- 1CAS Key Laboratory of Tropical Forest Ecology, Xishuangbanna Tropical Botanical Garden, Chinese Academy of Sciences, Mengla, China
- 2National Forest Ecosystem Research Station at Xishuangbanna, Xishuangbanna Tropical Botanical Garden, Chinese Academy of Sciences, Mengla, China
- 3College of Life Sciences, University of Chinese Academy of Sciences, Beijing, China
- 4Yunnan Provincial Forest Ecosystem Research Station at Xishuangbanna, Xishuangbanna Tropical Botanical Garden, Chinese Academy of Sciences, Mengla, China
- 5Party School of the Xishuangbanna Dai Nationality Autonomous Prefecture Committee of Communist Party of China, Yunnan Xishuangbanna Dai Nationality Autonomous Prefecture Committee and State Government, Jinghong, China
Introduction: Clonal fragmentation helps to assess clonal plants' growth resilience to human and environmental disturbance. Although clonal integration in epiphytes in tropical rubber plantations is important to understand their role in enhancing biodiversity and ecosystem services, research on this subject is limited. These plantations are typically monospecific economic forests that face increased anthropogenic disturbances.
Methods: In this study, we selected the clonal fern Pyrrosia nuda to study its survival status, biomass, maximum quantum yield of photosystem II (Fv/Fm), and frond length in response to the level of clonal fragmentation in a tropical rubber plantation.
Results and discussion: The results showed that (1) clonal fragmentation significantly negatively affected the survival rate, biomass, and frond length of clonal plants, but with minimal effects on Fv/Fm at different growth stages; (2) the performance of a ramet (e.g., biomass or frond length) increased with ramet developmental ages and decreased with the number of ramets in a clonal fragment. The age-dependent impacts of clonal fragmentation provide insights into the biodiversity conservation of epiphytes and forest management in man-made plantations. Therefore, to better conserve the biodiversity in tropical forests, especially in environment-friendly rubber plantations, there is a need to reduce anthropogenic disturbances and alleviate the level of fragmentation.
1 Introduction
Land-use change by human activities has brought extensive deforestation and alterations in various land types worldwide. This has not only severely damaged the species composition and biodiversity of tropical rainforests but also led to drastic changes in temperature and precipitation patterns in the tropics (Choat et al., 2012; Polson et al., 2016). These natural and anthropogenic disturbances have impacted the growth, flowering, and reproduction of plants (Willmer, 2012; Zambrano and Salguero-Gomez, 2014; Ruiz et al., 2018). The impacts of disturbances have directly or indirectly limited the availability of biotic resources crucial for human sustenance. To meet human resource demands, tropical rainforests are often exploited for agricultural, livestock, and forestry activities such as timber production. Unfortunately, such activities have undermined the structural and functional sustainability of tropical forests, resulting in insufficient ecological services to meet human needs. This exacerbates deforestation in biodiversity hotspots, destroying habitats and disrupting ecosystem equilibrium, and ultimately leading to the degradation and fragmentation of tropical forests (Jha, 2006; Morton et al., 2013). As a paramount type of monoculture artificial forest arising from land-use changes in tropical forests and as a strategic resource for many tropical nations, rubber plantation possesses indispensable economic and social value. However, because the environmental heterogeneity and microhabitat diversity in rubber plantations are lower compared to tropical rainforests, which makes epiphytes in forest canopies in these artificial plantations are more fragile. They even face the risk of species extinction (Baumbach et al., 2021) due to anthropogenic disturbances and fragmentation (Laurance et al., 2000; Malta et al., 2003; Barlow et al., 2007).
As a crucial refuge for endangered species in the tropics, forest canopies provide heterogeneous and diverse microhabitats for various plants and animals, playing a key role in maintaining flora and fauna diversity, community assembly, and ecosystem stability in tropical forests (Gargallo-Garriga et al., 2021; Roa-Fuentes et al., 2022). Nevertheless, extreme weather and human activities may exert influence on the structure and function (e.g., breaking branches, leaves and treetops, etc.) of the forest canopy, which subsequently reduces individual survival, the spread of species, and biotic interactions of host trees and epiphytes (Barlow et al., 2007; Zellweger et al., 2020). Epiphytes, growing in the tropical canopy for more light and fewer competitors, are essential components of tropical forest flora, contributing to species richness, and structural functionality (Kreft et al., 2004; Ellis, 2012). As epiphytes rely on the supportive structures of their hosts, the habitat fragmentation of tropical forests and clonal fragmentation of clonal epiphytes as a result of natural and anthropogenic disturbances pose direct and indirect threats to the performance and microhabitats of epiphytes. Fortunately, most nonvascular and vascular epiphytes can adapt to canopies with stressful resources and an unstable microenvironment through clonal reproduction (Lu et al., 2016, Lu et al., 2020).
Although the severe impacts of various disturbances on biodiversity and functionality can be mitigated by forest canopies and clonal reproduction, they could still negatively affect the productivity and lifespan of sensitive epiphytes, leading to cascading effects (Foster, 2001; Nadkarni and Solano, 2002; De Frenne et al., 2019). Though one of the most natures of clonal plants is physiological integration, which means sharing resources and information within interconnected fragments of a clone (Suzuki and Stuefer, 1999; Brezina et al., 2006). However, in previous studies, the whole clonal epiphytes decreased the growth and performance, while they relied on resource sharing within a clone and interspecific facilitation within canopy communities to mitigate severe stresses and negative effects (Lu et al., 2016, Lu et al., 2020; Dai et al., 2023). In particular, the growth and performance of two clonal plants, Pyrrosia nummulariifolia and Lemmaphyllum microphyllum, have been found to decline when they rely on clonal integration to deal with the negative effects of clonal fragmentation in a natural limestone forest (Dai et al., 2023). However, our understanding of the impact of clonal fragmentation resulting from extreme weather and human activities on epiphytes in artificial forests and/or plantations remains limited.
With land use changes and human activities, large areas of tropical forests have changed to tropical artificial plantations for decades. Rubber plantations are one of the main man-made forests in the tropics, especially in southeast Asia. The area of rubber plantation is about 573,333 ha in Xishuangbanna, southwest China (Liu et al., 2023; Qi et al., 2023). To improve the quality and efficiency of rubber plantations, researchers have focused on how to structure an environment friendly rubber plantation with higher biodiversity and interspecific facilitation to balance economic efficiency and ecological efficiency. In fact, epiphytes in artificial plantations play an important role in biodiversity maintenance in the tropics (Zheng et al., 2015; Bohnert et al., 2016). However, in rubber plantations, in addition to the disturbance and damage of clonal epiphytes by extreme weather and animals, rubber tapping will cut the clonal organs of epiphytes into several parts on rubber trees, and general management may also destroy and clear the whole cluster of epiphytes. The frequent occurrences of clonal fragmentation in clonal plants can lead to the splitting of intact clonal plants into potentially clonal individuals connected by one or a few ramets (Song et al., 2013b). Different degrees and/or levels of clonal fragmentation could be a function of the survival rate and individual performance of epiphytes in artificial plantations. For understanding the ecological strategies of clonal plants and maintaining biodiversity in man-made forests, it is crucial to study how clonal epiphytes respond to fragmentation in artificial plantations with various disturbances. It also holds practical significance for managing fragmented artificial forests and mitigating habitat disruption caused by human activities (Dong et al., 2012; Wang et al., 2018).
Clonal integration plays a crucial ecological role in mitigating the effects of clonal fragmentation caused by nature and humans. To improve the health of plant systems and the conservation of canopy biodiversity, it may be pertinent to reduce the level of fragmentation and promote system connectivity (Zotz and Hietz, 2001; Grimshaw et al., 2005; Zhang et al., 2009). Numerous studies have found that native plants and invasive plants in both wetland and terrestrial habitats exhibit excellent clonal growth capabilities under clonal fragmentation with severe disturbances, allowing rapid growth and reproduction not for native species but for invasive plants (Dong et al., 2012; Li et al., 2013; Zhang et al., 2023). It means that the physiological integration of resources could be varied in distances or extents for different native and invasive species. Thus, clonal fragments with different numbers of ramets (levels of fragmentation) of various plants in varied forests (e.g., natural and artificial forests) will respond to disturbances differently. Furthermore, various plant species or plants with different developmental stages may rely on clonal integration to different degrees and in terms of resource sharing and resilience of diseases. In general, younger stage individuals depend on resource sharing much more for survival and growth than older ones, for the distinction of resource absorption capability and resilience of stresses. However, little is known about the dependence degree of physiological integration on developmental ages for clonal epiphytes in artificial plantations.
To investigate the impact of clonal fragmentation (i.e. the extents of physiological integration) and its dependence on the development ages of a clonal epiphyte in an artificial forest, this study was carried out as a field in-situ experiment of P. nuda in the rubber plantation in Xishuangbanna Tropical Botanical Garden, Chinese Academy of Sciences. This study aims to address the following research questions: 1) Does increasing levels of clonal fragmentation correspond to greater negative impacts on P. nuda? 2) What is the effect of developmental stage on the survival and performance of P. nuda? 3) Do the effects of clonal fragmentation depend on the relative age of P. nuda in rubber plantation?
