- 1College of Resources and Environment, Interdisciplinary Research Center for Agriculture Green Development in Yangtze River Basin, Academy of Agricultural Sciences, Institute of Innovation and Entrepreneurship Hanhong College, Southwest University, Chongqing, China
- 2Key Laboratory of Green and Low-carbon Agriculture in Southwest Mountain, Ministry of Agriculture and Rural Affairs, Chongqing, China
- 3State Key Laboratory of Nutrient Use and Management, College of Resources and Environmental Sciences, China Agricultural University, Beijing, China
Introduction: In contemporary agriculture, the substitution of manure for chemical fertilizer based on phosphorus (P) input in vegetable production has led to a significant reduction in P fertilizer application rates, while, the effect of manure substitution rates on soil P transformation and uptake by root remain unclear.
Methods: This research conducts a pot experiment with varying manure substitution rates (0%, 10%, 20%, 30%, 40%, 50%, 75% and 100%) based on P nutrient content to elucidate the mechanisms through which manure substitution affects P uptake in pepper.
Results and discussion: The result showed that shoot and root biomass of pepper gradually increased as manure substitution rate from 10% to 40%, and then gradually decreased with further increases in the substitution rate. Soil alkaline phosphatase activity and arbuscular mycorrhizal (AM) colonization gradually increased with manure substitution rates improvement. Specifically, when the substitution rate reached 30%–40%, the alkaline phosphatase activity increased by 24.5%–33.8% compared to the fertilizer treatment. In contrast, phytase activity and the relative expression of phosphate transporter protein genes in the root system was declined after peaking at 30% manure substitution. Additionally, soil available P remained moderate under 30%–40% substitution rate, which was reduced by 8.6%–10.2% compared to that in chemical fertilizer treatment, while microbial biomass P was comparable. In the current study, soil labile P similar to or even higher than that in chemical fertilizer treatment when the substitution rate was ≤40%. Correlation heatmaps demonstrated a significant and positive relationship between soil available P and factors related to labile P and moderately labile P.
Conclusion: This finding suggested that substituting 30%–40% of chemical P with manure can effectively enhance root length, AM colonization, soil enzyme activity, soil labile P, and consequently improve P uptake in pepper. These findings provide valuable insights for future organic agricultural practices that prioritize P supply, aiming to standardize organic P management in farmland and achieve high crop yields and maintain soil health.
1 Introduction
Phosphorus (P) is a vital element in vegetable production, playing a crucial role in promoting root growth and increasing yields (Li et al., 2016; Wang et al., 2019; Bindraban et al., 2020). The demand for P in crop production in China led to the rapid growth of the P fertilizer industry since the late 20th century (Li et al., 2015). However, the extensive exploitation of P resources within a short timeframe has resulted in a depletion of these resources (Liu et al., 2020b; Gong et al., 2022). Notably, China annually produces approximately 5.7 billion tons of organic materials, equivalent to about 13 million tons of P2O5 (Niu and Ju, 2017), which are increasingly being utilized as substitutes for P in agriculture production, highlighting the importance of improving P recycling in agricultural waste for sustainable agriculture green development (Yi et al., 2021).
Pepper is a globally significant vegetable with high nutritional value, providing essential components such as capsaicin, carotene, and vitamins, which are crucial for a balanced diet (Baenas et al., 2019). China is a major producer and consumer of peppers, accounting for around 40% of the global planted area in 2021 (Food and Agriculture Organization of the United Nations, 2021, http://www.fao.org/faostat/en/). However, intensive vegetable production often leads to the accumulation of excess soil P and increased environmental stress due to the use of chemical P fertilizer. In this respect, many studies have explored the use of organic fertilizers to reduce chemical P fertilizer application and enhance P fertilizer use efficiency (Hua and Zhu, 2020; Zhang et al., 2021d). It has also been shown that various types of organic materials can significantly reduce soil total P leaching losses (Zhang et al., 2021c), underscoring the pivotal role of organic materials in meeting the P demand for vegetable production.
Over the years, numerous studies have demonstrated that the application of organic materials to agricultural land affects soil P biogeochemical processes by altering P forms. It is now understood that unstable P pools are more susceptible to changes due to fertilization (Zhang et al., 2021c). The application of organic materials has been shown to promote the conversion of soil P into labile P or microbial biomass phosphorus (MBP), thereby reducing the deposition of stable P and improving soil P bioavailability (Liu et al., 2020a; Fei et al., 2022). For instance, cattle manure, as a P-efficient organic material, can enhance soil microbial diversity, improve soil aeration and water-holding capacity, and promote pepper yield (Xiang et al., 2022; Hernandez et al., 2023; Wang et al., 2023b). However, the impact of the optimal manure substitution rate on soil P transformation and availability remains uncertain, especially in a red soil with high P fixation capacity.
Crop production, which relies on manure application, responds to root traits and the rhizosphere. An increasing body of evidence suggests that soil organic phosphorus (Po) cycling is highly dependent on the interaction between roots and the rhizosphere (Amadou et al., 2021; Zhang et al., 2023a). Long-term field trials have shown that a combination of organic and inorganic applications can synergistically improve root morphology and enhance the rhizosphere soil environment, including soil enzyme activity and root secretion content, effectively increasing crop yields (Zhang et al., 2023b). There is a rich literature available substantiating that increased organic fertilizer application leads to changes in the rhizosphere microbiome community structure, increased abundance and diversity of bacteria carrying phoD functional genes, and enhanced soil phosphatase activity, which, in turn, drives Po mineralization (Lu et al., 2023a). It has also been suggested that organic fertilizer enhance P effectiveness by inducing changes in soil pH and increasing soil organic carbon (SOC) levels, which release adsorbed P (Nobile et al., 2020). Additionally, the crop root system can improve its capacity to access P through its physiological functions, such as increasing root dry weight, root density, extending fine roots, root hairs, and establishing P uptake channels through symbiosis with AM fungi (Wang and Lambers, 2020; Amadou et al., 2021; Liu, 2021; Wang et al., 2022). However, little is currently known about how root and rhizosphere processes correspond to soil P transformations at varying P replacement levels.
This study aims to test the following hypotheses: (1) Soil labile P pools gradually decrease with increasing levels of manure as a substitute for P fertilizer. (2) The development of the pepper root system initially increases and then decreases with higher levels of manure substitution for P fertilizer. The rhizosphere soil environment improves with manure application and stabilizes with increasing manure application. (3) The interconversion between soil P pools plays a crucial role in driving the crop root system to forage for P. Importantly, we sought to determine the optimal matching ratio that balances crop yield and farmland environment sustainability in vegetable systems and quantify the contributions of soil P transformation and crop root and rhizosphere processes to efficient crop P uptake, elucidating the root-rhizosphere-soil interaction mechanisms underlying manure substitution for chemical P fertilizer.
2 Materials and methods
2.1 Soil and materials
A pot experiment was established in the solar greenhouse at the National Purple Soil Monitoring Station in Beibei, Chongqing, China (29°48’ N, 106°24’ E) during the 2022 growing season (March-June). The selected pepper variety was “Xinxiang No. 8”, a hybrid variety from Jiangxi Nongwang High-tech Limited Company. Cow manure was sourced from Chongqing Zheshu Agricultural Science and Technology Development Limited Company. The basic soil properties were as follows: pH 5.9, soil organic matter 34.7 g·kg-1, total nitrogen 0.71 g·kg-1, Olsen-P 36.1 mg·kg-1, alkaline dissolved nitrogen 132.3 mg·kg-1, and available potassium 65.7 mg·kg-1. The soil was collected from Shilin County, Yunnan Province, characterized as ultisol (US Soil Taxonomy), with a clay texture (Wang et al., 2023b). The basic soil properties were as follows: pH 5.9, soil organic matter 34.7 g·kg-1, total nitrogen 0.71 g·kg-1, Olsen-P 36.1 mg·kg-1, alkaline dissolved nitrogen 132.3 mg·kg-1, and available potassium 5.7 mg·kg-1.
2.2 Experimental design
The experiment comprised nine treatments with pots of 16 cm in diameter and 18 cm in height (Supplementary Figure S1), including a control with no P fertilizer (CK) and eight P fertilizer substitution levels using manure based on the total P content: fully chemical fertilizer (0M), 10% (10M), 20% (20M), 30% (30M), 40% (40M), 50% (50M), 75% (75M), and 100% (100M). Each treatment was replicated four times. Each pot contained 4.0 kg of dry soil, and three uniform seedlings were transplanted into each pot. All treatments received N (200 mg·kg-1 dry soil), P2O5 (550 mg·kg-1 dry soil), and K2O (480 mg·kg-1 dry soil) except for the no P control. The manure application rate was determined based on the total P substitution rate, and any remaining nutrient deficiencies were supplemented with chemical fertilizers (urea, calcium superphosphate, potassium chloride) to achieve the same level. The soil and fertilizer were thoroughly mixed before transplanting. Pepper seeds were disinfected with carbendazim, subsequently wrapped in nutrient-rich paper, and placed in a nutrient solution for seedling cultivation (Liu et al., 2017). After 40 days, uniformly grown pepper seedlings were selected for transplanting and watering, with soil water content maintained at approximately 70% of the field water holding capacity during the experimental period.
2.3 Sample collection and analysis
Samples were collected after 60 days (flowering stage) of potting culture for the following analysis: Plant parts (aboveground and roots) were washed, dried separately with deionized water, and heated at 105°C for 30 minutes to terminate enzymatic activity. Subsequently, they were dried to a constant weight at 65°C, milled using a ball milling machine, and stored for measurement. Soil samples were divided into two portions, with one portion dried in its natural state and sieved through 2 mm and 0.15 mm sieves for the analysis of soil physicochemical properties. The other portion was stored in a refrigerator at -20°C for analysis of soil MBP.
