- 1Research Group Neuroprotection, German Center for Neurodegenerative Diseases (DZNE), Magdeburg, Germany
- 2Department of Neurology, Medical Faculty, Otto von Guericke University, Magdeburg, Germany
- 3Department Performance, Neuroscience, Therapy and Health, Medical School Hamburg, University of Applied Sciences and Medical University, Hamburg, Germany
- 4Center for Behavioral Brain Sciences, Magdeburg, Germany
In general, it is well recognized that both acute physical exercises and regular physical training influence brain plasticity and cognitive functions positively. However, growing evidence shows that the same physical exercises induce very heterogeneous outcomes across individuals. In an attempt to better understand this interindividual heterogeneity in response to acute and regular physical exercising, most research, so far, has focused on non-modifiable factors such as sex and different genotypes, while relatively little attention has been paid to exercise prescription as a modifiable factor. With an adapted exercise prescription, dosage can be made comparable across individuals, a procedure that is necessary to better understand the dose–response relationship in exercise–cognition research. This improved understanding of dose–response relationships could help to design more efficient physical training approaches against, for instance, cognitive decline.
Introduction
In the last decades, the average time that people are physically active has decreased dramatically in Western countries (Owen et al., 2010; Church et al., 2011; Copeland et al., 2015), and physical inactivity has been named as a big, if not even the biggest, health problem of the twenty-first century (Blair, 2009). Remarkably, physical inactivity is associated with impaired cognitive functions (Aichberger et al., 2010; Falck et al., 2016; Ku et al., 2017; Tan et al., 2017) and higher risk of risk of neurodegenerative diseases (e.g., dementia) in the aging population (Laurin et al., 2001; Rovio et al., 2005; Ravaglia et al., 2008; Hamer and Chida, 2009; Paillard-Borg et al., 2009; Abe, 2012; Bowen, 2012; de Bruijn et al., 2013; Grande et al., 2014; Paillard, 2015). In order to counteract such negative effects of physical inactivity, an increase in the habitual physical activity level, which is typically engendered through a regular engagement in physical exercises, is empathically recommended (Hillman et al., 2008; Bherer et al., 2013; Erickson et al., 2013, 2014; Hötting and Röder, 2013; Paillard, 2015; Müller et al., 2017; Liu-Ambrose et al., 2018; Herold et al., 2019). It has been well demonstrated in the literature that a single bout of physical exercises (Chang et al., 2012a; Basso and Suzuki, 2017; Herold et al., 2018b; McSween et al., 2018; Moreau and Chou, 2019) as well as repeated sessions of physical exercises in the form of a training intervention (e.g., endurance training) (Colcombe et al., 2006; Erickson et al., 2011; Voelcker-Rehage et al., 2011; Herold et al., 2018b, 2019; Falck et al., 2019; Stern et al., 2019) induce substantial neurocognitive changes. Based on such positive effects of physical exercises and/or physical training on brain plasticity and on cognition, it is not surprising that many scientific disciplines (e.g., medicine, psychology, neuroscience, and sport science) pay attention to this research field. Although these different scientific disciplines use different approaches to understand the relationship between physical exercises and/or physical training and the central nervous system, it is undoubted that all of them are based on an appropriate exercise prescription that specifies exercise (e.g., exercise intensity, exercise duration) and/or training variables (e.g., frequency of training sessions) (Lightfoot, 2008; Williams et al., 2019). Furthermore, exercise prescription is the key for dosing (Wasfy and Baggish, 2016; Pontifex et al., 2018) and for individualization of acute physical exercises and physical training (Lightfoot, 2008). Individualization (personalizing) is an emerging approach aiming at maximizing the efficiency of an intervention by accounting for the interindividual heterogeneity in the response to acute physical exercises and/or physical training (Lightfoot, 2008; Buford et al., 2013; Barha et al., 2017b; Müller et al., 2017, 2018; Gallen and D’Esposito, 2019). Notably, what parameters are optimal to prescribe the best exercise for an individual is extensively discussed in the literature (Katch et al., 1978; Weltman et al., 1989, 1990; Gass et al., 1991; Meyer et al., 1999; Hofmann and Tschakert, 2010; Scharhag-Rosenberger et al., 2010; Mann et al., 2013; Weatherwax et al., 2016), but not all scientific disciplines investigating exercise–cognition are taking this issue into account sufficiently (Gronwald et al., 2018b, 2019a; Suwabe et al., 2018). Hence, the purpose of this article is to shed light on differences in exercise prescription and their relation to the dose and the interindividual heterogeneity in neurocognitive outcome measures.
Physical Activity, Physical Exercises, Physical Training – Where Are the Differences?
