- 1Department of Clinical Neurophysiology, University Medical Center – University of Göttingen, Göttingen, Germany
- 2Department of Medical Psychology and Medical Sociology, University of Göttingen, Göttingen, Germany
- 3Institute of Psychology, Friedrich Schiller University of Jena, Jena, Germany
- 4Clinic for Epileptology, Bonn, Germany
Stimulation using weak electrical direct currents has shown to be capable of inducing polarity-dependent diminutions or elevations in motor and visual cortical excitability. The aim of the present study was to test if reading during transcranial direct current stimulation (tDCS) is able to modify stimulation-induced plasticity in the visual cortex. Phosphene thresholds (PTs) in 12 healthy subjects were recorded before and after 10 min of anodal, cathodal, and sham tDCS in combination with reading. Reading alone decreased PTs significantly, compared to the sham tDCS condition without reading. Interestingly, after both anodal and cathodal stimulation there was a tendency toward smaller PTs. Our results support the observation that tDCS-induced plasticity is highly dependent on the cognitive state of the subject during stimulation, not only in the case of motor cortex but also in the case of visual cortex stimulation.
Introduction
Over the last 15 years transcranial direct current stimulation (tDCS) has become a promising tool in neuroplasticity research with perspectives in clinical neurophysiology (Nitsche and Paulus, 2011; Miniussi and Ruzzoli, 2013; Kuo et al., 2014). The primary effect of tDCS is a neuronal de- or hyperpolarization of transmembrane potentials (Creutzfeldt et al., 1962; Bindman et al., 1964), whereby the induced after-effects mainly depend on NMDA receptor-efficacy changes (Liebetanz et al., 2002). The most common way to evaluate cortical excitability changes induced by tDCS is by applying single-pulse transcranial magnetic stimulation (TMS) to the motor cortex (M1), since it allows the quantifiable measurements of its effects through the analysis of motor-evoked potentials (MEPs). In the resting muscle, anodal stimulation applied with 1 mA intensity for longer than 5 min increases the amplitude of MEPs, while cathodal stimulation decreases them (Nitsche and Paulus, 2000). With regard to the magnitude of the after effects induced by the stimulation besides the polarity, the combination of current strength – size of the stimulated area and duration of the stimulation are also relevant parameters (Agnew and McCreery, 1987; Batsikadze et al., 2013; Monte-Silva et al., 2013).
With regard to the visual cortex, it was shown in early animal experiments that the DC effect was less pronounced than on the M1, possibly due to the different cytoarchitecture, neurotransmitter level of the motor and visual areas and different spatial orientations of the neurons (Creutzfeldt et al., 1962). Later human studies confirmed these results, demonstrating that the tDCS after-effects are relatively short lasting in the visual areas compared to those of the M1, when using the same stimulation intensities and durations. Nevertheless, it was observed that the excitability of the visual cortex could be altered, as shown by the modulation of contrast thresholds (Antal et al., 2001; Kraft et al., 2010; Olma et al., 2011) and in the amplitude of the visual evoked potentials (Antal et al., 2004a; Accornero et al., 2007) after stimulation. The efficacy of tDCS over visual areas can also be demonstrated by measuring phosphene thresholds (PTs). TMS pulses delivered to the visual cortex can elicit visual sensations, called phosphenes (Meyer et al., 1991). The mean TMS intensity required to elicit phosphenes over multiple trials is defined as the PT. PT values are stable within subjects across time (Boroojerdi et al., 2000a, b). Therefore, the measurement of PTs is a frequently applied method in visual studies as a physiological index of cortical excitability, both in healthy participants and in clinical populations (Aurora et al., 1998, 2003; Mulleners et al., 2001; Brighina et al., 2002; Gerwig et al., 2005; Chadaide et al., 2007; Kammer and Baumann, 2010). In a previous work, we have elicited phosphenes by applying short trains of 5-Hz repetitive TMS delivered over the primary visual cortex (V1; Antal et al., 2003a). We found that cathodal stimulation over the V1 significantly increased PTs, probably due to diminished cortical excitability. Anodal stimulation resulted in the opposite effect, probably via induction of cortical hyperexcitability.