This study conducted the analysis of each indicator following standard soil agrochemical analysis methods. Plants were digested by the HNO3-H2O2 method and analyzed for nutrients (ICP-OES). Root morphology was scanned using a root scanner (Epson Perfection V850 Pro) and imported the data into Win-RHIZO software (Win-RHIZO Pro 2004b, Version 5.0, Canada) for scanning and digitization. Arbuscular mycorrhizal colonization was determined through microscopic observation after staining with Trypan Blue Stain Solution (Giovannetti and Mosse, 1980). The determination of soil properties involved various methods: SOC was determined by the potassium dichromate volumetric method (Bao, 2000); Olsen-P and CaCl2-P were extracted by 0.5 mol·L-1 NaHCO3 (pH 8.5, soil-water ratio 1:20) and 0.01 mol·L-1 CaCl2 (soil-water ratio 1:5) respectively and determined by the molybdovanado phosphatase method (Zhang et al., 2021b). Soil pH was determined by the potentiometric method (1:2.5 w/v in water) (FiveEasy Plus FE28). Soil acid phosphatase (ACP) and alkaline phosphatase (ALP) were determined using the colorimetric method of disodium benzene phosphate. Phytase (S-Phytase) was determined spectrophotometrically. β-glucosidase (S-β-GC) using 3,5-dinitrosalicylic acid (DNS) colorimetric method. MBP was analyzed by chloroform fumigation (Brookes et al., 1982). To categorize soil P forms, we followed the sequential extraction method proposed by Tiessen and Moir (1993), which divided P into nine types (Hedley et al., 1982), including Resin-P, NaHCO3-P, NaOH-P, HCl-P, and Residual-P. Notably, NaHCO3-P, NaOH-P, and HCl-P encompassed both organic and inorganic fractions. Based on the bioavailability of various P fractions, they are classified into four distinct P pools: the labile P pool consists of Resin-P, NaHCO3-Pi and NaHCO3-Po; the moderately labile P pool includes NaOH-Pi, NaOH-Po and dil.HCl-Pi; the sparingly labile P pool is made up of conc.HCl-Pi and conc.HCl-Po; and the Residual-P is considered to be within the non-labile P pool.
Total RNA from pepper root was extracted using the EZ-10 DNAaway RNA extraction kit. RNA integrity and concentration were determined by the NanoPhotometer Analyzer (N50 Touch, Serial No.: T51314) and 1% agarose gel, respectively. The cDNA was synthesized by reverse transcription using PrimeScript TM RT reagent kit with gDNA Eraser (Takara) and subjected to qPCR (StepOnePlus Real-Time PCR System, Applied Biosystems, Q1), Reaction conditions were: 95°C for 10.0 min, followed by 40 cycles of 95°C for 15.0 s, 60°C for 1.0 min. The relative expression levels of the target genes were calculated using the 2−ΔΔCt method relative to reference genes (Livak and Schmittgen, 2001). All the primers used are provided in Supplementary Table S1.
2.4 Data analysis and statistics
The following formula was used to calculate plant P accumulation (Jamal et al., 2023).
All data were analyzed by one-way ANOVA using SPSS 26. In cases where the ANOVA indicated significance, we compared means using the LSD test at a significance level of P < 0.05. All figures in this study were generated using Origin 2023.
3 Results
3.1 Pepper biomass, P concentration, and accumulation response to manure substitution
The aboveground and root biomass of pepper exhibited significant increases under all P treatments compared to the control, with a consistent trend in both plant parts (P < 0.05) (Figure 1). Aboveground biomass showed a substantial increase, ranging from 60.6% to 95.3% in the P application treatments compared to the no P control. Aboveground biomass was similar to the 0M treatment for manure substitution rates below 40%. The peak biomass occurred at the 30% rate, registering 1.15 times higher than that of the 100% manure treatment. However, the aboveground biomass was significantly suppressed at substitution rates exceeding 50%, reaching only 84.7% to 89.9% of the biomass observed with the chemical fertilizer treatment. In contrast, root growth responded differently. Root biomass was promoted to varying degrees at different substitution rates compared to the control, increasing by approximately 14.3% to 42.9%. When compared to the chemical fertilizer treatment, both low substitution rates (≤20%) and the full manure treatment significantly inhibited pepper root growth. Root biomass levels were similar at substitution rates between 30% and 50%, increasing by 4.4% to 11.1% and 17.5% to 25.0% when compared to the 0M and 100M treatments, respectively.
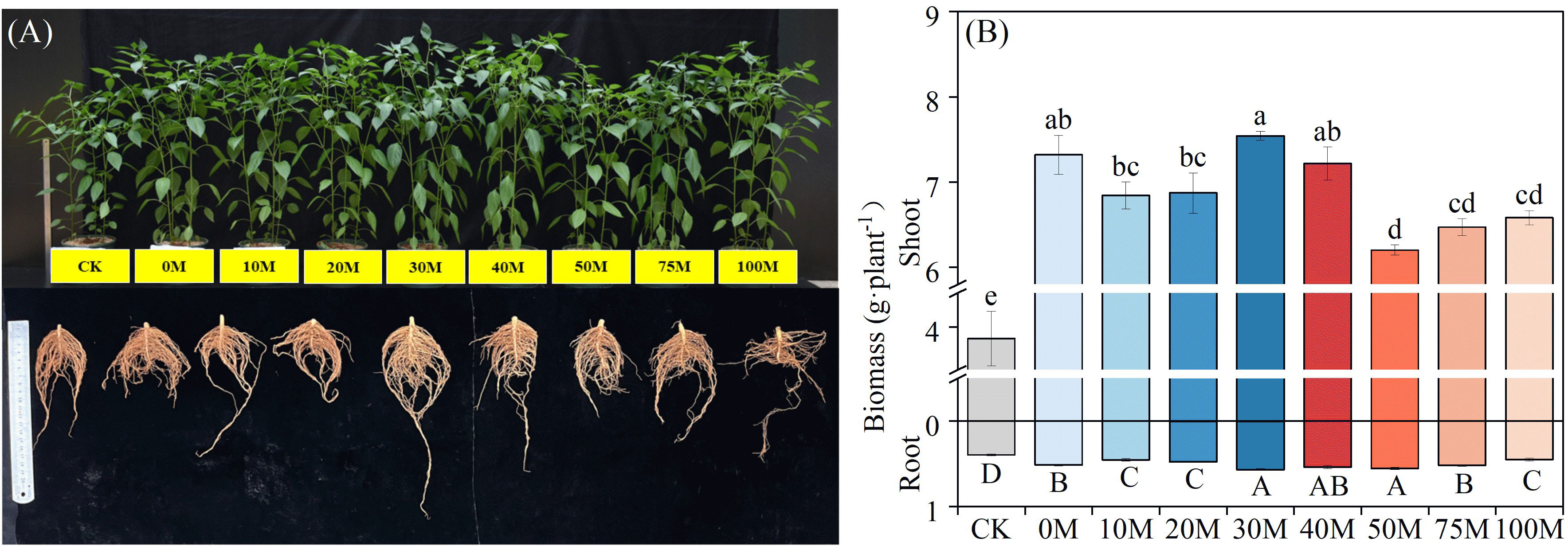
Figure 1 Pepper phenotype (A) and biomass (B) of shoot and root under different manure substitution rates. Different lowercase and uppercase letters indicate significant differences between the aboveground and root of different treatments, respectively (P < 0.05).
Total P concentration in pepper exhibited an overall increasing and then decreasing trend at different substitution rates (P < 0.05) (Figure 2A). The highest concentration was observed under the 40M treatment, which was 9.8% higher than both fully chemical and fully organic fertilizer treatments. Total P accumulation in pepper followed a similar trend as total biomass, with total P uptake under P application treatments being 63.6% to 104.4% higher than the control. The highest pepper P uptake was observed at manure substitution rates of 30% to 40%, significantly exceeding the chemical fertilizer treatment and high substitution rate treatments (50M, 75M, and 100M) (Figure 2B).
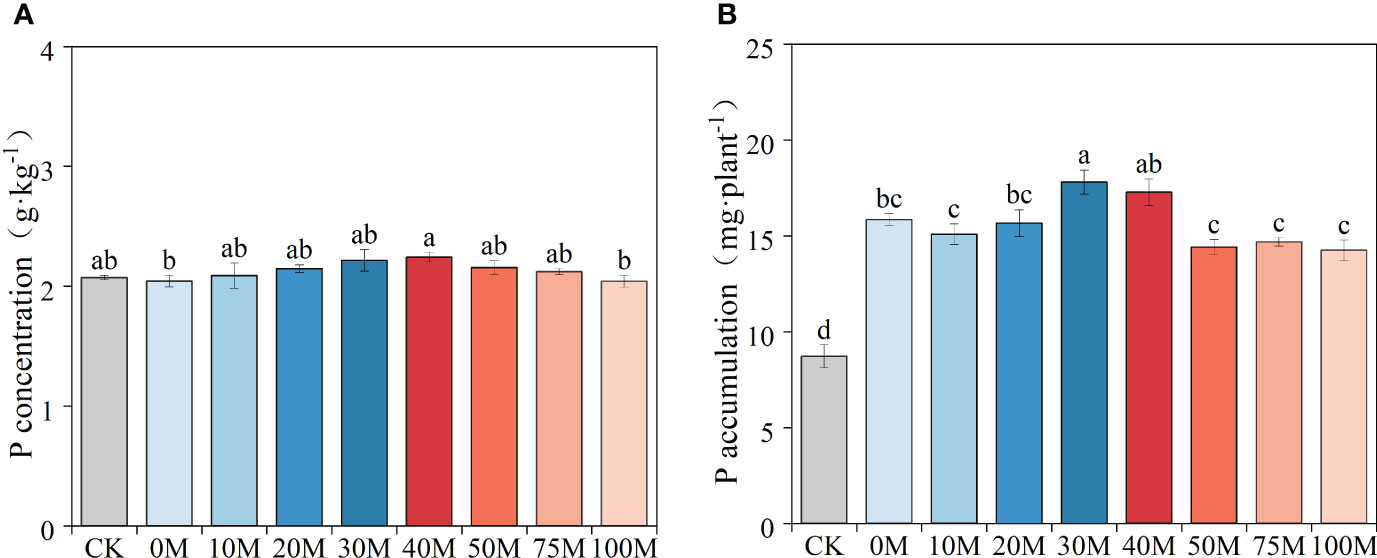
Figure 2 Total P concentration (A) and accumulation (B) under different manure substitution rates. Different lowercase letters indicate significant differences among the different treatments (P < 0.05).