2 Materials and methods
2.1 Study site
The study site is located in Xishuangbanna Tropical Botanical Garden (XTBG) (21°55′39″N, 101°15′55″E, 580 m a.s.l.) in MengLun, Xishuangbanna Prefecture, Yunnan Province, China. As a biodiverse hotspot, the topography of Xishuangbanna features mountains and-valleys, with the Hengduan Mountains running north-south, covering approximately 95% of the region’s covered by mountains and hills. This region has a typical tropical monsoon climate and is characterized by a half-year dry season (November-April) and a half-year rainy season (May-October) (Dai et al., 2023). According to the observation data from Xishuangbanna Station for Tropical Rainforest Ecosystem Studies (XSTRES), the monthly mean air temperature is approximately 22.5°C, and the mean annual precipitation is approximately 1500 mm (from 2005 to 2018), with more than 80% occurring during the rainy season. The soil in the study site is Ferralic Cambisol with a pH of around 5.0 developed from sandstone-derived alluvial deposits, which has a clayey texture with 23% coarse sand (2.0 - 0.05 mm), 30% silt (0.05 - 0.002 mm), and 47% clay (< 0.002 mm) and (Yang et al., 2020). The rubber plantation is a predominantly monoculture artificial community, and the rubber trees were cultivated at a spacing of 2.0 m × 4.5 m along the terraced slope following the clearance of primary vegetation in the late 1980s, and the most dominant epiphytic fern species is P. nuda in Xishuangbanna.
2.2 Experimental design
We selected Pyrrosia nuda, the most dominant clonal epiphytic fern, as the target species for in situ field experiments in the canopy of a rubber plantation. P. nuda has long creeping rhizomes of 1.2-2.1 mm in diameter and in cross section usually with a single, central sclerenchyma strand (State Key Laboratory of Systematic and Evolutionary Botany, 2023). Most Pyrrosia species are drought-tolerant (Wei et al., 2017; Derzhavina, 2020).
The experiment involved ramets at three developmental stages/ages (1st, 2nd, and 3rd ramet closest to the rhizome apex) with fiddlehead frond ramets, just-extended frond ramets, and mature frond ramets and included four levels of fragmentation as the treatment—single-, double-, triple- and intact-ramet treatments (see Figure 1). Based on previous experiments on clonal integration of epiphytic ferns, it is noted that water stress during the dry season may lead to the death of most single ramets; therefore, this study was focused on the influence of clonal fragmentation on the survival and performance in the rainy season with less water stress (Lu et al., 2015; Zhang et al., 2019; Lu et al., 2020; Zhang et al., 2020b; Dai et al., 2023).
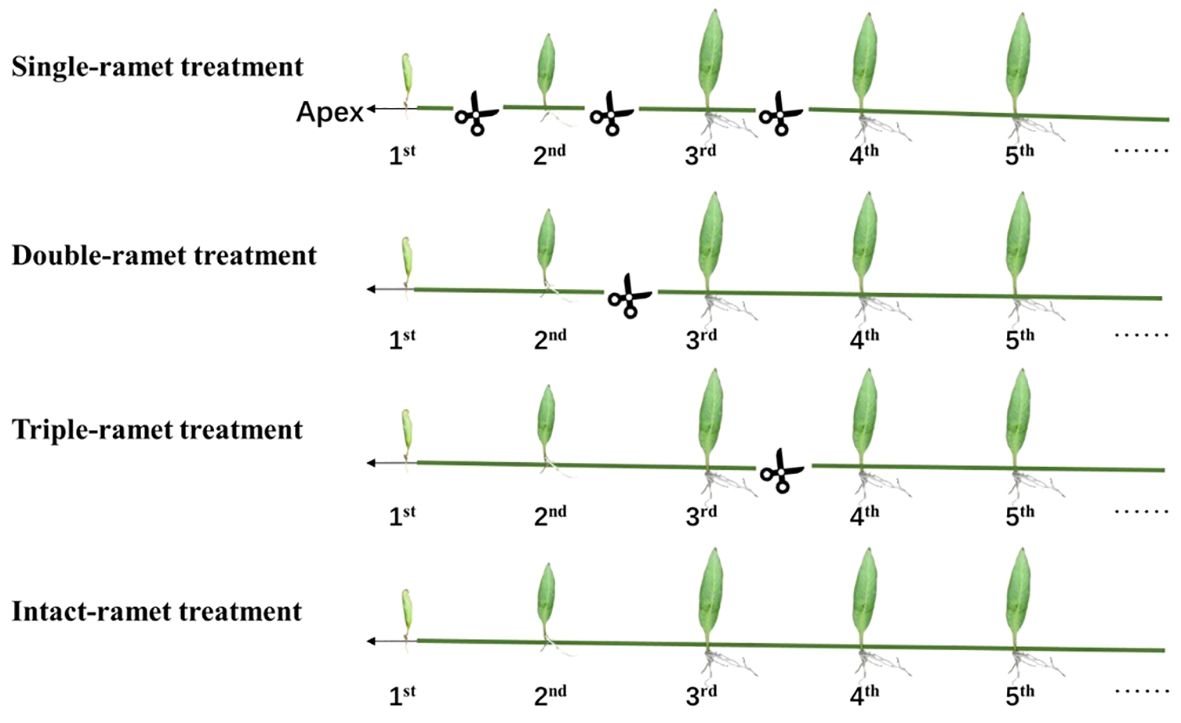
Figure 1 Experimental design. For each of the three epiphytic ferns and each of the 20 host trees, we selected four rhizomes with interconnected ramets. Within each rhizome, we identified the three adjacent ramets closest to the rhizome apex (labeled as 1st, 2nd, and 3rd). In the single-ramet treatment, we severed the rhizome midway between any two adjacent ramets among the first four ramets closest to the rhizome apex. For the double-ramet treatment, we severed the rhizome midway between the 2nd and 3rd ramet closest to the rhizome apex. In the triple-ramet treatment, the rhizome was severed midway between the 3rd and the 4th ramet closest to the rhizome apex. The intact-ramet treatment involved leaving the rhizome intact.
At the beginning of the rainy season, we selected 20 host trees with similar diameter at breast height (DBH) and tree height, which had P. nuda as the dominant epiphyte species in the rubber plantation. These rubber trees were planted at equal intervals, and the epiphytes were located in the same canopy height with consistent light conditions and humidity (Liu et al., 2018, 2020; Zeng et al., 2018; Lu et al., 2020). First, we selected a clone of interconnected ramets, then we chose four rhizomes. For each rhizome, we chose the three youngest ramets closest to the rhizome apex as our target materials (labeled as the 1st, 2nd, and 3rd ramet). We conducted in-situ experiments on epiphytic ferns on each host rubber tree at the height of 2 to 4 m on the trunk below the first branches, with 20 replications for each treatment as below. In the single-ramet treatment, we severed the rhizome midway between any two adjacent ramets of the first four ramets closest to the rhizome apex so that each of the three target ramets (1st, 2nd, and 3rd) was isolated from the rest of the clone. In the double-ramet treatment, we severed the rhizome midway between the 2nd and the 3rd ramet closest to the rhizome apex so that the first two ramets were still connected but isolated from the rest of the clone. In the triple-ramet treatment, we severed the rhizome midway between the 3rd and the 4th ramet closest to the rhizome apex so that the three target ramets were still connected but isolated from the rest of the clone. In the intact-ramet treatment, we left the rhizome intact so that the three target ramets remained connected and connected to the rest of the clone (Figure 1).
After 150 days, we assessed the survival status of each of the three target ramets (1st, 2nd, and 3rd ramet) of P. nuda, and harvested each survived ramet in situ experiment after measuring its frond length. A ramet was considered dead once all of its fronds were shed, dried, or withered. Biomass was determined after drying at 70 °CC for 48 h. Before harvesting, we assessed the maximum quantum yield of photosystem II (Fv/Fm) as a measure of photosynthetic capacity in P. nuda using a portable fluorometer (FMS-2, Hansatech, UK). The dark adaptation process involved securing healthy leaves with a Dark Leaf Clip (DLC-8) for a minimum of 20 minutes, avoiding the main veins. Subsequently, we measured the initial fluorescence (F0) by exposing the leaves to measuring light (< 0.5 μmol·m-2·s-1), followed by measuring the maximum fluorescence (Fm) using a saturating pulse (2800 μmol·m-2·s-1). This process was repeated, and the variable fluorescence (Fv) was calculated as Fv = Fm – F0. The maximum photochemical quantum yield of photosystem II (Fv/Fm) was then determined using the formula Fv/Fm = (Fm – F0)/Fm, as described in previous studies (Bolharnordenkampe et al., 1989; Heidbüchel and Hussner, 2020). After allowing fluorescence values to stabilize (approximately three to five minutes), we recorded the actual quantum yield and other fluorescence parameters. This standardized methodology ensures a precise assessment of the photosynthetic capacity in P. nuda fronds. Fv/Fm was calculated with the equation Fv/Fm = (Fm – F0)/Fm, where F0 and Fm are the minimum and maximum fluorescence yield of a dark-adapted sample after a saturation pulse (>5000 μmol photons m−2s−1 of actinic white light), respectively (Bolharnordenkampe et al., 1989; Lu et al., 2020). Fv/Fm reflects the photosynthetic performance and stress resistance of plants (Butler and Kitajima, 1975; Wei et al., 2019; Li et al., 2020), which indirectly indicates the ability of plants to absorb and the strength of plants to utilize light energy for photosynthesis. This value is positively correlated with plants’ growth and regeneration ability. Therefore, higher Fv/Fm can indirectly indicate greater survival rate, biomass, and frond length of epiphytic ferns, serving as an indicator to evaluate the growth ability and status of epiphytes (Gauslaa et al., 2001; Malta et al., 2003).
