- 1Institute of Dendrology, Polish Academy of Sciences, Kórnik, Poland
- 2Department of Microbiology, Faculty of Biological and Veterinary Sciences, Nicolaus Copernicus University, Toruń, Poland
- 3Department of Game Management and Forest Protection, Faculty of Forestry and Wood Technology, Poznań University of Life Sciences, Poznań, Poland
- 4Tianjin Institute of Industrial Biotechnology, University of Chinese Academy of Sciences, Tianjin, China
- 5Institute of Microbiology, University of Chinese Academy of Sciences, Beijing, China
- 6Department of Forest Entomology and Pathology, Faculty of Forestry and Wood Technology, Poznań University of Life Sciences, Poznań, Poland
Understanding belowground plant-microbial interactions is important for biodiversity maintenance, community assembly and ecosystem functioning of forest ecosystems. Consequently, a large number of studies were conducted on root and microbial interactions, especially in the context of precipitation and temperature gradients under global climate change scenarios. Forests ecosystems have high biodiversity of plants and associated microbes, and contribute to major primary productivity of terrestrial ecosystems. However, the impact of root metabolites/exudates and root traits on soil microbial functional groups along these climate gradients is poorly described in these forest ecosystems. The plant root system exhibits differentiated exudation profiles and considerable trait plasticity in terms of root morphological/phenotypic traits, which can cause shifts in microbial abundance and diversity. The root metabolites composed of primary and secondary metabolites and volatile organic compounds that have diverse roles in appealing to and preventing distinct microbial strains, thus benefit plant fitness and growth, and tolerance to abiotic stresses such as drought. Climatic factors significantly alter the quantity and quality of metabolites that forest trees secrete into the soil. Thus, the heterogeneities in the rhizosphere due to different climate drivers generate ecological niches for various microbial assemblages to foster beneficial rhizospheric interactions in the forest ecosystems. However, the root exudations and microbial diversity in forest trees vary across different soil layers due to alterations in root system architecture, soil moisture, temperature, and nutrient stoichiometry. Changes in root system architecture or traits, e.g. root tissue density (RTD), specific root length (SRL), and specific root area (SRA), impact the root exudation profile and amount released into the soil and thus influence the abundance and diversity of different functional guilds of microbes. Here, we review the current knowledge about root morphological and functional (root exudation) trait changes that affect microbial interactions along drought and temperature gradients. This review aims to clarify how forest trees adapt to challenging environments by leveraging their root traits to interact beneficially with microbes. Understanding these strategies is vital for comprehending plant adaptation under global climate change, with significant implications for future research in plant biodiversity conservation, particularly within forest ecosystems.
1 Introduction
Forest ecosystems, which cover one-third of the world’s land area and encompass more than 24.6 million square kilometers, are crucial for global ecosystem services and carbon cycling, particularly under the influence of climate change (Viet et al., 2022). These ecosystems, predominantly temperate and boreal forests, consist of diverse deciduous and coniferous trees that are well-adapted to a broad range of environmental conditions, especially in terms of temperature and precipitation (Tasenkevich et al., 2023). The soils of these forests support a rich array of prokaryotic and eukaryotic microbial communities, including bacteria and fungi. These organisms are essential for maintaining ecosystem health and are key players in ecosystem carbon and nutrient cycling (Bardgett et al., 2014a; Baldrian, 2017). Some microbes are particularly adept at decomposing refractory soil organic matter (SOM) and cellulose, a capability linked to their filamentous growth and ability to excrete extracellular enzymes (Bodeker et al., 2014; Gómez et al., 2020). Furthermore, these forest soils are home to diverse communities of saprotrophic and mycorrhizal fungi, integral to the ecosystem’s functioning and heavily reliant on the interactions within the belowground rhizosphere (Voříšková et al., 2014). Understanding these complex microbial interactions and the dynamics of below-ground carbon allocation via root exudation is vital for predicting carbon balance in terrestrial ecosystems, especially in the face of ongoing global changes (DeAngelis et al., 2015).
Fine roots of forest trees, highly active metabolic zones within the soil, are key in the interactions with subterranean microorganisms (Figure 1) (King et al., 2023). The communication between plants and soil microbes is predominantly mediated through chemical signals, as indicated by Pascale et al. (2020) and Williams and de Vries (2020). Root systems of forest trees excrete various chemical compounds, collectively known as metabolites, which are essential for diverse biological processes (Figure 1). These metabolites encompass primary metabolites such as amino acids, organic acids, and sugars, along with secondary metabolites like phenolic chemicals, and volatile compounds including terpenoids and sulfides. Such root exudations of forest trees have a significant impact on numerous ecosystem functions. For example, they affect soil microbial dynamics (Jing et al., 2023), enhance plant resilience to non-living stress factors (Williams and de Vries, 2020; Jiang et al., 2023), and contribute to nutrient cycling and the stability of soil structure (Mommer et al., 2016; Wang et al., 2021a; Wang et al., 2021b). Additionally, roots release various other metabolites, accounting for 10–50% of the carbon fixed by plants (Nguyen, 2003; Massalha et al., 2017; Prescott and Grayston, 2023), thereby playing an integral role in the global carbon cycle.
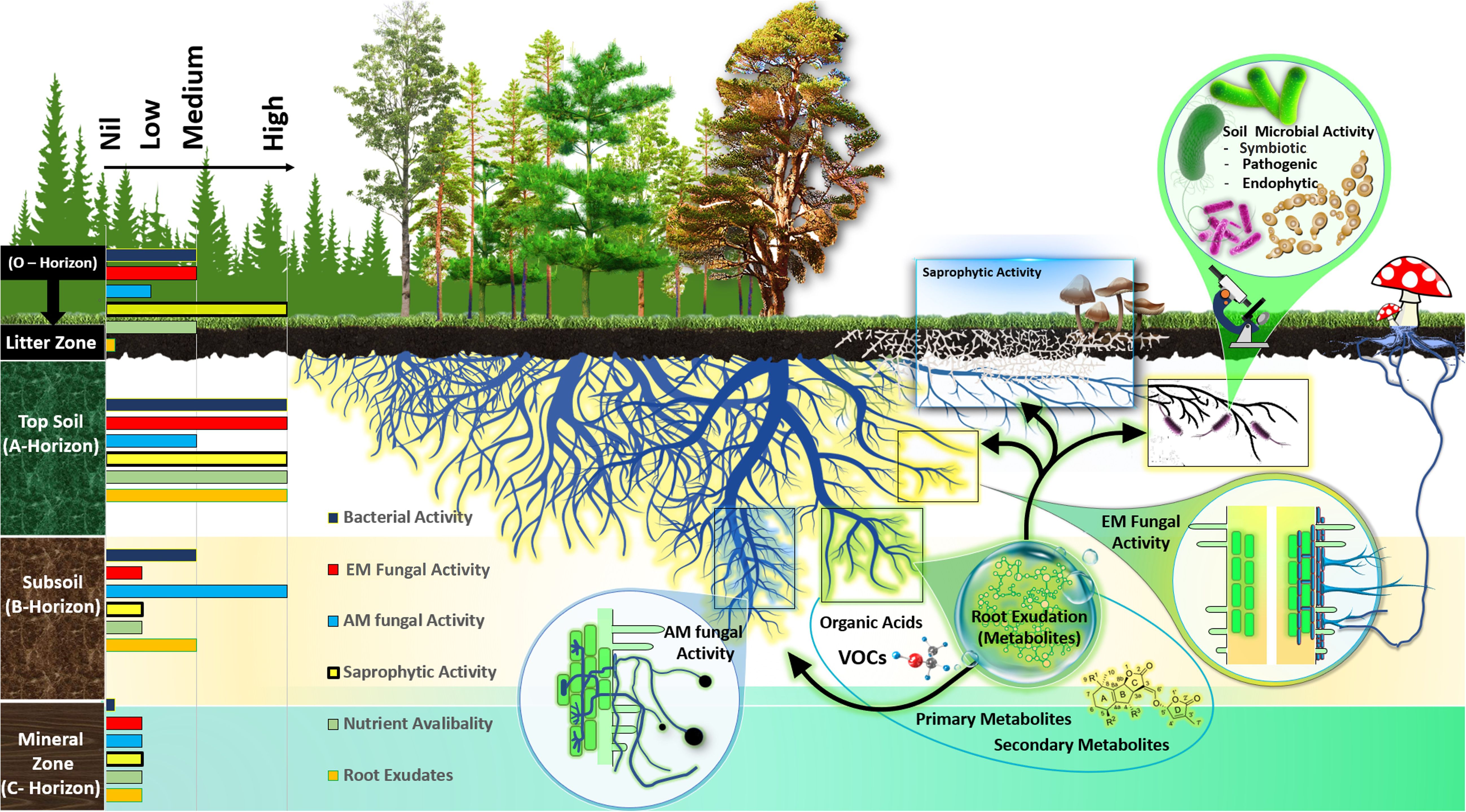
Figure 1 General overview of the metabolic niche of the rhizosphere microbial functional group in forest ecosystems. Different soil horizons differ in the activity of ectomycorrhizal fungi (ECM), arbuscular mycorrhizal fungi (AM), saprotrophic fungi, and bacterial communities as well as exudation of root metabolites and nutrient availability, demonstrated by currently available research.
Microbial diversity and abundance in the rhizosphere are affected by plant developmental stage, genotypes, and soil parameters (Ofek-Lalzar et al., 2014; Schlaeppi et al., 2014; Bulgarelli et al., 2015; Paredes and Lebeis, 2016). For instance, the main soil parameters, e.g. changing soil organic matter distribution with soil depth, influence root exudation as well as microbial diversity and functioning in temperate forest ecosystems (Tückmantel et al., 2017; Prada-Salcedo et al., 2022) (Figure 1). However, this assemblage of microbes in different soil horizons may change due to the changes in root morphology and exudation of forest trees along a wide range of environmental gradients, for example drought or temperature (Meier et al., 2020; Veach et al., 2020). It has even been hypothesized that plants dynamically recruit soil microbes by secreting metabolites in the rhizosphere that ideally stimulate rhizosphere microorganisms or endophytic fungi and bacteria that are advantageous to plant growth by helping them cope with abiotic stresses (Sasse et al., 2018; Hildebrand et al., 2023). Knowledge about the responses of root metabolites and microbial assemblages in the roots and rhizosphere in different horizons of forest soil along environmental gradients of drought and temperature will increase our aptitude to forecast the global change effects on soil organic matter decomposition and C cycling (Steidinger et al., 2019). Therefore, a better understanding of rhizosphere microbial interactions and below ground C allocation via root exudation is important for predicting C balance in the terrestrial ecosystem under global change scenarios.
In this review, we address the existing knowledge about the metabolic niche of rhizosphere microbes and questions which were previously unanswered. How microbial activity differ in different soil horizon of forest ecosystems? How do root traits and exudation change within soil profiles? What are the impacts of different metabolites on microbial functional groups? How is metabolite change linked to root traits to influence microbial functional groups in forest soil? And finally, how do climate factors influence root traits, root exudation and microbiome dynamics in forest ecosystem? The goal of this review is to answer these questions for forest ecosystems to summarize the current information about the interactions among root traits, rhizosphere metabolites and microbial communities, and about their response to climate change, which have particular implications for future research investigations into the microbial ecology of forest soils.
2 Microbial activity differs in different soil horizons
Soil horizons of forest areas are distinguished by their unique soil properties, exhibiting varied physical, chemical, and microbiological characteristics (Rumpel and Kögel-Knabner, 2011; Horodecki and Jagodziński, 2017; Horodecki et al., 2019). The O horizon, known as the litter zone, is rich in organic materials and darker in color, comprising decomposing plant materials like leaves, needles, and twigs (Horodecki and Jagodziński, 2017). The A horizon, or topsoil, contains a mix of decomposed plant matter and soil minerals. The C horizon, or mineral horizon, is marked by lower organic matter content, a result of organic matter decomposition (Voříšková et al., 2014; Hartemink et al., 2020). The B horizon represents a blend of mineral zone soil and subsoil components (Figure 1). The distribution of soil microbial communities in forest ecosystem varies across these horizons due to differing levels of organic matter decomposition (Jiao et al., 2018; Mundra et al., 2021; Luo et al., 2023). High-throughput sequencing has shown distinct fungal community clusters in different soil horizons (McGuire et al., 2013b). For example, in tropical forests, the O-horizon is predominantly inhabited by saprotrophic fungi, while the A-horizon below it is mainly occupied by ectomycorrhizal fungi (McGuire et al., 2013a). Furthermore, the horizontal separation of ectomycorrhizal and saprotrophic fungi in forest soil layers, reflecting their specific functional roles in decomposing organic matters at various stages of decay (Dickie et al., 2002; O'Brien et al., 2005; Lindahl et al., 2007; McGuire et al., 2013a; Lustenhouwer et al., 2020). For instances, in tropical, temperate and boreal forests reveal that ectomycorrhizal fungi are more abundant in the lower litter zone, while saprotrophs are prevalent in the upper layers (McGuire et al., 2013a; Carteron et al., 2021). This stratification is due to the ability of some ectomycorrhizal fungi to decompose organic matter for nitrogen, while they primarily source carbon from their host plants. On the other hand, saprotrophic fungi derive their carbon from decomposing organic matter and are predominantly found in shallower soil layers that are abundant in carbon-rich substrates (Lindahl et al., 2007; Carteron et al., 2021; Marañón-Jiménez et al., 2021). In pine forests, it has been observed that the overall fungal biomass is considerably more abundant in the litter and humus layers compared to the deeper mineral zone (Adamo et al., 2022). However, in oak forests, the dominant ectomycorrhizal fungi Cortinarius and Sebacina are more prevalent in the A horizon, while saprotrophic fungi like the genus Preussia dominate the litter layer (Adamo et al., 2022). Furthermore, in Beech and Oak forest ecosystems, the ectomycorrhizal genera Amanita, Melanogaster, Odontia, Sistotrema, and Telephora exhibited higher abundances in the topsoil compared to the subsoil (Frey et al., 2021). Moreover, in temperate forests, ectomycorrhizal fungi from the Boletales order were enriched in the organic layer (litter zone), while Russulales and Cantharellales were predominant in the topsoil (A-horizon) (Khokon et al., 2021). This pattern is attributed to the saprotrophic nature of Boletales, which are predominant in the organic layer, and the symbiotic nature of Russulales in the mineral soil, indicating territorial behavior among members of the these order, as indicated by Pena et al. (2017).