3.2 Changes in root/rhizosphere traits response to manure substitution rate
Table 1 summarizes the differences in pepper root traits under various manure substitution levels. The root length, root surface area, and root diameter under CK treatment were 68.2% to 91.8%, 71.4% to 86.1%, and 63.0% to 96.7% of the P treatment, respectively. Root length and root diameter exhibited an increasing and then decreasing trend with increasing substitution rates, with peak values observed at 30% to 40% substitution rates. Total root length did not differ significantly between the 0M treatment and low substitution rate treatments but was suppressed when the rate exceeded 50%. For example, the 100M treatment displayed only 77.5% and 74.3% of the root length compared to the 0M and 30M treatments, respectively. The application of chemical fertilizer alone significantly reduced root diameter by approximately 32.6% compared to the 40M treatment.
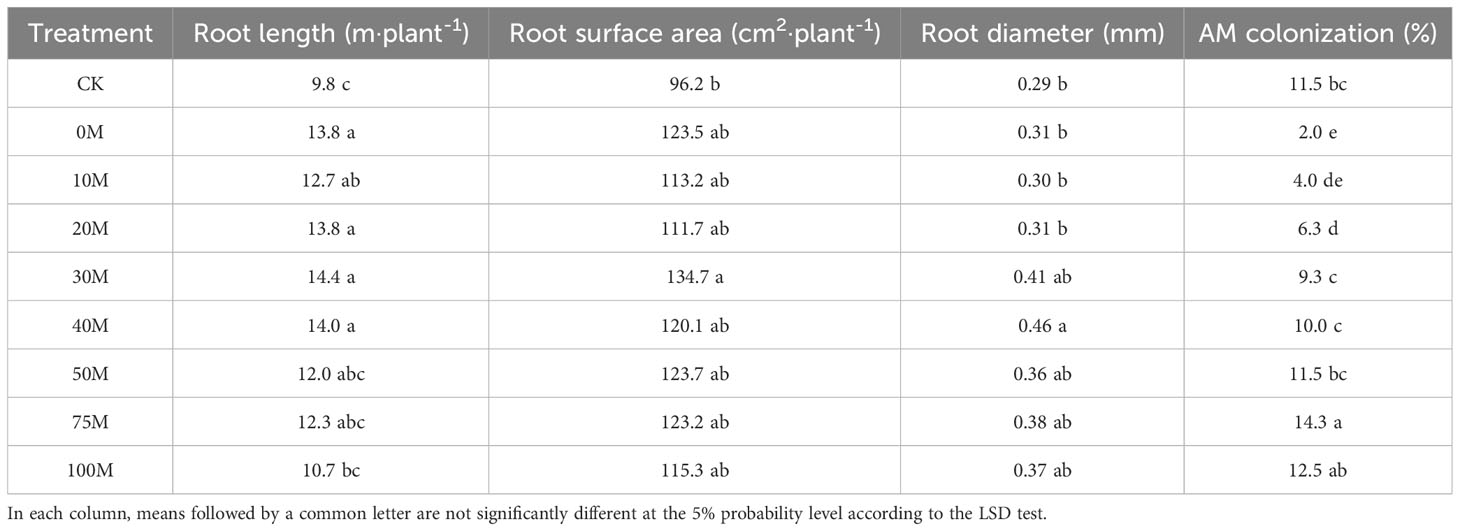
Table 1 Root length, root surface area, root diameter, and arbuscular mycorrhiza colonization of pepper under different manure substitution rates.
The AM colonization was significantly reduced by 82.6% under the 0M treatment compared to the control. Overall, the colonization rate initially increased and then decreased with an increased manure substitution rate. The maximum colonization was observed under the 75M treatment, which increased by 615.0% and 14.4% compared to the 0M and 100M treatments, respectively, with no significant difference between 100M and 75M.
Soil nutrient cycling enzymes exhibited distinct responses to each manure substitution level (Figure 3). Acid phosphatase activity remained unaffected by P fertilizer application and manure substitution rate, while alkaline phosphatase activity gradually increased with increasing substitution rate. Compared to CK, the chemical fertilizer treatment inhibited alkaline phosphatase activity, whereas the manure substitution treatment yielded positive effects. Alkaline phosphatase activity was 1.34 to 1.61-fold higher than the 0M treatment when the substitution rate was 40% or higher. Similarly, phytase activity exhibited inhibition under the 0M treatment. However, phytase activity increased initially and then decreased with the increasing manure application rate, with the highest activity recorded at the 30% substitution rate, showing increases of 125.6% and 200.0% compared to the 0M and 100M treatments, respectively. Soil β-glucosidase activity increased and then stabilized with the increasing substitution rate. Soil β-glucosidase activity under 0M treatment was 21.6% lower than the control. The highest enzyme activity was observed with 30% substitution rate, exhibiting an increase of 16.8% compared to the CK and 48.9% compared to the 0M treatments. In addition, the relative expression of phosphate transporter protein genes (PHT1.1, PHT1.2, and PHT1.6) was upregulated in pepper roots under manure treatments compared to the 0M treatment, with the highest expression levels observed in the 30M treatment. Excessive substitution rates led to decreased expression levels compared to the 30M treatment (Supplementary Figure S2).
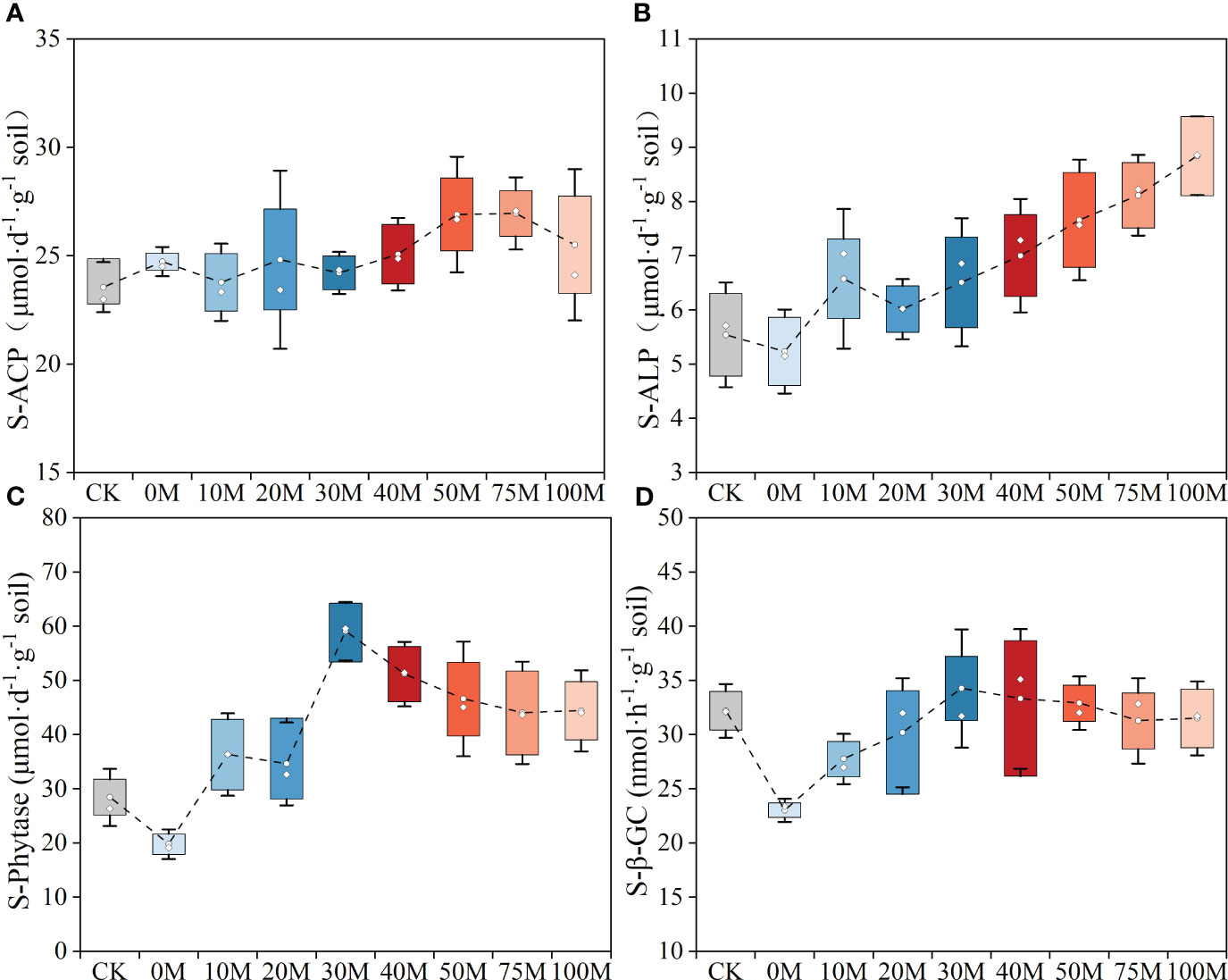
Figure 3 Changes in soil acid phosphatase (A), alkaline phosphatase (B), phytase (C), and β-glucosidase (D) activities under different manure substitution rates. The hollow circle on the box represents the average number of treatments, and the hollow diamond represents the median of each treatment.