2.3 Data analysis
The data were analyzed using (generalized) linear mixed models with R version 4.2.0 (R Core Team, 2022). The “glmer” function in the “lme4” package and the “lmer” function in the “lmerTest” package were employed (Bates et al., 2015; Kuznetsova et al., 2017). Initially, the fixed factors included fragmentation level (single-, double-, triple- and intact-ramet treatments), developmental stage/age (1st, 2nd, and 3rd ramet closest to the rhizome apex), and their interactions. For individual survival data with binomial error distributions, a generalized linear mixed model was then applied (“glmer” function), with the level of clonal fragmentation and age as fixed factors, the initial individual height (leaf size) as covariate and the host tree in the sampling site as a random variable. For the biomass, Fv/Fm, and frond length of surviving ramets, a linear mixed model was used (“lmer” function), with the level of clonal fragmentation and age as fixed factors, the initial individual height (leaf size) as covariate and the host tree in the sampling site as a random variable. The data were log-transformed if needed before analysis to improve the normality of the residuals.
In the (generalized) linear mixed models, log-likelihood ratio tests were conducted to assess the significance of the fixed factors (Bedogni, 2010). During the post-hoc analyses of significant differences among treatments, a ramet was considered dead and its data was treated as missing when all of its fronds were shed, dried, or withered. In performing the log-likelihood ratio test, the null data were excluded in the analysis. These tests involve comparing a model with the term of interest to a model without the term of interest. The calculated log-likelihood ratios approximated a χ2 distribution (Platt et al., 2004). Specifically, we sequentially removed the two-way interactions, each of the two key factors and the covariate, and then compared the fit of the simplified model to the more complex model. The statistical difference in model fit indicates that the effect of the removed factor is significant.
3 Results
Compared to the intact-ramet treatment, clonal fragmentation significantly reduced the clonal ramet survival, surviving ramet biomass, Fv/Fm, and frond length of P. nuda. With the increasing levels of clonal fragmentation, the negative effects of fragmentation increased (Table 1, Figures 2–4). In P. nuda, the fragmentation led to a marked decrease in ramet biomass, with single- (0.145 ± 0.010), double- (0.167 ± 0.011), and triple-ramet (0.159 ± 0.007) treatments had lower biomass than the intact-ramet (0.224 ± 0.008). The Fv/Fm, although reduced across all fragmentation levels (single- (0.785 ± 0.012), double- (0.785 ± 0.012), triple-ramet (0.789 ± 0.005)) relative to the intact-ramet (0.811 ± 0.003), showed no difference among the three severed treatments. Notably, frond length experienced a sharp decline from the intact-ramet (145.55 ± 3.73) to single- (93.06 ± 4.41), double- (93.06 ± 4.41), and triple-ramet (101.10 ± 3.20) treatments (Figure 4).
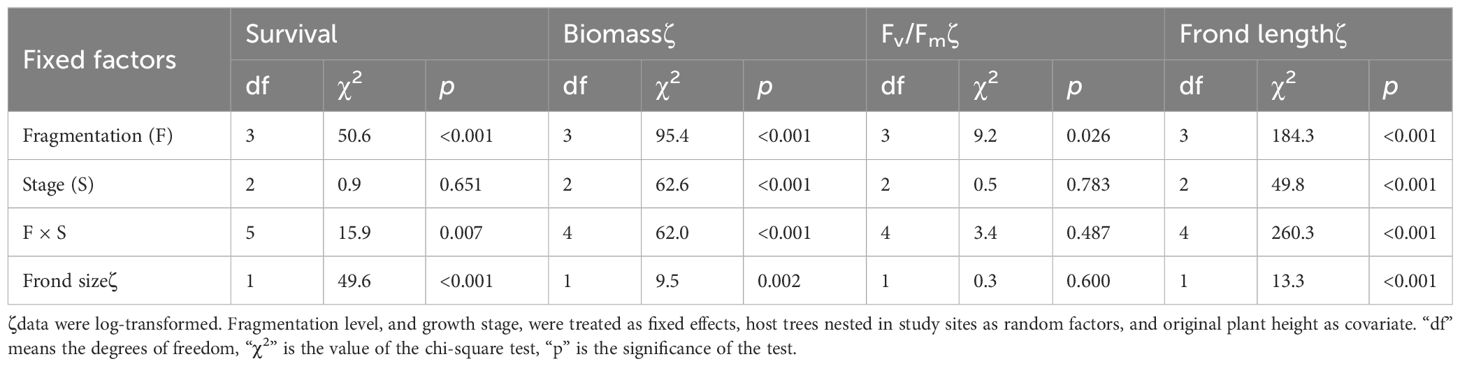
Table 1 Effects of fragmentation level (F), growth stage (S) and their interaction (F × S) on survival, growth, the maximum quantum yield of PS II (Fv/Fm), and frond length of P. nuda in (generalized) linear mixed models.
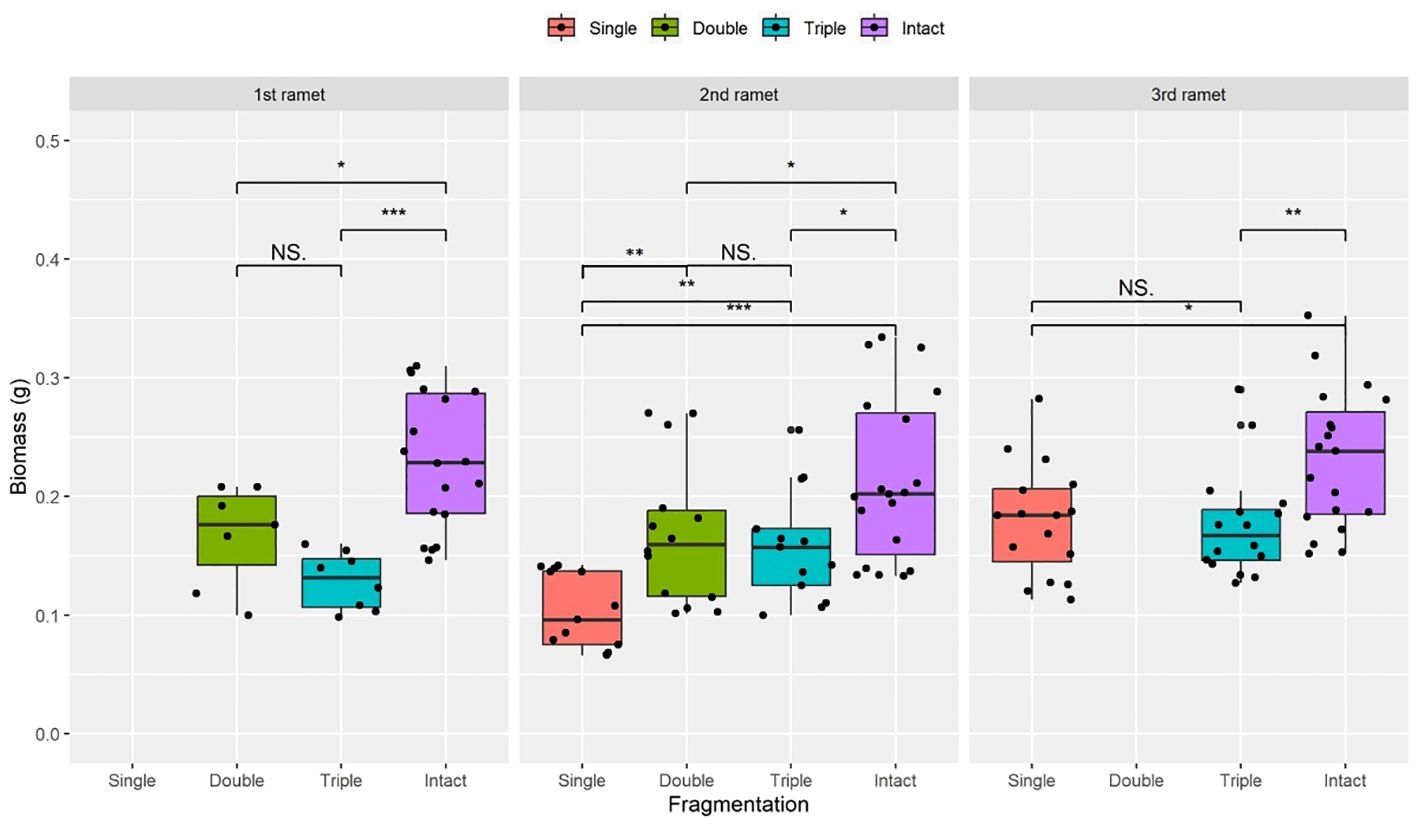
Figure 2 Biomass of surviving clonal ramet at different growth stages of each clonal fragment (single, double, triple, control/intact). Significant differences between each treatment is denoted by asterisks::*P < 0.05; **P <0.01; and ***P < 0.001; NS indicates non-significant differences between each treatment (P ≥ 0.05); Within each rhizome, the three adjacent ramets closest to the rhizome apex (labeled as 1st, 2nd, and 3rd).