A prevailing model about the spatial distribution of mycorrhizal types proposes that, in environments where they coexist, ectomycorrhizal (EcM) fungi are typically more abundant in organic soil layers and in topsoil, whereas arbuscular mycorrhizal (AM) fungi are more commonly found in mineral-rich sub soil horizons (Smith and Read, 2008). For instance, in soil ecosystems, the dominance of ectomycorrhizal fungi is more pronounced near the surface, while arbuscular mycorrhizal (AM) fungi are more abundant in deeper layers, showing a decrease in ectomycorrhizal compared to AM colonization as soil depth increases in northern hardwood forests (Nash et al., 2022). AM fungi’s enhanced ability to absorb phosphorus (P), especially inorganic P (Shen et al., 2020), compared to nitrogen (N), and the proficiency of ectomycorrhizal fungi in acquiring N (Bicharanloo et al., 2021), suggest that each group thrives in soil layers where their preferred nutrient is scarce (Read, 1991). This perspective is reinforced by research that highlights varying root colonization patterns in temperate and rain forest ecosystems where different types of mycorrhizae coexist (Moyersoen et al., 1998) and the distinct acquisition strategies (Phillips et al., 2013). This distribution pattern is exemplified in case of Populus tremuloides growing in boreal forests, where roots in the upper soil are predominantly colonized by ectomycorrhizal fungi, whereas deeper roots are mainly colonized by AM fungi (Neville et al., 2002). Moreover, the competitive dynamics between ectomycorrhizal and saprotrophic fungi influence their soil depth distribution. For instances, in temperate and boreal forests, competition for soil resources has been suggested to confine saprotrophic fungi primarily to the uppermost organic soil layers in areas dominated by ectomycorrhizal trees. Conversely, in AM fungi-dominated forests, a lower overlap in ecological niches allows saprotrophic fungi to extend their dominance to deeper soil layers (Carteron et al., 2021).
The structure of bacterial communities in soil, including the composition and abundance of different taxa, changes with soil depth, tending to be more diverse in the topsoil. This diversity and abundance is correlated with the soil’s physical properties and the availability of resources across soil horizons in different forest ecosystems (Eilers et al., 2012; Chu et al., 2016; Shao et al., 2019). These shifts in community structure are marked by changes in the relative abundance of dominant bacterial groups. For example, in a temperate forest ecosystem, there are notably high populations of Actinobacteria, Bacteroidetes, and Proteobacteria in topsoil areas that have a high concentration of soil organic matter (SOM) (Shao et al., 2019). This distribution pattern is similar to what is found in grassland and agricultural soils and is linked to variations in soil nutrients or SOM levels (Will et al., 2010; Sagova-Mareckova et al., 2016). Our analysis of the above literature about the vertical distribution of microbial communities allowed us to classify abundance and activity of bacterial and fungal communities as high, medium, and low across the forest soil horizons. This analysis is summarized in the left section of Figure 1. However, studies focusing on the characterization of bacterial functional group across different forest soil horizons, particularly mutualistic group, have been comparatively limited.
3 Impact of different metabolites on microbial functional groups
Recent research has increasingly highlighted the crucial role that root exudates play in the interactions between forest trees and microbes in the rhizosphere (Sasse et al., 2018; Pascale et al., 2020; Williams and de Vries, 2020; Oppenheimer-Shaanan et al., 2022; Jing et al., 2023; Staszel-Szlachta et al., 2024). In this context, it is well understood that plants supply photosynthetic carbon to rhizospheric microbes and release various key molecules (Figure 2) into the soil, thereby fostering a bidirectional relationship. In this interaction, microbes also contribute to plant growth by aiding in nutrient acquisition and enhancing tolerance to abiotic and biotic stress. These rhizosphere metabolites play diverse roles influencing various fungal and bacterial functional groups as well as their functions in belowground ecosystems as summarized below (Figure 2).
3.1 Root metabolites influence on fungal functional groups
Root metabolites in the rhizosphere soil have a wide range of potential for attracting and repelling distinct fungal strains and hence affect the rhizosphere fungal population (Chagas et al., 2018). Plants influence the enrolment of mycorrhizal fungi in the rhizosphere through the release of specific chemical signals into the soil (Korenblum et al., 2022). Studies have also shown that differences in rhizosphere communities between different tree species are the result of selective attraction of specific fungi through plant root secretions (Prescott and Grayston, 2013; Jing et al., 2023). For instances, ectomycorrhizal associations of forest trees typically lead to an increase in the exudation of organic acids, or a change in the types of organic acids released by roots (Johansson et al., 2008, 2009; Jourand et al., 2014; Abdulsalam et al., 2021). Furthermore, van Hees et al. (2006) studied Hebeloma ectomycorrhizal species in symbiosis with Pinus sylvestris (Scots pine) and discovered that these mycorrhizal associations led to the secretion of specific compounds. These included oxalate and ferricrocin, along with trace amounts of malonate and acetate, which were distinctly present in soils around mycorrhizal Scots pine but absent in soils surrounding non-mycorrhizal Scots pine. Similarly, a study on oak roots colonized by the ECM fungus Tuber indicum showed an accumulation of carbohydrates and organic acids in the ectomycorrhizal root tips (Li et al., 2018). Additionally, carbon produced by trees in the form of root exudates is rapidly transferred to mycorrhizal partners via mycelial networks in the soil (Kaiser et al., 2015; Gorzelak et al., 2020; Hawkins et al., 2023). Moreover, it’s hypothesized that fungal hyphae release labile carbon, such as carbohydrates and amino acids, into the soil away from the roots, potentially enhancing the decomposition of organic matter by free-living soil saprotrophs (Frey, 2019). While the knowledge of the specific functions of the specific root metabolites in relation to fungal functional groups in forest ecosystems is limited, the information presented below is based on studies conducted in vitro and in agricultural ecosystems have demonstrated their distinct roles. For instance, certain root exudates, such as strigolactone, have been found to trigger the activation of AM fungal genes related to the initiation of hyphal growth near plant roots (Martin et al., 2008; Clark and Bennett, 2023). Strigolactones enhance the hyphal branching of AM fungi, which is a crucial step in the colonization process of plant roots. This not only aids in the establishment of the symbiosis but also in the fungal acquisition of nutrients, particularly phosphorus, from the soil, which are then transferred to the plant, enhancing its nutrient uptake and stress tolerance. Studies by Akiyama and Hayashi (2006), for example, have demonstrated that the application of synthetic strigolactones can significantly increase the colonization of plant roots by AM fungi, underlining the direct impact of these metabolites on mycorrhizal fungi. Furthermore, symbiotic relationships between non-legumes and AM fungi involve root exudate compounds such as strigolactone 5-deoxystrigol (Yoneyama, 2019), sugars (Fang and St. Leger, 2010), and carbohydrates (Kiers et al., 2011). Moreover, plants that effectively battle root fungal pathogen invasion frequently produce unique volatile organic compounds that perform direct defensive functions (Delory et al., 2016). Plant roots secrete triterpenoids, which are among the most powerful antifungal defensive chemicals (Zhong et al., 2022). More precisely, saponins, glycosylated triterpenoids released by cereals, denote well recognized constitutive defense substances against fungal pathogens, as has been proved for the antifungal properties of avenacin secreted by oat roots (Osbourn, 2003). These studies reveal how different root metabolites can selectively influence fungal groups, offering insights that might be applicable to forest ecosystems. However, research specifically for forest trees in this area is still limited or yet to be described. Therefore, it remains to be verified whether these findings are compatible with forest ecosystems.
3.2 Root metabolites influence bacterial functional groups
The dynamic profile of metabolites at the root-soil interface correlates with microbial substrate preferences, which can often be predicted from genomic sequences. Different populations of forest trees secrete a variety of metabolites that have unique effects on the composition of bacterial communities. For instance, untargeted metabolite studies of Pinus tabulaeformis plants have shown that functional carbon metabolites, such as hippuric acid, cytidine-5´-monophosphate, lactic acid, glucose, and spermidine, influence the microbial populations in rhizosphere soil, including Actinobacteria, Acidobacteria, Proteobacteria under nitrogen addition in a subtropical forest ecosystems (Jing et al., 2023). Furthermore, previous investigation demonstrated that addition of sugars from pine root exudates to soil influences the abundance and activity of various bacterial taxa, like Proteobacteria, Actinobacteria, and Firmicutes (Shi et al., 2011). Moreover, Pinus sylvestris releases a variety of rhizosphere metabolites, such as fatty acids, monoacylglycerides, and diterpenes, in a temperate forest; these metabolites significantly affect the diversity and composition of rhizosphere bacterial communities (Bi et al., 2022). Moreover, sugars and organic acid released as root exudates influenced the diversity and community assembly of free-living N2 fixing diazotrophic communities in forest soil (Ding et al., 2021). For examples, in a desert forest ecosystem, the presence of key diazotroph species like Azotobacter, Azospirillum, Bradyrhizobium, and Mesorhizobium is significantly correlated with the levels of various sugars and organic acid exudates (Ding et al., 2021). However, research into how root exudates affect the soil bacteria and their functional groups (decomposers, mutualists, pathogens, and lithotrophs) is less extensive in forest ecosystems. Many studies conducted in vitro with model plants and in agricultural settings, as detailed in the information presented below, have demonstrated the specific roles of metabolites in fostering associations with certain bacterial functional groups. For instance, the plant growth-promoting bacteria Bacillus subtilis was recruited to the rhizosphere through malic acid in root exudates, particularly after infection by foliar pathogens (Rudrappa et al., 2008; Rekha et al., 2018). Recent findings also indicate that coumarins, a subset of phenolic compounds, display variable toxicity levels against pathogenic bacteria (Stringlis et al., 2018, 2019; Voges et al., 2019). Furthermore, flavonoids and strigolactones, are two additional naturally occurring phenolic metabolites that act as signaling molecules in the formation of well-studied mutualistic associations of plant hosts with Rhizobium bacteria (Subramanian et al., 2007; Dong and Song, 2020). Flavonoids have been linked to the control of nodulation genes expression in nitrogen-fixing rhizobia (Cesco et al., 2010; Hassan and Mathesius, 2012) as well as Pseudomonas aeruginosa quorum-sensing (QS) signals (Vandeputte et al., 2011). Flavonoids secreted by plant roots are detected by Rhizobium, which in turn produces Nod factors that stimulate root hair curling and nodule formation, leading to the establishment of a symbiotic relationship. This interaction significantly enhances the plant’s ability to fix atmospheric nitrogen. Empirical studies, such as that by Subramanian et al. (2007), have demonstrated that flavonoid-deficient mutants of legumes show reduced nodulation and symbiotic nitrogen fixation, highlighting the direct influence of these metabolites on nitrogen-fixing bacteria. Beyond phenolics, some other root secondary metabolites involved in the balance between roots and bacterial populations have been demonstrated, including benzoxazinoids (Hu et al., 2018; Cotton et al., 2019), triterpenes and camalexin (Huang et al., 2019). In addition, plants also release proteins as root exudates (De-la-Peña et al., 2010). In this context, lectins are among the most investigated proteins which act as defenses against pathogens and recognition factors in symbiotic associations (De Hoff et al., 2009; Rocha et al., 2015; Bellande et al., 2017). However, it’s important to note that while this evidence is compelling in controlled environments and agricultural settings, direct and specific knowledge about the functions of these metabolites towards bacterial functional groups in forest ecosystems is not as extensive. Therefore, while these findings offer valuable perspectives, caution should be exercised in directly applying these insights to forest ecosystems, and there is a need for more focused research in these natural environments to understand the roles of root metabolites and their associations with bacterial functional groups.
4 Root morphological traits influence root exudation
Environmental changes lead to a variety of phenotypic and metabolic alterations in forest tree roots, which in turn impact the profile of soil metabolites (Karst et al., 2017; Gargallo-Garriga et al., 2018; Hartman and Tringe, 2019). In previous investigations, the root morphological traits that influence rhizosphere metabolites in temperate, boreal and subtropical forest ecosystems were different root categories based on root diameter (i.e. fine, medium and large roots), biomass of absorptive and transportive roots and different fine root traits i.e. root tissue density (RTD), specific root length (SRL), and specific root area (SRA) (Prada-Salcedo et al., 2021; Sun et al., 2021). Traditionally, roots with a diameter < 2 mm have been classified as fine roots, characterized as short-lived and primarily involved in absorption. Conversely, roots with a diameter > 2 mm are typically classified as coarse roots, known for their longer lifespan and key role in water and nutrient transport (Finer et al., 2007). Fine roots are vital systems for plants to obtain nutrients and water, and their functions may have an impact on plant growth and survival (Comas et al., 2013; Jagodzinski et al., 2016). Besides the role of nutrient uptake, fine roots in forest trees also influence nutrient availability by depositing C as root exudates (Finzi et al., 2015). Previous studies demonstrated that higher root exudation in the rhizosphere soil of forest due to higher fine root biomass and surface area released more root exudates (Heinzle et al., 2023) (Figure 3). Therefore, root-exudate flux appears to be linked to fine-root functional trait coordination across nutrient availability, temperature, and moisture gradients in different forest ecosystems (Nakayama and Tateno, 2018; Sun et al., 2021). Furthermore, previous research suggests that root exudation in forest trees is positively linked to the SRL (Meier et al., 2020). For example, exudation amount of mature Quercus crispula plants was augmented fivefold when SRL was doubled (Nakayama and Tateno, 2018). This phenomenon is strengthened by a tissue physiological mechanism, because plentiful metabolites are enriched into the phloem, transported to the root, and then released at the root apical meristem, as size of xylem and phloem is related to high SRL (Farrar et al., 2003) (Figure 4). Other research in forest ecosystems has shown that root morphology has a profound impact on root metabolite secretion, as exudation rates were higher when roots were on average thinner and had more root tips (Meier et al., 2015; Zhao et al., 2023). In thinner roots of forest trees, the non-structural C concentration for root formation is lowered, allowing more C to be available for root exudation (Lv et al., 2023).