3.3 Soil available P and P fractions affected by manure substitution
Soil Olsen-P and CaCl2-P exhibited consistent overall trends (Table 2). P content was significantly higher in all P fertilizer treatments compared to the no P control and decreased gradually with increasing manure substitution rate. Soil Olsen-P content under the 0M treatment was significantly higher than that under the 30% to 100% substitution rate treatments, with increases of 9.4%, 11.4%, 13.5%, 18.5%, and 32.7%, respectively, while it did not differ from the ≤20% substitution rate treatments. CaCl2-P content was highest under the 0M treatment at 1.13 mg·kg-1, which was 4.7 folds higher than the no P control. Compared to the 0M treatment, CaCl2-P levels were comparable when the substitution rate was ≤30%, but they decreased significantly for substitution rates of 40% to 100%, with decreases of 21.2%, 36.3%, 54.9%, and 57.5%, respectively. P fertilizer also yielded a significant enhancement effect on MBP, with soil MBP levels under P fertilizer treatments being 2.2 to 3.2 folds higher than the control. There was no difference in MBP levels under the 0M and 30M treatments, which were significantly higher than the 100M treatment. Furthermore, SOM content increased progressively with increasing substitution rate, with increases of 10.0%, 10.6%, 12.8%, and 12.7% observed when the rate was 40% or higher compared to the 0M treatment. However, there were no difference among all manure treatments for SOM content. Soil pH exhibited significant differences under all treatments. Compared to CK, the application of P fertilizer significantly increased soil pH, with the pH gradually increasing with the manure substitution rate.
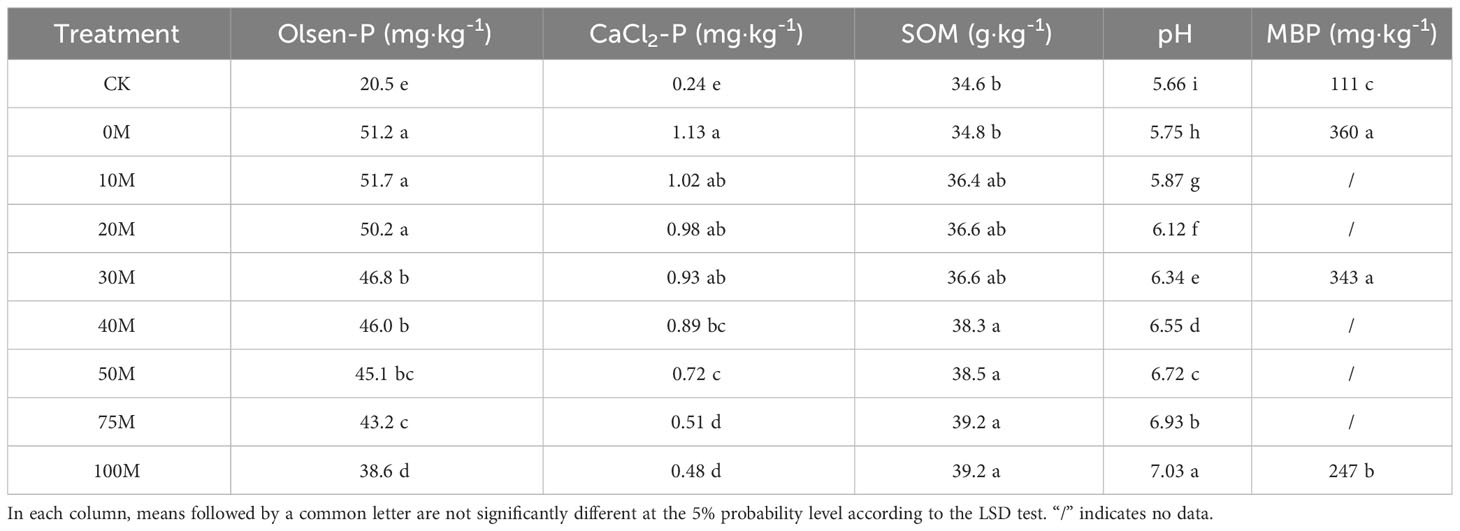
Table 2 Changes in soil Olsen-P, CaCl2-P, SOM, pH, and MBP under different manure substitution rates.
The levels of the nine soil P fractions, based on sequential extraction, differed in response to each substitution rate. Various P forms were categorized into four P pools: labile P, moderately labile P, sparingly labile P, and non-labile P. The P content and percentage of these P pools are shown in Figure 4. All P application treatments increased the content of each soil P pool compared to CK, with the greatest effect on the enhancement of labile P (increasing by 3.0% to 6.3%), while sparingly labile P decreased by 2.5% to 9.0%. Soil labile P and moderately labile P content and percentage decreased gradually with increasing substitution rate, while sparingly labile P and non-labile P exhibited the opposite trend. Soil P forms were primarily dominated by moderately labile P and sparingly labile P, accounting for about 75.9% to 80.7% of the total percentage. There was no reduction in the percentage of the soil labile P pool when the substitution rate was 40% or lower compared to the 0M treatment.
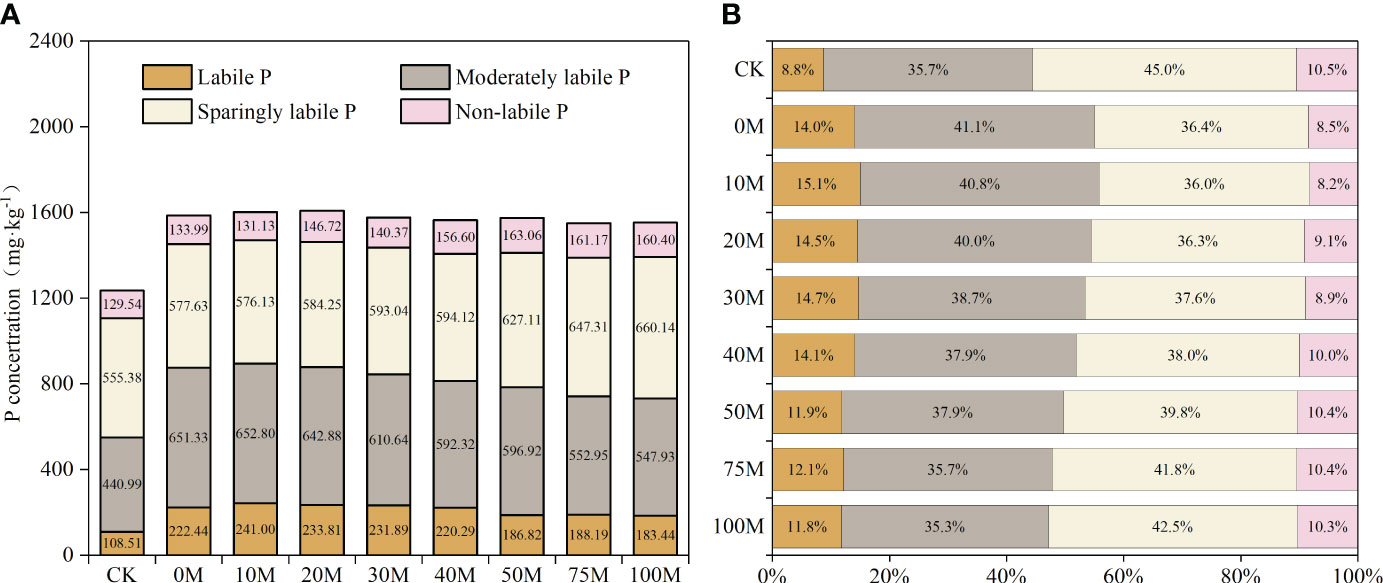
Figure 4 Changes in the content (A) and percentage (B) of four soil P pools at different manure substitution rates.
3.4 Relationship between soil properties and P fractions
As shown in Figure 5, both soil labile P (R2 = 0.44) and moderately labile P (R2 = 0.58) were significantly positively correlated with Olsen-P when fitting soil P pools to available P correlations (P < 0.001), indicating that soil Olsen-P increased with increasing levels of these two P pools. Among the nine P forms, NaOH-Po showed the highest correlation with Olsen-P, with an R2 value of 0.60 (Supplementary Figure S3).