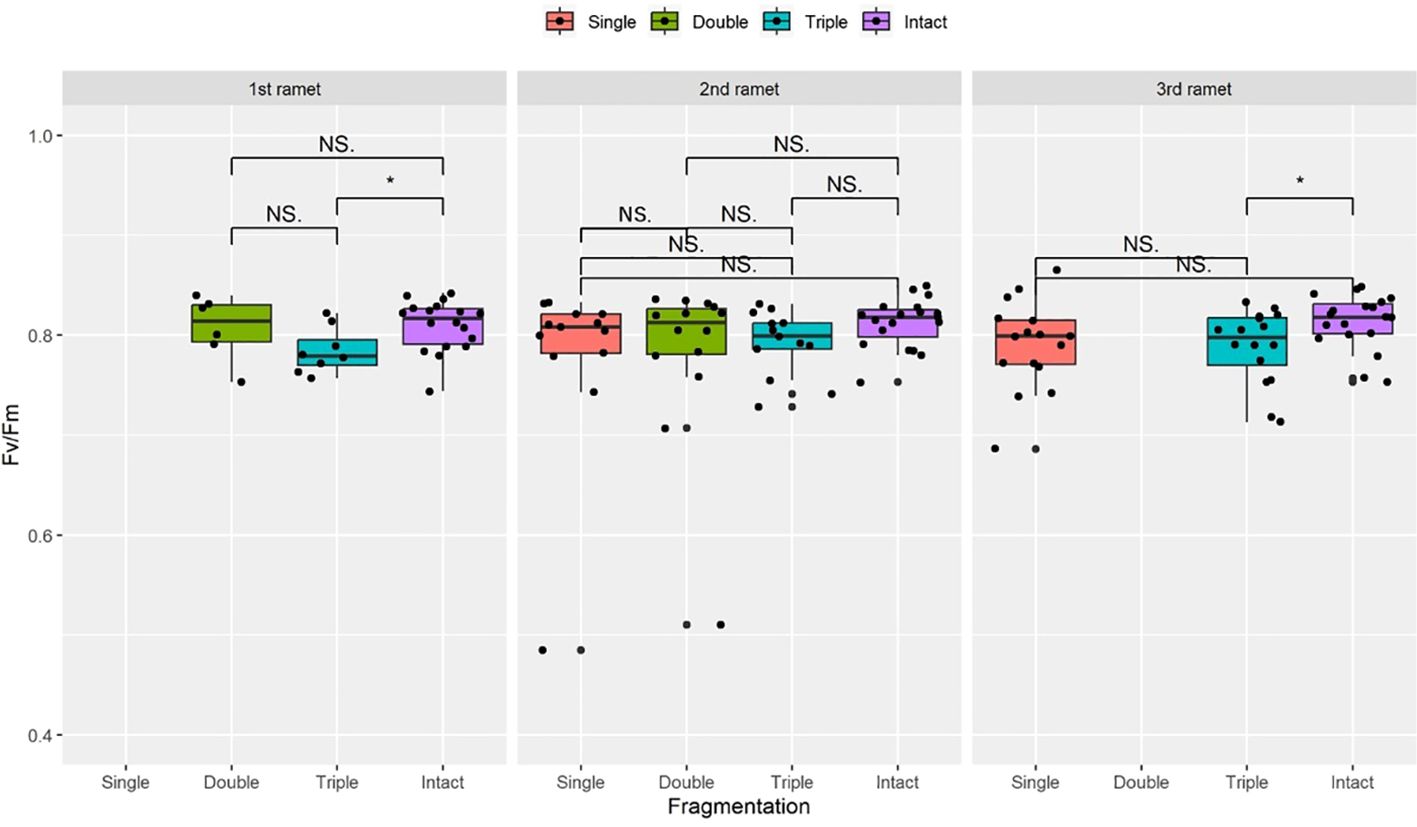
Figure 3 The maximum quantum yield of PS II (Fv/Fm) surviving clonal ramet at different growth stages of each clonal fragment (single, double, triple, control/intact). Significant differences between each treatment is denoted by asterisks:*P < 0.05; NS indicates non-significant differences between each treatment (P ≥ 0.05); Within each rhizome, the three adjacent ramets closest to the rhizome apex (labeled as 1st, 2nd, and 3rd).
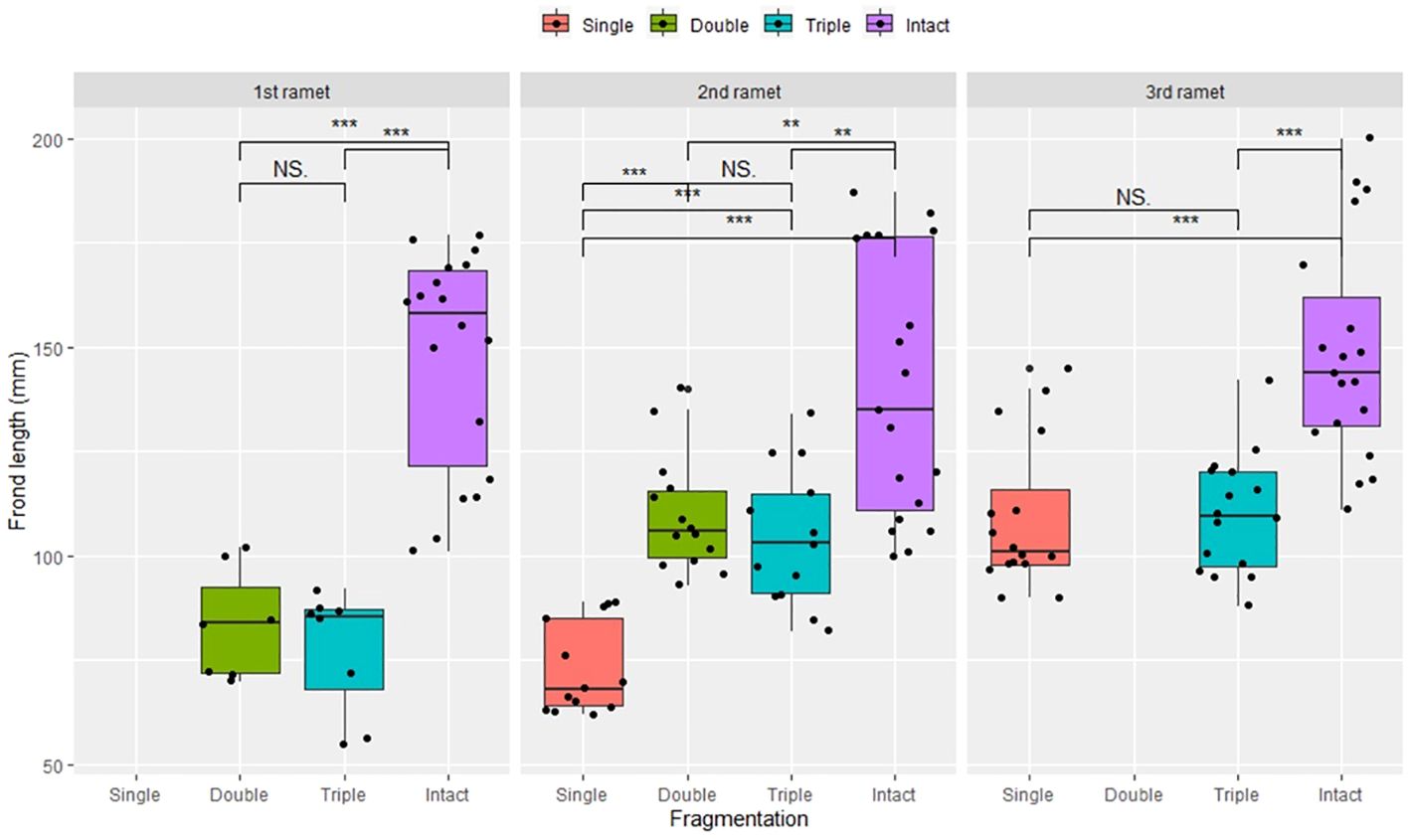
Figure 4 Frond length of surviving clonal ramet at different growth stages of each clonal fragment (single, double, triple, control/intact). Significant differences between each treatment is denoted by asterisks::*P < 0.05; NS indicates non-significant differences between each treatment (P ≥ 0.05); Within each rhizome, the three adjacent ramets closest to the rhizome apex (labeled as 1st, 2nd, and 3rd).