Prior to going more deeply into the topic of physical activity, physical exercise, and/or physical training, it is necessary to clarify these terms because they represent different concepts while it is, unfortunately, common behavior to use them interchangeably (Caspersen et al., 1985; Budde et al., 2016). “Physical activity” is defined as muscle-induced bodily movement that increases energy expenditure above ∼1.0/1.5 MET (metabolic equivalent of task; 1 MET = 1 kcal (4,184 kJ) × kg–1 × h–1) (Caspersen et al., 1985; Ainsworth et al., 2000; Budde et al., 2016). Hence, the term physical activity is a hypernym (i) that covers a wide range of physical activities that are conducted on a regular or unregular basis in a relatively unstructured and unplanned manner and (ii) that includes specific, planned, and structured forms of physical activities that are known as physical exercises (Caspersen et al., 1985; Howley, 2001; Budde et al., 2016). Physical exercises should be distinguished based on temporal characteristics into acute physical exercise (single bout) and chronic physical exercises (repeated bouts of acute exercises) (Scheuer and Tipton, 1977; Budde et al., 2016). A single bout of physical exercise is commonly referred to as an “acute (single) bout of physical exercise” or as “acute physical exercises” (Budde et al., 2016; Herold et al., 2018b). Furthermore, chronic physical exercises can be denoted as “physical training” when they are conducted regularly in a planned, structured, and purposive manner with the objective to increase (or maintain) individual capabilities in one or multiple fitness dimensions (Scheuer and Tipton, 1977; Caspersen et al., 1985; Howley, 2001; Budde et al., 2016; Herold et al., 2018a). In essence, distinguishing and using these terms carefully allows a better classification and interpretation of observed effects and of the underlying (neuro)biological mechanisms (Budde et al., 2016).
“Responder” or “Non-responder” – That Is the Question
Since every human is unique, there is a considerable amount of within-individual (intra-individual) (Katch et al., 1982; Coggan and Costill, 1984; Bagger et al., 2003; Skurvydas et al., 2010; Faude et al., 2017; Chrzanowski-Smith et al., 2019; Voisin et al., 2019) and between-individual (interindividual) heterogeneity (Karavirta et al., 2011; Chmelo et al., 2015; Bonafiglia et al., 2016; Greenham et al., 2018) in acute psychophysiological response(s) to the same acute physical exercises and/or long-term adaptions to the same physical training. Especially, the interindividual heterogeneity gained attention in the research of the recent years (Buford and Pahor, 2012; Buford et al., 2013; Mann et al., 2014; Sparks, 2017; Pickering and Kiely, 2018b; Ross et al., 2019) and is commonly observed in studies dealing with endurance (cardiovascular) training (Chmelo et al., 2015; Bonafiglia et al., 2016), resistance (strength) training (Hubal et al., 2005; Chmelo et al., 2015; Ahtiainen et al., 2016), or combined training (consisting of endurance and resistance training) (Karavirta et al., 2011). In order to account for this interindividual heterogeneity, the concept of (i) “responder” [also referred as “individuals with high sensitivity” (Booth and Laye, 2010)] and (ii) “non-responder” [also referred as “individuals with low-sensitivity” (Booth and Laye, 2010), limited responders (Burley et al., 2018), or “individuals which did not respond” (Pickering and Kiely, 2018b)] was introduced, however, with varying definitions (Booth and Laye, 2010; Buford and Pahor, 2012; Scharhag-Rosenberger et al., 2012; Buford et al., 2013; Mann et al., 2014). While the definition and methods to classify responders and non-responders are currently discussed in the literature (Atkinson and Batterham, 2015; Hecksteden et al., 2015, 2018; Bonafiglia et al., 2018, 2019a, b; Swinton et al., 2018; Atkinson et al., 2019; Dankel and Loenneke, 2019), it is relatively accepted that (i) not all outcome variables are affected equally by the responsiveness state (e.g., be a responder or non-responder) (Sparks, 2017; Pickering and Kiely, 2018b, 2019b; Toigo, 2019), (ii) measurement errors are inevitable in repeated measurements and are caused, for instance, by random biological fluctuations that do not represent a meaningful change in the outcome variable (Atkinson and Nevill, 1998; Scharhag-Rosenberger et al., 2012; Atkinson and Batterham, 2015; Williamson et al., 2017; Pickering and Kiely, 2019a), and (iii) some responses are likely to be transient, causing uncertainty regarding the time course of the responsiveness state (Pickering and Kiely, 2018b). Hence, the following working definitions can be proposed (see Table 1). Regarding the response to acute physical exercises and/or physical training, (i) responders are individuals who exhibit, at a certain time point, changes in a variable of interest that are above (below) a distinct threshold, and (ii) non-responders are individuals who exhibit, at a certain time point, changes in a variable of interest that are below (above) a distinct threshold. There is ongoing vivid discussion on how to define these critical thresholds (Atkinson and Batterham, 2015; Swinton et al., 2018; Atkinson et al., 2019; Dankel and Loenneke, 2019) and whether further subgroups should be established (Dankel and Loenneke, 2019). For instance, “adverse responders” (Bouchard et al., 2012) or “negative responders” (Leifer et al., 2014), have be defined as individuals who exhibit, at a certain time point, in response to acute physical exercise or physical training, unfavorable responses below (above) a distinct threshold. In addition, “above” and “below” need to be referenced relative to a specific outcome in the variable of interest. For instance, in a cognitive test, performance could be operationalized by “number of correct items” and “reaction time” (variables of interest). Regarding number of correct items, it is favorable to achieve a higher number of correct items (responder: above; non-responder: below). Regarding reaction time, on the other hand, it is favorable to react faster (responder: below; non-responder: above). Regardless of the ongoing discussion about how to classify the level of responsiveness, there is some evidence that the interindividual heterogeneity in response to acute physical exercise and/or physical training might contribute to the interindividual heterogeneity observed in neurocognitive outcomes. This evidence is outlined in the following section.