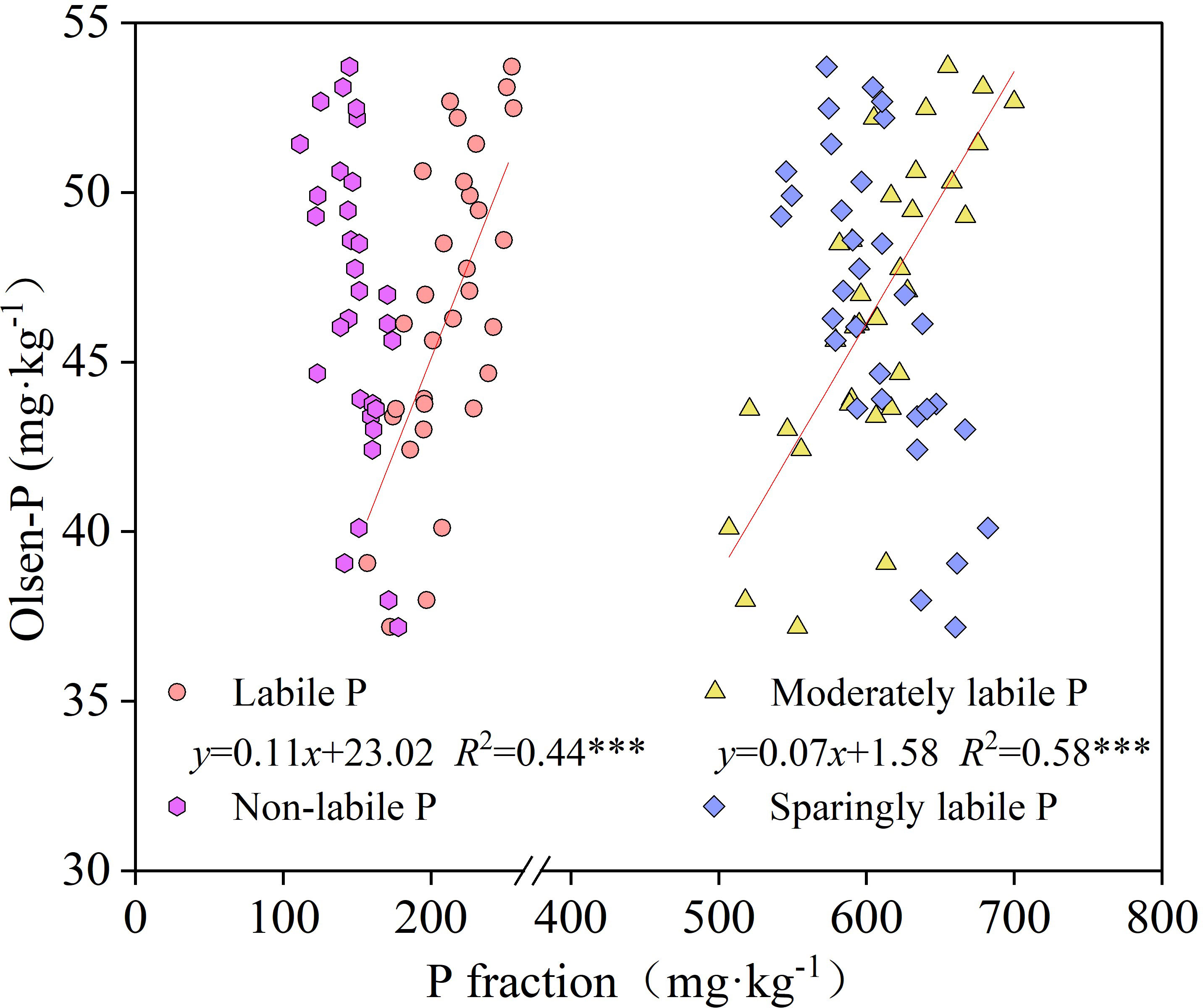
Figure 5 Relationship between soil Olsen-P and the four P pools (*** indicates significant regression at P < 0.001).
The correlation heat map in Figure 6 revealed that soil Olsen-P and CaCl2-P were primarily influenced by Resin-P, NaHCO3-Pi, NaOH-Pi, and NaOH-Po. These four P forms were significantly positively correlated with each other, suggesting their susceptibility to morphological transformations. NaHCO3-Po exhibited a significant negative correlation with some constituents of the sparingly labile P and non-labile P, indicating that NaHCO3-Po is predominantly converted to a highly effective P source for crop uptake after mineralization. Conc.HCl-Pi and conc.HCl-Po, as constituents of the sparingly labile P pool, exhibited an overall positive correlation with other P forms, with the order of positive correlation being Residual-P > dil.HCl-Pi > Resin-P > NaOH-Pi > NaHCO3-Pi. This suggested that conc.HCl-Pi and conc.HCl-Po were more readily immobilized or converted to partially moderately labile P forms. Soil MBP was significantly positively correlated with Olsen-P, while AM colonization showed a negative correlation, indicating a significant antagonistic relationship between P transformation and AM colonization.
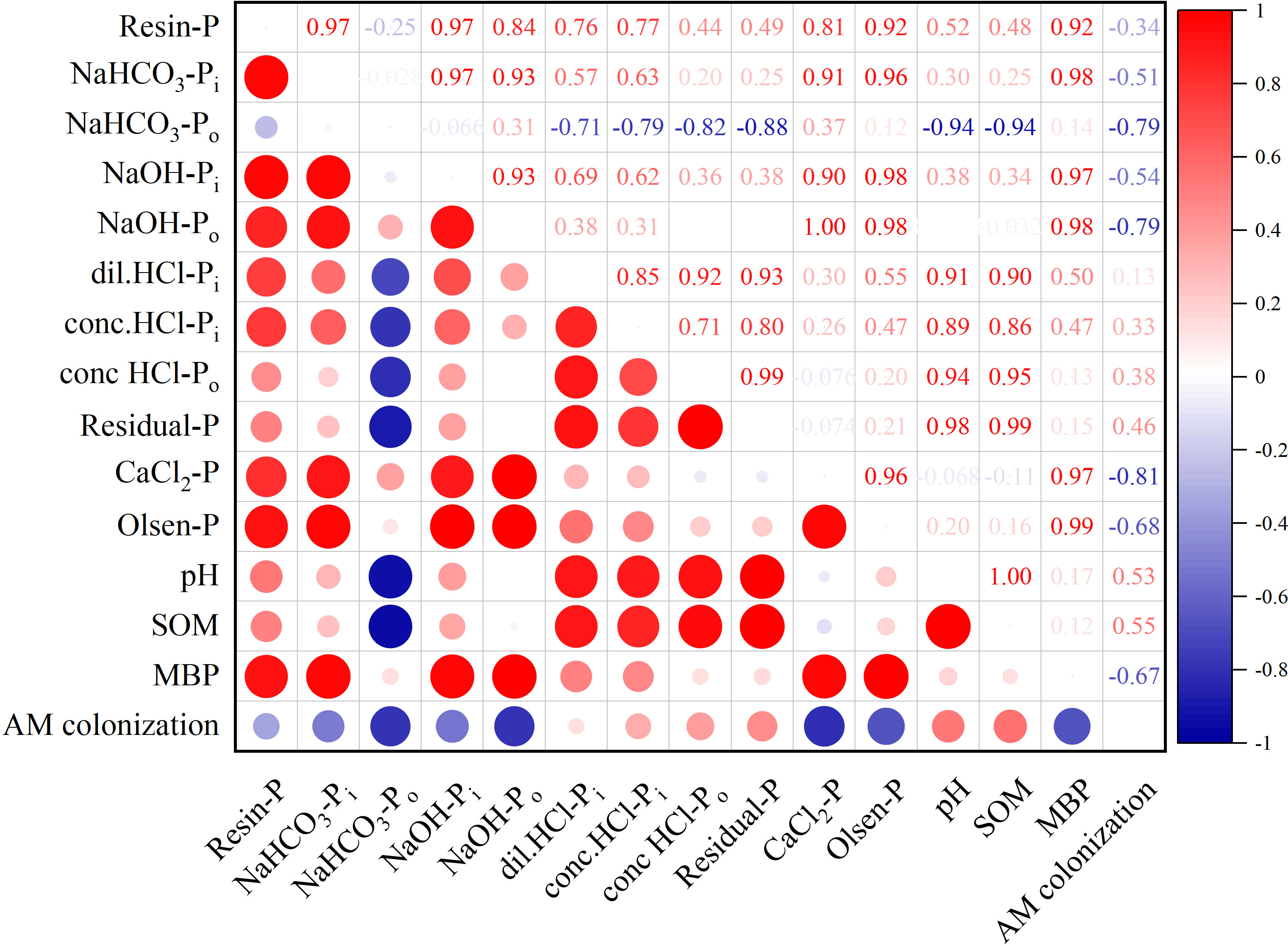
Figure 6 Correlation analysis of four P pools with soil properties (red and blue indicate positive and negative correlations, respectively; the shade of color and the size of the circle indicate the size of the correlation).
4 Discussion
4.1 Effect of manure substitution for chemical P fertilizer on biomass and P absorption of pepper
The substitution rate of manure for chemical fertilizers is a key factor in determining crop yield. In this study, pepper biomass and P uptake with 30%–40% manure substitution were equal to or higher than the chemical P fertilizer treatment (Figures 1, 2). This enhancement was credited to the integrative impact of the fertilization regime on exploiting the root biological potential and advancing soil P turnover. However, exceeding 50% of the total P with manure P significantly reduced both crop biomass and P uptake. This decline was ostensibly linked to a deficiency of labile P concentration, which proved inadequate for sustaining essential root development and nutrient uptake. Previous research on substituting organic fertilizer for nitrogen fertilizer has shown that moderate substitution levels (35%–70%) yield the greatest impact on vegetable yields, while fruiting vegetables benefit from lower substitution rates (<35%) (Li et al., 2021b; Wang et al., 2023a). While, maize crops have shown good growth and development under high manure inputs, improving nutrient use efficiency (Otinga et al., 2013). This variation is attributed to the characteristics of vegetables, such as their shallower root systems and higher nutrient demand, which make them more responsive to external nutrient inputs (Zhang et al., 2010). Current evidence suggests that the uptake of P by crops is mainly in the form of phosphate and is limited by short-term demand, reducing the role of a single P source, whether it is inorganic or organic phosphate. Previous study notes that simply converting to 100% organic production will inevitably lead to increased agricultural land use (Muller et al., 2017), and it can be challenging to maintain crop production even when organic P fertilizer is applied exclusively to cereal crops with well-developed root systems (Andriamananjara et al., 2019). Replacing 40% of P fertilizer with organic P fertilizer has been found to be more effective in improving the soil environment and crop production (Chen et al., 2021). The application of a manure substitution strategy on P can ensure crop production while promoting resource recycling and integrating agriculture and livestock. However, little is currently known on manure substitution based on P fertilizers, nor is it clear whether the manure substitution rate varies under different soil P level environments. Therefore, more research should be conducted to inform regional production practices in future farmland management.
