- 1Hawkesbury Institute for the Environment, Western Sydney University, Penrith, NSW, Australia
- 2Instituto Nacional de Investigación Agropecuaria, Colonia, Uruguay
- 3AgResearch, Ruakura Research Centre, Hamilton, New Zealand
- 4Department of Animal and Plant Sciences, The University of Sheffield, Sheffield, United Kingdom
Cool season grasses associate asymptomatically with foliar Epichloë endophytic fungi in a symbiosis where Epichloë spp. protects the plant from a number of biotic and abiotic stresses. Furthermore, many grass species can accumulate large quantities of silicon (Si), which also alleviates a similar range of stresses. While Epichloë endophytes may improve uptake of minerals and nutrients, their impact on Si is largely unknown. Likewise, the effect of Si availability on Epichloë colonization remains untested. To assess the bidirectional relationship, we grew tall fescue (Festuca arundinacea) and perennial ryegrass (Lolium perenne) hydroponically with or without Si. Grasses were associated with five different Epichloë endophyte strains [tall fescue: AR584 or wild type (WT); perennial ryegrass: AR37, AR1, or WT] or as Epichloë-free controls. Reciprocally beneficial effects were observed for tall fescue associations. Specifically, Epichloë presence increased Si concentration in the foliage of tall fescue by at least 31%, regardless of endophyte strain. In perennial ryegrass, an increase in foliar Si was observed only for plants associated with the AR37. Epichloë promotion of Si was (i) independent of responses in plant growth, and (ii) positively correlated with endophyte colonization, which lends support to an endophyte effect independent of their impacts on root growth. Moreover, Epichloë colonization in tall fescue increased by more than 60% in the presence of silicon; however, this was not observed in perennial ryegrass. The reciprocal benefits of Epichloë-endophytes and foliar Si accumulation reported here, especially for tall fescue, might further increase grass tolerance to stress.
Introduction
Symbiotic relationships between plants and fungi have a long evolutionary history with plant fossils containing fungal endophytes dating back 400-million-years (Krings et al., 2007). Worldwide, many cool-season (C3) grasses, including important wild and domesticated species, associate asymptomatically with Epichloë fungi (Ascomycota: Clavicipitaceae) (Leuchtmann, 1992; Kauppinen et al., 2016). Asexual Epichloë endophytes reside intercellularly (apoplastic space) in aerial plant parts, and are vertically transmitted via host seed (Leuchtmann, 1992; Christensen et al., 2008). Epichloë endophytes are true obligate symbionts, and their growth is tightly synchronized with their host plant (Christensen et al., 2008). Epichloë-grass associations are known to benefit grasses in a number of ways, including increased growth (Gundel et al., 2013), better tolerance to water deficits (Rho et al., 2018) and resistance to pathogens (Xia et al., 2018) and herbivores (Bastias et al., 2017), the latter mainly via production of endophyte specific protective alkaloids (nitrogenous compounds) (Bastias et al., 2017). While some studies suggest endophytes play a limited role in stress alleviation (Niones and Takemoto, 2014; Wiewióra et al., 2015; Heineck et al., 2020), and might have antagonistic responses under extreme resource limitations conditions (Cheplick et al., 1989; Cheplick, 2007; Saikkonen et al., 2016), it is recognized that their beneficial effects are now widely reported (Kuldau and Bacon, 2008; Perez et al., 2017). Because of these benefits, animal-safe endophytes strains (e.g., AR1, AR31, AR584), that don’t have the genes accountable for producing mammalian toxic alkaloids, are commercially available in marketed varieties of several perennial forage grasses such as tall fescue, perennial ryegrass, and cocksfoot (Gundel et al., 2013; Kauppinen et al., 2016). These animal-safe strains improve forage quality and persistence, and maintain endophyte-mediated resistance to insects pests without affecting grazing mammals (Gundel et al., 2013); some negative repercussions have been reported for horses, however (Munday et al., 2017).
Additionally, it is long known that grasses are high accumulators of silicon (Si) (Raven, 1983), especially perennial species that co-evolved with herbivores (Massey et al., 2007; Cooke and Leishman, 2011). Si can account for up to 10% of dry mass, and grasses take up more Si than any other inorganic constituent (Epstein, 1994; Kumar et al., 2017). Silicon is taken up by the roots as monosilicic acid (H4SiO4), using either passive (transpiration flow) or active (membrane transporters) mechanisms, before polymerizing in cell walls (Ma and Yamaji, 2015; Coskun et al., 2019). Plant silicification (anamorphous Si deposits) occurs in cell walls, cell lumens, intercellularly (apoplastic space), or within leaf surfaces (e.g., trichomes or phytoliths) (Kumar et al., 2017). Once polymerized, Si cannot be remobilized (Hartley et al., 2015). Silicification of plant tissues has been shown to alleviate a wide range of stresses, some of which are similarly alleviated by Epichloë endophyte associations. Stresses alleviated by Si include herbivory (Hartley et al., 2015; Reynolds et al., 2016), pathogens (Vivancos et al., 2015; Rasoolizadeh et al., 2018), low temperatures (Zhang et al., 2008), UV radiation (Tripathi et al., 2017), and nutrient deficiency (Miao et al., 2010). The mechanisms underpinning this stress alleviation remain controversial but silicification of plant tissues may provide physical resistance (Coskun et al., 2019) or indirectly cause changes in plant chemistry (Hall et al., 2019). Moreover, Si supplementation has also been reported to increase plant growth (Frew et al., 2018) and enhance uptake of major essential nutrients (Eneji et al., 2008), although these effects mostly take place during stressful conditions (Coskun et al., 2019). For these reasons, Si is promoted as a sustainable fertilizer (Eneji et al., 2008).
Despite endophytes and Si accumulation being important for many grass species, and given that they perform similar functions, their effects have mostly been studied separately. Thus, it is currently unknown if and how Epichloë endophytes and Si interact (Johnson et al., 2016). To date, only one field study noted that meadow fescue (Festuca pratensis) colonized with Epichloë uncinata contained 16% more Si relative to non-symbiotic plants (Huitu et al., 2014). Although this field study did not control for Si-availability, it provides indirect evidence that that endophytes might increase Si concentrations in grasses.
Several putative mechanisms could be envisaged for endophytes and Si impacting one another. For instance, both endophyte (Christensen et al., 2008) and Si (Kumar et al., 2017) can occupy the same apoplastic (intercellular) space in leaves. Therefore, Si deposition could reduce spatial niches for Epichloë-mycelium, thereby hindering colonization. Additionally, both endophyte (Cheplick and Faeth, 2009) and Si (Ma and Yamaji, 2015) acquisition come at a metabolic cost to the plant, thus, there may be an optimal balance between Si accumulation and endophyte colonization. Finally, although Epichloë colonize aboveground plant tissues, they are known to influence several belowground plant functions, including nutrient/mineral acquisition, thus, may increase Si uptake. These effects are rather indirect, mediated by changes in plant growth. For instance, Epichloë can increase leaf area and transpiration rate, and thus nutrient acquisition via mass flow (Malinowski and Belesky, 2000; Soto-Barajas et al., 2016; Llorens et al., 2019), can promote changes in root growth and architecture (e.g., increasing length, reduced diameter, and longer hairs) that increase nutrient concentration gradients (Malinowski and Belesky, 2000; Ren et al., 2007; García Parisi et al., 2015) or facilitate interception with nutrient pools in the soil (Malinowski et al., 1998, 2004; Malinowski and Belesky, 2000; Ren et al., 2007; Soto-Barajas et al., 2016). Endophytes might affect soil organisms that actively facilitate nutrient absorption processes (e.g., arbuscular mycorrhizae fungi) (Novas et al., 2011). Likewise, endophyte can increase root exudation of phenolic compounds that acidify the rhizosphere and improve mineral uptake (Malinowski et al., 1998, 2004).
The objective of this study was to determine whether Si interacts with different Epichloë endophytes strains in the two most used perennial pasture grasses worldwide; tall fescue and perennial ryegrass. Specifically, five different Epichloë strains were utilized, three animal-safe strains, AR584 in tall fescue, and AR1/AR37 in perennial ryegrass, as well as their wild type (WT) (mammalian toxic) counterparts for both grasses; along with Epichloë-free controls. Both extensively used commercial animal-safe (novel) and WT strains were utilized to determine if strain-specific effects occur. In particular, we addressed three specific research questions:
(i) Do Epichloë-endophytes increase Si concentrations in foliage?
(ii) Is endophyte-mediated variation in Si concentration dependent of symbiosis induced changes in plant growth?
(iii) Does Si supplementation affect endophyte colonization?
Materials and Methods
Experimental Procedure
Seeds of tall fescue (Festuca arundinacea) cv. INIA Fortuna and perennial ryegrass (Lolium perenne) cv. Samson were obtained from the Margot Forde Germplasm Centre (Palmerston North, New Zealand). Epichloë strains utilized were the novel AR584 and the common-toxic (WT) in tall fescue, and the novel AR1 and AR37, and the common-toxic (WT) for perennial ryegrass, along with Epichloë-free controls (Nil) (Hume et al., 2013) (see section “Epichloë Detection and Mycelium Mass”). These germplasm was utilized to evaluate the effect of endophyte on foliar silicon concentrations.