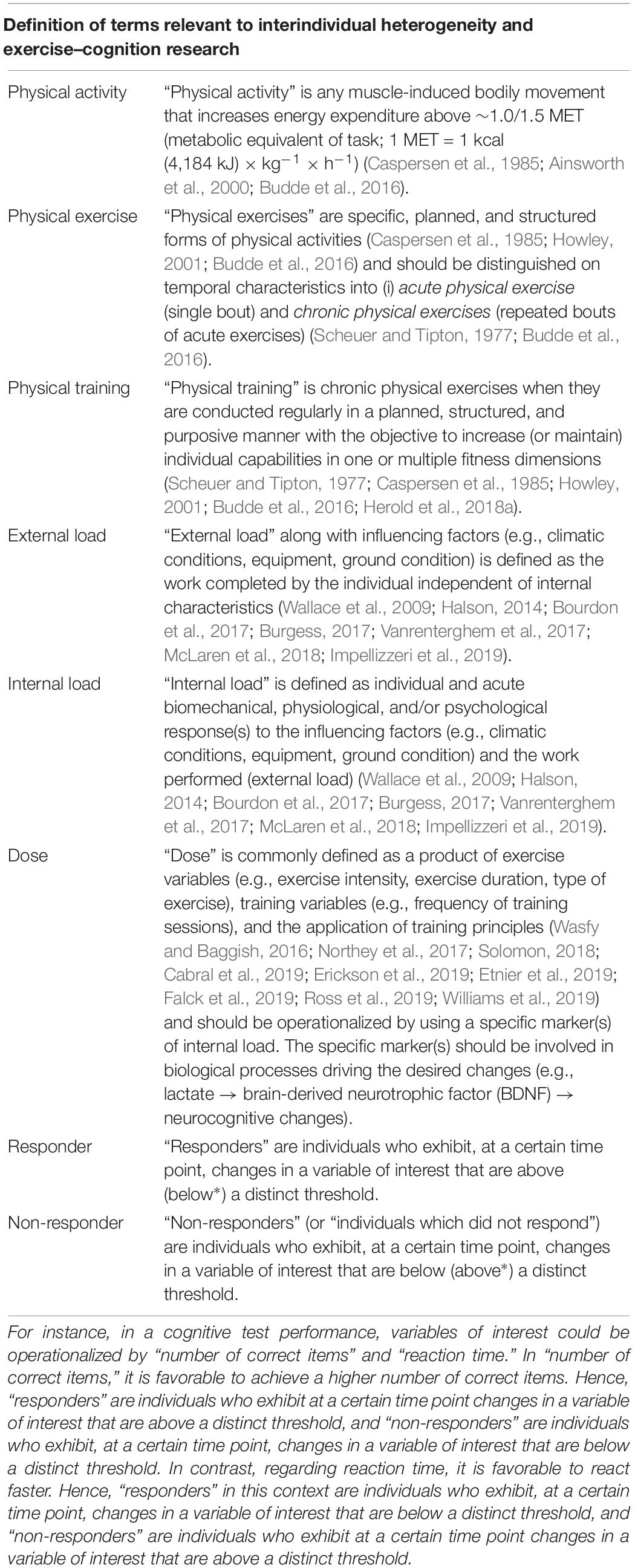
Table 1. Overview about the definitions of terms relevant to interindividual heterogeneity and exercise–cognition research ∗ Please note that “above” and “below” are relative to the favorable outcome in the variable of interest.
Responsiveness State, Functional and Structural Brain Changes, and Cognition
In the following, we will refer to acute endurance exercises and endurance training because (i) from a neuroevolutionary view, endurance capacities (e.g., running during foraging) are important to ensure physical and/or neurocognitive well-functioning (Mattson, 2012; Raichlen and Alexander, 2017), (ii) acute endurance exercises and/or endurance training are currently in the focus of exercise–cognition research (Hillman et al., 2008; Stimpson et al., 2018), (iii) endurance training induces substantial structural brain changes (Erickson et al., 2011; Stern et al., 2019), and (iv) endurance training entails greater benefits in cognitive performance than resistance training (Barha et al., 2017a).