The growth stage significantly influenced biomass and frond length of surviving ramet. Specifically, as ramets aged, both biomass and frond length increased. However, it did not affect the clonal ramet survival and Fv/Fm of surviving ramets of P. nuda, indicating higher survival and biomass in plants at the mature leaf stage (Table 1, Figures 2, 4). The older the ramet, the better the ramet grew during clonal fragmentation (Figures 2, 4).
There were significant interaction effects of clonal fragmentation and developmental stage on the clonal ramet survival, surviving ramet biomass and frond length of P. nuda. However, no such effects were observed for Fv/Fm of surviving ramets (Table 1, Figures 2-4). It meant that the negative influences of clonal fragmentation depend on growth stages, i.e. the survival rate and growth of younger ramets in small fragments (higher fragmentation levels) was lower than that in large fragments (lower fragmentation levels) and the difference was much lower for older ramets.
4 Discussion
4.1 Response of P. nuda to clonal fragmentation
As shown in the results, clonal fragmentation had a significantly negative effect on clonal ramet survival, biomass, Fv/Fm and frond length of surviving ramets of P. nuda, especially in the fiddlehead fern stage (P <0.001, Figures 2-4). This result is consistent with previous findings. There were negative effects of clonal fragmentation on the survival rate and biomass of two clonal ferns Pyrrosia nummulariifolia and Lemmaphyllum microphyllum with different ecotypes of epiphytic and lithophytic clonal ramets (Dai et al., 2023). This confirmed our initial research hypothesis in that the higher the fragmentation level, the more severe the negative influence. This result suggests that epiphytes in both limestone forests and artificial forests would decrease the survival rate and performance by clonal fragmentation resulting from natural and anthropogenic disturbances. Similar to fern species, the negative affects of clonal fragmentation also decreased the competitive ability and the number of ramets of seed plants. As clonal fragmentation significantly inhibits the growth of S. natans, it will result in a notable reduction in its competitive fitness. This underscores the detrimental impact of clonal fragmentation on the overall competitive capacity of the species (Dong et al., 2012; Zhang et al., 2020a). Clonal fragmentation destroys the interconnection spacers and narrows the range of physiological integration, resulting in resource sharing within a limited zone. Clonal epiphytes, such as Selliguea griffithiana, rely on physiological integration for surviving and growing in both canopy and understory habitats, especially in the canopies (Lu et al., 2015), so do some invasive or wetland clonal plants (Back et al., 2013; Song et al., 2022). However, clonal fragmentation has been observed to significantly augment the growth potential of certain aquatic and invasive plants, as detailed in research by Zhang et al. (2019), and Rosef et al. (2020). These studies have collectively demonstrated the consequential enhancement of proliferation and regenerative capabilities arising from such fragmentation (to compete with local species and occupy the new habitats). Moreover, specific attention has been directed towards the advantageous effects of root fragmentation in fast-growing and ecologically adaptable species, as highlighted by Huber et al. (2014).
In this study, the epiphytic fern of P. nuda could provide more support for survival and growth from interconnected clonal ramets under natural and anthropogenic disturbance. The adverse effects on survival rate and frond length are likely due to the limited resources for growth and adaptation in the juvenile fiddlehead leaf stage of P. nuda ramets subjected to fragmentation, leading to worse self-adjustment and adaptability of clonal ramets after disturbance (Rudolphi and Gustafsson, 2011; Lu et al., 2015; Song et al., 2022; Dai et al., 2023), and such effects on biomass are likely to be the inability of ramets to share resources between them, as the reduced regeneration capacity and the increased risk of infection after ramet fragmentation can prevent the allocation and share of resources between ramets (Song et al., 2013b; Lu et al., 2020). As the level of clonal fragmentation decreases, there is a positive effect on ramet survival, biomass, and frond length, which could be because of the connection between ramets allowing epiphytes to obtain more shared resources and to better cope with stresses (Lu et al., 2015, Lu et al., 2020; Dai et al., 2023) and resulting in improved growth and reproductive regeneration capabilities. There is also a significant effect on the clonal ramet Fv/Fm, which is likely because the fragmentation process leads to water deficiency in fragmented ramets, resulting in an insufficient supply of essential raw materials for photosynthesis (Wang et al., 2020). Rubber plantations and epiphytes in the canopy are susceptible to weather and disturbances, which, together with water scarcity that reduces carbon dioxide movement into the fronds, can lead to a lack of carbon and energy demand for photochemistry and photosynthesis (Zheng et al., 2015; Wei et al., 2019; Li et al., 2020).
4.2 Response of P. nuda to different growth ages
There were significant effects of developmental age on biomass and frond growth; that is, the higher the ramet developmental age, the larger the ramet biomass and frond length. Similar findings in previous studies suggest that this might be attributed to the insufficient self-regulation capacity of epiphytes, lack of nutrient reserves and rapid growth capability during the early stages of ramet formation when just-extended frond ramets are prevented from resource sharing (Lu et al., 2015; Lin et al., 2018). For example, the rooting position significantly influenced the growth of individual ramets, with the second and third most apical ramets exhibiting optimal growth when positioned as the most apical rooted ramet. This effect was more pronounced under higher nitrogen levels (Wang et al., 2017). In addition, the rapid development of clonal ramet root systems in the extended frond and mature stages potentially broadens their ecological niche, making them less susceptible to the affects of fragmentation (Truscott et al., 2006). As plant age increases, mature ramets share and transfer nutrients to young ramets, indicating that the growth of young ramets depends more on the closely related mature maternal ramets (Alpert, 1999; de Kroon et al., 2005; Song et al., 2013a). Therefore, severing the connection between the clonal offspring and the older parents’ ramets at a younger age may result in inadequate nutrient supply, and hindered sharing of water resources and photosynthetic products, thereby reducing their survival and growth rate.
The adjustment and adaptive performance of the 1st age younger fragmented clonal ramets may diminish after disturbance, with their reproductive and resistance capabilities enhanced with growth stage and maturity (Rudolphi and Gustafsson, 2011). There is relatively little variation in the impact on Fv/Fm and ramet survival with increased ramet developmental age (Table 1, Figures 2-4), which is in contrast to previous studies which, indicated that clonal integration has varying effects on the maximum photosynthetic efficiency of ramets. Clonal integration significantly influences the photosynthetic rates of Alternanthera philoxeroides and Fragaria vesca but does not affect the photosynthetic rate of the epiphytic fern species Diplopterygium glaucoma (Luo et al., 2014; Reynolds et al., 2014). The yield effect of benefits and resource acquisition does not necessarily rely entirely on the frond photochemistry to obtain the energy required for growth. The lack of significance could be linked to the depletion of most plant resources and energy for recovering damage after fragmentation. The impact on leaf photosynthesis can translate into varying effects on ramet survival and growth. Therefore, an extended period of resource accumulation is required to enhance plant photosynthetic performance and stress resistance (Wei et al., 2019; Li et al., 2020; Lu et al., 2020). Furthermore, the presence of the forest canopy may block some light, resulting in insignificant effects on Fv/Fm at different growth stages for clonal ramets situated in the canopy, under normal circumstances, the Fv/Fm in plants typically ranges from 0.70 to 0.80 (Butler and Kitajima, 1975; Bolharnordenkampe et al., 1989). After disconnection, the Fv/Fm is significantly reduced. The changes in the Fv/Fm depend on the species and the light condition of the plants (Wei et al., 2019; Li et al., 2020). The negative impact of clonal fragmentation on epiphytes is age-dependent and influenced by clonal integration effects (Lu et al., 2016, Lu et al., 2020).
4.3 High dependence of clonal fragmentation on growth stage
The interaction between the level of clonal fragmentation and the developmental stage of the ramet showed that clonal fragmentation had entirely different effects on the survival, biomass, and frond length of P. nuda ramets at different growth ages. This is consistent with previous research which found Pyrrosia nummulariifolia and Lemmaphyllum microphyllum exhibit significant interaction effects of clonal fragmentation and ages on ramet survival and growth in natural forests (Dai et al., 2023). This appears to stem from the transient reliance of relatively early-maturing clonal ramets on clonal integration (Saine et al., 2018). Slow-developing or mature epiphytes may exhibit a higher dependence on growth stages. The impact of clonal fragmentation on clonal ramet growth and dependence on clonal integration varied with growth stages for the same species, diminishing growth in younger ramets while enhancing growth in older ramets (Stuefer et al., 2001; Dong et al., 2012; Song et al., 2013b; Luo et al., 2014; Wang et al., 2014). Additionally, this age-dependent response to clonal fragmentation might be associated with the clonal division of labor in plants during ontogenesis. Clonal plants undergo morphological or functional specialization at different growth stages, enhancing their ability to acquire heterogeneous resources, thus elevating their position and role in competitive relationships (Fan et al., 2018). Consequently, the capacity of epiphytes to acquire resources varies at different growth stages, leading to distinct affects on clonal ramet survival and growth after clonal fragmentation.
