour of Rock Specimens with Specific Edge Crack Distributions under Triaxial Loading Conditions[J]. J Geophys Eng (2019)(5) 5. doi:10.1093/jge/gxz059
Keywords: reservoir earthquake, curved joints, seismic laws, three-dimensional shape parameters, mechanical properties
Citation: Duan G, Wang Y, Chen J and Zhang J (2022) Analysis of Reservoir Seismic Law Based on Three-Dimensional Morphological Characteristics of Joint Surface Related to Listric Fault. Front. Phys. 9:811609. doi: 10.3389/fphy.2021.811609
Received: 09 November 2021; Accepted: 13 December 2021;
Published: 05 January 2022.
Edited by:
Qingxiang Meng, Hohai University, ChinaReviewed by:
Yue Li, Sinosteel Maanshan General Institute of Mining Research Co.Ltd, ChinaZhipeng Xiang, Yangtze River Scientific Research Institute, China
Copyright © 2022 Duan, Wang, Chen and Zhang. This is an open-access article distributed under the terms of the Creative Commons Attribution License (CC BY). The use, distribution or reproduction in other forums is permitted, provided the original author(s) and the copyright owner(s) are credited and that the original publication in this journal is cited, in accordance with accepted academic practice. No use, distribution or reproduction is permitted which does not comply with these terms.
*Correspondence: Yanhai Wang, yanhaiwang@ctgu.edu.cn