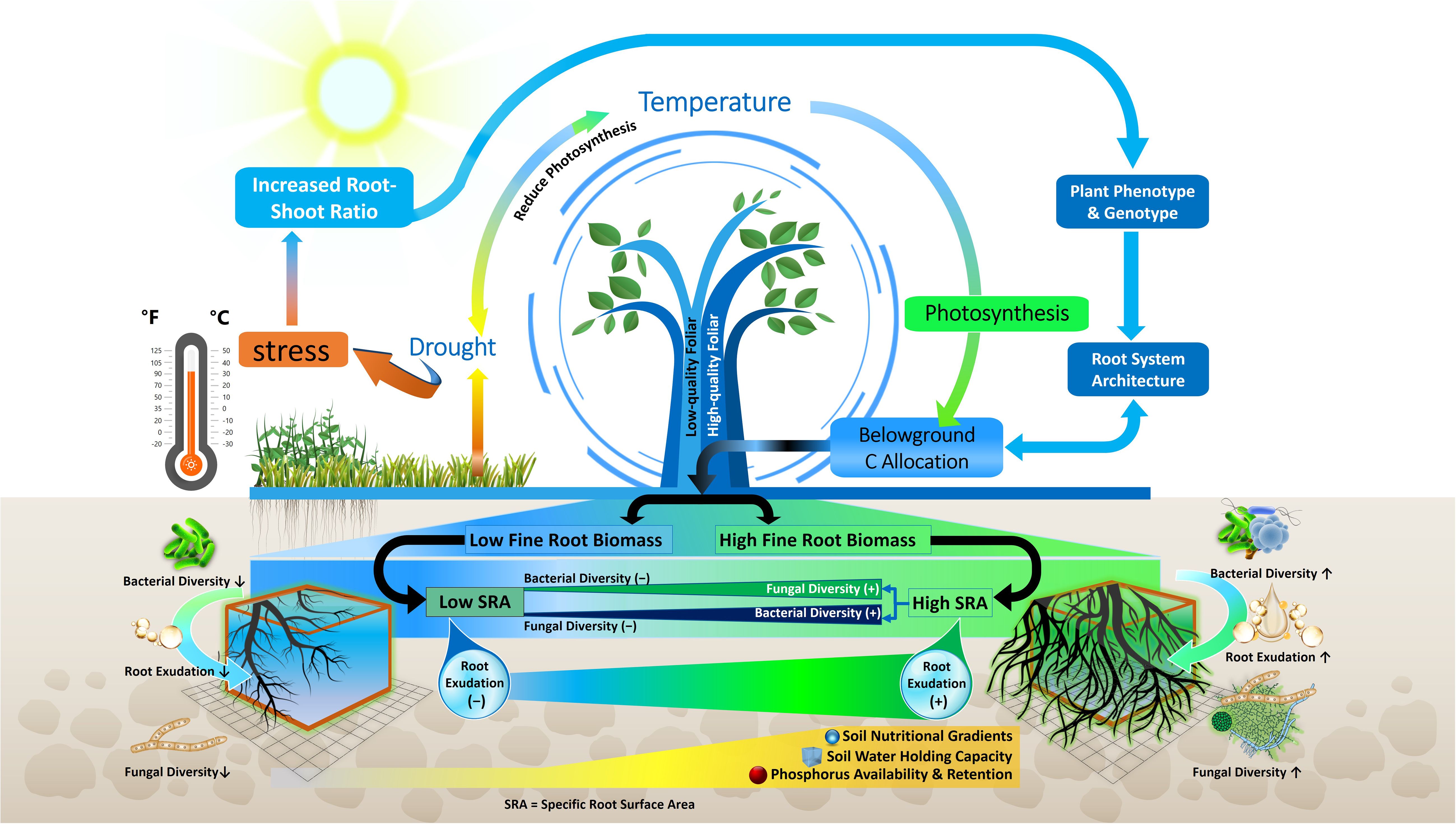
Figure 3 Schematic of the relationships among root traits, climate factors, root exudation and microbiome composition. Drought and temperature affect photosynthetic activity and belowground C allocation which in turn influence fine root biomass and specific root surface area (SRA), root exudation, microbial diversity and nutrient availability. Decreased root exudation (-); increased root exudation (+); higher fungal and bacterial diversity (↑); and Lower fungal and bacterial diversity (↓).
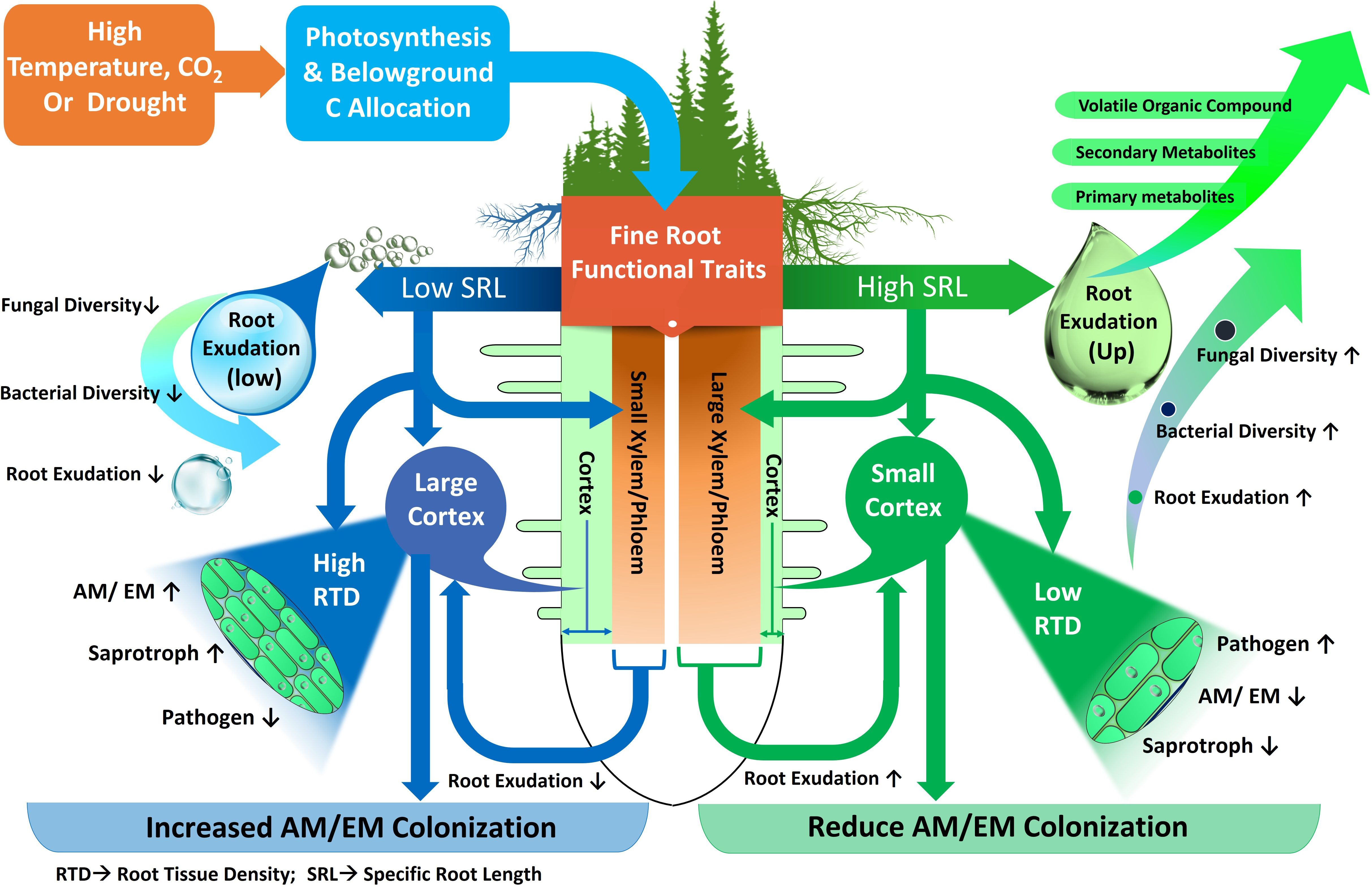
Figure 4 Fine root functional traits and their relationships with environmental variables, root metabolites and groups of microorganisms. Precipitation and temperature affect fine root functional traits with low or high specific root length (SRL). Roots with low SRL possess small Xylem-Phloem and large cortex with high root tissue density (RTD). Roots with high SRL possess big Xylem-Phloem and small cortex with low root tissue density (RTD). Decreased (↓) or increased (↑) fungi, bacteria, ectomycorrhiza (EM), arbuscular mycorrhiza (AM), saprotroph and pathogen diversity and activity.
The variation in root metabolite exudation of trees is influenced by several fine root functional traits, notably including root diameter, RTD, and root nitrogen content. These traits have been observed to account for significant variations in exudate metabolites. For instance, in a forest ecosystem, Williams and de Vries (2020) reported an increase in specific root metabolite exudation with larger root diameters and a decrease with higher RTD. This inverse relationship between metabolite exudation and RTD can be interpreted as an indicator of a conservative trait, which is typically associated with slower growth due to the higher carbon costs involved in structural root development and adaptability to environments with limited nutrient availability. Conservative traits, like high RTD, are usually linked to slower growth rates, greater resilience, and efficiency in resource use, often seen in environments with limited resources. Conversely, acquisitive or competitive traits are associated with faster growth and higher resource turnover, evident in environments with abundant resources. For example, in species like Poaceae and Quercus, studies by Guyonnet et al. (2018) and Sun et al. (Sun et al., 2017, 2021) found a positive correlation between root exudation and traits indicative of competitiveness (acquisitive traits) such as root respiration, root tissue nitrogen, SRL, and SRA. On the other hand, a negative correlation was observed with RTD, a conservative trait. This pattern suggests that root exudation tends to align more with acquisitive (competitive) rather than conservative traits. This implies that roots with higher nitrogen content and carbon respiration rates are more likely to release carbon-based root metabolites, while those with higher construction costs, reflecting conservative traits, tend to exude fewer metabolites.
5 Metabolite and root traits across soil horizons impact microbial functional groups
Root metabolites contribute plant-derived carbon to the forest’s soil, with the rate of root exudation varying over time, space, and across different environmental conditions (Gao et al., 2021; Keller et al., 2021; Chen et al., 2023). For instances, crucial soil parameters such as organic matter distribution, which varies with soil depth in temperate forest ecosystems, affect both root exudation and microbial composition and function (Schenk, 2005; Tückmantel et al., 2017) (Figure 1). Furthermore, a decline in root exudation from topsoil to subsoil is observed due to reductions in soil microbial biomass and nutrient availability, alongside an increase in aromatic carbon in soil organic matter as soil depth increases in a temperate forest ecosystem (Tückmantel et al., 2017). Roots in topsoil provide C-rich substrates that promote microbial breakdown of complex organic compounds (Phillips et al., 2012; Meier et al., 2015, 2017) (Figure 1). The allocation of carbon (C) to tree roots and root metabolites can vary under different nutrient regimes at various soil depths, influenced by diverse microbial interactions (Figure 1). For instance, ectomycorrhizal fungi enhance the quantity and alter the composition of carbon exuded by host plant’s roots across different soil layers in Norway spruce forest (Rineau and Garbaye, 2009). Furthermore, the decrease in tree root exudation with increasing soil depth is influenced by factors such as decreasing temperature, lower bulk density, and higher oxygen supply and organic matter input in the topsoil compared to the subsoil (Schenk, 2005; Neumann and Römheld, 2007; Rineau and Garbaye, 2009; Jakoby et al., 2020; Proctor and He, 2021) (Figure 1). In forest ecosystem, leaf and root litter, as well as root exudation, are principal sources of fresh organic C input to subsoils (Rumpel and Kögel-Knabner, 2011; Mueller et al., 2015; Angst et al., 2016; Rawlik et al., 2022) (Figure 1). Therefore, the structure of a forest plant’s root system is crucial in determining the vertical distribution of organic matter and nutrients within the soil (Iversen, 2010; Figure 1). The nature of fine roots of forest trees varies across soil depths, with deeper layers typically having primary roots and upper layers populated by finer, fibrous roots (Trocha et al., 2017; Pang et al., 2022) (Figure 1). This variation in root types across soil layers could affect root exudation patterns, with higher exudation and fine root turnover in topsoil compared to deep soil (De Graaff et al., 2014; Tückmantel et al., 2017) (Figure 1).
Distribution of different root categories (fine or coarse) across soil horizons in forest ecosystem also shape the microbial community composition (Makita et al., 2011; Li et al., 2020; Wang et al., 2020) (Figure 3). Fine roots in the topsoil of forest ecosystem attract more diverse and species-rich fungal and bacterial communities (Liu et al., 2022). Interestingly, fine roots, rather than coarse ones, exhibited higher bacterial abundance, suggesting that trees with a higher proportion of fine roots (or root hairs) might attract diverse microbial communities for mutualistic benefits (Pan et al., 2023). For instance, in a subtropical forest, abundance of Proteobacteria, Actinobacteria and Chloroflexi were higher in fine roots with high SRL (Pan et al., 2023). This may be due to the higher surface area of fine roots in the topsoil allowing greater nutrient and metabolite transport compared to coarse roots in forest ecosystem (Wang et al., 2020; Heinzle et al., 2023) (Figure 3). In this context of fine root traits, higher overall fungal richness in the topsoil is associated with higher SRL, as observed in the cultivation of single tree species like Carpinus betulus and Quercus robur (Prada-Salcedo et al., 2021) (Figure 4). Conversely, fungal richness is lower in soils associated with trees like Quercus cerris and Quercus ilex, which supply more resources for higher RTD to support longer root lifespan. Similarly, these root characteristics facilitate connections between symbiotic fungi and fine roots (Fernandez and Kennedy, 2016; Beidler and Pritchard, 2017) (Figure 4). The thickness of absorptive fine roots differs by tree species, and fine root diameter significantly impacts symbiotic fungal communities more than other root characteristics (Ma et al., 2018) because cortex segment size is crucial for nutrient acquisition via symbiotic mycorrhizal fungi (Bergmann et al., 2020) (Figure 4). SRL is a measure of the relative amount of very fine roots in a root system, and trees with high SRL have thinner roots (Ma et al., 2018), with lower cortex (Kong et al., 2014) and reduced colonization of AM or EM fungi (Brundrett and Tedersoo, 2018) in forest trees. Thus, it can be hypothesized that a reduced root diameter may lead to a smaller cortical area, potentially affecting the colonization by mycorrhizal fungi (Figure 4). This hypothesis is supported by the findings of Guo et al. (2008) and Liu et al. (2015), who explored the relationship between root diameter and mycorrhizal colonization in trees of temperate and subtropical forest ecosystems. They noted that a reduced root diameter provides a smaller cortical area, which can limit the environment available for mycorrhizal fungi. Furthermore, root diameter is positively correlated with AM, EM and saprotrophic fungal abundance and diversity but negatively correlated with pathogenic fungal abundance and diversity in different forest ecosystems (Eissenstat et al., 2015; Chen et al., 2018; Dai et al., 2023). This finding is also supported by Semchenko et al. (2018) in a temperate ecosystem, who demonstrated that roots of high SRL were linked with an increased diversity of fungal pathogens and decreased diversity of fungal symbionts, as SRL is inversely related to root diameter (Figure 4). Thus, the nature of root exudation and root traits, such as specific root length and diameter, which vary with soil depth, play a crucial role in shaping the composition and diversity of microbial populations, thereby impacting the ecological balance and nutrient cycling within forest ecosystems.