The effect of clonal fragmentation on clonal ramets depended on growth stages. Clonal fragmentation affects early juvenile fiddlehead, extended frond, and mature P. nuda ramets differently, with a significantly negative impact on survival during the early growth stage. But there is no significant effect on survival during the extended frond and mature stages. Clonal fragmentation is likely to have a negative impact on the growth of young ramets, while positively influence the growth of older ramets (Ortego et al., 2002; Dong et al., 2012; Zhang et al., 2023). Stored carbohydrates in clonal ramets might be used to prevent the risk of future ramet death (Suzuki and Stuefer, 1999), which could be a result of a ramet response to the current growth potential and a trade-off between the current growth potential and future risk regulation that could explain the observed differences. This is further supported by the enhanced resistance of mature ramets to clonal fragmentation, especially in epiphytic ferns (Wei et al., 2019; Zhang et al., 2023). This highlights the high dependence of clonal integration on growth ages, and resource sharing facilitated by clonal integration contributes significantly to the survival and performance of epiphytic ferns.
5 Conclusion
In artificial rubber plantations, clonal fragmentation exerted negative age-dependent effects on survival and performance of the epiphytic fern P. nuda, especially at the juvenile fiddlehead leaf stage. When clonal ramets are fragmented by natural or anthropogenic disturbance, the plant’s resource sharing will be limited to fragments of small numbers of interconnected ramets, leading to resource scarcity and declined performance. Such negative effects of clonal fragmentation were much more severe on juvenile individuals than aged ones, which may have resulted from the higher stress-resilience and resource-storage of adults. Based on the results of the survival, biomass, and frond length analysis of the P. nuda clonal ramets, it is imperative to minimize and avoid anthropogenic disturbance causing fragmentation in artificial forests, such as tropical rubber plantations, to mitigate its impact on plant biodiversity. This research indicates that clonal integration plays a crucial role in the growth, reproduction, and performance of clonal plants in tropical artificial forests under adverse stress conditions. It sheds light on the biodiversity conservation and maintenance during the establishment of environment-friendly rubber plantations establishment with increased human activities, land-use changes and extreme weather.
Data availability statement
The original contributions presented in the study are included in the article/supplementary material. Further inquiries can be directed to the corresponding author.
Author contributions
XY: Data curation, Visualization, Writing – original draft, Writing – review & editing. NJ: Data curation, Supervision, Writing – review & editing, Writing – original draft. RB: Data curation, Writing – review & editing, Visualization, Writing – original draft. YM: Data curation, Writing – review & editing, Visualization, Investigation. XP: Data curation, Writing – review & editing, Investigation. JL: Writing – review & editing, Visualization. H-ZL: Supervision, Writing – review & editing, Visualization, Data curation, Investigation, Writing – original draft, Conceptualization, Funding acquisition, Methodology.
Funding
The author(s) declare financial support was received for the research, authorship, and/or publication of this article. This study was supported by the Yunnan Revitalization Talent Support Program (H-ZL), the National Natural Science Foundation of China (No.31872685), the 14th Five-Year Plan of the Xishuangbanna Tropical Botanical Garden Chinese Academy of Sciences (E3ZKFF7B, E3ZKFF2B, E3ZKFF9B), the CAS-UCLA Scholarship (H-ZL), and the Yunnan Natural Science Foundation (2019FB037).
Acknowledgments
We thank Mr. Yuan-Xiang Lu and Ms. Yu-Xian Hu for their fieldwork. We also appreciate Ms. Yaqiong Cui and Ms. Yang Chen for wording revision and the center laboratory of public technology for measuring devices.
Conflict of interest
The authors declare that the research was conducted in the absence of any commercial or financial relationships that could be construed as a potential conflict of interest.
Publisher’s note
All claims expressed in this article are solely those of the authors and do not necessarily represent those of their affiliated organizations, or those of the publisher, the editors and the reviewers. Any product that may be evaluated in this article, or claim that may be made by its manufacturer, is not guaranteed or endorsed by the publisher.
References
Alpert, P. (1999). Clonal integration in Fragaria chiloensis differs between populations: ramets from grassland are selfish. Oecologia. 120, 69–76. doi: 10.1007/s004420050834
Back, C. L., Holomuzki, J. R., Klarer, D. M., Whyte, R. S. (2013). Herbiciding invasive reed: indirect effects on habitat conditions and snail-algal assemblages one year post-application. Wetl. Ecol. Manage. 21, 155–156. doi: 10.1007/s11273-013-9296-4
Barlow, J., Gardner, T. A., Araujo, I. S., Avila-Pires, T. C., Bonaldo, A. B., Costa, J. E., et al. (2007). Quantifying the biodiversity value of tropical primary, secondary, and plantation forests. Proc. Natl. Acad. Sci. U. S. A. 104, 18555–18560. doi: 10.1073/pnas.0703333104
Bates, D., Mächler, M., Bolker, B. M., Walker, S. (2015). Fitting linear mixed-effects models using lme4. J. Stat. Software 67, 1–48. doi: 10.18637/jss.v067.i01
Baumbach, L., Warren, D. L., Yousefpour, R., Hanewinkel, M. (2021). Climate change may induce connectivity loss and mountaintop extinction in Central American forests. Commun. Biol. 4, 869. doi: 10.1038/s42003-021-02359-9
Bedogni, G. (2010). A beginner’s guide to R. J. R. Stat. Soc. Ser. A-Stat. Soc 173, 697–698. doi: 10.1111/j.1467-985X.2010.00646_12.x
Bohnert, T., Wenzel, A., Altenhövel, C., Beeretz, L., Tjitrosoedirdjo, S. S., Meijide, A., et al. (2016). Effects of land-use change on vascular epiphyte diversity in Sumatra (Indonesia). Biol. Conserv. 202, 20–29. doi: 10.1016/j.biocon.2016.08.008
Bolharnordenkampe, H. R., Long, S. P., Baker, N. R., Oquist, G., Schreiber, U., Lechner, E. G. (1989). Chlorophyll fluorescence as a probe of the photosynthetic competence of leaves in the field: a review of current instrumentation. Funct. Ecol. 3, 497–514. doi: 10.2307/2389624
Brezina, S., Koubek, T., Münzbergová, Z., Herben, T. (2006). Ecological benefits of integration of Calamagrostis epigejos ramets under field conditions. Flora. 201, 461–467. doi: 10.1016/j.flora.2005.10.003
Butler, W. L., Kitajima, M. (1975). Fluorescence quenching in photosystem II of chloroplasts. Biochim. Biophys. Acta 376, 116–125. doi: 10.1016/0005-2728(75)90210-8
Choat, B., Jansen, S., Brodribb, T. J., Cochard, H., Delzon, S., Bhaskar, R., et al. (2012). Global convergence in the vulnerability of forests to drought. Nature. 491, 752–75+. doi: 10.1038/nature11688
Dai, Q., Mo, Y. X., Chen, Q., Song, L., Zhang, L. M., Dossa, G. G. O., et al. (2023). Clonal fragmentation drives performance deterioration of epiphytic and lithophytic ferns in a karst forest. Flora. 302, 152258. doi: 10.1016/j.flora.2023.152258
De Frenne, P., Zellweger, F., Rodríguez-Sánchez, F., Scheffers, B. R., Hylander, K., Luoto, M., et al. (2019). Global buffering of temperatures under forest canopies. Nat. Ecol. Evol. 3, 744–749. doi: 10.1038/s41559-019-0842-1
de Kroon, H., Huber, H., Stuefer, J. F., van Groenendael, J. M. (2005). A modular concept of phenotypic plasticity in plants. New Phytol. 166, 73–82. doi: 10.1111/j.1469-8137.2004.01310.x
Derzhavina, N. M. (2020). Ecological morphology of proto-epiphyte fern lemmaphyllum microphyllum C. Presl and its relation to the adaptogenesis. Contemp. Probl. Ecol. 13, 205–213. doi: 10.1134/S1995425520030051
Dong, B. C., Alpert, P., Guo, W., Yu, F. H. (2012). Effects of fragmentation on the survival and growth of the invasive, clonal plant Alternanthera philoxeroides. Biol. Invasions. 14, 1101–1110. doi: 10.1007/s10530-011-0141-5
Ellis, C. (2012). Lichen epiphyte diversity: A species, community and trait-based review. Perspect. Plant Ecol. Evol. Syst. 14, 131–152. doi: 10.1016/j.ppees.2011.10.001
Fan, B. L., Zhao, C. M., Zhang, X. W., Sun, K. (2018). Impacts of sand burial and wind erosion on regeneration and growth of a desert clonal shrub. Front. Plant Sci. 9. doi: 10.3389/fpls.2018.01696