Acute Physical Exercises
With regard to acute physical exercises, it was observed that individual baseline working memory function was linked with changes in working memory performance following acute very-light-to-moderate-intensity endurance exercises (Yamazaki et al., 2018). Furthermore, responders, who showed improved cognitive performance after a single bout of very-light-to-moderate-intensity endurance exercise, exhibited a higher level of prefrontal activation during exercising (Yamazaki et al., 2017). This finding suggests that not only peripheral systems are affected by the individual responsiveness state but also the central nervous system itself.
Physical Training
Changes in cardiorespiratory fitness (CRF) measures in response to a 20 weeks endurance training program tended to vary tremendously among individuals (Bouchard et al., 1999). Exemplarily, Karavirta et al. (2011) observed, after a 21 weeks long combined exercise intervention in older adults, changes in CRF levels [assessed via maximal oxygen uptake (VO2 max.)] ranging from -8 to 42%. Such interindividual differences in response to endurance training also affect the expression of neurotrophic factors [e.g., brain-derived neurotrophic factor (BDNF)] (Heisz et al., 2017), which play an important role in neuroplasticity (Erickson et al., 2010, 2011; Brigadski and Leßmann, 2014) and cognition (Erickson et al., 2010; Brigadski and Leßmann, 2014; Leckie et al., 2014). For instance, after 6 weeks of high-intensity endurance training, responders who improved their CRF [assessed via peak oxygen uptake (VO2 peak)] to a greater extent than non-responders exhibited a significantly higher increase in serum BDNF (Heisz et al., 2017). This finding suggests that the state of responsiveness influences important mechanisms involved in neuroplasticity and cognition. Notably, a higher level of CRF level [mostly operationalized by VO2 max. (highest value attainable by a subject) or VO2 peak (highest, “system-limited” value attained during the CRF test) (Day et al., 2003)] is associated with (i) better cognitive performance in younger adults (Suwabe et al., 2017; Wengaard et al., 2017; Fortune et al., 2019), older adults (Erickson et al., 2011; Bugg et al., 2012; Verstynen et al., 2012; Albinet et al., 2014; Freudenberger et al., 2016; Hayes et al., 2016; Bherer et al., 2019; Castalanelli et al., 2019; Pentikäinen et al., 2019), and older adults with mild cognitive impairments (Sobol et al., 2018); (ii) favorable functional brain changes in younger adults (Stillman et al., 2018) and older adults (Colcombe et al., 2004; Albinet et al., 2014; Dupuy et al., 2015; Hyodo et al., 2016); and (iii) favorable structural brain changes in older adults (Colcombe et al., 2003; Erickson et al., 2009; Szabo et al., 2011; Bugg et al., 2012) and individuals with Alzheimer’s disease (Burns et al., 2008; Honea et al., 2009; Vidoni et al., 2012). Furthermore, in response to endurance training, increases in VO2 max. (iv) mediate the improvement in cognitive functions in younger adults (Stern et al., 2019) and (v) are associated with increases in hippocampal volumes in older adults (Erickson et al., 2011).
In sum, based on these associations between measures of CRF, measures of brain function and structure, and cognitive performance measures (albeit these correlations are not strictly causal in nature), it seems plausible to hypothesize that the large interindividual heterogeneity in measures of CRF may also contribute, among other factors, to the interindividual heterogeneity in measures of neurocognition in response to endurance training. However, to clarify the validity of these assumptions, further research is required.
Master (of) the Fate? – How Genetics and Lifestyle Contribute to Interindividual Heterogeneity
The interindividual responsiveness to physical exercises and/or physical training and, in turn, the interindividual heterogeneity in outcomes are caused by several moderators, including both non-modifiable factors (e.g., sex or genotypes) and modifiable factors (e.g., nutrition, social or cognitive activities, exercise prescription) (Spiering et al., 2008; Bamman et al., 2014; Mann et al., 2014; Erickson et al., 2015; Sparks, 2017; Pickering and Kiely, 2018b). Notably, these factors can also be classified as endogenous factors (factors attributable to the individual such as sex or genotypes) and exogenous factors (factors attributable to external inputs, e.g., generated by exercise prescription) (Sparks, 2017). Currently, the roles of non-modifiable (endogenous) factors such as sex (Barha et al., 2017a, b, 2019; Barha and Liu-Ambrose, 2018; Cobbold, 2018; Loprinzi and Frith, 2018; Dao et al., 2019) and genotypes (Booth and Laye, 2010; Timmons et al., 2010; Timmons, 2011; Bouchard, 2012, 2019; Mann et al., 2014; Bouchard et al., 2015; Jones et al., 2016; Pickering and Kiely, 2017a, b,c,2018a; Del Coso et al., 2018) are investigated most. Among these factors, it has been shown that a considerable amount (approximately up to half of the variance) of the interindividual heterogeneity in physical outcomes (Bouchard and Rankinen, 2001; Timmons et al., 2010; Davidsen et al., 2011; Timmons, 2011; Bouchard, 2012; Wilson et al., 2019), cognitive outcomes (McClearn, 1997; Goldberg and Weinberger, 2004; Blokland et al., 2008; Erickson et al., 2008; Friedman et al., 2008; Canivet et al., 2015, 2017), and brain structure outcomes (Thompson et al., 