Previous studies suggested that a mental or physical activity in combination with tDCS applied over the M1 can modify the direction of stimulation-induced after-effects (Quartarone et al., 2004; Antal et al., 2007; Bradnam et al., 2010; Miyaguchi et al., 2013). Motor imagery undertaken following stimulation prolonged the effect of cathodal stimulation and abolished the effect of anodal stimulation (Quartarone et al., 2004). In another study, anodal stimulation combined with motor activity became inhibitory, while a cognitive task canceled the effect of both stimulation polarities (Antal et al., 2007). The effect of stimulation over M1 is highly dependent on the state (resting or active) of the muscle during stimulation (Bradnam et al., 2010; Miyaguchi et al., 2013). With regard to the combined stimulation of the visual cortex and any kind of activity, we are not aware of any published data. Therefore, the aim of this study was to investigate, whether a simple reading task can interact with tDCS applied over the visual cortex. We hypothesized that reading increases cortical excitability, and when combined with anodal stimulation this effect will be amplified. However, when it is in combination with cathodal stimulation, the net cortical excitability will not change.
Materials and Methods
Subjects
Twelve healthy volunteers (six male; aged between 18 and 30 years) were informed about all aspects of the study and gave written consent to participate. None of the subjects suffered from any neurological and psychological disorders, and neither had metallic implants/implanted electric devices nor took any medication regularly. The study conformed to the Declaration of Helsinki and the experimental protocol was approved by the local Ethics Committee.
Transcranial Direct Current Stimulation
Direct currents were transferred via a pair of saline-soaked surface sponge electrodes (5 cm × 7 cm) fixed to the scalp and delivered by a specially developed battery-driven current stimulator (NeuroConn GmbH, Ilmenau, Germany). One electrode was placed over the V1 (3 cm above the inion), while the other electrode was located at the Cz EEG position. The type of stimulation (anodal or cathodal) refers to the polarity of electrode above the V1. The subjects and investigators were blinded as to the polarity of tDCS in each experimental session. The current was applied for 10 min with an intensity of 1.0 mA. The fade in/fade out interval was set to 8 s. With regard to the sham stimulation there was only a 30 s stimulation with anodal or cathodal polarity.
PT Measurements
PTs were elicited using a MagPro-Stimulator (Medtronic Functional Diagnostics, Skovlunde, DK butterfly coil: MC-B70, biphasic pulse) and were measured before and after the tDCS sessions in order to determine the excitability of the V1. During the measurement, participants were seated in a comfortable chair. The coil was first positioned 2 cm over the inion with the handle pointing upward. In order to find the lowest PT (the best position for each individual participant) the coil was first moved to the left and then right side of the head in 1 cm steps. The optimal position was marked and measured using a measuring tape. Thereafter the stimulation was increased to suprathreshold intensity and was slowly decreased in 5%-steps until the participant reported the absence of the phosphenes. Around this value, the intensity of the stimulation was decreased and increased in 1%-steps until the participants reported seeing a visual sensation. This procedure was repeated four times and the intensity of the stimulation was recorded at each time. The means of PTs at a given time point were entered into the statistical analysis (see below).
Experimental Procedure
The experimental sessions were conducted in a repeated measurement design using a randomized order, with a break of at least 4 days between each session. There have been four experimental conditions: sham stimulation without reading, sham stimulation with reading, anodal stimulation with reading, and cathodal stimulation with reading. The volunteers were asked to bring a book that they are currently reading in their leisure time (this criteria excluded e-books, newspapers, and study material). The participants were seated in a comfortable chair. First the baseline PT measurement was performed. This was followed by the stimulation phase of the experiment, where, depending on the condition, subject received active stimulation combined with reading, or sham stimulation with or without reading (in the latter case, participants were instructed to sit passively during the stimulation phase, simply looking at the wall). PT measurements were repeated immediately, 10 and 20 min post-stimulation.
Statistical Analysis
The PT values were normalized to the baseline. Repeated-measures ANOVAs were used to test for differences in PTs with the factors CONDITION (sham vs. sham + reading; anodal vs. sham + reading; cathodal vs. sham + reading) and TIME (0, 10, and 20 min post-stimulation). Conditional on significant F-values, Fisher LSD test was used to describe the main effects or interactions as revealed by the ANOVA. A p-value of = 0.05 was considered significant. Data are given as mean ± SEM.