Foster, P. (2001). The potential negative impacts of global climate change on tropical montane cloud forests. Earth-Sci. Rev. 55, 73–106. doi: 10.1016/S0012-8252(01)00056-3
Gargallo-Garriga, A., Sardans, J., Alrefaei, A. F., Klem, K., Fuchslueger, L., Ramírez-Rojas., I., et al. (2021). Tree species and epiphyte taxa determine the “metabolomic niche” of canopy suspended soils in a species-rich lowland tropical rainforest. Metabolites. 11, 718. doi: 10.3390/metabo11110718
Gauslaa, Y., Ohlson, M., Solhaug, K. A., Bilger, W., Nybakken, L. (2001). Aspect-dependent high-irradiance damage in two transplanted foliose forest lichens, Lobaria pulmonaria and Parmelia sulcata. Can. J. For. Res. 31, 1639–1649. doi: 10.1139/x01-098
Grimshaw, H. J., Sharfstein, B., East, T. (2005). The effects of shading on Chara zeylanica Klein ex Wild. and associated epiphytes. Archiv für Hydrobiologie. 162, 253–266. doi: 10.1127/0003-9136/2005/0162-0253
Heidbüchel, P., Hussner, A. (2020). Fluorescence quenching in photosystem II of chloroplasts. Aquat. Bot. 160, 103164. doi: 10.1016/j.aquabot.2019.103164
Huber, H., Visser, E. J. W., Clements, G., Peters, J. L. (2014). Flooding and fragment size interact to determine survival and regrowth after fragmentation in two stoloniferous Trifolium species. Aob Plants. 6, plu024. doi: 10.1093/aobpla/plu024
Jha, S., Bawa, K. S. (2006). Population growth, human development, and deforestation in biodiversity hotspots. S.Conserv. Biol. 20, 906–912. doi: 10.1111/j.1523-1739.2006.00398.x
Kreft, H., Köster, N., Küper, W., Nieder, J., Barthlott, W. (2004). Diversity and biogeography of vascular epiphytes in Western Amazonia, Yasuni, Ecuador. J. Biogeogr. 31, 1463–1476. doi: 10.1111/j.1365-2699.2004.01083.x
Kuznetsova, A., Brockhoff, P. B., Christensen, R. H. B. (2017). lmerTest package: tests in linear mixed effects models. J. Stat. Software 82, 1–26. doi: 10.18637/jss.v082.i13
Laurance, W. F., Delamônica, P., Laurance, S. G., Vasconcelos, H. L., Lovejoy, T. E. (2000). Conservation -: Rainforest fragmentation kills big trees. Nature. 404, 836–836. doi: 10.1038/35009032
Li, Q., Liu, X., Chai, Y. F., Yue, M., Quan, J. X. (2020). Physiological integration ameliorates the effects of UV-B radiation in the clonal herb Duchesnea indica. Folia Geobot. 55, 141–150. doi: 10.1007/s12224-020-09369-1
Li, X. X., Shen, Y. D., Huang, Q. Q., Fan, Z. W., Huang, D. D. (2013). Regeneration capacity of small clonal fragments of the invasive Mikania micrantha H.B.K.: effects of burial depth and stolon internode length. PloS One 8, e84657. doi: 10.1371/journal.pone.0084657
Lin, H. F., Alpert, P., Zhang, Q., Yu, F. H. (2018). Facilitation of amphibious habit by physiological integration in the clonal, perennial, climbing herb Ipomoea aquatica. Sci. Total Environ. 618, 262–268. doi: 10.1016/j.scitotenv.2017.11.025
Liu, J. Q., Liu, W. J., Li, W. X., Jiang, X. J., Wu, J. E. (2018). Effects of rainfall on the spatial distribution of the throughfall kinetic energy on a small scale in a rubber plantation. Hydrol. Sci. J. 63, 1078–1090. doi: 10.1080/02626667.2018.1473580
Liu, J. Q., Liu, W. J., Zhu, K. (2020). Fluorescence quenching in photosystem II of chloroplasts. Catena 161, 113–121. doi: 10.1016/j.catena.2017.10.014
Liu, W. G., Zhang, J. Q., Yan, Y., Beckschäfer, P., Kleinn, C., Dossa, G. G. O., et al. (2023). Encouraging the reconversion of rubber plantations by developing a combined payment system. Glob. Ecol. Conserv. 43, e02415. doi: 10.1016/j.gecco.2023.e02415
Lu, H. Z., Brooker, R., Song, L., Liu, W. Y., Sack, L., Zhang, J. L., et al. (2020). When facilitation meets clonal integration in forest canopies. New Phytol. 225, 135–142. doi: 10.1111/nph.16228
Lu, H. Z., Liu, W. Y., Yu, F. H., Song, L., Xu, X. L., Wu, C. S., et al. (2015). Higher clonal integration in the facultative epiphytic fern Selliguea griffithiana growing in the forest canopy compared with the forest understorey. Ann. Bot. 116, 113–122. doi: 10.1093/aob/mcv059
Lu, H. Z., Song, L., Liu, W. Y., Xu, X. L., Hu, Y. H., Shi, X. M., et al. (2016). Survival and growth of epiphytic ferns depend on resource sharing. Front. Plant Sci. 7. doi: 10.3389/fpls.2016.00416
Luo, F. L., Chen, Y., Huang, L., Wang, A., Zhang, M. X. (2014). Shifting effects of physiological integration on performance of a clonal plant during submergence and de-submergence. H.Ann. Bot. 113, 1265–1274. doi: 10.1093/aob/mcu057
Malta, E., Rijstenbil, J. W., Brouwer, P. E. M., Kromkamp, J. C. (2003). Vertical heterogeneity in physiological characteristics of Ulva spp. mats. Mar. Biol. 143, 1029–1038. doi: 10.1007/s00227-003-1134-4
Morton, D. C., Le, Page., Y., DeFries, R., Collatz, G. J., Hurtt, G. C. (2013). Understorey fire frequency and the fate of burned forests in southern Amazonia. Philos. Trans. R. Soc B-Biol. Sci. 368, 20120163. doi: 10.1098/rstb.2012.0163
Nadkarni, N. M., Solano, R. (2002). Potential effects of climate change on canopy communities in a tropical cloud forest: an experimental approach. Oecologia. 131, 580–586. doi: 10.1007/s00442-002-0899-3
Ortego, J., Bonal, R., Muñoz, A. (2002). Genetic consequences of habitat fragmentation in long-lived tree species: the case of the mediterranean holm oak (Quercus ilex, L.). J. Hered. 101, 717–726. doi: 10.1093/jhered/esq081
Platt, L., Hickman, M., Rhodes, T., Mikhailova, L., Karavashkin, V., Vlasov, A., et al. (2004). The prevalence of injecting drug use in a Russian city: implications for harm reduction and coverage. Addiction. 99, 1430–1438. doi: 10.1111/j.1360-0443.2004.00848.x
Polson, D., Hegerl, G. C., Solomon, S. (2016). Precipitation sensitivity to warming estimated from long island records. Environ. Res. Lett. 11, 7. doi: 10.1088/1748-9326/11/7/074024
Qi, D. L., Yang, C., Yun, T., Wu, Z. X. (2023). The main service functions and driving forces of rubber (Hevea brasiliensis) plantation ecosystem in China. J. Rubber Res. 26, 155–164. doi: 10.1007/s42464-023-00202-w
Reynolds, P. L., Richardson, J. P., Duffy, J. E. (2014). Field experimental evidence that grazers mediate transition between microalgal and seagrass dominance. Limnol. Oceanogr. 59, 1053–1064. doi: 10.4319/lo.2014.59.3.1053
Roa-Fuentes, L., Villamizar-Peña, L. A., Mantilla-Carreño, J. A., Jaramillo, M. A. (2022). Functional diversity and species diversity in flooded and unflooded tropical forests. Acta Oecol.-Int. J. Ecol. 114, 103814. doi: 10.1016/j.actao.2022.103814
Rosef, L., Ingebrigtsen, H. H., Heegaard, E. (2020). Vegetative propagation of Solidago canadensis - do fragment size and burial depth matter? Weed Res. 60, 132–141. doi: 10.1111/wre.12395
Rudolphi, J., Gustafsson, L. (2011). Forests regenerating after clear-cutting function as habitat for bryophyte and lichen species of conservation concern. PloS One 6, e18639. doi: 10.1371/journal.pone.0018639
Ruiz, J. M., Marín-Guirao, L., García-Muñoz, R., Ramos-Segura, A., Bernardeau-Esteller, J., Pérez, M., et al. (2018). Experimental evidence of warming-induced flowering in the Mediterranean seagrass. Posidonia oceanica. 134, 49–54. doi: 10.1016/j.marpolbul.2017.10.037
Saine, S., Aakala, T., Purhonen, J., Launis, A., Tuovila, H., Kosonen, T., et al. (2018). Effects of local forest continuity on the diversity of fungi on standing dead pines. For. Ecol. Manage. 409, 757–765. doi: 10.1016/j.foreco.2017.11.045
Song, Z. L., Huang, Y. Y., Liu, Q., Hu, X. K. (2022). Discovering the characteristics of community structures and functional properties of epiphytic bacteria on spartina alterniflora in the coastal salt marsh area. J. Mar. Sci. Eng. 10, 1981. doi: 10.3390/jmse10121981
Song, Y. B., Yu, F. H., Keser, L. H., Dawson, W., Fischer, M., Dong, M., et al. (2013a). United we stand, divided we fall: a meta-analysis of experiments on clonal integration and its relationship to invasiveness. Oecologia. 171, 317–327. doi: 10.1007/s00442-012-2430-9
Song, Y. B., Yu, F. H., Li, J. M., Keser, L. H., Fischer, M., Dong, M., et al. (2013b). Plant invasiveness is not linked to the capacity of regeneration from small fragments: an experimental test with 39 stoloniferous species. Biol. Invasions. 15, 1367–1376. doi: 10.1007/s10530-012-0374-y
State Key Laboratory of Systematic and Evolutionary Botany. (2023). Institute of Botany, Chinese Academy of Sciences. Flora of China. http://www.iplant.cn/foc. [Accessed October 12, 2023].