2001; Toga and Thompson, 2005; Bueller et al., 2006) are explained by genetics. However, considering current evidence, lifestyle factors may equalize a “genetic handicap” since people with a high CRF level but “unfavorable” genetic polymorphisms do not need to perform significantly poorer than individuals with low CRF level but “favorable” genetic equipment (Brown et al., 2019). These findings suggest that a genetic handicap can be counteracted by other factors (Flück, 2018) and that “overemphasizing” genetics for the individualization of exercise prescriptions is counter-productive (Carlsten and Burke, 2006; Kohane, 2009; Horwitz et al., 2013; Joyner and Lundby, 2018; Peck, 2018; Joyner, 2019). However, analysis of the genetics of participants is undoubtedly helpful in supporting individualization of acute physical exercise and/or physical training by aiding, for instance, the identification of potential responders and non-responders (Lightfoot, 2008; Pescatello, 2008; Booth and Laye, 2010; Timmons et al., 2010; Timmons, 2011; Pickering and Kiely, 2019a, b). Remarkably, it has also been highlighted that no “global non-responders” exist (Ross et al., 2015; Bonafiglia et al., 2016; Montero and Lundby, 2017; Pickering and Kiely, 2017c, 2018b; Toigo, 2019). Moreover, it is assumed that non-responsiveness can best be counteracted by modifying the dose of the physical exercise and/or physical training (Churchward-Venne et al., 2015; Ross et al., 2015; Montero and Lundby, 2017; Toigo, 2019). The latter suggests that the dose of physical interventions per se contributes significantly to the observed interindividual heterogeneity in (neurocognitive) outcomes.
What Dose (It) Means?
The terminus dose is differently defined in the literature (Voils et al., 2012), but in exercise(-cognition) research, “dose” is commonly referred to as the product of exercise variables (e.g., exercise intensity, exercise duration, type of exercise; see Table 2) when considering an acute bout of physical exercises (Wasfy and Baggish, 2016; Pontifex et al., 2018). In training studies, dose can be seen as the product of exercise variables (e.g., exercise intensity, exercise duration, type of exercise), training variables (e.g., frequency of training sessions), and the application of training principles (Wasfy and Baggish, 2016; Northey et al., 2017; Solomon, 2018; Cabral et al., 2019; Erickson et al., 2019; Etnier et al., 2019; Falck et al., 2019; Ross et al., 2019; Williams et al., 2019). In reverse, dose could be modified in acute physical exercise studies by adjusting the exercise variables, while in physical training studies, exercise variables, training variables, and training principles must be taken into account (see Table 2). Such a purposeful modification is referred to as the adjustment of the exercise prescription.
In the context of exercise prescription, it is also imperative to clarify the terms “external load” and “internal load.” While external load along with influencing factors (e.g., climatic conditions, equipment, ground condition) is defined as the work completed by the individual independent of internal characteristics (Wallace et al., 2009; Halson, 2014; Bourdon et al., 2017; Burgess, 2017; Vanrenterghem et al., 2017; McLaren et al., 2018; Impellizzeri et al., 2019), internal load is defined as individual and acute biomechanical, physiological, and/or psychological response(s) to the influencing factors and the work performed (Wallace et al., 2009; Halson, 2014; Bourdon et al., 2017; Burgess, 2017; Vanrenterghem et al., 2017; McLaren et al., 2018; Impellizzeri et al., 2019). According to the definition of internal load, which states that internal load is characterized by the individual and acute psychophysiological response(s) to the external load, it appears that internal load can be adjusted by modifying the external load. However, given that exercise variables such as exercise intensity can be operationalized using parameters of either external load (e.g., running with a speed of 10 km/h) or internal load (e.g., running with 60 of maximal heart rate), current definitions of dose are rather broad. Since dose is an essential factor for triggering neurobiological processes (e.g., release of neurotrophins such as BDNF; Dinoff et al., 2017), which in turn lead to neuroplastic and cognitive changes (Cotman et al., 2007; Voss et al., 2011, 2013a; Lucas et al., 2015; Zimmer et al., 2015; Basso and Suzuki, 2017; Stimpson et al., 2018), it is crucial to agree on an appropriate concept of dose. Although markers of internal load could be more difficult to measure (compared to markers of external load), we suggest that dose should be operationalized by using a specific marker or specific markers of internal load as a proxy. The two reasons for this assumption are outlined in the following.
Why Internal Load Should Be Used as a Proxy for Dose
Given (i) that internal load equals, per definition, the individual and acute psychophysiological response(s) to a given external load (Wallace et al., 2009; Halson, 2014; Bourdon et al., 2017; Burgess, 2017; Vanrenterghem et al., 2017; McLaren et al., 2018; Impellizzeri et al., 2019) and (ii) that neurocognitive changes are triggered by such distinct psychophysiological responses (Cotman et al., 2007; Zimmer et al., 2015; Basso and Suzuki, 2017; Stimpson et al., 2018), it seems reasonable to assume that internal load is a better proxy for dose than external load.