All seeds were surface sterilized and transferred to trays with wet perlite growing media in a naturally lit glasshouse under controlled environmental conditions of 22/18°C (day/night), 50% relative humidity, at the Hawkesbury Institute for the Environment, Richmond, NSW, Australia. Eleven-days after germination, uniformly sized individual seedlings were transferred to polypropylene 50-mL LightSafe tubes (Sigma-Aldrich, St. Louis, MO, United States) containing 45-mL of nutrient solution with (+Si) or without (−Si) silicon (Si), as described in Hall et al. (2020) adapted from Jung et al. (2015) and Hall et al. (2020).
The solution contained: 1 mM KNO3, 1 mM Ca(NO3)2, 1 mM KH2PO4, 0.6 mM MgSO4, 100 μM NaCl, 15 μM H3BO3, 0.5 μM MnCl2, 0.7 μM ZnSO4, 0.8 μM Na2MoO4, 0.8 μM CuSO4, 100 μM NaFe EDTA (Sigma Aldrich, St. Louis, MO, United States). To generate +Si treatments, liquid potassium silicate was added to the nutrient solution (K2SiO3; Agsil32, PQ Australia, SA, Australia) at a concentration equivalent to 2 mM SiO2. Chemically, silicic acid polymerizes to form silica gel (SiO2 nH2O) when the concentration of silicic acid exceeds 2 mM (Ma and Yamaji, 2006). To balance the additional potassium (K) and chloride (Cl) ions in the +Si treatments, potassium chloride (KCl) was added to the control nutrient solution (−Si). Lastly, the pH of both solutions (+Si/−Si) was adjusted to 5.6-6 using hydrochloric acid (2M HCl) to reduce the polymerization of silicates (Ma and Yamaji, 2006).
The combination of grass species, endophyte strain and Si supply resulted in 14 treatments performed in two experimental stages. Experimental stages were deployed 4 weeks apart with at least 10 replicates of each treatment (183 plants in total; see Supplementary Table S1 for initial and final replication). The first stage was limited to tall fescue either infected with AR584 or not (Nil) and supplied with (+Si) or without Si (−Si), resulting in four treatments. The second stage included all Epichloë by genotype combinations tested in a factorial combination with and without Si.
Plants were grown in their corresponding Si treatment for a further 7 week period to ensure functional Epichloë-grass symbiosis (Hume et al., 2013; Kaur et al., 2015). Tubes within the glasshouse were shifted randomly every week to minimize position bias, and the nutrient solution was refreshed three times a week to ensure optimal growth conditions.
At each harvest (Supplementary Figure S1) two of the thickest tillers of each plant were blotted for Epichloë detection (see section “Epichloë Detection and Mycelium Mass”). Individual shoots and roots were separated, oven-dried at 60°C, weighed (MS-TA Analytical balances; Mettler Toledo) and milled.
Epichloë Detection and Mycelium Mass
Before initiating experiments, 100 mature seeds from each germplasm were stained with aniline blue and examined under the microscope (40×) to corroborate presence or absence (Nil) of endophyte hyphae in the aleurone cells (Latch and Christensen, 1985). Histological detection confirmed infection rates higher than 95% in infected seeds, and less than 3% infection in Nil. However, seed detection does not test endophyte viability.
Consequently, Epichloë viability in planta, a measure of vegetative colonization efficacy, was performed in all 183 experimental plants using tissue-print immunoblotting and histological detection immediately after harvest (Hahn et al., 2003). For tissue-print immunoblotting, the fresh cut end of each tiller was pressed onto a nitrocellulose membrane (Hahn et al., 2003; di Menna et al., 2012). Tissue-print immunoblotting results were further confirmed through histological staining (aniline blue), whereby a section of the epidermal strips from the outermost leaf sheath of 20% of the plants in each Epichloë germplasm combination (n = 7) were examined under a light microscope to corroborate presence or absence (Nil) of endophyte hyphae in intercellular spaces (di Menna et al., 2012). Immunoblotting results and histological tissue staining coincided for the subset of plants evaluated. A total of 17 plants had the wrong endophyte status (e.g., Nil that were positive) and were removed from all analyses (final replication provided in Supplementary Table S1 and in Figures 1, 2, 4).
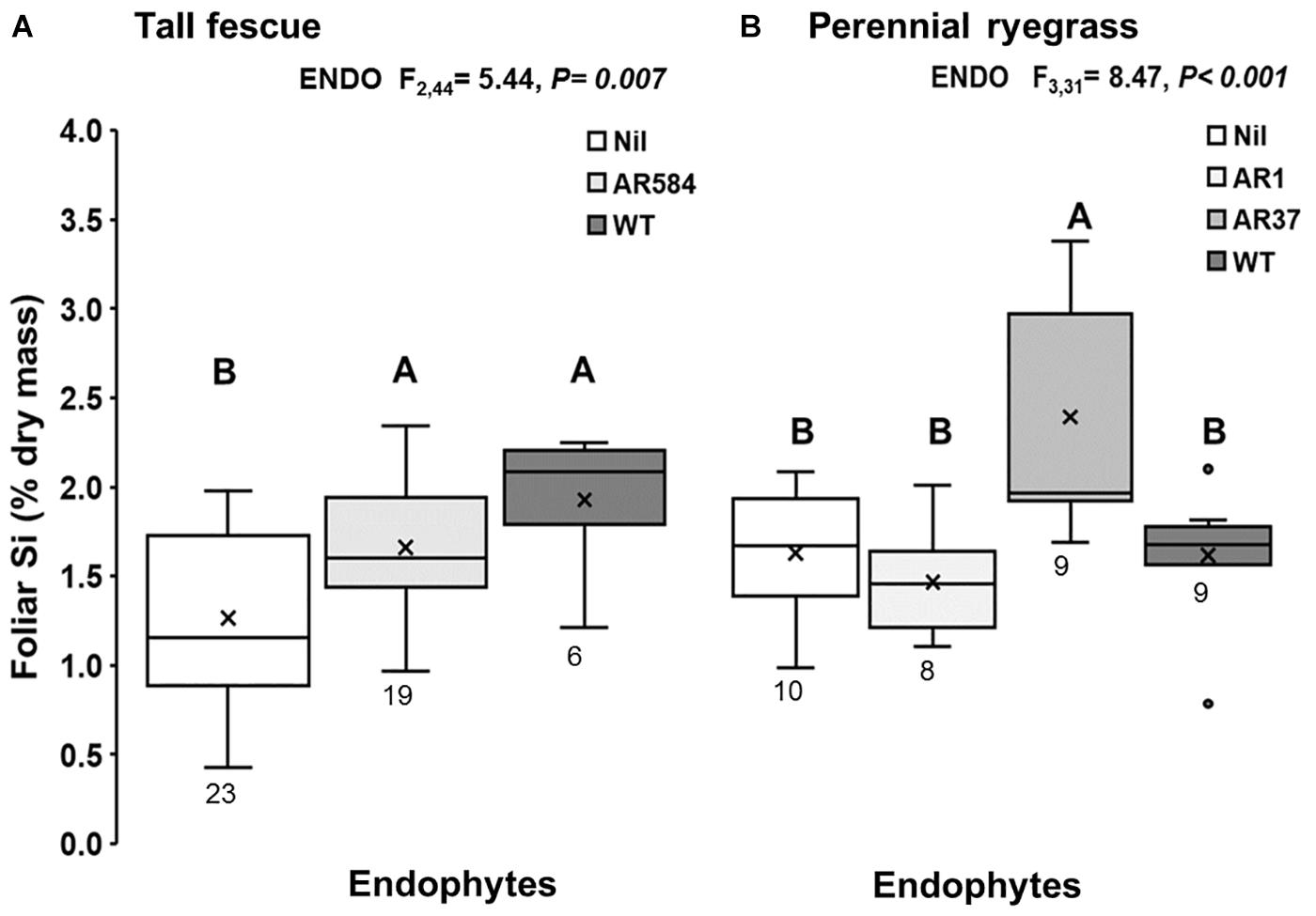
Figure 1. Foliar Si concentration of (A) tall fescue and (B) perennial ryegrass plants without endophytes (Nil) or colonized with different Epichloë endophyte strains growing hydroponically in the presence of silicon (Si). Mean values indicated with ‘X’ and lines depict inclusive median and interquartile range; resulting N indicated below each box plot (see Supplementary Table S1). Different letters indicate significant differences (P < 0.05).