6 Influence of climate factors on root traits, root exudation and microbiome
6.1 Drought effects
Change in plant traits in forest ecosystem is a crucial strategy by which tree species can survive under adverse environmental conditions (Henn et al., 2018; Pérez-Ramos et al., 2019). It is well recognized that tree leaf and root characteristics exhibit considerable pliability in response to climate change (Bardgett et al., 2014b; Paź-Dyderska et al., 2020; Paź-Dyderska and Jagodziński, 2023) such as variation in sunlight, temperature (Keenan and Niinemets, 2016) and precipitation (Fry et al., 2018). For instances, tree species adapted to arid environments often show increased root-to-shoot ratios, aiding in drought resistance by optimizing water uptake relative to water loss through transpiration (Kozlowski and Pallardy, 2002; Hartmann, 2011). Although drought typically reduces fine root biomass, thus conserving water by reducing transpirational surface area, the stability of certain traits like specific root length (SRL) and root tissue density (RTD) contributes to maintaining water and nutrient uptake efficiency under stress conditions (Arend et al., 2011; Herzog et al., 2014). Furthermore, adjustments in root architecture, such as increased biomass in very fine roots, enhance the plant’s ability to explore soil for moisture, thereby improving drought resilience (Olmo et al., 2014). These trait adaptations collectively enhance the drought resistance of plants, allowing for more efficient water usage and better survival during dry periods. Thus, drought stress significantly alters root traits and metabolite profiles in plants, profoundly influencing the rhizosphere microbiome (Figure 4). Drought-induced changes in the rhizosphere microbiome are partly governed by plant-specific responses, especially in root characteristics, including changes in root morphology or shifts in belowground carbon distribution patterns, crucial for forest trees adaptation to drought (Ma et al., 2018; Hildebrand et al., 2023). For example, in a forest ecosystem, metabolomic analyses of the Populus trichocarpa plant have shown that drought conditions increase the plant’s investment in carbon (C) and nitrogen (N) metabolisms, as evidenced by the root tissue profiles of amino acids, fatty acids, and phenolic glycosides (Veach et al., 2020) and thus tolerance to drought condition. Notably, several of these metabolites have been found to positively correlate with the alpha-diversity of root-associated fungal and bacterial communities, although such correlations were not observed in soil microbial communities (Veach et al., 2020). However, Timm et al. (2018) found that the bacterial alpha-diversity of Populus deltoides rhizosphere was reduced under drought stress and certain core bacterial operational taxonomic units (OTUs) were correlated with specific plant metabolites in the roots, such as amino acids and aromatics. Additionally, the fungal response to drought varies significantly by trophic guild, linked to root morphological adjustments to water scarcity. For instance, in forest ecosystems, specifically regarding Populus euphratica under drought conditions, it was observed that the abundance of symbiotrophic microbes was negatively correlated with SRL. Conversely, the abundance of saprotrophic microbes showed a positive correlation with SRL (Xia et al., 2023). This implies that drought conditions influence the relationship between root morphology and the types of microbial communities present in the rhizosphere. However, Lv et al. (2023), observed that drought increased root diameter, tissue density, nitrogen concentration, exudation C, and mycorrhizal colonization, but decreased non-structural C concentration, and specific root length and area in a temperate forest ecosystem which may increase the drought tolerance of plants. While the specific impacts of drought on rhizosphere microbial groups in forest ecosystems, particularly through changes in root morphology and root metabolites, are not fully understood, studies in grasslands and agricultural ecosystems, as detailed in the information presented below, offer relevant insights. For example, under drought conditions, some plants modify their root architecture, often increasing the root:shoot ratio by developing finer roots to enhance water uptake with a lower carbon investment and reduced reliance on symbiotic fungi (Comas et al., 2013). In contrast, other species develop thicker roots and are more dependent on symbiotic relationships for water and nutrient acquisition (Comas et al., 2012; Lozano et al., 2022). This response is coupled with changes in root exudation patterns due to decreased photosynthetic activity, impacting the microbial community in the rhizosphere (Hartman and Tringe, 2019; Chen et al., 2022). The shift in root exudates under drought, including various primary (e.g. organic acids) and secondary metabolites (e.g. terpenoids), creates a different soil microenvironment. This alteration, influenced by plant-specific metabolite exudation, affects the microbial community dynamics, potentially impacting microbial species abundance and diversity (Fitzpatrick et al., 2018; Ochoa-Hueso et al., 2018; Williams and de Vries, 2020). These metabolites exudation change helps plants resilience to drought stress by enhancing beneficial symbiotic interactions. For example, strigolactone and flavonoids excretion plays a crucial role in enhancing plants’ resilience to abiotic stress. For example, there is a notable association between the amount of strigolactones and flavonoids released and the rate of AM fungal colonization in plants under water scarcity conditions (Ruiz-Lozano et al., 2016). Plant root exudates can selectively attract certain bacteria that contribute to enhancing drought resilience. For instance, under drought conditions, plants shown to secrete higher levels of organic acids, notably malic acid, in addition to fumaric, malonic, succinic, and oxalic acids (Henry et al., 2007). Malic acid acts as a potent attractant for the bacterium Bacillus subtilis, observed within the soybean rhizosphere (Allard-Massicotte et al., 2016), a microbial chemoattractant known to bolster drought tolerance of plants through the stimulation of osmolyte production (Gagné-Bourque et al., 2016). Additionally, beneficial interactions have been documented between plants and other bacteria such as Pseudomonas putida and Bacillus amyloliquefaciens through these organic acid exudation, which help mitigate the adverse effects of drought (Kumar et al., 2016). While these studies provide valuable information, further research is needed to understand the specific dynamics of microbial groups and their relationships with root metabolites and morphological traits in forests under drought conditions.
6.2 Influence of temperature
Temperature is another environmental factor which influenced root morphology and exudation. It was demonstrated that root metabolite exudation of forest trees increases at higher mean annual temperature (MAT) and atmospheric CO2 (Meier et al., 2020) and thus influences rhizosphere microbes. Zadworny et al. (2017) revealed that distinct root morphological characteristics of Scots pine are linked to MAT, with bigger root diameter and lower SRL and RTD with lower MAT in northern Europe. The declining MAT caused an upsurge of structural protection (increased xylem/phloem layer) in fine transport roots of Pinus sylvestris (Zadworny et al., 2017) which might constrain the growth of pathogens. By contrast, low MAT enhance the growth of mutualistic and saprotrophic fungi in Pinus sylvestris, as larger cortex with high RTD offers more space for fungal colonization (Zadworny et al., 2017). In beech forests, Leuschner et al. (2022) found that root exudate release significantly increased, nearly tripling, as average daily temperatures rose from 10°C to 20°C. This points to a substantial temperature impact on carbon flux from roots to soil. However, the study by Yang et al. (2023) revealed that in an alpine coniferous forest, air temperature had a significant effect on root exudation by altering the characteristics and biomass of fine roots. Their findings suggest that the adaptation of carbon provision by roots and the morphological traits of fine roots to lower temperatures are key factors leading to decreased trees root exudation. Complementing this, Pausch and Kuzyakov (2018) observed that higher temperatures in beech forests stimulate the plant’s demand for nitrogen and other nutrients, leading to increased root exudation to support soil microbes, thereby boosting microbial activity. Furthermore, warming significantly influences tree root exudation and, consequently, the rhizosphere microbiome (Yu et al., 2024). For instance, in a temperate forest, Yu et al. (2024) observed that warming led to a reduction in secondary metabolite exudation, causing a decline in the complexity of soil bacterial and fungal communities in the rhizosphere, unlike in non-rhizosphere soil. Furthermore, long-term warming in temperate forest influenced root exudation (Heinzle et al., 2023) and decreased fine root biomass (Wang et al., 2021c), and influenced the rhizosphere microbial community. Together, these studies underscore the impact of warming on root-soil-microbe interactions, highlighting the importance of both the quantity and diversity of tree root metabolites in maintaining soil microbial diversity and ecosystem functioning under changing climate conditions (Philippot et al., 2013; Venturi and Keel, 2016; Yu et al., 2024).
6.3 Effects of elevated CO2 and N availability
The response of soil microbes to nitrogen (N) deposition and elevated CO2 in forest ecosystems varies, likely influenced by the unique survival strategies of these environments which involve alterations in the root traits and exudates of trees. For example, rising levels of atmospheric CO2 are expected to enhance the supply of carbon (C) and nitrogen (N) to fine roots, especially in N-deficient forest ecosystems (Norby and Jackson, 2000). This increase in fine-root distribution affects soil C storage and N cycling since fine roots in forests have a rapid turnover rate (Gill and Jackson, 2000) and contributes significantly to the soil’s C and N contents (Iversen et al., 2008), thereby impacting soil microbial communities. Studies have found that trees in CO2-enriched environments increase C allocation to roots via root exudates, providing energy for microbial processes that convert unavailable forms of N into forms that forest plants can use (Langley et al., 2009; Schleppi et al., 2012). These C-rich exudates stimulate free-living microbes to release enzymes that break down N-rich soil organic matter. In addition, under high CO2 conditions, trees may directly supply C to mycorrhizal fungi to access N from soil organic matter (Talbot and Treseder, 2010). However, soil N availability might negatively impact root exudation. For instance, in a forest ecosystem, the addition of nitrogen was observed to negatively impact the root exudates of Pinus tabulaeformis, leading to an inhibition of fungal and bacterial populations in the rhizosphere soil (Jing et al., 2023). However, it was observed that roots of different diameters responded differently to nitrogen (N) addition and microbial colonization. Specifically, N addition enhanced the concentration of carbon (C) metabolites in roots, with finer roots showing more pronounced changes than thicker roots and increased the rhizosphere microbial diversity and bacterial quantity, but decreased fungal quantity (Jing et al., 2023). Furthermore, Meier et al. (2020) found that at acidic, N-poor sites, the quantity of C released with root exudation is closely positively related to SRL and soil acidity and negatively to fungal abundance and activity in a mature beech forest. Moreover, studies in tropical forest ecosystems showed a positive relationship between the amount of root exudation and the nitrogen content in roots, while there was a negative correlation with the density of root tissues (Sun et al., 2021), which might have some influence on microbial populations. For example, Weng et al. (2013) found that nitrogen (N) application increased the abundance of bacterial in the rhizosphere and this change was correlated to an increase in the production of amino acids and carbohydrates in the root exudates in a mangrove forest. N availability also plays a critical role in shaping the development and structure of the trees fine root system. It has been shown that N availability in a temperate forest leads to changes in root traits, such as increased SRL, reduced root diameter, and higher root biomass allocation (Li et al., 2021), thereby affecting mutualistic or pathogenic fungal communities (Figure 4). Furthermore, in temperate forest systems, research on three Quercus species revealed a significant link between root exudation and the nitrogen content in root tissues (Ataka et al., 2020), which in turn influenced the woodland microbial community.
Thus, significant changes in root morphological characteristics and metabolomics profiles along drought, temperature, elevated atmospheric CO2 and N availability gradients, explain the changes of root microbial communities and adaptive strategies of plants under global climate change scenarios.
7 Conclusions
In conclusion, this review summarizes the general changes in the relative abundance and activity of soil microbial communities, alongside alterations in root traits and metabolites across soil horizons, and their associations with climatic drivers of change. We have concluded that different soil horizons, root characteristics, and environmental conditions collectively influence microbial communities in forest ecosystems. It explains that various soil layers, each with distinct physical and chemical properties, harbor specific microbial populations. The diversity in microbial life is largely dependent on the soil’s organic matter content and nutrient availability, with particular emphasis on the distinct distributions of fungi such as ectomycorrhizal and saprotrophic species across different soil horizons. Root exudates, significantly influenced by root morphology including diameter, tissue density, and specific root length, are crucial in shaping the composition and activity of these soil microbial communities. Furthermore, the review underscores the role of environmental factors like drought, temperature, and nutrient availability in altering root traits and exudation patterns. These environmental changes, in turn, affect the structure and function of microbial communities. Stated simply, linking rhizospheric microbial interactions to root morphology and metabolomics may help us to reserve below ground resources for maintenance and enhancement of natural ecosystem services in the context of climate change. Furthermore, this review explains the adaptive strategies of plants by harnessing root trait plasticity and microbial interactions under global climate change scenarios. However, there remain some open questions regarding the environmental effects on plant traits and their implications for the rhizosphere microbiome in forest ecosystems. These questions are particularly pertinent to plant functional traits concerning the functions of root metabolites, changes in root morphology, and their relationships to microbial functional groups.
Author contributions
PM: Conceptualization, Data curation, Formal analysis, Methodology, Writing – original draft, Writing – review & editing. KH: Investigation, Supervision, Validation, Writing – review & editing. AS: Data curation, Validation, Writing – review & editing. AJ: Resources, Supervision, Writing – review & editing. JA-R: Methodology, Visualization, Writing – review & editing. DM: Methodology, Writing – review & editing. JM: Conceptualization, Investigation, Methodology, Project administration, Supervision, Writing – review & editing.