Stuefer, J. F., Erschbamer, B., Huber, H., Suzuki, J. I. (2001). The ecology and evolutionary biology of clonal plants: an introduction to the proceedings of Clone-2000. Evol. Ecol. 15, 223–230. doi: 10.1023/A:1016017822027
Suzuki, J. I., Stuefer, J. F. (1999). On the ecological and evolutionary significance of resource storage in clonal plants. Plant Species Biol. 14, 11–17. doi: 10.1046/j.1442-1984.1999.00002.x
Truscott, A. M., Soulsby, C., Palmer, S. C. F., Newell, L., Hulme, P. E. (2006). The dispersal characteristics of the invasive plant Mimulus guttatus and the ecological significance of increased occurrence of high-flow events. J. Ecol. 94, 1080–1091. doi: 10.1111/j.1365-2745.2006.01171.x
Wang, P., Alpert, P., Yu, F. H. (2017). Clonal integration affects allocation in the perennial herb Alternanthera philoxeroides in N-limited homogeneous environments. Folia Geobot. 52, 303–315. doi: 10.1007/s12224-016-9273-9
Wang, M. Z., Bu, X. Q., Li, L., Dong, B. C., Li, H. L., Yu, F. H. (2018). Constraints on the evolution of phenotypic plasticity in the clonal plant hydrocotyle vulgaris. J. Evol. Biol. 31, 1006–1017. doi: 10.1111/jeb.13281
Wang, P., Xu, Y. S., Dong, B. C., Xue, W., Yu, F. H. (2014). Effects of clonal fragmentation on intraspecific competition of a stoloniferous floating plant. Plant Biol. 16, 1121–1126. doi: 10.1111/plb.12170
Wang, J. Y., Xu, T. T., Wang, Y., Li, G. Y., Abdullah, I., Zhong, Z. W., et al. (2020). A meta-analysis of effects of physiological integration in clonal plants under homogeneous vs. heterogeneous environments. Funct. Ecol. 35, 578–589. doi: 10.1111/1365-2435.13732
Wei, Q., Li, Q., Jin, Y., Wu, S. L., Fan, L. H., Lei, N. F., et al. (2019). Transportation or sharing of stress signals among interconnected ramets improves systemic resistance of clonal networks to water stress. Funct. Plant Biol. 46, 613–623. doi: 10.1071/FP18232
Wei, X. P., Qi, Y. D., Zhang, X. C., Luo, L., Shang, H., Wei, R., et al. (2017). Phylogeny, historical biogeography and characters evolution of the drought resistant fern Pyrrosia Mirbel (Polypodiaceae) inferred from plastid and nuclear markers. Sci. Rep. 7, 12757. doi: 10.1038/s41598-017-12839-w
Willmer, P. (2012). Ecology: pollinator-plant synchrony tested by climate change. Curr. Biol. 22, R131–R132. doi: 10.1016/j.cub.2012.01.009
Yang, B., Zhang, W. J., Meng, X. J., Singh, A. K., Zakari, S., Song, L., et al. (2020). Effects of a funnel-shaped canopy on rainfall redistribution and plant water acquisition in a banana (Musa spp.) plantation. Soil Tillage Res. 203, 104686. doi: 10.1016/j.still.2020.104686
Zambrano, J., Salguero-Gomez, R. (2014). Forest fragmentation alters the population dynamics of a late-successional tropical tree. Biotropica. 46, 556–564. doi: 10.1111/btp.12144
Zellweger, F., De Frenne, P., Lenoir, J., Vangansbeke, P., Verheyen, K., Bernhardt-Römermann, M. (2020). Forest microclimate dynamics drive plant responses to warming. Science. 368, 772–77+. doi: 10.1126/science.aba6880
Zeng, H. H., Wu, J. E., Singh, A. K., Zhu, X. A., Zhang, W. J., Hahn, P., et al. (2018). Effect of intercrops complexity on water uptake patterns in rubber plantations: Evidence from stable isotopes (C-H-O) analysis. Agric. Ecosyst. Environ. 338, 108086. doi: 10.1016/j.agee.2022.108086
Zhang, L. M., Alpert, P., Si, C., Yu, F. H. (2019). Interactive effects of fragment size, nutrients, and interspecific competition on growth of the floating, clonal plant Salvinia natans. Aquat. Bot. 153, 81–87. doi: 10.1016/j.aquabot.2018.12.001
Zhang, Q., Chen, J. W., Li, B. G., Cao, K. F. (2009). The effect of drought on photosynthesis in two epiphytic and two terrestrial tropical fern species. Photosynthetica. 47, 128–132. doi: 10.1007/s11099-009-0020-9
Zhang, R., Chen, Z. H., Lyu, W. Y., Hu, Y. F., Yang, J. X., Xin, J. C., et al. (2023). Effects of fragmentation, cadmium, and nutrients on the growth of a wetland invasive plant Alternanthera philoxeroides. Plant Spec. Biol. 38, 180–191. doi: 10.1111/1442-1984.12405
Zhang, L. M., Jin, Y., Yao, S. M., Lei, N. F., Chen, J. S., Zhang, Q., et al. (2020b). Growth and morphological responses of Duckweed to clonal fragmentation, nutrient availability, and population density. Front. Plant Sci. 11. doi: 10.3389/fpls.2020.00618
Zhang, J., Li, N. N., Song, A., You, W. H., Du, D. L. (2023). Clonal integration can promote the growth and spread of Alternanthera philoxeroides in cadmium-contaminated environments. Plant Physiol. Biochem. 202, 107966. doi: 10.1016/j.plaphy.2023.107966
Zhang, L. M., Yao, S. M., Jin, Y., Yao, S. M., Lei, N. F., Chen, J. S., et al. (2020a). Effects of clonal fragmentation and nutrient availability on the competitive ability of the floating plant Salvinia natans. Folia Geobot. 55, 63–71. doi: 10.1007/s12224-020-09365-5
Zheng, G., Li, S. Q., Yang, X. D. (2015). Spider diversity in canopies of Xishuangbanna rainforest (China) indicates an alarming juggernaut effect of rubber plantations. For. Ecol. Manage. 338, 200–207. doi: 10.1016/j.foreco.2014.11.031
Keywords: clonal fragmentation, rubber plantation, physiological integration, restoration potential, epiphytic ferns
Citation: Yu X, Jin N, Bai R, Mo Y, Pu X, Li J and Lu H-Z (2024) Effects of clonal fragmentation on Pyrrosia nuda depend on growth stages in a rubber plantation. Front. Plant Sci. 15:1371040. doi: 10.3389/fpls.2024.1371040
Received: 15 January 2024; Accepted: 10 April 2024;
Published: 29 April 2024.
Edited by:
Wei Xue, Taizhou University, ChinaReviewed by:
Qiaoli Ayi, Southwest University, ChinaBi-Cheng Dong, Beijing Forestry University, China
Copyright © 2024 Yu, Jin, Bai, Mo, Pu, Li and Lu. This is an open-access article distributed under the terms of the Creative Commons Attribution License (CC BY). The use, distribution or reproduction in other forums is permitted, provided the original author(s) and the copyright owner(s) are credited and that the original publication in this journal is cited, in accordance with accepted academic practice. No use, distribution or reproduction is permitted which does not comply with these terms.
*Correspondence: Hua-Zheng Lu, luhuazheng@xtbg.ac.cn