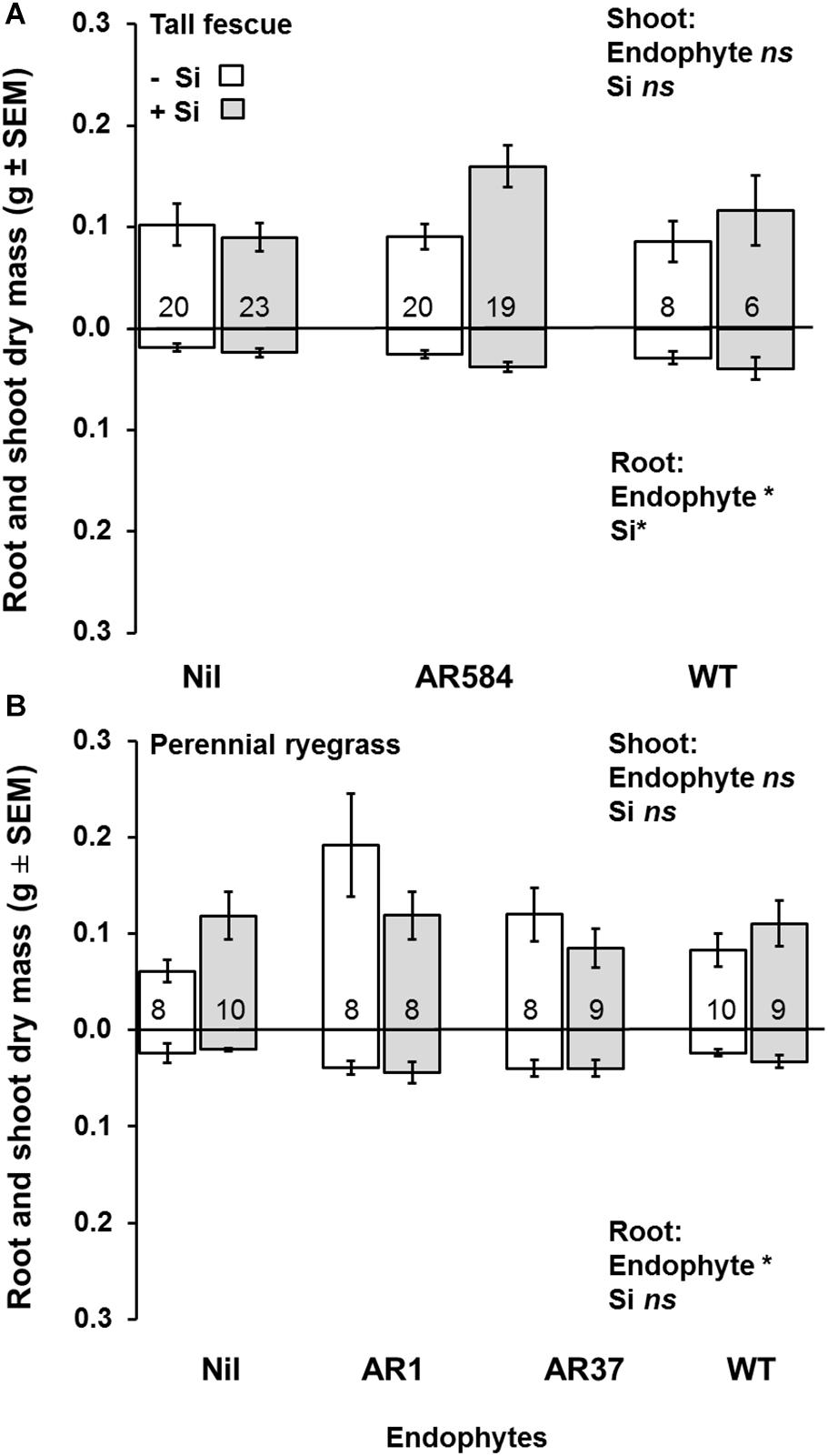
Figure 2. Variation in shoot (top) and root (bottom) dry mass for (A) tall fescue and (B) perennial ryegrass plants without endophytes (Nil) or colonized with different Epichloë endophyte strains growing hydroponically in the absence (−Si) or presence (+Si) of silicon (Si). Mean values ± standard error shown with N indicated within each bar. N is unbalanced due to removal of plants with wrong endophyte status (see Supplementary Table S1). Models with significant main effects and/or interactions (P < 0.05) noted with *, ns = non-significant effects (see Table 1 for full analysis).
Epichloë-mycelial mass was further quantified in at least five replicates per treatment (Supplementary Table S2) using sandwich ELISA following Easton et al. (2002) procedures, modified from Miles et al. (1998). Epichloë-mycelial mass was used as a measure of tissue-colonization (Easton et al., 2002). Briefly, approximately 20 mg of milled shoot tissue per plant were utilized. Samples were extracted utilizing 10 mL of phosphate buffered saline with Tween (1%), inverted to mix, incubated with the anti-endophyte antibody for 3 h at 37°C, and stored in the fridge overnight (4°C). An aliquot of 150 μL was taken for the ELISA. ELISA standard curves were prepared for each assay using Neotyphodium lolii (now E. festucae var. lolii) as standards (Miles et al., 1998; Easton et al., 2002). To minimize the effects of interplate variation, each grass sample was analyzed twice. A405 was measured with a Bio-Rad model 3550 microplate reader. The plates were washed three times with PBS after the antibody-coating and -blocking steps and twice with PBS-Tween (0.05%) and once with PBS after the sample and conjugate incubation steps (Miles et al., 1998; Easton et al., 2002). Undetectable levels of mycelium following sandwich ELISA confirmed absence of endophyte in all Nil plants.
Foliar Si
Foliar Si concentration was measured on all replicates (166 plants) on ∼80–100 mg of ground shoot tissue using X-ray fluorescence spectrometry (Epsilon 3x; PANalytical, EA Almelo, Netherlands), following Reidinger et al. (2012) procedures.
Specifically, milled samples were placed individually inside small-mass holders (PANalytical, B.V., Netherlands), and Si concentrations were measured in the presence of helium (Hiltpold et al., 2016). Si was expressed as % of dry mass, and samples were calibrated against a certified control (NCS ZC73018, Citrus leaves, China National Institute for Iron and Steel) (Hiltpold et al., 2016). −Si treatment was verified, given that all −Si plants contained undetectable levels of Si (Hiltpold et al., 2016).
Statistical Analysis
R (version 4.0.0; R Core Team, 2020) was utilized for all statistical analyses. Sample sizes were unbalanced due to removal of plants with wrong Epichloë status (Supplementary Table S1). Assumptions of normality for residuals were verified according to inspection of quantile–quantile plots. Moreover, since the experiment was performed in two time-lapsed stages, experimental ‘stage’ was accounted as a random effect in all models (Supplementary Table S1).
Root, shoot, and total biomass were analyzed with a two-way ANOVA with endophyte and Si status, and their interaction included as factors for each grass species in separate models. Biomass traits were additionally analyzed using a one-way ANOVA for each endophyte strain individually with Si status as a factor. Since endophytes increased root growth (see below), we tested the independent effects of endophytes on foliar Si concentration using a one-way ANCOVA, with endophyte status as a fixed factor and root mass fitted as a covariate. Additionally, a Spearman’s rank correlation coefficient test using the ‘cor.test’ function in R was commuted to analyze the relationship between foliar Si concentrations and Epichloë colonization for each Epichloë-strain separately. Lastly, colonization of each Epichloë-strain was analyzed with a one-way ANOVA using Si treatment as a predictor to determine the effects of Si on endophyte mycelium mass (proxy for colonization).
Results
Results showed evidence of strong interaction between Epichloë endophyte and Si. The presence of Epichloë consistently increased foliar Si concentrations in tall fescue plants with increases of 31% in plants associated with AR584 and 52% in plants associated with WT endophyte, relative to Epichloë-free (Nil) plants (Figure 1A). In perennial ryegrass, foliar Si concentrations increased by 47% only in plants associated with AR37 endophyte (Figure 1B); while the other plants displayed similar concentrations to those of Nil plants (Figure 1B).
Neither endophytes nor the addition of Si influenced shoot biomass for either grass species. Root biomass, however, was significantly increased by endophytes in both species (Figure 2 and Table 1). Including root dry mass as a covariate in ANCOVA indicated that the increased root mass associated with endophyte presence did not fully explain observed increases in foliar Si concentrations in either grass species; tall fescue (F1,44 = 1.55, P = 0.22) nor perennial ryegrass (F1,31 = 1.63, P = 0.21). In short, foliar Si concentrations were higher in endophyte colonized plants irrespective of the increased root mass observed in these plants. When traits were analyzed for each endophyte strain separately, Si significantly increased shoot, root, and total biomass only for AR584-symbiotic tall fescue (Table 2). Other than for AR584, Si did not impact growth traits of the other strains tested in either tall fescue or in perennial ryegrass (Table 2).
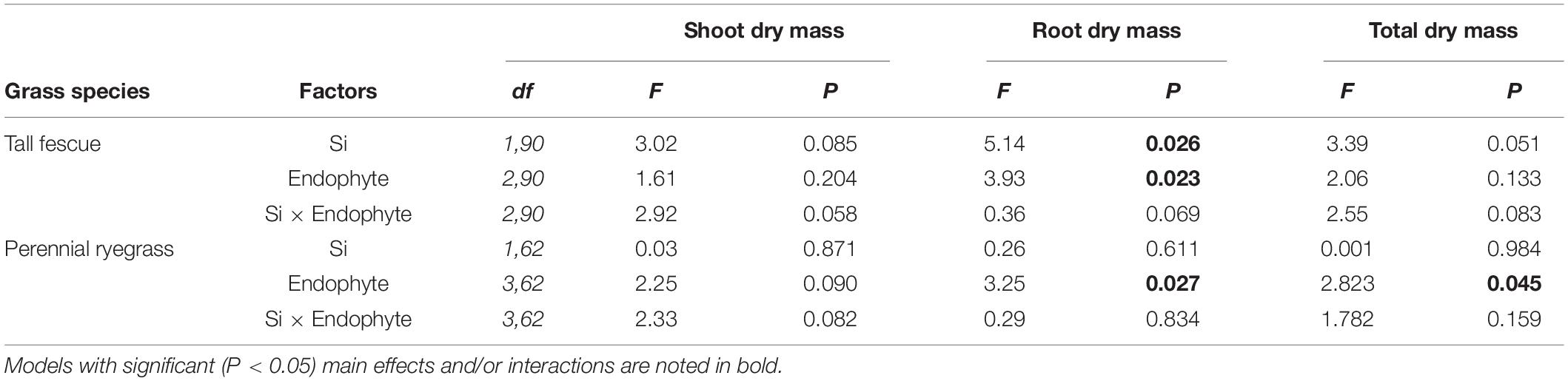
Table 1. Results from multiple comparison tests (Tukey-adjusted P-values) for changes in plant traits as affected by Si and Epichloë endophytes for tall grass fescue and perennial ryegrass.