Funding
The author(s) declare that financial support was received for the research, authorship, and/or publication of this article. This research was supported by the National Science Centre, Poland, grant 2019/35/B/NZ8/01361 and by home institute of the authors.
Conflict of interest
The authors declare that the research was conducted in the absence of any commercial or financial relationships that could be construed as a potential conflict of interest.
Publisher’s note
All claims expressed in this article are solely those of the authors and do not necessarily represent those of their affiliated organizations, or those of the publisher, the editors and the reviewers. Any product that may be evaluated in this article, or claim that may be made by its manufacturer, is not guaranteed or endorsed by the publisher.
References
Abdulsalam, O., Wagner, K., Wirth, S., Kunert, M., David, A., Kallenbach, M., et al. (2021). Phytohormones and volatile organic compounds, like geosmin, in the ectomycorrhiza of Tricholoma vaccinum and Norway spruce (Picea abies). Mycorrhiza 31, 173–188. doi: 10.1007/s00572-020-01005-2
Adamo, I., Dashevskaya, S., Alday, J. G. (2022). Fungal perspective of Pine and Oak colonization in Mediterranean degraded ecosystems. Forests 13, 88. doi: 10.3390/f13010088
Akiyama, K., Hayashi, H. (2006). Strigolactones: Chemical signals for fungal symbionts and parasitic weeds in plant roots. Ann. Bot. 97, 925–931. doi: 10.1093/aob/mcl063
Allard-Massicotte, R., Tessier, L., Lécuyer, F., Lakshmanan, V., Lucier, J., Garneau, D., et al. (2016). Bacillus subtilis early colonization of Arabidopsis thaliana roots involves multiple chemotaxis receptors. mBio 7, e01664–e01616. doi: 10.1128/mBio.01664-16
Angst, G., John, S., Mueller, C., Kögel-Knabner, I., Rethemeyer, J. (2016). Tracing the sources and spatial distribution of organic carbon in subsoils using a multi-biomarker approach. Sci. Rep. 6, 29478. doi: 10.1038/srep29478
Arend, M., Kuster, T., Günthardt-Goerg, M. S., Dobbertin, M. (2011). Provenance-specific growth responses to drought and air warming in three European oak species (Quercus robur, Q. petraea and Q. pubescens). Tree Physiol. 31, 287–297. doi: 10.1093/treephys/tpr004
Ataka, M., Sun, L., Nakaji, T., Katayama, A., Hiura, T. (2020). Five-year nitrogen addition affects fine root exudation and its correlation with root respiration in a dominant species, Quercus crispula, of a cool temperate forest, Japan. Tree Physiol. 40, 367–376. doi: 10.1093/treephys/tpz143
Baldrian, P. (2017). Forest microbiome: diversity, complexity, and dynamics. FEMS Microbiol. Rev. 41, 109–130. doi: 10.1093/femsre/fuw040
Bardgett, R. D., Mommer, L., De Vries, F. T. (2014b). Going underground: root traits as drivers of ecosystem processes. Trends Ecol. Evol. 29, 692–699. doi: 10.1016/j.tree.2014.10.006
Bardgett, R. D., van der Putten, W. H. (2014a). Belowground biodiversity and ecosystem functioning. Nature 515, 505–511. doi: 10.1038/nature13855
Beidler, K. V., Pritchard, S. G. (2017). Maintaining connectivity: understanding the role of root order and mycelial networks in fine root decomposition of woody plants. Plant Soil 420, 19–36. doi: 10.1007/s11104-017-3393-8
Bellande, K., Bono, J.-J., Savelli, B., Jamet, E., Canut, H. (2017). Plant lectins and lectin receptor-like kinases: How do they sense the outside? Int. J. Mol. Sci. 18, 1164. doi: 10.3390/ijms18061164
Bergmann, J., Weigelt, A., Plas, F. V., Laughlin, D. C., Kuyper, T. W. (2020). The fungal collaboration gradient dominates the root economics space in plants. Sci. Adv. 6, eaba3756. doi: 10.1126/sciadv.aba3756
Bi, B., Yuan, Y., Zhang, H., Wu, Z., Wang, Y., Han, F. (2022). Rhizosphere soil metabolites mediated microbial community changes of Pinus sylvestris var. mongolica across stand ages in the Mu Us Desert. Appl. Soil Ecol. 169, 104222. doi: 10.1016/j.apsoil.2021.104222
Bicharanloo, B., Cavagnaro, T. R., Keitel, C., Dijkstra, F. A. (2021). Nitrogen fertilisation increases specific root respiration in ectomycorrhizal but not in arbuscular mycorrhizal plants: A meta-analysis. Front. Plant Sci. 12. doi: 10.3389/fpls.2021.711720
Bodeker, I. T. M., Clemmensen, K. E., de Boer, W., Martin, F., Olson, A., Lindahl, B. D. (2014). Ectomycorrhizal Cortinarius species participate in enzymatic oxidation of humus in northern forest ecosystems. New Phytol. 203, 245–256. doi: 10.1111/nph.12791
Brundrett, M. C., Tedersoo, L. (2018). Evolutionary history of mycorrhizal symbioses and global host plant diversity. New Phytol. 220, 1108–1115. doi: 10.1111/nph.14976
Bulgarelli, D., Ruben Garrido-Oter, R., Münch, P. C., Weiman, A., Dröge, J., Pan, Y., et al. (2015). Structure and function of the bacterial root microbiota in wild and domesticated barley. Cell Host Microbe 17, 392–403. doi: 10.1016/j.chom.2015.01.011
Carteron, A., Vellend, M., Laliberté, E. (2021). Mycorrhizal dominance reduces local tree species diversity across US forests. Nat. Ecol. Evol. 6, 370–374. doi: 10.1038/s41559-021-01634-6
Cesco, S., Neumann, G., Tomasi, N., Pinton, R., Weisskopf, L. (2010). Release of plant-borne flavonoids into the rhizosphere and their role in plant nutrition. Plant Soil 329, 1–25. doi: 10.1007/s11104-009-0266-9
Chagas, F. O., Pessotti, R. C., Caraballo-Rodríguez, A. M., Pupo, M. T. (2018). Chemical signaling involved in plant–microbe interactions. Chem. Soc Rev. 47, 1652–1704. doi: 10.1039/C7CS00343A
Chen, M., Yao, X., Cheng, H., Fan, A., Lin, R., Wang, X., et al. (2023). Changes in Chinese fir plantations root exudation strategies seasonally and as tree age. For. Ecol. Manage. 545, 121239. doi: 10.1016/j.foreco.2023.121239
Chen, W., Koide, R. T., Eissenstat, D. M. (2018). Root morphology and mycorrhizal type strongly influence root production in nutrient hot spots of mixed forests. J. Ecol. 106, 148–156. doi: 10.1111/1365-2745.12800
Chen, Y., Yao, Z., Sun, Y., Wang, E., Tian, C., Sun, Y., et al. (2022). Current Studies of the effects of drought stress on root exudates and rhizosphere microbiomes of crop plant species. Int. J. Mol. Sci. 23, 2374. doi: 10.3390/ijms23042374
Chu, H., Sun, H., Tripathi, B. M., Adams, J. M., Huang, R., Zhang, Y., et al. (2016). Bacterial community dissimilarity between the surface and subsurface soils equals horizontal differences over several kilometers in the western Tibetan Plateau. Appl. Microbiol. Int. 18, 1523–1533. doi: 10.1111/1462-2920.13236
Clark, J., Bennett, T. (2023). Cracking the enigma: understanding strigolactone signalling in the rhizosphere. J. Exp. Bot. 75, 1159–1173. doi: 10.1093/jxb/erad335
Comas, L. H., Becker, S. R., Cruz, V. M. V., Byrne, P. F., Dierig, D. A. (2013). Root traits contributing to plant productivity under drought. Front. Plant Sci. 4. doi: 10.3389/fpls.2013.00442
Comas, L. H., Mueller, K. E., Taylor, L. L., Midford, P. E., Callahan, H. S., Beerling, D. J. (2012). Evolutionary patterns and biogeochemical significance of angiosperm root traits. Int. J. Plant Sci. 173, 584–595. doi: 10.1086/665823
Cotton, T. E. A., Pétriacq, P., Cameron, D. D., Meselmani, M. A., Schwarzenbacher, R., Rolfe, S. A., et al. (2019). Metabolic regulation of the maize rhizobiome by benzoxazinoids. ISME J. 13, 1647–1658. doi: 10.1038/s41396-019-0375-2
Dai, D., Yang, J., Wu, Y., Zhang, W., Wu, X., Liu, Y., et al. (2023). Correlation between fine root traits and pathogen richness depends on plant mycorrhizal types. Oikos. 2023 (1), e09354. doi: 10.1111/oik.09354
DeAngelis, K. M., Grace, P., Begüm, T. D., van Diepen Linda, T. A., Rebecca, V. M., Jeffrey, B. L., et al. (2015). Long-term forest soil warming alters microbial communities in temperate forest soils. Front. Microbiol. 6. doi: 10.3389/fmicb.2015.00104
De Graaff, M.-A., Jastrow, J. D., Gillette, S., Johns, A., Wullschleger, S. D. (2014). Differential priming of soil carbon driven by soil depth and root impacts on carbon availability. Soil Biol. Biochem. 69, 147–156. doi: 10.1016/j.soilbio.2013.10.047
De Hoff, P. L., Brill, L. M., Hirsch, A. M. (2009). Plant lectins: the ties that bind in root symbiosis and plant defense. Mol. Genet. Genomics 282, 1–15. doi: 10.1007/s00438-009-0460-8
De-la-Peña, C., Badri, D. V., Lei, Z., Watson, B. S., Brandão, M. M., Silva-Filho, M. C., et al. (2010). Root secretion of defense-related proteins is development-dependent and correlated with flowering time. J. Biol. Chem. 285, 30654–30665. doi: 10.1074/jbc.M110.119040
Delory, B. M., Delaplace, P., Fauconnier, M.-L., du Jardin, P. (2016). Root-emitted volatile organic compounds: can they mediate belowground plant-plant interactions? Plant Soil 402, 1–26. doi: 10.1007/s11104-016-2823-3
Dickie, I. A., Xu, B., Koide, R. T. (2002). Vertical niche differentiation of ectomycorrhizal hyphae in soil as shown by T-RFLP analysis. New Phytol. 156, 527–535. doi: 10.1046/j.1469-8137.2002.00535.x
Ding, X., Liu, K., Yan, Q., Liu, X., Chen, N., Wang, G., et al. (2021). Sugar and organic acid availability modulate soil diazotroph community assembly and species co-occurrence patterns on the Tibetan Plateau. Appl. Microbiol. Biotechnol. 105, 8545–8560. doi: 10.1007/s00253-021-11629-9
Dong, W., Song, Y. (2020). The significance of flavonoids in the process of biological nitrogen fixation. Int. J. Mol. Sci. 21, 5926. doi: 10.3390/ijms21165926
Eilers, K. G., Debenport, S., Anderson, S., Fierer, N. (2012). Digging deeper to find unique microbial communities: The strong effect of depth on the structure of bacterial and archaeal communities in soil. Soil Biol. Biochem. 50, 58–65. doi: 10.1016/j.soilbio.2012.03.011
Eissenstat, D. M., Kucharski, J. M., Zadworny, M., TS, A., Koide, R. T. (2015). Linking root traits to nutrient foraging in arbuscular mycorrhizal trees in a temperate forest. New Phytol. 208, 114–124. doi: 10.1111/nph.13451
Fang, W., St. Leger, R. J. (2010). Mrt, a gene unique to fungi, encodes an oligosaccharide transporter and facilitates rhizosphere competency in Metarhizium robertsii. Plant Physiol. 154, 1549–1557. doi: 10.1104/pp.110.163014
Farrar, J., Hawes, M., Jones, D. L., Lindow, S. (2003). How roots control the flux of carbon to the rhizosphere. Ecology 84, 827–837. doi: 10.1890/0012-9658(2003)084[0827:HRCTFO]2.0.CO;2
Fernandez, C. W., Kennedy, P. G. (2016). Revisiting the ‘Gadgil effect’: do interguild fungal interactions control carbon cycling in forest soils? New Phytol. 209, 1382–1394. doi: 10.1111/nph.13648
Finer, L., Helmisaari, H. S., Lohmus, K., Majdi, H., Brunner, I., Borja, I., et al. (2007). Variation in fine root biomassof three European tree species: beech (Fagus sylvatica L.), Norway spruce (Piceaabies L. Karst.), and Scots pine (Pinus sylvestris L.). Plant Biosyst. 141, 394–405. doi: 10.1080/11263500701625897
Finzi, A. C., Abramoff, R. Z., Spiller, K. S., Brzostek, E. R., Darby, B. A., Kramer, M. A., et al. (2015). Rhizosphere processes are quantitatively important components of terrestrial carbon and nutrient cycles. Glob. Change Biol. 21, 2082–2094. doi: 10.1111/gcb.12816
Fitzpatrick, C. R., Copeland, J., Wang, P. W., Guttman, D. S., Kotanen, P. M., Johnson, M. T. J. (2018). Assembly and ecological function of the root microbiome across angiosperm plant species. Proc. Natl. Acad. Sci. U S A. 115, E1157–E1165. doi: 10.1073/pnas.1717617115
Frey, B., Walthert, L., Perez-Mon, C., Stierli, B., Köchli, R., Dharmarajah, A., et al. (2021). Deep soil layers of drought-exposed forests harbor poorly known bacterial and fungal Communities. Front. Microbiol. 12. doi: 10.3389/fmicb.2021.674160
Frey, S. D. (2019). Mycorrhizal fungi as mediators of soil organic matter dynamics. Annu. Rev. Ecol. Evol. Syst. 50, 237–259. doi: 10.1146/annurev-ecolsys-110617-062331
Fry, E. L., Savage, J., Hall, A. L., Oakley, S., Pritchard, W. J., Ostle, N. J., et al. (2018). Soil multifunctionality and drought resistance are determined by plant structural traits in restoring grassland. Ecology 99, 2260–2271. doi: 10.1002/ecy.2437