Why a Specific Marker of Internal Load Is Needed as a Proxy for Dose
There are several markers of internal load that can be used to prescribe the exercise intensity in acute endurance exercises and/or endurance training [e.g., oxygen uptake, heart rate, or heart rate variability (HRV)]. For instance, HRV, i.e., the beat-to-beat variation over a distinct time period, under rest conditions or while exercising is an interesting marker of internal load because the internal load quantification by HRV indices is progressive and takes the individual fitness level as well as daily readiness and actual health state into account (Thayer et al., 2012; Plews et al., 2013; Vesterinen et al., 2013, 2016; Gronwald et al., 2016, 2018a, 2019b, 2019c). Furthermore, resting-state HRV is associated with cognitive performance (Hansen et al., 2003; Frewen et al., 2013; Gillie et al., 2014; Zeki Al Hazzouri et al., 2014; Colzato et al., 2018).
However, currently, several hypotheses exist in literature that explain the positive effects of acute physical exercises and physical training on brain plasticity and cognition (Kramer et al., 1999; Smiley-Oyen et al., 2008; Davenport et al., 2012; McMorris and Hale, 2015; McMorris, 2016a,b,c,d; McMorris et al., 2016; Voss, 2016; Raichlen and Alexander, 2017; Pontifex et al., 2018; Stimpson et al., 2018; Audiffren and André, 2019). Among them, one of the most popular hypotheses is the “neurotrophic hypothesis,” which posits that in response to physical exercises, the organism releases several neurochemicals (e.g., neurotrophic factors such as BDNF), which in turn trigger neuroplasticity and facilitate cognitive enhancement (Voss et al., 2013b; Basso and Suzuki, 2017; Stimpson et al., 2018; Audiffren and André, 2019). Hence, it seems more promising to use a marker or markers of internal load that are related to changes in neurotrophic molecules in order to individualize and adjust exercise prescription (Pedersen, 2019). In this regard, the peripheral level of blood lactate could be a promising marker of internal load because peripheral blood lactate (e.g., from muscles) can cross the blood–brain barrier and provides energy to the brain (Kemppainen et al., 2005; Quistorff et al., 2008; van Hall et al., 2009; Dennis et al., 2015; Proia et al., 2016; Taher et al., 2016; Riske et al., 2017; Brooks, 2018; Sobral-Monteiro-Junior et al., 2019). Hence, it is not surprising that relative changes in peripheral levels of blood lactate are correlated significantly with cognitive performance levels after high-intensity interval endurance exercises (Lee et al., 2014; Tsukamoto et al., 2016; Hashimoto et al., 2017). The crucial role of blood lactate for neuroplasticity is further emphasized by findings of peripheral blood lactate levels being associated with the peripheral serum BDNF levels (Ferris et al., 2007; Schiffer et al., 2011). However, the exact molecular mechanisms of increased BDNF production in response to physical exercising are not fully understood (for review, see Jiménez-Maldonado et al., 2018). BDNF in the brain is involved in neuroplasticity (Brigadski and Leßmann, 2014), and serum levels of BDNF have been shown to be directly linked to cognitive performance after an acute bout of high-intensity endurance exercises (Hwang et al., 2016). Moreover, (i) serum BDNF mediates improvements in cognitive functions following a 1-year aerobic endurance training (Leckie et al., 2014), (ii) greater serum BDNF concentration changes in response to a 1-year-long aerobic endurance training are linked to hippocampal volume changes (Erickson et al., 2011), and (iii) reduced levels of serum BDNF are related to a decline in hippocampal volume and poorer memory performance (Erickson et al., 2010). In sum, a specific marker or specific markers of internal load such as the peripheral blood lactate level seems to constitute a promising proxy for dose. However, the optimal marker(s) that is (are), with regard to neuroplasticity and cognition, the most suitable proxy for the dose of physical exercise and/or physical training has yet to be discovered.
Become Personal – How to Individualize the Exercise Prescription?