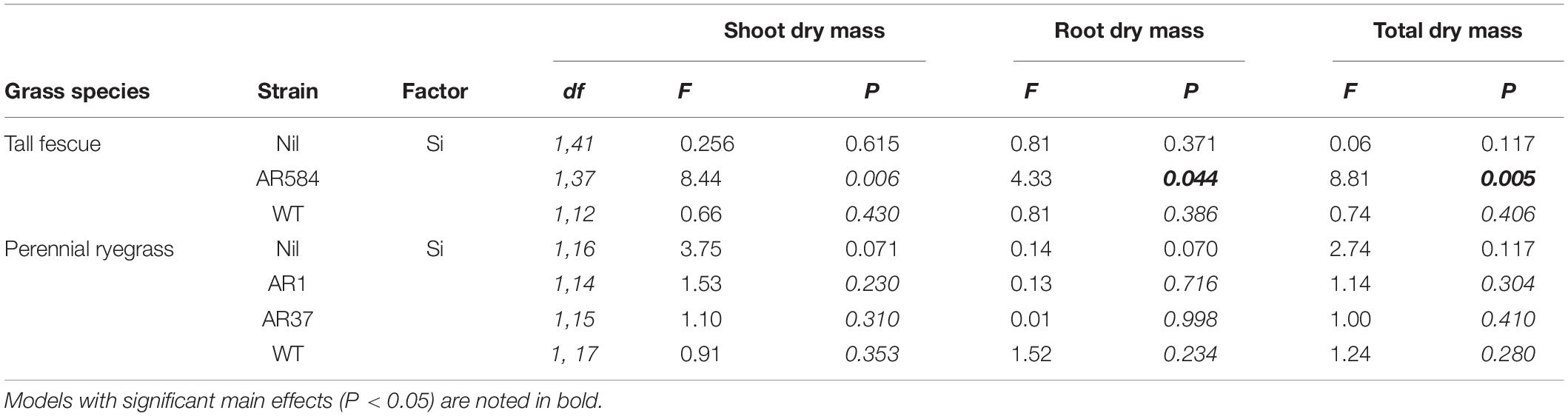
Table 2. Results from the multiple comparison tests (Tukey-adjusted P-values) for changes in plant traits as affected by Si on individual Epichloë endophyte strain for fescue and ryegrass, respectively.
Whenever endophyte effects on foliar Si concentration were observed, positive relationships between Epichloë mycelial mass and foliar Si concentrations were also detected in both tall fescue (i.e., AR584 and WT strains; Figure 3A) and perennial ryegrass (i.e., AR37 strain; Figure 3B).
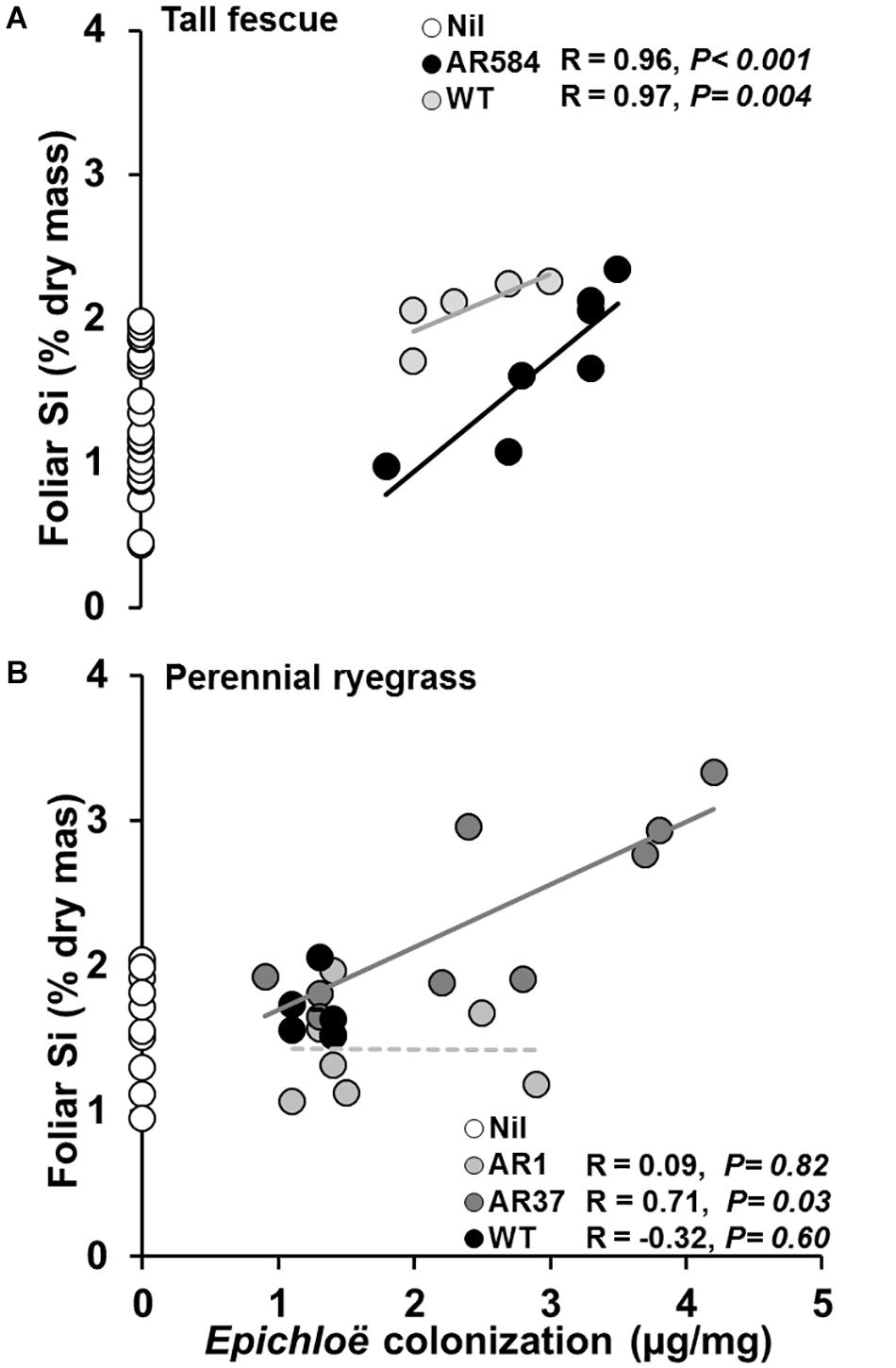
Figure 3. Relationships between Epichloë endophyte colonization and foliar Si concentration in (A) tall fescue and (B) perennial ryegrass with (filled circles) and without (Nil, white circles) endophytes growing hydroponically in the presence of silicon (Si). Lines represent linear regression through data points for each endophyte strain tested. Significant and non-significant relationships represented as solid or dashed lines, respectively. White circles represent Si concentrations from control plants (Nil) confirming lack of endophyte infection and displaying variability of Si in foliar tissue.
Lastly, there was a significant effect of Si supply on endophyte colonization in tall fescue, but not for perennial ryegrass. Specifically, tall fescue plants in the +Si treatment had 80 and 60% higher mycelial masses than their Nil counterparts for AR584 (F1,13 = 11.52, P = 0.004) and WT (F1,10 = 5.54, P = 0.05) strains, respectively (Figure 4A). For perennial ryegrass, the effects of Si on endophyte mycelial mass were non-significant for all endophyte strains tested (AR1: F1,14 = 0.06, P = 0.80, AR37: F1,15 = 0.08, P = 0.77, WT: F1,13 = 0.32, P = 0.56) (Figure 4B).