Results
All of the subjects tolerated tDCS and no side effects were reported during or after the stimulation.
With regard to the sham vs. sham + reading comparison rmANOVA revealed no main effect of CONDITION [F(1,11) = 0.25, p = 0.63, = 0.06]. A significant CONDITION and TIME interaction was found [F(2,22) = 4.31; p = 0.027, = 0.29]. The post hoc test revealed a significant PT decrease 20 min post-stimulation in the sham + reading condition compared to the sham condition (p = 0.009), and a reduction in PT values within the sham + reading condition between the measurements taken at 0 and 20 minutes post-stimulation (p = 0.009; Figure 1).
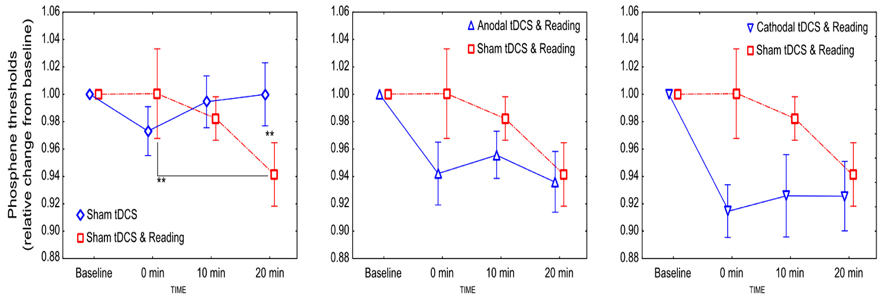
FIGURE 1. The effect of tDCS combined with reading on the excitability of the visual cortex. Values are normalized to baseline. Stars denote significant within- and between-condition differences. Error bars represent ±SEM.
When real stimulation was combined with reading, the PTs decreased independently from the stimulation polarity (Figure 1). Concerning the comparison of the sham + reading and anodal + reading conditions, rmANOVA revealed no significant effect of CONDITION [F(1,11) = 2.37, p = 0.15, = 0.18], TIME [F(2,22) = 2.55, p = 0.1, = 0.19] and CONDITION × TIME interaction [F(2,22) = 1.16; p = 0.33, = 0.09], although a small reduction in the PTs in the anodal + reading condition was observed.
Concerning the comparison of the sham + reading and cathodal + reading conditions, rmANOVA revealed a significant main effect of CONDITION [F(1,11) = 7.15, p = 0.022, = 0.39], showing that the PT values after cathodal stimulation were smaller compared to the sham + reading condition. However, the main effect of TIME [F(2,22) = 1.72, p = 0.21, = 0.14] and the CONDITION × TIME interaction [F(2,22) = 2.07; p = 0.15, = 0.16] were not significant.
Discussion
In the present study, we have demonstrated that reading alone can increase cortical excitability significantly. Furthermore, tDCS-induced neuronal plasticity over V1 was modified by reading: instead of showing the previously observed bidirectional response of anodal and cathodal stimulations on PTs (Antal et al., 2003a, b), application of both stimulation polarities resulted in a tendency toward an increase in cortical excitability that was significant after cathodal stimulation compared to sham. In a previous study examining task modulation of tDCS effects over the M1, it was reported that tDCS-induced alterations in cortical excitability could be modified by task engagement, for example by paying attention to a mental activity (IQ test) or by repeated contractions of the target muscle during stimulation (Antal et al., 2007). In this study, during the passive condition, anodal stimulation increased whilst cathodal stimulation decreased the amplitude of MEPs, as described by many previous studies. However, when performing a motor exercise, the M1 excitability was lower both after anodal and cathodal stimulation, when compared to the resting condition. Since the execution of a motor task alone can result in a decrease in cortical excitability, it was suggested that the stimulation here had no effect during the combined (tDCS + motor exercise) condition. We suppose that in the present study the significant increase in cortical excitability is due to the combined effect of the reading process and the electrical stimulation. Although phosphene perception can be used as a biomarker, when measuring visual cortical excitability (like the MEP amplitude with regard to the M1), PT detection is probably not a close enough analog to the MEP (Taylor et al., 2010). Furthermore, in the present study an increase in cortical excitability was observed after reading alone. Anodal stimulation probably facilitated this effect by further enhancing synaptic functioning. However, it is difficult to explain the excitability increasing effect of cathodal stimulation. This effect can be related to an increase in the signal-to-noise-ratio (Antal et al., 2004b) or in combination with reading, may induce metaplastic-like effects (Karabanov and Siebner, 2012).