Based on the large interindividual heterogeneity (i) in psychophysiological responses to acute physical exercises and (ii) in long-term adaptions to a physical training, it is assumed that tailoring of these to the characteristics and needs of a particular person is well suited to maximize their efficiency (Buford and Pahor, 2012; Buford et al., 2013; Müller et al., 2017, 2018; Cobbold, 2018; Pickering and Kiely, 2018b). Such an individualization of acute physical exercises and/or physical training could be achieved by adjusting the exercise prescription (e.g., exercise intensity) (Lightfoot, 2008), which influences, in turn, the dose (objectified by a specific marker or specific markers of internal load; see previous section and Figure 1A). In order to illustrate our thoughts in practical terms, we focus on exercise intensity because a full discussion of all exercise variables, training variables, and training principles is beyond the scope of this article. As outlined in the previous section, using markers of internal load to prescribe exercise intensity is preferable instead of using parameters of external load such as speed in running specific exercises. Therefore, traditional markers of internal load such as the fixed percentage of the maximally achievable value of oxygen uptake or heart rate are often used (Garber et al., 2011; Suwabe et al., 2018). Using a fixed percentage of a maximally achievable value of oxygen uptake or heart rate involves a considerable amount of interindividual heterogeneity in other markers of internal load (e.g., metabolic responses objectified by, for instance, peripheral blood lactate) (Weltman et al., 1989, 1990; Meyer et al., 1999; Vollaard et al., 2009; Scharhag-Rosenberger et al., 2010). Metabolic responses (e.g., peripheral blood lactate level) constitute specific markers of internal load that are likely to be proxies for the dose that triggers neuroplastic processes and cognitive changes (see “Why a specific marker of internal load is needed as a proxy for dose”). Hence, traditional exercise prescriptions lead to largely varying individual doses as revealed by the marker(s) of internal load. This may lead, among other factors, to the observed interindividual heterogeneity in neurocognitive outcomes (see Figure 1B). Consequently, approaches that ensure that a comparable dose is provided to each individual (e.g., adapted exercise prescriptions that ensure a comparable level of peripheral blood lactate) may lower the interindividual heterogeneity regarding neurocognitive outcomes. Hence, such approaches are favorable in exercise–cognition research (see Figure 1). In this context, individual threshold concepts (aerobic and anaerobic threshold) that are based on individual metabolic (or respiratory) responses could be used to determine an individual’s initial exercise intensity (Meyer et al., 1999; Hofmann and Tschakert, 2010; Scharhag-Rosenberger et al., 2010; Weatherwax et al., 2016). However, while there is a strong theoretical basis for the application of a threshold-based exercise prescription for endurance exercises and endurance training, the challenges and pitfalls of determining such individual thresholds may explain why many researchers continue to favor exercise intensity prescriptions based on relative percentages of maximum values (Hofmann and Tschakert, 2010; Mann et al., 2013). Although our assumptions are well grounded on possible neurobiological mechanisms, they are mostly theoretical in nature, and thus, further research comparing, for instance, traditional versus adapted exercise prescriptions with regard to neuroplasticity and cognition is urgently needed.
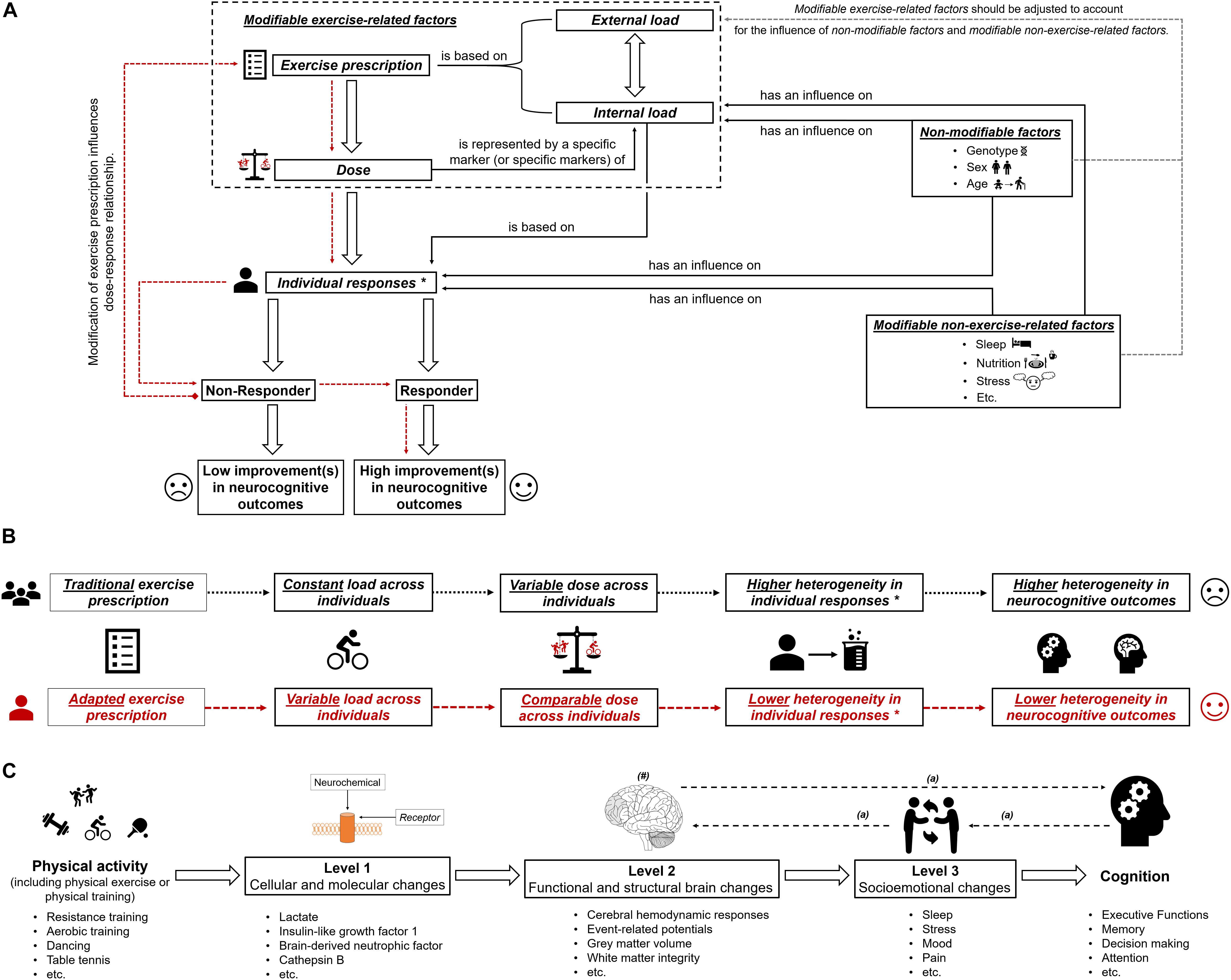