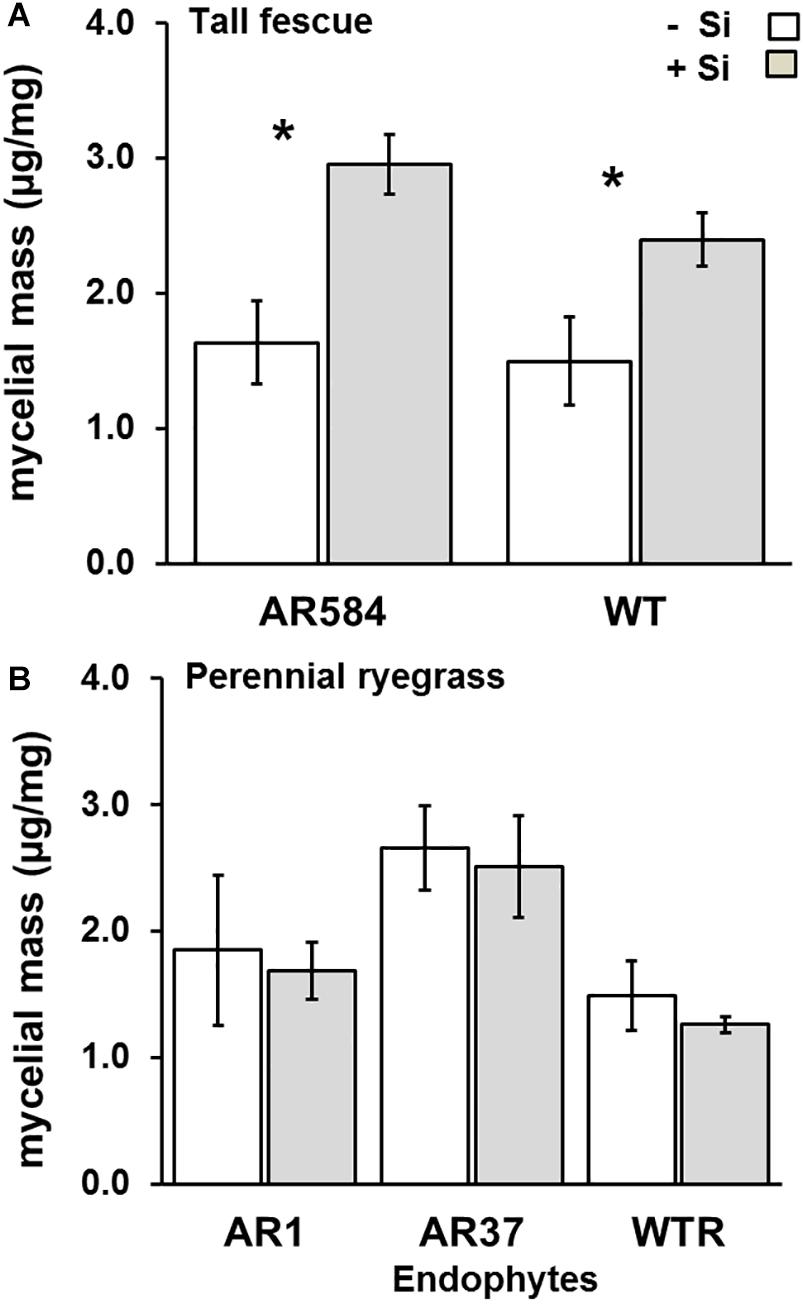
Figure 4. Variation in Epichloë endophyte colonization for (A) tall fescue and (B) perennial ryegrass with colonized different Epichloë endophyte strains growing hydroponically in the absence (–Si) or presence (+Si) of silicon (Si). Mean values ± standard error shown with N indicated within each bar. N is unbalanced due insufficient material for ELISA (see Supplementary Table S2). Asterisks indicate significant differences (P < 0.05) within each endophyte strains.
Discussion
In this study, we provide evidence that some Epichloë endophyte strains increased Si concentrations in the foliage of the two most common pasture grasses. Further we show that, whenever endophyte effects on foliar Si concentration were observed, the magnitude of this increase is positively associated with the amount of Epichloë mycelia in the host plant, and is independent of endophyte stimulation of plant growth. Moreover, using a hydroponic approach, which allows total Si exclusion (all soils contain at least some silicon), we provide novel evidence that Si-supply increases endophyte colonization in tall fescue.
While interactions varied depending on species and endophytic strain in perennial rygrass, reflecting some diversity and complexity in the relationship, for tall fescue, these results provide clear evidence of a reciprocally beneficial interaction between Epichloë-endophytes and Si. The novelty of these findings means that we have limited information about the possible mechanisms. Nonetheless, relevant studies suggest several mechanistic possibilities which we summarize below. While speculative, this provides a basis for future hypothesis-driven experiments including those conducted under more realistic field conditions.
Foliar Si Concentration Increased With Some Endophyte Strains
Endophyte presence in tall fescue, and particularly the AR37 strain in perennial ryegrass, increased Si concentrations in foliar tissue of their host by more than 30%. This supports the only existing evidence for such effects, based on field data, by Huitu et al. (2014). Huitu et al. (2014) reported higher foliar Si concentrations in endophyte-colonized meadow fescue (Schedonorus pratensis) field plots compared to endophyte-free plots. While providing original evidence of a relationship between Epichloë and Si, Huitu et al. (2014) could not determine whether increases in foliar Si arose indirectly through endophyte induced changes in plant traits (e.g., increased root mass). Moreover, as a field soil-based study, Si supply could not be controlled, so it was unclear whether Si affected endophyte colonization. Accurate quantification of plant growth and full control of Si-supply, using the current hydroponic approach, allowed these questions to be addressed in the present study.
In this study, Epichloë-mediated increases in Si were independent of responses in root growth and positively correlated with endophyte colonization, supporting evidence for an intrinsic endophyte effect. One possibility is that symbionts may increase plant transpiration rates resulting in higher passive uptake of Si (Frew et al., 2017; Dastogeer, 2018). For instance, arbuscular mycorrhizae (AM) fungi (Frew et al., 2017), nitrogen fixing bacteria (Hartley and Gange, 2009) and Epichloë endophytes (Llorens et al., 2019) have all been reported to increase plant growth and modify photosynthetic processes through increased stomatal conductance (Malinowski and Belesky, 2000). In line with this, symbionts might also directly affect number and activity of plant aquaporins (water channels that facilitate transport of water between cells) which could promote active Si uptake. For instance, expression of transporter genes in hosts associated with nickel accumulation can be altered by Epichloë (Mirzahossini et al., 2015). Similarly, observed increases in Si could possibly be related to symbionts having aquaporins that operate similarly to those taking-up Si in plant roots (Frew et al., 2017). Interestingly, when elemental composition of AM-fungi spores and extraradical mycelia was investigated, AM-fungi displayed selective uptake of various elements, including Si (Hammer et al., 2011). Unfortunately, to our knowledge, the elemental composition of Epichloë-structures has not been investigated so far.
Another putative mechanism could be through endophyte-mediated alteration of host endogenous defense, or defense-priming (Bastias et al., 2017). Essentially, to maintain functional symbiosis, symbionts increase endogenous levels of the defense hormone jasmonic acid (JA) in their host plant (Martinez-Medina et al., 2016). This induction has been reported for obligate symbionts including AM-fungi (Jung et al., 2012), rhizobial bacteria (Dean et al., 2014), as well as, Epichloë endophytes (Bastias et al., 2017). Triggering the JA-pathway, either using chemical stimulation (methyl-jasmonate) or authentic herbivory, has been shown to promote Si-uptake (Ye et al., 2013; Kim et al., 2014; Hall et al., 2019, 2020). Consequently, the resulting spike in JA mediated by Epichloë endophyte symbiosis establishment, may lead to enhanced Si-uptake. However, JA-mediated increases in Si, while significant, have been shown to be relatively small, e.g., 12 and 10% as reported in Hall et al. (2020, 2019, respectively), compared to > 30% increased Si concentration found in this study. This suggests that the stimulation of JA pathway alone is unlikely to be the sole mechanism for increased foliar Si observed in endophyte-symbiotic plants.
Moreover, morphological changes in hosts as a result of endophyte infection have resulted in, for instance, greater number of vascular bundles in perennial ryegrass (Franco et al., 2020). In line with this, Si has been reported in high concentrations both in the vascular bundles of perennial ryegrass (Dinsdale et al., 1979) and in tall fescue (Vandegeer et al., 2020). Thus, indirect morphological changes in hosts as a result of endophyte infection might further increase Si in host tissues. Further, the amount of Si in grass leaves is known to differ greatly between grass species (Massey et al., 2007), including fescue (Buckner et al., 1967) and perennial ryegrass (Buckner et al., 1967; Moore, 1984). And particularly for tall fescue the amount of Si in grass leaves also varies between varieties, with harsh varieties having higher Si content and more spines on their leaf surface than soft varieties (Hartley et al., 2015). However, for fescue and ryegrass comparable amount of Si concentrations were found for Festuca ovina and Lolium perenne in both controlled (Massey and Hartley, 2009) and field conditions (Massey and Hartley, 2006), and has been directly linked with increasing leaf abrasiveness, thus reduced herbivory (Massey and Hartley, 2006; Massey et al., 2006, 2007). Thus, morphological changes in hosts by both endophyte and Si deposition might be strain and variety specific, highlighting the complexity of these interactions even further.
Si Supply Increases Endophyte Colonization in Tall Fescue
Si supply did not reduce endophyte colonization in tall fescue nor in perennial ryegrass. This suggests that endophyte development in the apoplast of leaves is not hindered by Si deposition. Instead, endophyte colonization in tall fescue was actually increased by Si supply. The reasons for this remain speculative, but the natural evolution of a positive/neutral interaction is not unlikely, since grasses have a long evolutionary history with both endophytes (Young et al., 2014) and Si (Cooke and Leishman, 2012).
Si incorporation into cell walls is known to alter its structure (Kumar et al., 2017), and negative correlations between Si, cellulose and lignin have been reported (Klotzbücher et al., 2018). Likewise, Epichloë endophytes have been shown to decrease concentrations of lignin in perennial ryegrass regardless of plant genotype, and in contrast, showed no effect for tall fescue (Soto-Barajas et al., 2016). Epichloë-effects on host lignin are related to intracellular hyphae obtaining a supplementary source of carbon through hydrolysis of cell wall components (carbohydrates) (Rasmussen et al., 2012). Thus, if Si-supplemented plants have reduced lignin, this might affect perennial ryegrass endophyte more so than tall fescue. Thus, instead of interfering with endophyte colonization, Si deposition might in fact create additional niches for Epichloë mycelium (Christensen et al., 2008).