4.2 Root/rhizosphere response under manure substitution strategy
The root-rhizosphere interface represents the most frequent and sensitive area for material exchange between plants and soil, making it essential to understand crop root systems and rhizosphere processes when evaluating fertilization practices (Zhang et al., 2023a). In this study, manure P substitution at 30%–40% of chemical P yielded the most significant positive impact on root traits, and AM colonization increased with higher manure application rates. This phenomenon can be attributed to the regulation of AM colonization by various factors. The application of organic fertilizers has been shown to affect crop nutrient acquisition through the AM pathway, but the effect, whether positive or negative, remains inconclusive (Fornara et al., 2020; Lu et al., 2023b). Furthermore, AM fungi can use carbon sources from organic fertilizers to increase their biomass, and the colonization rate is partly regulated by soil P levels (Jiang et al., 2021). The present study found that AM colonization was regulated by the ratio of organic and inorganic P fertilizers. Excessively low or high manure substitution rates were inhibited by high P levels and carbon oversaturation, respectively, while moderate available P levels and organic carbon inputs created a favorable soil environment for AM colonization and sporulation, in line with the literature (Zhang et al., 2021a).
The application of a combination of organic and inorganic fertilizers is an effective approach to increase soil enzyme activity and accelerate P turnover (Zhang et al., 2022). In the current study, alkaline phosphatase activity increased gradually with higher manure substitution rates, while acid phosphatase was not affected by the fertilization strategy, consistent with previous research (Spohn and Kuzyakov, 2013). Research has shown that acid phosphatase is mainly secreted by living plants, while alkaline phosphatase is significantly influenced by soil microorganisms (Krämer and Green, 2000; Wan et al., 2021). Therefore, under manure substitution measures, the dynamics of soil enzymatic activity may be influenced by changes in soil microbial communities. Notably, phytase activity was suppressed under the fully chemical fertilizer strategy, but it showed an increasing and then decreasing trend with organic management. Another study observed that phytase activity increased to a certain level and then stabilized as manure substitution rates increased (Li et al., 2012). This phenomenon is related to the biological fixation of P by soil microorganisms, with the fixed P primarily consisting of Po, such as DNA and phospholipids (Peng et al., 2021), which is converted into plant-available forms through phytase digestion (Azeem et al., 2015).
4.3 P effectiveness and characterization of P forms under manure substitution strategy
Olsen-P is often used to characterize soil P that is readily available for plant uptake, while CaCl2-P indicates the fraction dissolved in the soil solution (Zhang et al., 2021b). In this study, the 0M and low substitution (≤20%) treatments exhibited similar soil Olsen-P levels. Chemical P fertilizers play a crucial role in increasing soil P effectiveness; however, manure P is released too slowly to meet crop demand. This characteristic of chemical P fertilizers resulted in significantly higher soil CaCl2-P levels compared to other treatments when the substitution rate was ≤30%, increasing soil environmental stress. It is well-established that soil MBP is biologically fixed by soil microorganisms and exhibits an extremely fast turnover rate (Peng et al., 2022). MBP serves as a supplementary energy source for plant-available P and plays a role as a “transit station” in the soil P cycle (Zhang et al., 2018). In the present study, MBP content was similar to the fully chemical P treatment when the substitution rate was 30% and could maintain a high potential for P supply, but it significantly decreased under the 100M treatment. Therefore, substituting 30%–40% of P fertilizer with manure is a green and sustainable management strategy that balances crop production and soil health. Previous studies have suggested that partial organic fertilizer treatments tend to result in higher available P levels, which differs from the results of this study. The monitoring of soil runoff indicated that soil available P loss under chemical fertilizer treatment was much higher than organic fertilizer treatment, and soil P loss and degree of P saturation (DPS) decreased significantly with 30% manure substitution, reducing the risk of P loss (Li et al., 2021a; Jin et al., 2023). However, this study was conducted in a closed environment with minimal P loss. In a long-term field study, soil available P under 100% manure treatment was much lower than under chemical fertilizer treatment, resulting in lower yields (Lu et al., 2021), consistent with the results of this study.
Soil P fractionation provides insights into the soil P supply capacity to crops. More active P components are preferable for direct crop uptake and utilization. The present study found that the percentage of soil labile P was higher when the manure substitution rate was less than 40% compared to the chemical fertilizer treatment. In other words, manure substitution of less than 40% ensures that nutrient requirements for crops are met in a timely manner. Moderately labile P gradually decreased with increasing substitution rate, while sparingly labile P and non-labile P exhibited the opposite trend, which matched soil Olsen-P. Additionally, the transformation of soil P pools is regulated by soil environmental factors, including soil pH and SOM. The correlation heatmap reveals that soil environmental factors are significantly correlated with various P components. Soil P effectiveness is an important factor in crop yield response to P fertilization and is often used as a guide for agricultural production (Buczko et al., 2018; Meyer et al., 2020; Ibrahim et al., 2022). Many studies have shown that P fertilizers with organic materials increase crop productivity by enhancing plant-available P (Carneiro et al., 2021; Jamal et al., 2023). In this study, excessively high manure application rates led to a significant reduction in soil P effectiveness, which was the primary reason for the reduced growth of pepper.
4.4 Efficient application of manure based on P management
At present, there is a considerable amount of organic resources in livestock development, but the recovery rate is less than 50% (Liu, 2018; Liu et al., 2023). Additionally, P reserves in China are depleting (Liu et al., 2020b). Indeed, returning organic resources to farmland is an effective solution to address these challenges and achieve a balance between crop yield and environmental sustainability in agriculture. Partial substitution strategies for manure based on P can maximize crop biological potential (root traits, AM colonization, etc.), enhance soil fertility, promote soil P turnover, and reduce the risk of nutrient surpluses. Overall, for vegetable crops with underdeveloped root systems, especially pepper, regulating the manure substitution rate at 30%–40% offers the most advantages. While 100% manure models improve the soil environment, they may lead to reduced crop productivity. This is also influenced by the duration of organic fertilizer management practices. Current evidence has demonstrated that under a regime of sustained manure substitution, the discontinuation of fertilization does not precipitate a decline in soil available P levels, which remain comparatively high for at least two years, or possibly even longer (De Figueiredo et al., 2020). In other words, under the extended organic management, it may be necessary to further reduce the optimal rate of manure substitution, and to implement controls on the total P application in order to mitigate environmental risks.
5 Conclusion
The manure substitution rate is a determining factor in crop productivity and soil P turnover under equal P input conditions. Crops can enhance root trait development, increase biomass and P uptake, and maintain moderate soil available P and MBP levels with manure substitution of 30%–40% for chemical P. Both excessively high (≥50% manure P) and low (≤20% manure P) manure substitution rates can reduce pepper productivity. Additionally, the analysis of soil P sequential fractionation showed that when the substitution rate was below 40%, the percentage of labile P in the soil remained stable at 14.1%–15.1%. These levels were found to be comparable to those observed in the complete chemical fertilizer treatment. Therefore, substituting 30%–40% of chemical P with manure ensures crop production and soil health and has practical significance for guiding environmentally friendly vegetable production, especially for crops like pepper. The efficient transformation of P in organic fertilizers can also be influenced by factors such as the source of organic fertilizer (ruminant and monogastric animals), composting processes, and microbial action. Therefore, further research is still needed in the future on organic fertilizer application methods based on different organic fertilizer sources and environmental conditions to attain green agricultural development.
Data availability statement
The original contributions presented in the study are included in the article/Supplementary Material. Further inquiries can be directed to the corresponding author.
Author contributions
KS: Conceptualization, Data curation, Formal analysis, Investigation, Visualization, Writing – original draft, Writing – review & editing. YC: Investigation, Writing – review & editing. LS: Investigation, Writing – review & editing. BW: Investigation, Writing – review & editing. YW: Writing – review & editing. SL: Investigation, Writing – review & editing. CZ: Investigation, Writing – review & editing. YW: Conceptualization, Writing – review & editing. WZ: Conceptualization, Formal analysis, Funding acquisition, Writing – review & editing.
Funding
The author(s) declare that financial support was received for the research, authorship, and/or publication of this article. This work was supported by the National Key Research and Development Program of China (2023YFE0105000), Fundamental Research Funds for the Central Universities (SWU119033 and SWU120071), the Deutsche Forschungsgemeinschaft (DFG)-328017493/GRK 2366 (Sino-German IRTG AMAIZE-P), Chongqing Municipal Training Program of Innovation and Entrepreneurship for Undergraduates (S202310635116).
Conflict of interest
The authors declare that the research was conducted in the absence of any commercial or financial relationships that could be construed as a potential conflict of interest.
Publisher’s note
All claims expressed in this article are solely those of the authors and do not necessarily represent those of their affiliated organizations, or those of the publisher, the editors and the reviewers. Any product that may be evaluated in this article, or claim that may be made by its manufacturer, is not guaranteed or endorsed by the publisher.
Supplementary material
The Supplementary Material for this article can be found online at: https://www.frontiersin.org/articles/10.3389/fpls.2024.1356861/full#supplementary-material
References
Amadou, I., Houben, D., Faucon, M. P. (2021). Unravelling the role of rhizosphere microbiome and root traits in organic phosphorus mobilization for sustainable phosphorus fertilization. A Rev. Agronomy-Basel 11, 2267. doi: 10.3390/agronomy11112267
Andriamananjara, A., Rakotoson, T., Razafimbelo, T., Rabeharisoa, L., Razafimanantsoa, M. P., Masse, D. (2019). Farmyard manure improves phosphorus use efficiency in weathered P deficient soil. Nutr. Cycl. Agroecosys. 115, 407–425. doi: 10.1007/s10705-019-10022-3
Azeem, M., Riaz, A., Chaudhary, A. N., Hayat, R., Hussain, Q., Tahir, M. I., et al. (2015). Microbial phytase activity and their role in organic P mineralization. Arch. Agron. Soil Sci. 61, 751–766. doi: 10.1080/03650340.2014.963796
Baenas, N., Belovic, M., Ilic, N., Moreno, D. A., Garcia-Viguera, C. (2019). Industrial use of pepper (Capsicum annum L.) derived products: Technological benefits and biological advantages. Food Chem. 274, 872–885. doi: 10.1016/j.foodchem.2018.09.047
Bao, S. D. (2000). Soil and Agriculture Chemistry Analysis, 3rd Edn (Beijing: China Agriculture Press).