Gagné-Bourque, F., Bertrand, A., Claessens, A., Aliferis, K. A., Jabaji, S. (2016). Alleviation of drought stress and metabolic changes in Timothy (Phleum pratense L.) colonized with Bacillus subtilis B26. Front. Plant Sci. 7. doi: 10.3389/fpls.2016.00584
Gao, W., Wang, Q., Zhu, X., Liu, Z., Li, N., Xiao, J., et al. (2021). The vertical distribution pattern of microbial- and plant-derived carbon in the rhizosphere in alpine coniferous forests. Rhizosphere 20, 100436. doi: 10.1016/j.rhisph.2021.100436
Gargallo-Garriga, A., Preece, C., Sardans, J., Oravec, M., Urban, O., Peñuelas, J. (2018). Root exudate metabolomes change under drought and show limited capacity for recovery. Sci. Rep. 8, 12696. doi: 10.1038/s41598-018-30150-0
Gill, R. A., Jackson, R. B. (2000). Global patterns of root turnover for terrestrial ecosystem. New Phytol. 147, 13–31. doi: 10.1046/j.1469-8137.2000.00681.x
Gómez, E. J., Delgado, J. A., González, J. M. (2020). Persistence of microbial extracellular enzymes in soils under different temperatures and water availabilities. Ecol. Evol. 10, 10167–10176. doi: 10.1002/ece3.6677
Gorzelak, M. A., Ellert, B. H., Tedersoo, L. (2020). Mycorrhizas transfer carbon in a mature mixed forest. Mol. Ecol. 29, 2315–2317. doi: 10.1111/mec.15520
Guo, D., Xia, M. X., X., W., Chang, W. J., Liu, Y., Wang, Z. Q. (2008). Anatomical traits associated with absorption and mycorrhizal colonization are linked to root branch order in twenty-three Chinese temperate tree species. New Phytol. 180, 673–683. doi: 10.1111/j.1469-8137.2008.02573.x
Guyonnet, J. P., Cantarel, A. A. M., Simon, L., Haichar., F. Z. (2018). Root exudation rate as functional trait involved in plant nutrient-use strategy classification. Ecol. Evol. 8, 8573–8581. doi: 10.1002/ece3.4383
Hartmann, H. (2011). Will a 385 million year-struggle for light become a struggle for water and for carbon?- how trees may cope with more frequent climate change-type drought events. Glob. Change Biol. 17, 642–655. doi: 10.1111/j.1365-2486.2010.02248.x
Hartemink, A. E., Zhang, Y., Bockheim, J. G., Curi, N., Silva, S. H. G., Grauer-Gray, J., et al. (2020). Soil horizon variation: A review. Adv. Agron. 160, 125–185. doi: 10.1016/bs.agron.2019.10.003
Hartman, K., Tringe, S. G. (2019). Interactions between plants and soil shaping the root microbiome under abiotic stress. Biochem. J. 476, 2705–2724. doi: 10.1042/BCJ20180615
Hassan, S., Mathesius, U. (2012). The role of flavonoids in root-rhizosphere signalling: opportunities and challenges for improving plant-microbe interactions. J. Exp. Bot. 63, 3429–3444. doi: 10.1093/jxb/err430
Hawkins, H. J., Cargill, R. I. M., Van Nuland, M. E., Hagen, S. C., Field, K. J., Sheldrake, M., et al. (2023). Mycorrhizal mycelium as a global carbon pool. Curr. Biol. 33, R560–R573. doi: 10.1016/j.cub.2023.02.027
Heinzle, J., Liu, X., Tian, Y., Kwatcho Kengdo, S., Heinze, B., Nirschi, A., et al. (2023). Increase in fine root biomass enhances root exudation by long-term soil warming in a temperate forest. Front. For. Glob. Change 6. doi: 10.3389/ffgc.2023.1152142
Henn, J. J., Buzzard, V., Enquist, B. J., Halbritter, A. H., Klanderud, K., Maitner, B. S., et al. (2018). Intraspecific trait variation and phenotypic plasticity mediate Alpine plant species response to climate change. Front. Plant Sci. 9. doi: 10.3389/fpls.2018.01548
Henry, A., Doucette, W., Norton, J., Bugbee, B. (2007). Changes in crested wheatgrass root exudation caused by flood, drought, and nutrient stress. J. Environ. Qual. 36, 904. doi: 10.2134/jeq2006.0425sc
Herzog, C., Steffen, J., Graf Pannatier, E., Hajdas, I., Brunner, I. (2014). Nine years of irrigation cause vegetation and fine root shifts in a water-limited pine forest. PloS One 9, e96321. doi: 10.1371/journal.pone.0096321
Hildebrand, G. A., Honeker, L. K., Freire-Zapata, V., Ayala-Ortiz, C., Rajakaruna, S., Fudyma, J., et al. (2023). Uncovering the dominant role of root metabolism in shaping rhizosphere metabolome under drought in tropical rainforest plants. Sci. Total Environ. 899, 165689. doi: 10.1016/j.scitotenv.2023.165689
Horodecki, P., Jagodziński, A. M. (2017). Tree species effects on litter decomposition in pure stands on afforested post-mining sites. For. Ecol. Manage. 406, 1–11. doi: 10.1016/j.foreco.2017.09.059
Horodecki, P., Nowiński, M., Jagodziński, A. M. (2019). Advantages of mixed tree stands in restoration of upper soil layers on postmining sites: A five-year leaf litter decomposition experiment. Land Degrad. Dev. 30, 3–13. doi: 10.1002/ldr.3194
Hu, L., Robert, C. A. M., Cadot, S., Zhang, X., Ye, M., Li, B., et al. (2018). Root exudate metabolites drive plant-soil feedbacks on growth and defense by shaping the rhizosphere microbiota. Nat. Commun. 9, 2738. doi: 10.1038/s41467-018-05122-7
Huang, A. C., Jiang, T., Liu, Y. X., Bai, Y. C., Reed, J., Qu, B., et al. (2019). A specialized metabolic network selectively modulates arabidopsis root microbiota. Science 10, 364. doi: 10.1126/science.aau6389
Iversen, C. M. (2010). Digging deeper: fine-root responses to rising atmospheric CO2 concentration in forested ecosystems. New Phytol. 186, 346–357. doi: 10.1111/j.1469-8137.2009.03122.x
Iversen, C. M., Ledford, J., Norby, R. J. (2008). CO2 enrichment increases carbon and nitrogen input from fine roots in a deciduous forest. New Phytol. 179, 837–847. doi: 10.1111/j.1469-8137.2008.02516.x
Jagodzinski, A. M., Ziółkowski, J., Warnkowska, A., Prais, H. (2016). Tree age effects on fine root biomass and morphology over chronosequences of Fagus sylvatica, Quercus robur and Alnus glutinosa stands. PloS One 11, e0148668. doi: 10.1371/journal.pone.0148668
Jakoby, G., Rog, I., Megidish, S., Klein, T. (2020). Enhanced root exudation of mature broadleaf and conifer trees in a Mediterranean forest during the dry season. Tree Physiol. 40, 1595–1605. doi: 10.1093/treephys/tpaa092
Jiang, Z., Fu, Y., Zhou, L., He, Y., Zhou, G., Dietrich, P., et al. (2023). Plant growth strategy determines the magnitude and direction of drought-induced changes in root exudates in subtropical forests. Glob. Change Biol. 29, 3476–3488. doi: 10.1111/gcb.16685
Jiao, S., Chen, W., Wang, J., Du, N., Li, Q., Wei, G., et al. (2018). Soil microbiomes with distinct assemblies through vertical soil profiles drive the cycling of multiple nutrients in reforested ecosystems. Microbiome 6, 146. doi: 10.1186/s40168-018-0526-0
Jing, H., Wang, H., Wang, G., Liu, G., Cheng, Y. (2023). Hierarchical traits of rhizosphere soil microbial community and carbon metabolites of different diameter roots of Pinus tabuliformis under nitrogen addition. Carbon Res. 2, 47. doi: 10.1007/s44246-023-00081-1
Johansson, E. M., Fransson, P. M. A., Finlay, R. D., Van Hees, P. A. W. (2008). Quantitative analysis of root and ectomycorrhizal exudates as a response to Pb, Cd and As stress. Plant Soil 313, 39–54. doi: 10.1007/s11104-008-9678-1
Johansson, E. M., Fransson, P. M. A., Finlay, R. D., van Hees, P. A. W. (2009). Quantitative analysis of soluble exudates produced by ectomycorrhizal roots as a response to ambient and elevated CO2. Soil Biol. Biochem. 41, 1111–1116. doi: 10.1016/j.soilbio.2009.02.016
Jourand, P., Hannibal, L., Majorel, C., Mengant, S., Ducousso, M., Lebrun, M. (2014). Ectomycorrhizal Pisolithus albus inoculation of Acacia spirorbis and Eucalyptus globulus grown in ultramafic topsoil enhances plant growth and mineral nutrition while limits metal uptake. J. Plant Physiol. 171, 164–172. doi: 10.1016/j.jplph.2013.10.011
Kaiser, C., Kilburn, M. R., Clode, P. L., Fuchslueger, L., Koranda, M., Cliff, J. B., et al. (2015). Exploring the transfer of recent plant photosynthates to soil microbes: mycorrhizal pathway vs direct root exudation. New Phytol. 205, 1537–1551. doi: 10.1111/nph.13138
Karst, J., Gaster, J., Wiley, E., Landhäusser, S. M. (2017). Stress differentially causes roots of tree seedlings to exude carbon. Tree Physiol. 37, 154–164. doi: 10.1093/treephys/tpw090
Keenan, T., Niinemets, Ü. (2016). Global leaf trait estimates biased due to plasticity in the shade. Nat. Plants 3, 16201. doi: 10.1038/nplants.2016.201
Keller, A. B., Brzostek, E. R., Craig, M. E., Fisher, J. B., Phillips, R. P. (2021). Root-derived inputs are major contributors to soil carbon in temperate forests, but vary by mycorrhizal type. Ecol. Lett. 24, 626–635. doi: 10.1111/ele.13651
Khokon, A. M., Schneider, D., Daniel, R., Polle, A. (2021). Soil layers matter: vertical stratification of root-associated fungal assemblages in temperate forests reveals differences in habitat colonization. Microorganisms 9, 2131. doi: 10.3390/microorganisms9102131
Kiers, E. T., Duhamel, M., Beesetty, Y., Mensah, J. A., Franken, O., Verbruggen, E., et al. (2011). Reciprocal rewards stabilize cooperation in the mycorrhizal symbiosis. Science 333, 880–882. doi: 10.1126/science.1208473
King, W. L., Yates, C. F., Cao, L., O'rourke-Ibach, S., Fleishman, S. M., Richards, S. C., et al. (2023). Functionally discrete fine roots differ in microbial assembly, microbial functional potential, and produced metabolites. Plant Cell Environ. 46, 3919–3932. doi: 10.1111/pce.14705
Kong, D., Ma, C., Zhang, Q., Li, L., Chen, X., Zeng, H., et al. (2014). Leading dimensions in absorptive root trait variation across 96 subtropical forest species. New Phytol. 203, 863–872. doi: 10.1111/nph.12842
Korenblum, E., Massalha, H., Aharoni, A. (2022). Plant–microbe interactions in the rhizosphere via a circular metabolic economy. Plant Cell 34, 3168–3182. doi: 10.1093/plcell/koac163
Kozlowski, T. T., Pallardy, S. G. (2002). Acclimation and adaptive responses of woody plants to environmental stresses. Bot. Rev. 68, 270–334. doi: 10.1663/0006-8101
Kumar, M., Mishra, S., Dixit, V., Kumar, M., Agarwal, L., Chauhan, P. S., et al. (2016). Synergistic effect of Pseudomonas putida and Bacillus amyloliquefaciens ameliorates drought stress in chickpea (Cicer arietinum L.). Plant Signal. Behav. 11, e1071004. doi: 10.1080/15592324.2015.1071004
Langley, J. A., McKinley, D. C., Wolf, A. A., Hungate, B. A., Drake, B. G., Megonigal, J. P. (2009). Priming depletes soil carbon and releases nitrogen in a scrub-oak ecosystem exposed to elevated CO2. Soil Biol. Biochem. 41, 54–60. doi: 10.1016/j.soilbio.2008.09.016
Leuschner, C., Tückmantel, T., Meier, I. C. (2022). Temperature effects on root exudation in mature beech (Fagus sylvatica L.) forests along an elevational gradient. Plant Soil 481, 147–163. doi: 10.1007/s11104-022-05629-5
Li, F. L., McCormack, M. L., Liu, X., Hu, H., Feng, D. F., Bao, W. K., et al. (2020). Vertical fine-root distributions in five subalpine forest types shifts with soil properties across environmental gradients. Plant Soil 456, 129–143. doi: 10.1007/s11104-020-04706-x
Li, Q., Yan, L., Ye, L., Zhou, J., Zhang, B., Peng, W., et al. (2018). Chinese black truffle (Tuber indicum) alters the ectomycorrhizosphere and endoectomycosphere microbiome and metabolic profiles of the host tree Quercus aliena. Front. Microbiol. 9. doi: 10.3389/fmicb.2018.02202
Li, W., Shi, Y., Zhu, D., Wang, W., Liu, H., Li, J., et al. (2021). Fine root biomass and morphology in a temperate forest are influenced more by the nitrogen treatment approach than the rate. Ecol. Indic. 130, 108031. doi: 10.1016/j.ecolind.2021.108031
Lindahl, B. D., Ihrmark, K., Boberg, J., Trumbore, S. E., Högberg, P., Stenlid, J., et al. (2007). Spatial separation of litter decomposition and mycorrhizal nitrogen uptake in a boreal forest. New Phytol. 173, 611–620. doi: 10.1111/j.1469-8137.2006.01936.x
Liu, B., Han, F., Ning, P., Li, H., Rengel, Z. (2022). Root traits and soil nutrient and carbon availability drive soil microbial diversity and composition in a northern temperate forest. Plant Soil 479, 281–299. doi: 10.1007/s11104-022-05516-z
Liu, B., Li, H., Zhu, B., Koide, R. T., Eissenstat, D. M., Guo, D. (2015). Complementarity in nutrient foraging strategies of absorptive fine roots and arbuscular mycorrhizal fungi across 14 coexisting subtropical tree species. New Phytol. 208, 125–136. doi: 10.1111/nph.13434