Further, Si accumulation in cell walls in lieu of carbon-rich structural constituents may also make more carbon available for metabolic processes (Cooke and Leishman, 2011), which could be another mechanism by which Si supply increased endophyte colonization in tall fescue. Likewise, since the endophytic fungal partner benefits from the symbiosis withdrawing assimilated photosynthetic carbon, the greater availability of carbon might strengthen the mutualism between Epichloë and its host grass (Rozpa̧dek et al., 2015). Lastly, higher mycelial mass was correlated with increased production of loline-protective alkaloids in Schedonorus pratensis (Cagnano et al., 2020). It is therefore also possible that Si-supply might increase endophyte produced alkaloids in tall fescue, at least, but this remains to be investigated.
Conclusion
There is increasing dependency on grass monocultures (e.g., tall fescue) due to reduced natural grasslands suitable for forage; therefore sustainable strategies such as use of novel endophytes or Si supplementation to provide better tolerance to biotic stressors are required. Our results provide novel evidence that some Epichloë grass associations increase foliar Si concentrations in both tall fescue and perennial ryegrass, that Si supply can increase Epichloë colonization, and that these effects depend on the specific host species–endophyte strain association considered.
These reciprocal benefits of Epichloë endophytes and Si accumulation in tall fescue were observed in both WT (mammalian-toxic) and, more importantly, for the animal-safe (novel) AR584 Epichloë strains. Naturally, this needs to occur without major effects on digestibility for livestock, which needs to be further explored. While Si has been reported to cause small reductions in digestible organic matter (Van Soest and Jones, 1968; O’Reagain and Mentis, 1989), it’s considered that many ruminants are able to cope with increased Si in forage crops (Jones and Handreck, 1965; Melzer et al., 2010; Vandevenne et al., 2013). Consequently, the potential for greater resilience to stress by combining endophyte and Si is clear, particularly for tall fescue.
Moreover, tall fescue-endophyte associations seem to increase resistance to stresses more so than those perennial ryegrass–endophyte associations (Young et al., 2013). Both endophytes and Si accumulation can individually suppress pests but the current findings, albeit from highly controlled conditions, suggest there is at least the potential for them to work in combination. Future studies should explore these interactions with the inclusion of herbivores and in natural field conditions to determine whether this potential can be realized.
Data Availability Statement
The original contributions presented in the study are included in the article/Supplementary Material, further inquiries can be directed to the corresponding author.
Author Contributions
XC-S, JP, and SJ planned and designed the research. XC-S conducted the experimental work, collected the data, and analyzed the data with input from JP and SJ. XC-S led the writing of the manuscript with significant input from SJ, JP, FL, AP, and SH. All authors contributed to the article and approved the submitted version.
Funding
This research was supported by the Australian Research Council Discovery (grant no. DP170102278).
Conflict of Interest
The authors declare that the research was conducted in the absence of any commercial or financial relationships that could be construed as a potential conflict of interest.
Acknowledgments
We acknowledge Dr. Jan Sprosen and Dr. Anouck de Bonth for assistance on immunoblotting and ELISAs. The Margot Forde Germplasm Centre (Palmerston North, New Zealand) for providing the grass seeds and Juan Raul Bentancur for technical assistance. Special thanks go to Dr. Silvina Stewart, Dr. Rebecca K. Vandegeer, Dr. Casey Hall, and Rocky Putra for feedback on the manuscript.
Supplementary Material
The Supplementary Material for this article can be found online at: https://www.frontiersin.org/articles/10.3389/fpls.2020.593198/full#supplementary-material
References
Bastias, S., Martínez-Ghersa, M., Ballaré, C., and Gundel, P. (2017). Epichloë fungal endophytes and plant defenses: not just alkaloids. Trends Plant Sci. 22, 939–948. doi: 10.1016/j.tplants.2017.08.005
Buckner, R. C., Todd, J. R., Burrus, P. B., and Barnes, R. F. (1967). Chemical composition, palatability, and digestibility of ryegrass-tall fescue Hybrids, ‘Kenwell’, and ‘Kentucky 31’ tall fescue varieties. Agron. J. 59, 345–349. doi: 10.2134/agronj1967.00021962005900040018x
Cagnano, G., Lenk, I., Roulund, N., Jensen, C. S., Cox, M. P., and Asp, T. (2020). Mycelial biomass and concentration of loline alkaloids driven by complex population structure in Epichloë uncinata and meadow fescue (Schedonorus pratensis). Mycologia 112, 474–490. doi: 10.1080/00275514.2020.1746607
Cheplick, G. P. (2007). Costs of fungal endophyte infection in Lolium perenne genotypes from Eurasia and North Africa under extreme resource limitation. Environ. Exp. Bot. 60, 202–210. doi: 10.1016/j.envexpbot.2006.10.001
Cheplick, G. P., Clay, K., and Marks, S. (1989). Interactions between infection by endophytic fungi and nutrient limitation in the grasses Lolium perenne and Festuca arundinacea. New Phytol. 111, 89–97. doi: 10.1111/j.1469-8137.1989.tb04222.x
Cheplick, G. P., and Faeth, S. H. (2009). Ecology and evolution of the grass-endophyte symbiosis. Oxford: Oxford University Press, 1–253.
Christensen, M. J., Bennett, R. J., Ansari, H. A., Koga, H., Johnson, R. D., Bryan, G. T., et al. (2008). Epichloë endophytes grow by intercalary hyphal extension in elongating grass leaves. Fungal Genet. Biol. 45, 84–93. doi: 10.1016/j.fgb.2007.07.013
Cooke, J., and Leishman, M. (2011). Is plant ecology more siliceous than we realise? Trends Plant Sci. 16, 61–68. doi: 10.1016/j.tplants.2010.10.003
Cooke, J., and Leishman, M. (2012). Tradeoffs between foliar silicon and carbon-based defences: evidence from vegetation communities of contrasting soil types. Oikos 121, 2052–2060. doi: 10.1111/j.1600-0706.2012.20057.x
Coskun, D., Deshmukh, R., Sonah, H., Menzies, J. G., Reynolds, O., Ma, J. F., et al. (2019). The controversies of silicon’s role in plant biology. New Phytol. 221, 67–85. doi: 10.1111/nph.15343
Dastogeer, K. M. G. (2018). Influence of fungal endophytes on plant physiology is more pronounced under stress than well-watered conditions: a meta-analysis. Planta 248, 1403–1416. doi: 10.1007/s00425-018-2982-y
Dean, J. M., Mescher, M. C., and De Moraes, C. M. (2014). Plant dependence on rhizobia for nitrogen influences induced plant defenses and herbivore performance. Int. J. Mol. Sci. 15, 1466–1480. doi: 10.3390/ijms15011466
di Menna, M. E., Finch, S. C., Popay, A. J., and Smith, B. L. (2012). A review of the Neotyphodium lolii / Lolium perenne symbiosis and its associated effects on animal and plant health, with particular emphasis on ryegrass staggers. N. Z. Vet. J. 60, 315–328. doi: 10.1080/00480169.2012.697429
Dinsdale, D., Gordon, A. H., and George, S. (1979). Silica in the mesophyll cell walls of italian rye grass (Lolium multiflorum Lam. cv. RvP). Ann. Bot. 44, 73–77. doi: 10.1093/oxfordjournals.aob.a085708
Easton, H. S., Latch, G. C. M., Tapper, B. A., and Ball, O. J. P. (2002). Ryegrass host genetic control of concentrations of endophyte-derived alkaloids. Crop Sci. 42, 51–57. doi: 10.2135/cropsci2002.0051
Eneji, A. E., Inanaga, S., Muranaka, S., Li, J., Hattori, T., An, P., et al. (2008). Growth and nutrient use in four grasses under drought stress as mediated by silicon fertilizers. J. Plant Nutr. 31, 355–365. doi: 10.1080/01904160801894913
Epstein, E. (1994). The anomaly of silicon in plant biology. Proc. Natl. Acad. Sci. U.S.A. 91, 11–17. doi: 10.1073/pnas.91.1.11
Franco, M. F., Colabelli, M. N., Echeverría, M de M, and Ispizúa, V. N. (2020). Epichloë endophyte modifies the foliar anatomy of Lolium multiflorum Lam. Symbiosis 81, 313–319. doi: 10.1007/s13199-020-00702-y
Frew, A., Powell, J. R., Allsopp, P. G., Sallam, N., and Johnson, S. N. (2017). Arbuscular mycorrhizal fungi promote silicon accumulation in plant roots, reducing the impacts of root herbivory. Plant Soil 419, 423–433. doi: 10.1007/s11104-017-3357-z
Frew, A., Weston, L. A., Reynolds, O. L., and Gurr, G. M. (2018). The role of silicon in plant biology: a paradigm shift in research approach. Ann. Bot. 121, 1265–1273. doi: 10.1093/aob/mcy009
García Parisi, P. A., Lattanzi, F. A., Grimoldi, A. A., and Omacini, M. (2015). Multi-symbiotic systems: functional implications of the coexistence of grass-endophyte and legume-rhizobia symbioses. Oikos 124, 553–560. doi: 10.1111/oik.01540
Gundel, P. E., Pérez, L. I., Helander, M., and Saikkonen, K. (2013). Symbiotically modified organisms: nontoxic fungal endophytes in grasses. Trends Plant Sci. 18, 420–427. doi: 10.1016/j.tplants.2013.03.003
Hahn, H., Huth, W., Schöberlein, W., and Diepenbrock, W. (2003). Detection of endophytic fungi in Festuca spp. by means of tissue print immunoassay. Plant Breed. 122, 217–222. doi: 10.1046/j.1439-0523.2003.00855.x
Hall, C. R., Mikhael, M., Hartley, S. E., and Johnson, S. N. (2020). Elevated atmospheric CO2 suppresses jasmonate and silicon-based defences without affecting herbivores. Funct. Ecol. 34, 993–1003. doi: 10.1111/1365-2435.13549
Hall, C. R., Waterman, J. M., Vandegeer, R. K., Hartley, S. E., and Johnson, S. N. (2019). The role of silicon in antiherbivore phytohormonal signalling. Front. Plant Sci. 10:1132. doi: 10.3389/fpls.2019.01132
Hammer, E. C., Nasr, H., Pallon, J., Olsson, P. A., and Wallander, H. (2011). Elemental composition of arbuscular mycorrhizal fungi at high salinity. Mycorrhiza 21, 117–129. doi: 10.1007/s00572-010-0316-4
Hartley, S. E., Fitt, R. N., McLarnon, E. L., and Wade, R. N. (2015). Defending the leaf surface: intra- and inter-specific differences in silicon deposition in grasses in response to damage and silicon supply. Front. Plant Sci. 6:35. doi: 10.3389/fpls.2015.00035
Hartley, S. E., and Gange, A. C. (2009). Impacts of plant symbiotic fungi on insect herbivores: mutualism in a multitrophic context. Annu. Rev. Entomol. 54, 323–342. doi: 10.1146/annurev.ento.54.110807.090614
Heineck, G. C., Qiu, Y., Ehlke, N. J., and Watkins, E. (2020). The fungal endophyte Epichloë festucae var. lolii plays a limited role in mediating crown rust severity in perennial ryegrass. Crop Sci. 60, 1090–1104. doi: 10.1002/csc2.20095
Hiltpold, I., Demarta, L., Johnson, S. N., Moore, B. D., Power, S. A., and Mitchell, C. (2016). “Silicon and other essential element composition in roots using X-ray fluorescence spectroscopy: a high throughput approach. Invertebrate Ecology of Australasian Grasslands,” in Proceedings of the Ninth ACGIE (Hawkesbury, NSW), 191–196.