Although the number of currently available studies assessing the effects of tDCS over the V1 is still limited, it has been repeatedly demonstrated that direct currents can alter visual performance bidirectionally (Antal et al., 2001, 2003a, b; Accornero et al., 2007; Kraft et al., 2010; Olma et al., 2011). However, the results are still contradictory. Efforts have been made to enhance our understanding of the reasons behind the reported heterogeneous effects, e.g., by explaining the impact of electrode position, target area of stimulation, and the type of task. Nevertheless, we suggest that one of the contributing factors behind the contradictory results might come from the fact that the experimental conditions during stimulation were not standardized (e.g., in several laboratories during an experimental session a variety of activities are permitted: for example, reading, surfing the internet, and eating are often allowed both during the stimulation itself and during pauses between repeated measurements, see also recent review, Horvath et al., 2014). Further studies systematically probing not only stimulation parameters but environmental effects might be needed to explore the reasons for the inconsistencies among studies examining electrical stimulation effects over the visual cortex.
Conflict of Interest Statement
The authors declare that the research was conducted in the absence of any commercial or financial relationships that could be construed as a potential conflict of interest.
Acknowledgments
The authors acknowledge support by the German Research Foundation and the Open Access Publication Funds of the University of Göttingen.
References
Accornero, N., Li Voti, P., La Riccia, M., and Gregori, B. (2007). Visual evoked potentials modulation during direct current cortical polarization. Exp. Brain Res. 178, 261–266. doi: 10.1007/s00221-006-0733-y
Agnew, W. F., and McCreery, D. B. (1987). Considerations for safety in the use of extracranial stimulation for motor evoked potentials. Neurosurgery 20, 143–147. doi: 10.1097/00006123-198701000-00030
Antal, A., Kincses, T. Z., Nitsche, M. A., and Paulus, W. (2003a). Manipulation of phosphene thresholds by transcranial direct current stimulation in man. Exp. Brain Res. 150, 375–378. doi: 10.1007/s00221-003-1459-8
Antal, A., Kincses, T. Z., Nitsche, M. A., and Paulus, W. (2003b). Modulation of moving phosphene thresholds by transcranial direct current stimulation of V1 in human. Neuropsychologia 41, 1802–1807. doi: 10.1016/S0028-3932(03)00181-7
Antal, A., Kincses, T. Z., Nitsche, M. A., Bartfai, O., and Paulus, W. (2004a). Excitability changes induced in the human primary visual cortex by transcranial direct current stimulation: direct electrophysiological evidence. Invest. Ophthalmol. Vis. Sci. 45, 702–707. doi: 10.1167/iovs.03-0688
Antal, A., Nitsche, M. A., Kruse, W., Kincses, T. Z., Hoffmann, K. P., and Paulus, W. (2004b). Direct current stimulation over V5 enhances visuomotor coordination by improving motion perception in humans. J. Cogn. Neurosci. 16, 521–527. doi: 10.1162/089892904323057263
Antal, A., Nitsche, M. A., and Paulus, W. (2001). External modulation of visual perception in humans. Neuroreport 12, 3553–3555. doi: 10.1097/00001756-200111160-00036
Antal, A., Terney, D., Poreisz, C., and Paulus, W. (2007). Towards unravelling task-related modulations of neuroplastic changes induced in the human motor cortex. Eur. J. Neurosci. 26, 2687–2691. doi: 10.1111/j.1460-9568.2007.05896.x
Aurora, S. K., Ahmad, B. K., Welch, K. M., Bhardhwaj, P., and Ramadan, N. M. (1998). Transcranial magnetic stimulation confirms hyperexcitability of occipital cortex in migraine. Neurology 50, 1111–1114. doi: 10.1212/WNL.50.4.1111
Aurora, S. K., Welch, K. M., and Al-Sayed, F. (2003). The threshold for phosphenes is lower in migraine. Cephalalgia 23, 258–263. doi: 10.1046/j.1468-2982.2003.00471.x
Batsikadze, G., Moliadze, V., Paulus, W., Kuo, M. F., and Nitsche, M. A. (2013). Partially non-linear stimulation intensity-dependent effects of direct current stimulation on motor cortex excitability in humans. J. Physiol. 591(Pt 7), 1987–2000. doi: 10.1113/jphysiol.2012.249730
Bindman, L. J., Lippold, O. C., and Redfearn, J. W. (1964). The action of brief polarizing currents on the cerebral cortex of the rat (1) during current flow and (2) in the production of ong-lasting after-effects. J. Physiol. 172, 369–382.