Lozano, Y. M., Aguilar-Trigueros, C. A., Ospina, J. M., Rillig, M. C. (2022). Drought legacy effects on root morphological traits and plant biomass via soil biota feedback. New Phytol. 236, 222–234. doi: 10.1111/nph.18327
Luo, X., Gong, Y., Xu, F., Wang, S., Tao, Y., Yang, M. (2023). Soil horizons regulate bacterial community structure and functions in Dabie Mountain of the East China. Sci. Rep. 13, 15866. doi: 10.1038/s41598-023-42981-7
Lustenhouwer, N., Maynard, D. S., Bradford, M. A., Lindner, D. L., Oberle, B., Zanne, A. E., et al. (2020). A trait-based understanding of wood decomposition by fungi. Proc. Natl. Acad. Sci. U.S.A. 117, 11551–11558. doi: 10.1073/pnas.1909166117
Lv, C., Wang, C., Li, Y., Zhou, Z. (2023). Coordination among root exudation C, mycorrhizal colonization, and functional traits and their responses to drought in five temperate tree species. For. Ecol. Manage. 546, 121316. doi: 10.1016/j.foreco.2023.121316
Ma, Z., Guo, D., Xu, X., Lu, M., Bardgett, R. D., Eissenstat, D. M., et al. (2018). Evolutionary history resolves global organization of root functional traits. Nature 555, 94–97. doi: 10.1038/nature25783
Makita, N., Hirano, Y., Mizoguchi, T., Kominami, Y., Dannoura, M., Ishii, H., et al. (2011). Very fine roots respond to soil depth: biomass allocation, morphology, and physiology in a broad-leaved temperate forest. Environ. Res. 26, 95–104. doi: 10.1007/s11284-010-0764-5
Marañón-Jiménez, S., Radujković, D., Verbruggen, E., Grau, O., Cuntz, M., Peñuelas, J., et al. (2021). Shifts in the abundances of saprotrophic and ectomycorrhizal fungi with altered leaf litter inputs. Front. Plant Sci. 12. doi: 10.3389/fpls.2021.682142
Martin, F., Aerts, A., Ahren, D., Brun, A., Danchin, E. G. J., Duchaussoy, F., et al. (2008). The genome of Laccaria bicolor provides insights into mycorrhizal symbiosis. Nature 452, 88–92. doi: 10.1038/nature06556
Massalha, H., Korenblum, E., Tholl, D., Aharoni, A. (2017). Small molecules below-ground: The role of specialized metabolites in the rhizosphere. Plant J. 90, 788–807. doi: 10.1111/tpj.13543
McGuire, K. L., Allison, S. D., Fierer, N., Treseder, K. K. (2013a). Ectomycorrhizal-dominated boreal and tropical forests have distinct fungal communities, but analogous spatial patterns across soil horizons. PloS One 8, e68278. doi: 10.1371/journal.pone.0068278
McGuire, K. L., Payne, S. G., Palmer, M. I., Gillikin, C. M., Keefe, D., Kim, S. J., et al. (2013b). Digging the New York City skyline: soil fungal communities in green roofs and city parks. PloS One 8, e58020. doi: 10.1371/journal.pone.0058020
Meier, I. C., Finzi, A. C., Phillips, R. P. (2017). Root exudates increase N availability by stimulating microbial turnover of fast-cycling N pools. Soil Biol. Biochem. 106, 119–128. doi: 10.1016/j.soilbio.2016.12.004
Meier, I. C., Pritchard, S. G., Brzostek, E. R., McCormack, M. L., Phillips, R. P. (2015). Rhizosphere and hyphosphere differ in their impacts on carbon and nitrogen cycling in forests exposed to elevated CO2. New Phytol. 205, 1164e1174. doi: 10.1111/nph.13122
Meier, I. C., Tückmantel, T., Heitkötter, J., Müller, K., Preusser, S., Wrobel, T. J., et al. (2020). Root exudation of mature beech forests across a nutrient availability gradient: the role of root morphology and fungal activity. New Phytol. 226, 583–594. doi: 10.1111/nph.16389
Mommer, L., Kirkegaard, J., van Ruijven, J. (2016). Root–root interactions: towards a rhizosphere framework. Trends Plant Sci. 21, 209–217. doi: 10.1016/j.tplants.2016.01.009
Moyersoen, B., Fitter, A. H., Alexander, I. J. (1998). Spatial distribution of ectomycorrhizas and arbuscular mycorrhizas in Korup National Park rain forest, Cameroon, in relation to edaphic parameters. New Phytol. 139, 311–320. doi: 10.1046/j.1469-8137.1998.00190.x
Mueller, K. E., Hobbie, S. E., Chorover, J., Reich, P. B., Eisenhauer, N., Castellano, M. J., et al. (2015). Effects of litter traits, soil biota, and soil chemistry on soil carbon stocks at a common garden with 14 tree species. Biogeochemistry 123, 313–327. doi: 10.1007/s10533-015-0083-6
Mundra, S., Kjønaas, O. J., Morgado, L. N., Krabberød, A. K., Ransedokken, Y., Kauserud, H. (2021). Soil depth matters: shift in composition and inter-kingdom co-occurrence patterns of microorganisms in forest soils. FEMS Microbiol. Ecol. 97, fiab022. doi: 10.1093/femsec/fiab022
Nakayama, M., Tateno, R. (2018). Solar radiation strongly influences the quantity of forest tree root exudates. Trees 32, 871–879. doi: 10.1007/s00468-018-1685-0
Nash, J. M., Diggs, F. M., Yanai, R. D. (2022). Length and colonization rates of roots associated with arbuscular or ectomycorrhizal fungi decline differentially with depth in two northern hardwood forests. Mycorrhiza 32, 213–219. doi: 10.1007/s00572-022-01071-8
Neumann, G., Römheld, V. (2007). “The release of root exudates as affected by the plant physiological status,” in The rhizosphere: biochemistry and organic substances at the soil-plant interface, vol. 2 . Eds. Pinton, R., Zeno, V., Paolo, N. (CRC Press, Boca Raton, FL, USA), 23–72.
Neville, J., Tessier, J. L., Morrison, I., Scarratt, J., Canning, B., Klironomos, J. N. (2002). Soil depth distribution of ecto- and arbuscular mycorrhizal fungi associated with Populus tremuloides within a 3-year-old boreal forest clear-cut. Appl. Soil Ecol. 19, 209–216. doi: 10.1016/S0929-1393(01)00193-7
Nguyen, C. (2003). Rhizodeposition of organic C by plants: mechanisms and controls. Agronomie 23, 375–396. doi: 10.1051/agro:2003011
Norby, R. J., Jackson, R. B. (2000). Root dynamics and global change seeking an ecosystem perspective. New Phytol. 147, 3–12. doi: 10.1046/j.1469-8137.2000.00676.x
O'Brien, H. E., Parrent, J. L., Jackson, J. A., Moncalvo, J. M., Vilgalys, R. (2005). Fungal community analysis by large-scale sequencing of environmental samples. Appl. Environ. Microbiol. 71, 5544–5550. doi: 10.1128/AEM.71.9.5544-5550.2005
Ochoa-Hueso, R., Collins, S. L., Delgado-Baquerizo, M., Hamonts, K., Pockman, W. T., Sinsabaugh, R. L., et al. (2018). Drought consistently alters the composition of soil fungal and bacterial communities in grasslands from two continents. Glob. Change Biol. 24, 2818–2827. doi: 10.1111/gcb.14113
Ofek-Lalzar, M., Sela, N., Goldman-Voronov, M., Green, S. J., Hadar, Y., Minz, D. (2014). Niche and host-associated functional signatures of the root surface microbiome. Nat. Commun. 5, 4950. doi: 10.1038/ncomms5950
Olmo, M., Lopez-Iglesias, B., Villar, R. (2014). Drought changes the structure and elemental composition of very fine roots in seedlings of ten woody tree species. Implications drier climate. Plant Soil 384, 113–129. doi: 10.1007/s11104-014-2178-6
Oppenheimer-Shaanan, Y., Jakoby, G., Starr, M. L., Karliner, R., Eilon, G., Itkin, M., et al. (2022). A dynamic rhizosphere interplay between tree roots and soil bacteria under drought stress. eLife 11, e79679. doi: 10.7554/eLife.79679.sa2
Osbourn, A. E. (2003). Saponins in cereals. Phytochemistry 62, 1–4. doi: 10.1016/S0031-9422(02)00393-X
Pan, J., Wu, H., Xiang, W., Ouyang, S., Chen, L., Zeng, Y., et al. (2023). Soil microbial richness and community composition are primarily mediated by functional trait diversity of fine roots in subtropical forests. Plant Soil. doi: 10.1007/s11104-023-06408-6
Pang, Y., Tian, J., Yang, H., Zhang, K., Wang, D. (2022). Responses of fine roots at different soil depths to different thinning intensities in a secondary forest in the Qinling Mountains, China. Biology 11, 351. doi: 10.3390/biology11030351
Paredes, S. H., Lebeis, S. L. (2016). Giving back to the community: Microbial mechanisms of plant–soil interactions. Funct. Ecol. 30, 1043–1052. doi: 10.1111/1365-2435.12684
Pascale, A., Silvia, P., Iakovos, S. P., Ioannis, A. S. (2020). Modulation of the root microbiome by plant molecules: the basis for targeted disease suppression and plant growth promotion. Front. Plant Sci. 10. doi: 10.3389/fpls.2019.01741
Pausch, J., Kuzyakov, Y. (2018). Carbon input by roots into the soil: Quantification of rhizodeposition from root to ecosystem scale. Glob. Change Biol. 24, 1–12. doi: 10.1111/gcb.13850
Paź-Dyderska, S., Dyderski, M. K., Nowak, K., Jagodziński, A. M. (2020). On the sunny side of the crown – quantification of intra-canopy SLA variation among 179 taxa. For. Ecol. Manage. 472, 118254. doi: 10.1016/j.foreco.2020.118254
Paź-Dyderska, S., Jagodziński, A. M. (2023). In search of a perfect trait set: A workflow presentation based on the conservation status assessment of Poland’s dendroflora. Ecol. Evol. 13, e9979. doi: 10.1002/ece3.9979
Pena, R., Lang, C., Lohaus, G., Boch, S., Schall, P., Schöning, I., et al. (2017). Phylogenetic and functional traits of ectomycorrhizal assemblages in top soil from different biogeographic regions and forest types. Mycorrhiza 27, 233–245. doi: 10.1007/s00572-016-0742-z
Pérez-Ramos, I. M., Matías, L., Gómez-Aparicio, L., Godoy, O. (2019). Functional traits and phenotypic plasticity modulate species coexistence across contrasting climatic conditions. Nat. Commun. 10, 2555. doi: 10.1038/s41467-019-10453-0
Philippot, L., Raaijmakers, J. M., Lemanceau, P., van der Putten, W. H. (2013). Going back to the roots: The microbial ecology of the rhizosphere. Nat. Rev. Microbiol. 11, 789–799. doi: 10.1038/nrmicro3109
Phillips, R. P., Brzostek, E., Midgley, M. G. (2013). The mycorrhizal-associated nutrient economy: a new framework for predicting carbon–nutrient couplings in temperate forests. New Phytol. 199, 41–51. doi: 10.1111/nph.12221
Phillips, R. P., Meier, I. C., Bernhardt, E. S., Grandy, A. S., Wickings, K., Finzi, A. C. (2012). Roots and fungi accelerate carbon and nitrogen cycling in forests exposed to elevated CO2. Ecol. Lett. 15, 1042–1049. doi: 10.1111/j.1461-0248.2012.01827.x
Prada-Salcedo, L. D., Prada-Salcedo, J. P., Heintz-Buschart, A., Buscot, F., Goldmann, K. (2022). Effects of tree composition and soil depth on structure and functionality of belowground microbial communities in temperate European forests. Front. Microbiol. 13. doi: 10.3389/fmicb.2022.920618
Prada-Salcedo, L. D., Wambsganss, J., Bauhus, J., Buscot, F., Goldmann, K. (2021). Low root functional dispersion enhances functionality of plant growth by influencing bacterial activities in European forest soils. Environ. Microbiol. 23, 1889–1906. doi: 10.1111/1462-2920.15244
Prescott, C. E., Grayston, S. J. (2013). Tree species influence on microbial communities in litter and soil: Current knowledge and research needs. For. Ecol. Manage. 309, 19–27. doi: 10.1016/j.foreco.2013.02.034
Prescott, C. E., Grayston, S. J. (2023). TAMM review: Continuous root forestry—Living roots sustain the belowground ecosystem and soil carbon in managed forests. For. Ecol. Manage. 532, 120848. doi: 10.1016/j.foreco.2023.120848
Proctor, C., He, Y. (2021). Modeling root exudate accumulation gradients to estimate net exudation rates by peatland soil depth. Plants 10, 106. doi: 10.3390/plants10010106
Rawlik, K., Kasprowicz, M., Nowiński, M., Jagodziński, A. M. (2022). The after life of herbaceous plant species: A litter decomposition experiment in a temperate oak-hornbeam forest. For. Ecol. Manage. 507, 120008. doi: 10.1016/j.foreco.2022.120008
Rekha, K., Baskar, B., Srinath, S., Usha, B. (2018). Plant-growth-promoting rhizobacteria Bacillus subtilis RR4 isolated from rice rhizosphere induces Malic acid biosynthesis in rice roots. Can. J. Microbiol. 64, 20–27. doi: 10.1139/cjm-2017-0409
Rineau, F., Garbaye, J. (2009). Effects of liming on ectomycorrhizal community structure in relation to soil horizons and tree hosts. Fungal Ecol. 2, 103–109. doi: 10.1016/j.funeco.2009.01.006