Huitu, O., Forbes, K. M., Helander, M., Julkunen-Tiitto, R., Lambin, X., Saikkonen, K., et al. (2014). Silicon, endophytes and secondary metabolites as grass defenses against mammalian herbivores. Front. Plant Sci. 5:478. doi: 10.3389/fpls.2014.00478
Hume, D. E., Card, S. D., and Rolston, M. P. (2013). “Effects of storage conditions on endophyte and seed viability in pasture grasses,” in Proceedings of the 22nd International Grassland Congress (Sydney), 405–408.
Johnson, S. N., Benefer, C. M., Frew, A., Griffiths, B. S., Hartley, S. E., Karley, A. J., et al. (2016). New frontiers in belowground ecology for plant protection from root-feeding insects. Appl. Soil. Ecol. 108, 96–107. doi: 10.1016/j.apsoil.2016.07.017
Jones, L. H. P., and Handreck, K. A. (1965). The relation between the silica content of the diet and the excretion of silica by sheep. J. Agric. Sci. 65, 129–134. doi: 10.1017/s0021859600085439
Jung, H., Yan, J., Zhai, Z., and Vatamaniuk, O. K. (2015). “Gene functional analysis using protoplast transient assays,” in Plant Functional Genomics: Methods and Protocols, 2th Edn, ed. E. Grotewold (Totowa, NJ: Humana Press), 1–526.
Jung, S. C., Martinez-Medina, A., Lopez-Raez, J. A., and Pozo, M. J. (2012). Mycorrhiza-induced resistance and priming of plant defenses. J. Chem. Ecol. 38, 651–664. doi: 10.1007/s10886-012-0134-6
Kauppinen, M., Saikkonen, K., Helander, M., Pirttilä, A. M., and Wäli, P. R. (2016). Epichloë grass endophytes in sustainable agriculture. Nat. Plants 2:15224.
Kaur, J., Ekanayake, P. N., Tian, P., van Zijll de Jong, E., Dobrowolski, M. P., Rochfort, S. J., et al. (2015). Discovery and characterisation of novel asexual Epichloë endophytes from perennial ryegrass (Lolium perenne L.). Crop Pasture Sci. 66, 1058–1070. doi: 10.1071/cp14332
Kim, Y. H., Khan, A. L., Waqas, M., Jeong, H. J., Kim, D. H., Shin, J. S., et al. (2014). Regulation of jasmonic acid biosynthesis by silicon application during physical injury to Oryza sativa L. J. Plant Res. 127, 525–532. doi: 10.1007/s10265-014-0641-3
Klotzbücher, T., Klotzbücher, A., Kaiser, K., Vetterlein, D., Jahn, R., and Mikutta, R. (2018). Variable silicon accumulation in plants affects terrestrial carbon cycling by controlling lignin synthesis. Glob. Chang. Biol. 24, e183–e189.
Krings, M., Taylor, T. N., Hass, H., Kerp, H., Dotzler, N., and Hermsen, E. J. (2007). Fungal endophytes in a 400-million-yr-old land plant: infection pathways, spatial distribution, and host responses. New Phytol. 174, 648–657. doi: 10.1111/j.1469-8137.2007.02008.x
Kuldau, G., and Bacon, C. (2008). Clavicipitaceous endophytes: their ability to enhance resistance of grasses to multiple stresses. Biol. Control 46, 57–71. doi: 10.1016/j.biocontrol.2008.01.023
Kumar, S., Soukup, M., and Elbaum, R. (2017). Silicification in grasses: variation between different cell types. Front. Plant Sci. 8:438. doi: 10.3389/fpls.2017.00438
Latch, G. C. M., and Christensen, M. J. (1985). Artificial infection of grasses with endophytes. Ann. Appl. Biol. 107, 17–24. doi: 10.1111/j.1744-7348.1985.tb01543.x
Leuchtmann, A. (1992). Systematics, distribution, and host specificity of grass endophytes. Nat. Toxins 1, 150–162. doi: 10.1002/nt.2620010303
Llorens, E., Sharon, O., Camañes, G., García-Agustín, P., and Sharon, A. (2019). Endophytes from wild cereals protect wheat plants from drought by alteration of physiological responses of the plants to water stress. Environ. Microbiol. 21, 3299–3312. doi: 10.1111/1462-2920.14530
Ma, J. F., and Yamaji, N. (2006). Silicon uptake and accumulation in higher plants. Trends Plant Sci. 11, 392–397. doi: 10.1016/j.tplants.2006.06.007
Ma, J. F., and Yamaji, N. (2015). A cooperative system of silicon transport in plants. Trends Plant Sci. 20, 435–442. doi: 10.1016/j.tplants.2015.04.007
Malinowski, D. P., Alloush, G. A., and Belesky, D. P. (1998). Evidence for chemical changes on the root surface of fall fescue in response to infection with the fungal endophyte Neotyphodium coenophialum. Plant Soil 205, 1–12.