Boroojerdi, B., Bushara, K. O., Corwell, B., Immisch, I., Battaglia, F., Muellbacher, W., et al. (2000a). Enhanced excitability of the human visual cortex induced by short-term light deprivation. Cereb. Cortex 10, 529–534. doi: 10.1093/cercor/10.5.529
Boroojerdi, B., Prager, A., Muellbacher, W., and Cohen, L. G. (2000b). Reduction of human visual cortex excitability using 1-Hz transcranial magnetic stimulation. Neurology 54, 1529–1531. doi: 10.1212/WNL.54.7.1529
Bradnam, L. V., Stinear, C. M., Lewis, G. N., and Byblow, W. D. (2010). Task-dependent modulation of inputs to proximal upper limb following transcranial direct current stimulation of primary motor cortex. J. Neurophysiol. 103, 2382–2389. doi: 10.1152/jn.01046.2009
Brighina, F., Piazza, A., Daniele, O., and Fierro, B. (2002). Modulation of visual cortical excitability in migraine with aura: effects of 1 Hz repetitive transcranial magnetic stimulation. Exp. Brain Res. 145, 177–181. doi: 10.1007/s00221-002-1096-7
Chadaide, Z., Arlt, S., Antal, A., Nitsche, M. A., Lang, N., and Paulus, W. (2007). Transcranial direct current stimulation reveals inhibitory deficiency in migraine. Cephalalgia 27, 833–839. doi: 10.1111/j.1468-2982.2007.01337.x
Creutzfeldt, O. D., Fromm, G. H., and Kapp, H. (1962). Influence of transcortical d-c currents on cortical neuronal activity. Exp. Neurol. 5, 436–452. doi: 10.1016/0014-4886(62)90056-0
Gerwig, M., Niehaus, L., Kastrup, O., Stude, P., and Diener, H. C. (2005). Visual cortex excitability in migraine evaluated by single and paired magnetic stimuli. Headache 45, 1394–1399. doi: 10.1111/j.1526-4610.2005.00272.x
Horvath, J. C., Carter, O., and Forte, J. D. (2014). Transcranial direct current stimulation: five important issues we aren’t discussing (but probably should be). Front. Syst. Neurosci. 8:2. doi: 10.3389/fnsys.2014.00002
Kammer, T., and Baumann, L. W. (2010). Phosphene thresholds evoked with single and double TMS pulses. Clin. Neurophysiol. 121, 376–379. doi: 10.1016/j.clinph.2009.12.002
Karabanov, A., and Siebner, H. R. (2012). Unravelling homeostatic interactions in inhibitory and excitatory networks in human motor cortex. J. Physiol. 590, 5557–5558. doi: 10.1113/jphysiol.2012.244749
Kraft, A., Roehmel, J., Olma, M. C., Schmidt, S., Irlbacher, K., and Brandt, S. A. (2010). Transcranial direct current stimulation affects visual perception measured by threshold perimetry. Exp. Brain Res. 207, 283–290. doi: 10.1007/s00221-010-2453-6
Kuo, M. F., Paulus, W., and Nitsche, M. A. (2014). Therapeutic effects of non-invasive brain stimulation with direct currents (tDCS) in neuropsychiatric diseases. Neuroimage 85(Pt 3), 948–960. doi: 10.1016/j.neuroimage.2013.05.117
Liebetanz, D., Nitsche, M. A., Tergau, F., and Paulus, W. (2002). Pharmacological approach to the mechanisms of transcranial DC-stimulation-induced after-effects of human motor cortex excitability. Brain 125, 2238–2247. doi: 10.1093/brain/awf238
Meyer, B. U., Diehl, R., Steinmetz, H., Britton, T. C., and Benecke, R. (1991). Magnetic stimuli applied over motor and visual cortex: influence of coil position and field polarity on motor responses, phosphenes, and eye movements. Electroencephalogr. Clin. Neurophysiol. Suppl. 43, 121–134.