Bindraban, P. S., Dimkpa, C. O., Pandey, R. (2020). Exploring phosphorus fertilizers and fertilization strategies for improved human and environmental health. Biol. Fert. Soils 56, 299–317. doi: 10.1007/s00374-019-01430-2
Brookes, P. C., Powlson, D. S., Jenkinson, D. S. (1982). Measurement of microbial biomass phosphorus in soil. Soil Biol. Biochem. 14, 319–329. doi: 10.1016/0038-0717(82)90001-3
Buczko, U., van Laak, M., Eichler-Lobermann, B., Gans, W., Merbach, I., Panten, K., et al. (2018). Re-evaluation of the yield response to phosphorus fertilization based on meta-analyses of long-term field experiments. Ambio 47, 50–61. doi: 10.1007/s13280-017-0971-1
Carneiro, J. S. D., Ribeiro, I. C. A., Nardis, B. O., Barbosa, C. F., Lustosa, J. F., Melo, L. C. A. (2021). Long-term effect of biochar-based fertilizers application in tropical soil: Agronomic efficiency and phosphorus availability. Sci. Total Environ. 760. doi: 10.1016/j.scitotenv.2020.143955
Chen, S., Jiang, J. Y., Wei, L. L., Lei, J. L., Fenton, O., Daly, K., et al. (2021). Partial substitution of chemical fertilizers with manure alters soil phosphorus fractions and optimizes vegetable production in alkaline soil. Arch. Agron. Soil Sci 69, 583–598. doi: 10.1080/03650340.2021.2018575
De Figueiredo, C. C., Pinheiro, T. D., De Oliveira, L. E. Z., De Araujo, A. S., Coser, T. R., Paz-Ferreiro, J. (2020). Direct and residual effect of biochar derived from biosolids on soil phosphorus pools: A four-year field assessment. Sci. Total Environ. 739. doi: 10.1016/j.scitotenv.2020.140013
Fei, C., Zhang, S. R., Li, J. F., Zhang, L., Liang, B., Li, J. L., et al. (2022). Substituting manure with rice husk to improve phosphorus adsorption and immobilization in high phosphorus accumulated greenhouse vegetable soil. J. Soil Sci. Plant Nutt. 22, 1665–1675. doi: 10.1007/s42729-022-00761-8
Food and Agriculture Organization of the United Nations. (2021). Available at: http://www.fao.org/faostat/en/. [Accessed July 20, 2023].
Fornara, D. A., Flynn, D., Caruso, T. (2020). Improving phosphorus sustainability in intensively managed grasslands: The potential role of arbuscular mycorrhizal fungi. Sci. Total Environ. 706. doi: 10.1016/j.scitotenv.2019.135744
Giovannetti, M., Mosse, B. (1980). An evaluation of techniques for measuring vesicular arbuscular mycorrhizal infection in roots. New Phytol. 84, 489–500. doi: 10.1111/j.1469-8137.1980.tb04556.x
Gong, H. Q., Xiang, Y., Wu, J. C., Nkebiwe, P. M., Feng, G., Jiao, X. Q., et al. (2022). Using knowledge-based management for sustainable phosphorus use in China. Sci. Total Environ. 814. doi: 10.1016/j.scitotenv.2021.152739
Hedley, M. J., Stewart, J. W. B., Chauhan, B. S. (1982). Changes in inorganic and organic soil-phosphorus fractions induced by cultivation practices and by laboratory incubations. Soil Sci. Soc Am. J. 46, 970–976. doi: 10.2136/sssaj1982.03615995004600050017x
Hernandez, A. Y. M., Neto, F. D., Antunes, J. E. L., Bonifácio, A., De Freitas, A. D. S., Araújo, F. F., et al. (2023). Co-inoculation of Beijerinckia indica subsp. indica and Cunninghamella elegans spp. enriches cattle manure and promotes the growth of pepper (Capsicum baccatum). Int. J. Recycling Org. 12, 457–466. doi: 10.30486/ijrowa.2023.1957320.1455
Hua, K. K., Zhu, B. (2020). Phosphorus loss through surface runoff and leaching in response to the long-term application of different organic amendments on sloping croplands. J. Soil. Sediment. 20, 3459–3471. doi: 10.1007/s11368-020-02675-3
Ibrahim, M., Iqbal, M., Tang, Y. T., Khan, S., Guan, D. X., Li, G. (2022). Phosphorus mobilization in plant-soil environments and inspired strategies for managing phosphorus: A review. Agronomy-Basel 12. doi: 10.3390/agronomy12102539
Jamal, A., Saeed, M. F., Mihoub, A., Hopkins, B. G., Ahmad, I., Naeem, A. (2023). Integrated use of phosphorus fertilizer and farmyard manure improves wheat productivity by improving soil quality and P availability in calcareous soil under subhumid conditions. Front. Plant Sci. 14. doi: 10.3389/fpls.2023.1034421
Jiang, S. T., An, X. R., Shao, Y. D., Kang, Y. L., Chen, T. S., Mei, X. L., et al. (2021). Responses of arbuscular mycorrhizal fungi occurrence to organic fertilizer: A meta-analysis of field studies. Plant Soil 469, 89–105. doi: 10.1007/s11104-021-05153-y
Jin, J. W., Fang, Y. Y., He, S., Liu, Y., Liu, C. L., Li, F. Y., et al. (2023). Improved phosphorus availability and reduced degree of phosphorus saturation by biochar-blended organic fertilizer addition to agricultural field soils. Chemosphere 317. doi: 10.1016/j.chemosphere.2023.137809
Krämer, S., Green, D. M. (2000). Acid and alkaline phosphatase dynamics and their relationship to soil microclimate in a semiarid woodland. Soil Biol. Biochem. 32, 179–188. doi: 10.1016/S0038-0717(99)00140-6
Li, X., Dong, C. X., Liu, Y. R., Liu, Y. X., Shen, Q. R., Xu, Y. C. (2012). Interactive effects from combining inorganic and organic fertilisers on phosphorus availability. Soil Res. 50, 607–615. doi: 10.1071/SR12232
Li, F. Y., Jin, Y. B., He, S., Jin, J. W., Wang, Z. W., Khan, S., et al. (2021a). Use of polyacrylamide modified biochar coupled with organic and chemical fertilizers for reducing phosphorus loss under different cropping systems. Agric. Ecosyst. Environ. 310. doi: 10.1016/j.agee.2021.107306
Li, H. G., Liu, J., Li, G. H., Shen, J. B., Bergstrom, L., Zhang, F. S. (2015). Past, present, and future use of phosphorus in Chinese agriculture and its influence on phosphorus losses. Ambio 44, S274–S285. doi: 10.1007/s13280-015-0633-0
Li, X. X., Zeng, R. S., Liao, H. (2016). Improving crop nutrient efficiency through root architecture modifications. J. Integr. Plant Biol. 58, 193–202. doi: 10.1111/jipb.12434
Li, Y. J., Zheng, Q., Yang, R., Zhuang, S., Lin, W., Li, Y. Z. (2021b). Evaluating microbial role in reducing N2O emission by dual isotopocule mapping following substitution of inorganic fertilizer for organic fertilizer. J. Clean. Prod. 326. doi: 10.1016/j.jclepro.2021.129442
Liu, X. Y. (2018). Study on nutrients balance and requirement in agricultural production in China. [dissertation]. [China]: Chin. Acad. Agric. Sci.
Liu, D. (2021). Root developmental responses to phosphorus nutrition. J. Integr. Plant Biol. 63, 1065–1090. doi: 10.1111/jipb.13090
Liu, Z. G., Gao, K., Shan, S., Gu, R. C., Wang, Z. K., Craft, E. J., et al. (2017). Comparative analysis of root traits and the associated QTLs for maize seedlings grown in paper roll, hydroponics and vermiculite culture system. Front. Plant Sci. 8. doi: 10.3389/fpls.2017.00436
Liu, J., Han, C. Q., Zhao, Y. H., Yang, J. J., Cade-Menun, B. J., Hu, Y. F., et al. (2020a). The chemical nature of soil phosphorus in response to long-term fertilization practices: Implications for sustainable phosphorus management. J. Clean. Prod. 272. doi: 10.1016/j.jclepro.2020.123093
Liu, X. W., Yuan, Z. W., Liu, X., Zhang, Y., Hua, H., Jiang, S. Y. (2020b). Historic trends and future prospects of waste generation and recycling in China’s phosphorus cycle. Environ. Sci. Technol. 54, 5131–5139. doi: 10.1021/acs.est.9b05120
Liu, X. W., Zhang, Y., Cheng, M. J., Jiang, S. Y., Yuan, Z. W. (2023). Recycling phosphorus from waste in China: Recycling methods and their environmental and resource consequences. Resour. Conserv. Recy. 188. doi: 10.1016/j.resconrec.2022.106669
Livak, K. J., Schmittgen, T. D. (2001). Analysis of relative gene expression data using real-time quantitative PCR and the 2-ΔΔCT method. Methods 25, 402–408. doi: 10.1006/meth.2001.1262
Lu, Q. W., Bunn, R., Whitney, E., Feng, Y. Y., DeVetter, L. W., Tao, H. Y. (2023b). Arbuscular mycorrhizae influence raspberry growth and soil fertility under conventional and organic fertilization. Front. Microbiol. 14. doi: 10.3389/fmicb.2023.1083319
Lu, Y. H., Gao, Y. J., Nie, J., Liao, Y. L., Zhu, Q. D. (2021). Substituting chemical P fertilizer with organic manure: effects on double-rice yield, phosphorus use efficiency and balance in subtropical China. Sci. Rep-Uk. 11, 8629. doi: 10.1038/s41598-021-87851-2
Lu, P., Zhang, Y. M., Ji, B. J., Yan, Y., Wang, Z. P., Yang, M., et al. (2023a). PhoD harboring microbial community and alkaline phosphatase as affected by long term fertilization regimes on a calcareous soil. Agronomy-Basel 13, 363. doi: 10.3390/agronomy13020363
Meyer, G., Bell, M. J., Doolette, C. L., Brunetti, G., Zhang, Y. Q., Lombi, E., et al. (2020). Plant-available phosphorus in highly concentrated fertilizer bands: Effects of soil type, phosphorus form, and coapplied potassium. J. Agr. Food Chem. 68, 7571–7580. doi: 10.1021/acs.jafc.0c01287
Muller, A., SChader, C., Scialabba, N. E. H., Bruggemann, J., Isensee, A., Erb, K. H., et al. (2017). Strategies for feeding the world more sustainably with organic agriculture. Nat. Commun. 8, 1290. doi: 10.1038/s41467-017-01410-w
Niu, X. S., Ju, X. T. (2017). Organic fertilizer resources and utilization in China. J. Plant Nutr. Fertilizers 23, 1462–1479.