Malinowski, D. P., and Belesky, D. P. (2000). Adaptations of endophyte-infected cool-season grasses to environmental stresses: mechanisms of drought and mineral stress tolerance. Crop Sci. 40, 923–940. doi: 10.2135/cropsci2000.404923x
Malinowski, D. P., Zuo, H., Belesky, D. P., and Alloush, G. A. (2004). Evidence for copper binding by extracellular root exudates of tall fescue but not perennial ryegrass infected with Neotyphodium spp. endophytes. Plant Soil 267, 1–12. doi: 10.1007/s11104-005-2575-y
Martinez-Medina, A., Flors, V., Heil, M., Mauch-Mani, B., Pieterse, C. M. J., Pozo, M. J., et al. (2016). Recognizing plant defense priming. Trends Plant Sci. 21, 818–822. doi: 10.1016/j.tplants.2016.07.009
Massey, F., Ennos, A., and Hartley, S. (2006). Silica in grasses as a defence against insect herbivores: contrasting effects on folivores and a phloem feeder. J. Anim. Ecol. 75, 595–603. doi: 10.1111/j.1365-2656.2006.01082.x
Massey, F., and Hartley, S. (2009). Physical defences wear you down : progressive and irreversible impacts of silica on insect herbivores. J. Anim. Ecol. 78, 281–291. doi: 10.1111/j.1365-2656.2008.01472.x
Massey, F. P., Ennos, A. R., and Hartley, S. E. (2007). Grasses and the resource availability hypothesis: the importance of silica-based defences. J. Ecol. 95, 414–424. doi: 10.1111/j.1365-2745.2007.01223.x
Massey, F. P., and Hartley, S. E. (2006). Experimental demonstration of the antiherbivore effects of silica in grasses: impacts on foliage digestibility and vole growth rates. Proc. R. Soc. B Biol. Sci. 273, 2299–2304. doi: 10.1098/rspb.2006.3586
Melzer, S. E., Knapp, A. K., Kirkman, K. P., Smith, M. D., Blair, J. M., and Kelly, E. F. (2010). Fire and grazing impacts on silica production and storage in grass dominated ecosystems. Biogeochemistry 97, 263–278. doi: 10.1007/s10533-009-9371-3
Miao, B. H., Han, X. G., and Zhang, W. H. (2010). The ameliorative effect of silicon on soybean seedlings grown in potassium-deficient medium. Ann. Bot. 105, 967–973. doi: 10.1093/aob/mcq063
Miles, C. O., Di Menna, M. E., Jacobs, S. W. L., Garthwaite, I., Lane, G. A., Prestidge, R. A., et al. (1998). Endophytic fungi in indigenous Australasian grasses associated with toxicity to livestock. Appl. Environ. Microbiol. 64, 601–606. doi: 10.1128/aem.64.2.601-606.1998
Mirzahossini, Z., Shabani, L., Sabzalian, M. R., and Sharifi-Tehrani, M. (2015). ABC transporter and metallothionein expression affected by NI and Epichloe endophyte Infection in tall fescue. Ecotoxicol. Environ. Saf. 120, 13–19. doi: 10.1016/j.ecoenv.2015.05.025
Moore, D. (1984). The role of silica in protecting Italian ryegrass (Lolium multiflorum) from attack by dipterous stem boring larvae (Oscinella frit and other related species). Ann. Appl. Biol. 104, 161–166. doi: 10.1111/j.1744-7348.1984.tb05598.x
Munday, J. S., Finch, S. C., Vlaming, J. B., Sutherland, B. L., and Fletcher, L. R. (2017). Pathological changes seen in horses in New Zealand grazing Mediterranean tall fescue (Lolium arundinaceum) infected with selected endophytes (Epichloë coenophiala) causing equine fescue oedema. N. Z. Vet. J. 65, 147–151. doi: 10.1080/00480169.2016.1248516
Niones, J. T., and Takemoto, D. (2014). An isolate of Epichloë festucae, an endophytic fungus of temperate grasses, has growth inhibitory activity against selected grass pathogens. J. Gen. Plant Pathol. 80, 337–347. doi: 10.1007/s10327-014-0521-7
Novas, M. V., Iannone, L. J., Godeas, A. M., and Scervino, J. M. (2011). Evidence for leaf endophyte regulation of root symbionts: effect of Neotyphodium endophytes on the pre-infective state of mycorrhizal fungi. Symbiosis 55, 19–28. doi: 10.1007/s13199-011-0140-4
O’Reagain, P. J., and Mentis, M. T. (1989). Leaf silicification in grasses–a review. J. Grassl. Soc. South Africa 6, 37–43. doi: 10.1080/02566702.1989.9648158
Perez, L. I., Gundel, P. E., Marrero, H. J., Arzac, A. G., and Omacini, M. (2017). Symbiosis with systemic fungal endophytes promotes host escape from vector-borne disease. Oecologia 184, 237–245. doi: 10.1007/s00442-017-3850-3
R Core Team (2020). R: A Language and Environment for Statistical Computing. Vienna: R Foundation for Statistical Computing. Available online at: https://www.R-project.org/
Rasmussen, S., Liu, Q., Parsons, A., Xue, H., Sinclair, B., and Newman, J. (2012). Grass–endophyte interactions : a note on the role of monosaccharide transport in the Neotyphodium lolii–Lolium perenne symbiosis. New Phytol. 196, 7–12. doi: 10.1111/j.1469-8137.2012.04250.x
Rasoolizadeh, A., Labbé, C., Sonah, H., Deshmukh, R. K., Belzile, F., Menzies, J. G., et al. (2018). Silicon protects soybean plants against Phytophthora sojae by interfering with effector-receptor expression. BMC Plant Biol. 18:97. doi: 10.1186/s12870-018-1312-7
Raven, J. A. (1983). The transport and function of silicon in plants. Biol. Rev. 58, 179–207. doi: 10.1111/j.1469-185x.1983.tb00385.x
Reidinger, S., Ramsey, M. H., and Hartley, S. H. (2012). Rapid and accurate analyses of silicon and phosphorus in plants using a portable X-ray fluorescence spectrometers. New Phytol. 195, 699–706. doi: 10.1111/j.1469-8137.2012.04179.x
Ren, A. Z., Gao, Y. B., and Zhou, F. (2007). Response of Neotyphodium lolii-infected perennial ryegrass to phosphorus deficiency. Plant Soil Environ. 53, 113–119. doi: 10.17221/2225-pse
Reynolds, O. L., Padula, M. P., Zeng, R., and Gurr, G. M. (2016). Silicon: potential to promote direct and indirect effects on plant defense against arthropod pests in agriculture. Front. Plant Sci. 7:744. doi: 10.3389/fpls.2016.00744
Rho, H., Hsieh, M., Kandel, S. L., Cantillo, J., Doty, S. L., and Kim, S.-H. (2018). Do endophytes promote growth of host plants under stress? A Meta-Analysis on plant stress mitigation by endophytes. Microb. Ecol. 75, 407–418. doi: 10.1007/s00248-017-1054-3
Rozpa̧dek, P., Wêżowicz, K., Nosek, M., Ważny, R., Tokarz, K., Lembicz, M., et al. (2015). The fungal endophyte Epichloë typhina improves photosynthesis efficiency of its host orchard grass (Dactylis glomerata). Planta 242, 1025–1035. doi: 10.1007/s00425-015-2337-x
Saikkonen, K., Young, C. A., Helander, M., and Schardl, C. L. (2016). Endophytic Epichloë species and their grass hosts: from evolution to applications. Plant Mol. Biol. 90, 665–675. doi: 10.1007/s11103-015-0399-6
Soto-Barajas, M. C., Zabalgogeazcoa, I., Gómez-Fuertes, J., González-Blanco, V., and Vázquez-de-Aldana, B. R. (2016). Epichloë endophytes affect the nutrient and fiber content of Lolium perenne regardless of plant genotype. Plant Soil 405, 265–277. doi: 10.1007/s11104-015-2617-z
Tripathi, D. K., Singh, S., Singh, V. P., Prasad, S. M., Dubey, N. K., and Chauhan, D. K. (2017). Silicon nanoparticles more effectively alleviated UV-B stress than silicon in wheat (Triticum aestivum) seedlings. Plant Physiol. Biochem. 110, 70–81. doi: 10.1016/j.plaphy.2016.06.026
Van Soest, P. J., and Jones, L. H. P. (1968). Effect of silica in forages upon digestibility. J. Dairy Sci. 51, 1644–1648. doi: 10.3168/jds.s0022-0302(68)87246-7
Vandegeer, R. K., Zhao, C., Cibils-Stewart, X., Wuhrer, R., Hall, C. R., Hartley, S. E., et al. (2020). Silicon deposition on guard cells increases stomatal sensitivity as mediated by K+ efflux and consequently reduces stomatal conductance. Physiol. Plant. doi: 10.1111/ppl.13202
Vandevenne, F. I., Barão, A. L., Schoelynck, J., Smis, A., Ryken, N., Van Damme, S., et al. (2013). Grazers: biocatalysts of terrestrial silica cycling. Proc. R. Soc. B Biol. Sci. 280.
Vivancos, J., Labbé, C., Menzies, J. G., and Bélanger, R. R. (2015). Silicon-mediated resistance of Arabidopsis against powdery mildew involves mechanisms other than the salicylic acid (SA)-dependent defence pathway. Mol. Plant Pathol. 16, 572–582. doi: 10.1111/mpp.12213
Wiewióra, B., Zurek, G., and Zurek, M. (2015). Endophyte-mediated disease resistance in wild populations of perennial ryegrass (Lolium perenne). Fungal Ecol. 15, 1–8. doi: 10.1016/j.funeco.2015.01.004
Xia, C., Li, N., Zhang, Y., Li, C., Zhang, X., and Nan, Z. (2018). Role of Epichloë endophytes in defense responses of cool-season grasses to pathogens: a Review. Plant Dis. 102, 2061–2073. doi: 10.1094/pdis-05-18-0762-fe
Ye, M., Zeng, R., Cai, K., Long, J., Zhu-Salzman, K., Wang, R., et al. (2013). Priming of jasmonate-mediated antiherbivore defense responses in rice by silicon. Proc. Natl. Acad. Sci. U.S.A. 110, E3631–E3639.
Young, C. A., Charlton, N. D., Takach, J. E., Swoboda, G. A., Trammell, M. A., Huhman, D. V., et al. (2014). Characterization of Epichloë coenophiala within the US: are all tall fescue endophytes created equal? Front. Chem. 2:95. doi: 10.3389/fchem.2014.00095
Young, C. A., Hume, D. E., and McCulley, R. L. (2013). Forages and pastures symposium: fungal endophytes of tall fescue and perennial ryegrass: pasture friend or foe? J. Anim. Sci. 91, 2379–2394. doi: 10.2527/jas.2012-5951
Keywords: silica, Epichloë, hydroponics, perennial ryegrass (Lolium perenne L.), tall fescue (Festuca arundinacea S.)
Citation: Cibils-Stewart X, Powell JR, Popay AJ, Lattanzi FA, Hartley SE and Johnson SN (2020) Reciprocal Effects of Silicon Supply and Endophytes on Silicon Accumulation and Epichloë Colonization in Grasses. Front. Plant Sci. 11:593198. doi: 10.3389/fpls.2020.593198
Received: 10 August 2020; Accepted: 07 October 2020;
Published: 27 October 2020.
Edited by:
Pedro Emilio Gundel, University of Buenos Aires, ArgentinaReviewed by:
Chunjie Li, Lanzhou University, ChinaKari Saikkonen, University of Turku, Finland
Benjamin Fuchs, University of Turku, Finland
Copyright © 2020 Cibils-Stewart, Powell, Popay, Lattanzi, Hartley and Johnson. This is an open-access article distributed under the terms of the Creative Commons Attribution License (CC BY). The use, distribution or reproduction in other forums is permitted, provided the original author(s) and the copyright owner(s) are credited and that the original publication in this journal is cited, in accordance with accepted academic practice. No use, distribution or reproduction is permitted which does not comply with these terms.
*Correspondence: Ximena Cibils-Stewart, xcibils@inia.org.uy