Rocha, R. O., Morais, J. K., Oliveira, J. T., Oliveira, H. D., Sousa, D. O., Souza, C. E., et al. (2015). Proteome of soybean seed exudates contains plant defense-related proteins active against the root-knot nematode Meloidogyne incognita. J. Agric. Food Chem. 63, 5335–5343. doi: 10.1021/acs.jafc.5b01109
Rudrappa, T., Czymmek, K. J., Paré, P. W., Bais, H. P. (2008). Root-secreted Malic acid recruits beneficial soil bacteria. Plant Physiol. 148, 1547–1556. doi: 10.1104/pp.108.127613
Ruiz-Lozano, J. M., Aroca, R., Zamarreño, A. M., Molina, S., Andreo-Jiménez, B., Porcel, R., et al. (2016). Arbuscular mycorrhizal symbiosis induces strigolactone biosynthesis under drought and improves drought tolerance in lettuce and tomato. Plant Cell Environ. 39, 441–452. doi: 10.1111/pce.12631
Rumpel, C., Kögel-Knabner, I. (2011). Deep soil organic matter – a key but poorly understood component of terrestrial C cycle. Plant Soil 338, 143–158. doi: 10.1007/s11104-010-0391-5
Sagova-Mareckova, M., Zadorova, T., Penizek, V., Omelka, M., Tejnecky, V., Pruchova, P., et al. (2016). The structure of bacterial communities along two vertical profiles of a deep colluvial soil. Soil Biol. Biochem. 101, 65–73. doi: 10.1016/j.soilbio.2016.06.026
Sasse, J., Martinoia, E., Northen, T. (2018). Feed your friends: Do plant exudates shape the root microbiome? Trends Plant Sci. 23, 25–41. doi: 10.1016/j.tplants.2017.09.003
Schenk, H. J. (2005). Vertical vegetation structure below ground: scaling from root to globe. Prog. Bot. 66, 341e373. doi: 10.1007/3-540-27043-4_14
Schlaeppi, K., Dombrowski, N., Oter, R. G., van Themaat, E. V. L., Schulze-Lefert, P. (2014). Quantitative divergence of the bacterial root microbiota in Arabidopsis thaliana relatives. Proc. Natl. Acad. Sci. U.S.A. 111, 585–592. doi: 10.1073/pnas.1321597111
Schleppi, P., Bucher-Wallin, I., Hagedorn, F., Körner, C. (2012). Increased nitrate availability in the soil of a mixed mature temperate forest subjected to elevated CO2 concentration (canopy FACE). Glob. Change Biol. 18, 757–768. doi: 10.1111/j.1365-2486.2011.02559.x
Semchenko, M., Leff, J. W., Lozano, Y. M., Saar, S., Davison, J., Wilkinson, A., et al. (2018). Fungal diversity regulates plant-soil feedbacks in temperate grassland. Sci. Adv. 4, eaau4578. doi: 10.1126/sciadv.add4468
Shao, P., Liang, C., Rubert-Nason, K., Li, X., Xie, H., Bao, X. (2019). Secondary successional forests undergo tightly-coupled changes in soil microbial community structure and soil organic matter. Soil Biol. Biochem. 128, 56–65. doi: 10.1016/j.soilbio.2018.10.004
Shen, K., Cornelissen, J. H. C., Wang, Y., Wu, C., He, Y., Ou, J., et al. (2020). AM fungi alleviate phosphorus limitation and enhance nutrient competitiveness of invasive plants via mycorrhizal networks in karst areas. Front. Ecol. Evol. 8. doi: 10.3389/fevo.2020.00125
Shi, S., Richardson, A. E., O'Callaghan, M., DeAngelis, K. M., Jones, E. E., Stewart, A., et al. (2011). Effects of selected root exudate components on soil bacterial communities. FEMS Microbiol. Ecol. 77, 600–610. doi: 10.1111/j.1574-6941.2011.01150.x
Staszel-Szlachta, K., Lasota, J., Szlachta, A., Błońska, E. (2024). The impact of root systems and their exudates in different tree species on soil properties and microorganisms in a temperate forest ecosystem. BMC Plant Biol. 24, 45. doi: 10.1186/s12870-024-04724-2
Steidinger, B. S., Crowther, T. W., Liang, J., Van Nuland, M. E., Werner, G. D. A., Reich, P. B., et al. (2019). Climatic controls of decomposition drive the global biogeography of forest-tree symbioses. Nature 569, 404–408. doi: 10.1038/s41586-019-1128-0
Stringlis, I. A., de Jonge, R., Pieterse, C. M. J. (2019). The age of coumarins in plant–microbe interactions. Plant Cell Physiol. 60, 1405–1419. doi: 10.1093/pcp/pcz076
Stringlis, I. A., Yu, K., Feussner, K., de Jonge, R., Van Bentum, S., Van Verk, M. C., et al. (2018). MYB72-dependent coumarin exudation shapes root microbiome assembly to promote plant health. Proc. Natl. Acad. Sci. U S A. 29, E5213–E5222. doi: 10.1073/pnas.1722335115
Subramanian, S., Stacey, G., Yu, O. (2007). Distinct, crucial roles of flavonoids during legume nodulation. Trends Plant Sci. 12, 282–285. doi: 10.1016/j.tplants.2007.06.006
Sun, L., Ataka, M., Han, M., Han, Y., Gan, D., Xu, T., et al. (2021). Root exudation as a major competitive fine-root functional trait of 18 coexisting species in a subtropical forest. New Phytol. 229, 259–271. doi: 10.1111/nph.16865
Sun, L., Ataka, M., Kominami, Y., Yoshimura, K. (2017). Relationship between fine-root exudation and respiration of two Quercus species in a Japanese temperate forest. Tree Physiol. 37, 1011–1020. doi: 10.1093/treephys/tpx026
Talbot, J. M., Treseder, K. K. (2010). Controls over mycorrhizal uptake of organic nitrogen. Pedobiologia 53, 169–179. doi: 10.1016/j.pedobi.2009.12.001
Tasenkevich, L., Boratyński, A., Skrypec, K., Seniv, M., Khmil, T., Walas, L. (2023). Biodiversity of high-mountain woody plants in the east Carpathians in Ukraine. Dendrobiology 89, 1–19. doi: 10.12657/denbio.089.001
Timm, C. M., Carter, K. R., Carrell, A. A., Jun, S.-R., Jawdy, S., Vélez, J. M., et al. (2018). Abiotic stresses shift belowground Populus-associated bacteria toward a core stress microbiome. mSystems 3, e00070–e00017. doi: 10.1128/mSystems.00070-17
Trocha, L. K., Bułaj, B., Kutczyńska, P., Mucha, J., Rutkowski, P., Zadworny, M. (2017). The interactive impact of root branch order and soil genetic horizon on root respiration and nitrogen concentration. Tree Physiol. 37, 1055–1068. doi: 10.1093/treephys/tpx096
Tückmantel, T., Leuschner, C., Preusser, S., Kandeler, E., Angst, G., Mueller, C. W., et al. (2017). Root exudation patterns in a beech forest: dependence on soil depth, root morphology, and environment. Soil Biol. Biochem. 107, 188–197. doi: 10.1016/j.soilbio.2017.01.006
Vandeputte, O. O., Kiendrebeogo, M., Rasamiravaka, T. (2011). The flavanone naringenin reduces the production of quorum sensing-controlled virulence factors in Pseudomonas aeruginosa PAO1. Microbiology 157, 2120–2132. doi: 10.1099/mic.0.049338-0
van Hees, P. A. W., Rosling, A., Lundström, U. S., Finlay, R. D. (2006). The biogeochemical impact of ectomycorrhizal conifers on major soil elements (Al, Fe, K and Si). Geoderma 136, 364–377. doi: 10.1016/j.geoderma.2006.04.001
Veach, A. M., Chen, H., Yang, Z. K., Labbe, A. D., Engle, N. L., Tschaplinski, T. J., et al. (2020). Plant hosts modify belowground microbial community response to extreme drought. mSystems 5, e00092–e00020. doi: 10.1128/mSystems.00092-20
Venturi, V., Keel, C. (2016). Signaling in the rhizosphere. Trends Plant Sci. 21, 187–198. doi: 10.1016/j.tplants.2016.01.005
Viet, H. D. X., Tymińska-Czabańska, L., Socha, J. (2022). Drivers of site productivity for oak in Poland. Dendrobiology 88, 81–93. doi: 10.12657/denbio.088.006
Voges, M. J. E. E. E., Bai, Y., Schulze-Lefert, P., Sattely, E. S. (2019). Plant-derived coumarins shape the composition of an arabidopsis synthetic root microbiome. Proc. Natl. Acad. Sci. U.S.A. 116, 12558–12565. doi: 10.1073/pnas.1820691116
Voříšková, J., Brabcová, V., Cajthaml, T., Baldrian, P. (2014). Seasonal dynamics of fungal communities in a temperate oak forest soil. New Phytol. 201, 269–278. doi: 10.1111/nph.12481
Wang, J., Defrenne, C., McCormack, M. L., Yang, L., Tian, D., Luo, Y., et al. (2021c). Fine-root functional trait responses to experimental warming: a global meta-analysis. New Phytol. 230, 1856–1867. doi: 10.1111/nph.17279
Wang, L., Pang, X., Li, N., Qi, K., Huang, J., Yin, C. (2020). Effects of vegetation type, fine and coarse roots on soil microbial communities and enzyme activities in eastern Tibetan plateau. CATENA 194, 104694. doi: 10.1016/j.catena.2020.104694
Wang, Q., Xiao, J., Ding, J., Zou, T., Zhang, Z., Liu, Q., et al. (2021a). Differences in root exudate inputs and rhizosphere effects on soil N transformation between deciduous and evergreen trees. Plant Soil 458, 277–289. doi: 10.1007/s11104-019-04156-0
Wang, Q., Chen, L., Xu, H., Ren, K., Xu, Z., Tang, Y., et al. (2021b). The effects of warming on root exudation and associated soil N transformation depend on soil nutrient availability. Rhizosphere 17, 100263. doi: 10.1016/j.rhisph.2020.100263
Weng, B., Xie, X., Yang, J., Liu, J., Lu, H., Yan, C. (2013). Research on the nitrogen cycle in rhizosphere of Kandelia obovata under ammonium and nitrate addition. Mar. pollut. Bull. 76, 227–240. doi: 10.1016/j.marpolbul.2013.08.034
Will, C., Thürmer, A., Wollherr, A., Nacke, H., Herold, N., Schrumpf, M., et al. (2010). Horizon-specific bacterial community composition of german grassland soils, as revealed by pyrosequencing-based analysis of 16S rRNA genes. Appl. Environ. Microbiol. 76, 6751–6759. doi: 10.1128/AEM.01063-10
Williams, A., de Vries, F. T. (2020). Plant root exudation under drought: implications for ecosystem functioning. New Phytol. 225, 1899–1905. doi: 10.1111/nph.16223
Xia, Z., He, Y., Xu, J., Zhu, Z., Korpelainen, H., Li, C, et al. (2023). Rhizosphere microbe populations but not root traits induced by drought in Populus euphratica males. Soil Ecol. Lett. 5, 220152. doi: 10.1007/s42832-022-0152-4
Yang, H., Zhang, P., Wang, Q., Deng, S., He, X., Zhang, X., et al. (2023). Temperature rather than N availability determines root exudation of alpine coniferous forests on the eastern Tibetan Plateau along elevation gradients. Tree Physiol. 43, 1479–1492. doi: 10.1093/treephys/tpad067
Yoneyama, K. (2019). How do strigolactones ameliorate nutrient deficiencies in plants? Cold Spring Harb. Perspect. Biol. 11, a034686. doi: 10.1101/cshperspect.a034686
Yu, Y., Zhou, Y., Janssens, I. A., Deng, Y., He, X., Liu, L., et al. (2024). Divergent rhizosphere and non-rhizosphere soil microbial structure and function in long-term warmed steppe due to altered root exudation. Glob. Change Biol. 30, e17111. doi: 10.1111/gcb.17111
Zadworny, M., McCormack, M. L., Żytkowiak, R., Karolewski, P., Mucha, J., Oleksyn, J. (2017). Patterns of structural and defense investments in fine roots of Scots pine (Pinus sylvestris L.) across a strong temperature and latitudinal gradient in Europe. Glob. Change Biol. 23, 1218–1231. doi: 10.1111/gcb.13514
Zhao, J., Ye, Y., Sun, X., Shi, L., Chen, X., Guan, Q. (2023). Root exudation patterns of Chinese fir after thinning relating to root characteristics and soil conditions. For. Ecol. Manage. 541, 121068. doi: 10.1016/j.foreco.2023.121068
Keywords: soil microbes, root exudation, soil horizon, root traits, drought, temperature
Citation: Maitra P, Hrynkiewicz K, Szuba A, Jagodziński AM, Al-Rashid J, Mandal D and Mucha J (2024) Metabolic niches in the rhizosphere microbiome: dependence on soil horizons, root traits and climate variables in forest ecosystems. Front. Plant Sci. 15:1344205. doi: 10.3389/fpls.2024.1344205
Received: 25 November 2023; Accepted: 18 March 2024;
Published: 05 April 2024.
Edited by:
Luciano Kayser Vargas, State Secretariat of Agriculture, Livestock and Irrigation, BrazilReviewed by:
Collin M. Timm, Johns Hopkins University, United StatesJulio Javier diez Casero, University of Valladolid, Spain
Copyright © 2024 Maitra, Hrynkiewicz, Szuba, Jagodziński, Al-Rashid, Mandal and Mucha. This is an open-access article distributed under the terms of the Creative Commons Attribution License (CC BY). The use, distribution or reproduction in other forums is permitted, provided the original author(s) and the copyright owner(s) are credited and that the original publication in this journal is cited, in accordance with accepted academic practice. No use, distribution or reproduction is permitted which does not comply with these terms.
*Correspondence: Pulak Maitra, maitrap@man.poznan.pl
†ORCID: Pulak Maitra, orcid.org/0000-0001-7141-1497
Katarzyna Hrynkiewicz, orcid.org/0000-0002-6606-685X
Agnieszka Szuba, orcid.org/0000-0001-6278-3897
Andrzej M. Jagodziński, orcid.org/0000-0001-6899-0985
Jubair Al-Rashid, orchid.org/0000-0002-4918-9145
Dipa Mandal, orcid.org/0009-0008-7874-433X
Joanna Mucha, orcid.org/0000-0002-1290-9639