Miniussi, C., and Ruzzoli, M. (2013). Transcranial stimulation and cognition. Handb. Clin. Neurol. 116, 739–750. doi: 10.1016/B978-0-444-53497-2.00056-5
Miyaguchi, S., Onishi, H., Kojima, S., Sugawara, K., Tsubaki, A., Kirimoto, H., et al. (2013). Corticomotor excitability induced by anodal transcranial direct current stimulation with and without non-exhaustive movement. Brain Res. 1529, 83–91. doi: 10.1016/j.brainres.2013.07.026
Monte-Silva, K., Kuo, M. F., Hessenthaler, S., Fresnoza, S., Liebetanz, D., Paulus, W., et al. (2013). Induction of late LTP-like plasticity in the human motor cortex by repeated non-invasive brain stimulation. Brain Stimul. 6, 424–432. doi: 10.1016/j.brs.2012.04.011
Mulleners, W. M., Chronicle, E. P., Palmer, J. E., Koehler, P. J., and Vredeveld, J. W. (2001). Visual cortex excitability in migraine with and without aura. Headache 41, 565–572. doi: 10.1046/j.1526-4610.2001.041006565.x
Nitsche, M. A., and Paulus, W. (2000). Excitability changes induced in the human motor cortex by weak transcranial direct current stimulation. J. Physiol. 527(Pt 3), 633–639. doi: 10.1111/j.1469-7793.2000.t01-1-00633.x
Nitsche, M. A., and Paulus, W. (2011). Transcranial direct current stimulation – update 2011. Restor. Neurol. Neurosci. 29, 463–492. doi: 10.3233/RNN-2011-0618
Olma, M. C., Kraft, A., Roehmel, J., Irlbacher, K., and Brandt, S. A. (2011). Excitability changes in the visual cortex quantified with signal detection analysis. Restor. Neurol. Neurosci. 29, 453–461. doi: 10.3233/RNN-2011-0607
Quartarone, A., Morgante, F., Bagnato, S., Rizzo, V., Sant’angelo, A., Aiello, E., et al. (2004). Long lasting effects of transcranial direct current stimulation on motor imagery. Neuroreport 15, 1287–1291. doi: 10.1097/01.wnr.0000127637.22805.7c
Keywords: tDCS, visual cortex excitability, phosphene, TMS
Citation: Antal A, Ambrus GG and Chaieb L (2014) Toward unraveling reading-related modulations of tDCS-induced neuroplasticity in the human visual cortex. Front. Psychol. 5:642. doi: 10.3389/fpsyg.2014.00642
Received: 12 February 2014; Accepted: 05 June 2014;
Published online: 20 June 2014.
Edited by:
Olga Lucía Gamboa Arana, Goethe-University Frankfurt am Main, GermanyCopyright © 2014 Antal, Ambrus and Chaieb. This is an open-access article distributed under the terms of the Creative Commons Attribution License (CC BY). The use, distribution or reproduction in other forums is permitted, provided the original author(s) or licensor are credited and that the original publication in this journal is cited, in accordance with accepted academic practice. No use, distribution or reproduction is permitted which does not comply with these terms.
*Correspondence: Andrea Antal, Department of Clinical Neurophysiology, University Medical Center – University of Göttingen, Robert Koch Straβe 40, 37075 Göttingen, Germany e-mail:YWFudGFsQGd3ZGcuZGU=