Nobile, C. M., Bravin, M. N., Becquer, T., Paillat, J. M. (2020). Phosphorus sorption and availability in an andosol after a decade of organic or mineral fertilizer applications: Importance of pH and organic carbon modifications in soil as compared to phosphorus accumulation. Chemosphere 239. doi: 10.1016/j.chemosphere.2019.124709
Otinga, A. N., Pypers, P., Okalebo, J. R., Njoroge, R., Emong’Ole, M., Six, L., et al. (2013). Partial substitution of phosphorus fertiliser by farmyard manure and its localised application increases agronomic efficiency and profitability of maize production. Field Crop Res. 140, 32–43. doi: 10.1016/j.fcr.2012.10.003
Peng, Y., Duan, Y. S., Huo, W. G., Xu, M. G., Yang, X. Y., Wang, X. H., et al. (2021). Soil microbial biomass phosphorus can serve as an index to reflect soil phosphorus fertility (Apr, 10.1007/s00374-021-01559-z 2021). Biol. Fert. Soils 57, 657–669. doi: 10.1007/s00374-021-01559-z
Peng, Y., Duan, Y. S., Huo, W. G., Zhang, Z. J., Huang, D., Xu, M. G., et al. (2022). C:P stoichiometric imbalance between soil and microorganisms drives microbial phosphorus turnover in the rhizosphere. Biol. Fert. Soils. 58, 421–433. doi: 10.1007/s00374-022-01633-0
Spohn, M., Kuzyakov, Y. (2013). Distribution of microbial- and root-derived phosphatase activities in the rhizosphere depending on P availability and C allocation - Coupling soil zymography with C-14 imaging. Soil Biol. Biochem. 67, 106–113. doi: 10.1016/j.soilbio.2013.08.015
Tiessen, H., Moir, J. O. (1993). “Characterization of available P by sequential extraction,” in Soil Sampling and Methods of Analysis. Ed. Carter, M. R.. Boca Raton: Lewis Publishers, 75–86.
Wan, W. J., He, D. L., Li, X., Xing, Y. H., Liu, S., Ye, L. P., et al. (2021). Adaptation of phoD-harboring bacteria to broader environmental gradients at high elevations than at low elevations in the Shennongjia primeval forest. Geoderma 401. doi: 10.1016/j.geoderma.2021.115210
Wang, Y. L., Lambers, H. (2020). Root-released organic anions in response to low phosphorus availability: recent progress, challenges and future perspectives. Plant Soil 447, 135–156. doi: 10.1007/s11104-019-03972-8
Wang, S. B., Lv, R., Yin, X. H., Feng, P. Y., Hu, K. L. (2023a). Effects of the ratio of substituting mineral fertilizers with manure nitrogen on soil properties and vegetable yields in China: A meta-analysis. Plants-Basel 12, 964. doi: 10.3390/plants12040964
Wang, R., Shi, W. M., Li, Y. L. (2019). Phosphorus supply and management in vegetable production systems in China. Front. Agric. Sci. Eng. 6, 348–356. doi: 10.15302/J-FASE-2019277
Wang, R., Shi, W. M., Li, Y. L. (2022). Link between aeration in the rhizosphere and P-acquisition strategies: constructing efficient vegetable root morphology. Front. Env. Sci-Switz. 10. doi: 10.3389/fenvs.2022.906893
Wang, Y., Zhang, W., Muller, T., Lakshmanan, P., Liu, Y., Liang, T., et al. (2023b). Soil phosphorus availability and fractionation in response to different phosphorus sources in alkaline and acid soils: a short-term incubation study. Sci. Rep. 13, 5677. doi: 10.1038/s41598-023-31908-x
Xiang, Y. Z., Li, Y., Luo, X. Q., Liu, Y., Yue, X. J., Yao, B., et al. (2022). Manure properties, soil conditions and managerial factors regulate greenhouse vegetable yield with organic fertilizer application across China. Front. Plant Sci. 13. doi: 10.3389/fpls.2022.1009631
Yi, X. Y., Yu, L. R., Chang, S., Yin, C. B., Wang, H., Zhang, Z. F. (2021). The effects of China’s Organic-Substitute-Chemical-Fertilizer (OSCF) policy on greenhouse vegetable farmers. J. Clean. Prod. 297. doi: 10.1016/j.jclepro.2021.126677
Zhang, L., Ding, X. D., Peng, Y., George, T. S., Feng, G. (2018). Closing the loop on phosphorus loss from intensive agricultural soil: A microbial immobilization solution? Front. Microbiol. 9. doi: 10.3389/fmicb.2018.00104
Zhang, Y. J., Gao, W., Luan, H. A., Tang, J. W., Li, R. N., Li, M. Y., et al. (2021c). Long-term organic substitution management affects soil phosphorus speciation and reduces leaching in greenhouse vegetable production. J. Clean. Prod. 327. doi: 10.1016/j.jclepro.2021.129464
Zhang, Y. J., Gao, W., Luan, H. A., Tang, J. W., Li, R. N., Li, M. Y., et al. (2022). Effects of a decade of organic fertilizer substitution on vegetable yield and soil phosphorus pools, phosphatase activities, and the microbial community in a greenhouse vegetable production system. J. Integr. Agr. 21, 2119–2133. doi: 10.1016/S2095-3119(21)63715-2
Zhang, Y. C., Li, R. N., Wang, L. Y., Zhai, C. X., Chen, L. L., Wu, X. P., et al. (2010). Threshold of soil Olsen-P in greenhouses for tomatoes and cucumbers. Commun. Soil Sci. Plan. 41, 2383–2402. doi: 10.1080/00103624.2010.511371
Zhang, S. Y., Luo, P. Y., Yang, J. F., Irfan, M., Dai, J., An, N., et al. (2021a). Responses of arbuscular mycorrhizal fungi diversity and community to 41-year rotation fertilization in brown soil region of northeast China. Front. Microbiol. 12. doi: 10.3389/fmicb.2021.742651
Zhang, X. Q., Xu, Y. J., Cao, C. F., Chen, H. (2023b). Long-term located fertilization causes the differences in root traits, rhizosphere soil biological characteristics and crop yield. Arch. Agron. Soil Sci. 69, 151–167. doi: 10.1080/03650340.2021.1964018
Zhang, Y. P., Yan, J., Rong, X. M., Han, Y. L., Yang, Z. Y., Hou, K., et al. (2021d). Responses of maize yield, nitrogen and phosphorus runoff losses and soil properties to biochar and organic fertilizer application in a light-loamy fluvo-aquic soil. Agr. Ecosyst. Environ. 314. doi: 10.1016/j.agee.2021.107433
Zhang, W., Zhang, Y. W., An, Y. L., Chen, X. P. (2021b). Phosphorus fractionation related to environmental risks resulting from intensive vegetable cropping and fertilization in a subtropical region. Environ. pollut. 269. doi: 10.1016/j.envpol.2020.116098
Keywords: manure substitution, phosphorus, root traits, phosphorus fraction, pepper
Citation: Sun K, Cui Y, Sun L, Wei B, Wang Y, Li S, Zhou C, Wang Y and Zhang W (2024) Optimizing the manure substitution rate based on phosphorus fertilizer to enhance soil phosphorus turnover and root uptake in pepper (Capsicum). Front. Plant Sci. 15:1356861. doi: 10.3389/fpls.2024.1356861
Received: 16 December 2023; Accepted: 19 February 2024;
Published: 05 March 2024.
Edited by:
M. J. I. Shohag, University of Florida, United StatesReviewed by:
Meng Xu, Chinese Academy of Agricultural Sciences, ChinaKamel Mohamed Eltohamy, Zhejiang University, China
Umit Baris Kutman, Gebze Technical University, Türkiye
Copyright © 2024 Sun, Cui, Sun, Wei, Wang, Li, Zhou, Wang and Zhang. This is an open-access article distributed under the terms of the Creative Commons Attribution License (CC BY). The use, distribution or reproduction in other forums is permitted, provided the original author(s) and the copyright owner(s) are credited and that the original publication in this journal is cited, in accordance with accepted academic practice. No use, distribution or reproduction is permitted which does not comply with these terms.
*Correspondence: Yixia Wang, wyx0524@swu.edu.cn; Wei Zhang, zw0730@swu.edu.cn