Figure 1. (A) Schematic illustration of the possible influence of exercise prescription on dose, and individual responsiveness (responder and non-responder) with the assumed extent of improvements (high improvements in neurocognitive outcomes and low improvements in neurocognitive outcomes). The dotted red lines show that by using an appropriate exercise prescription, non-responders could be turned into responders. In part (B) of the figure, the difference between “traditional exercise prescription” and “adapted exercise prescription” regarding the load, the dose, the individual response(s), and the corresponding heterogeneity in outcomes is illustrated. “∗” with regard to subsequent neurobiological processes. In part (C) of the figure, the multiple levels on which physical activity (including physical exercise and physical training) could affect cognitive performance are shown (Stillman et al., 2016). “#” indicates that the brain could be seen as outcome, mediator, or predictor (Stillman and Erickson, 2018). “a” indicates that there are several possibilities in which way structural and functional brain changes, socioemotional changes and cognitive changes are intertwined (Stillman et al., 2016).
Progress Is Not Without Limitations
Since the level and detail of description required to extensively describe and discuss the influence of all exercise variables, training variables, training principles, and factors influencing exercise–cognition interaction go far beyond the scope and intent of this article, our assumptions still remain imperfect because other exercise-related factors such as movement frequency (e.g., cycling cadence) (Ludyga et al., 2015, 2016) or psychological factors such as affective response (e.g., enjoyment or expectations) (Davidson et al., 2000; Davidson and McEwen, 2012; Burnet et al., 2018; Lindheimer et al., 2019) have not been considered. Nevertheless, given (i) that our knowledge of the dose–response relationship between acute physical exercises and/or physical training, neurobiological processes (e.g., neuroplasticity), and cognitive changes is still limited (Etnier et al., 2006, 2019; Hillman et al., 2008; Chang et al., 2012a, b; Barha et al., 2017b; Ströhlein et al., 2017; Tait et al., 2017; Pontifex et al., 2018; Stimpson et al., 2018; Erickson et al., 2019; Falck et al., 2019; Sanders et al., 2019), (ii) that peripheral blood lactate levels constitute an established marker of internal load (Hofmann and Tschakert, 2010; Beneke et al., 2011; Soligard et al., 2016; Impellizzeri et al., 2019), and (iii) that peripheral blood lactate levels are easily quantifiable by portable devices, the use of peripheral blood lactate as a proxy for dose seems a reasonable starting point. Nevertheless, lactate monitoring suffers from the drawbacks that (i) it necessitates blood sampling, which could be impractical in daily practice, and (ii) it requires a graded exercise test to calculate an individual threshold to prescribe the exercise intensity. Regarding the first objection, new methods to non-invasively determine critical physiological thresholds (e.g., lactate threshold) by means of muscle near-infrared spectroscopy (Wang et al., 2006; Xu et al., 2011; Bellotti et al., 2013; Borges and Driller, 2016; Driller et al., 2016) may constitute a more appropriate approach in daily practice, but this has yet to be investigated. With regard to the second objection, it is worth mentioning that graded exercise tests are relatively complex and time consuming and that exercise intensity could be more easily determined by using specific formulas (e.g., Karvonen formula to determine a target heart rate) (Karvonen and Vuorimaa, 1988; Tanaka et al., 2001; Gellish et al., 2007; Zhu et al., 2010; Nes et al., 2013; Shargal et al., 2015). However, a graded exercise test should be an integral part of the process of a proper exercise prescription because, currently, exercise intensity cannot be accurately predicted by specific formulas (Strzelczyk et al., 2001; Robergs and Landwehr, 2002; Silva et al., 2007; Sarzynski et al., 2013; Correa Mesa et al., 2015; Esco et al., 2015; Arena et al., 2016), and a fixed percentage of a maximally achievable value of heart rate leads to a considerable amount of interindividual heterogeneity in metabolic responses (e.g., blood lactate) (Meyer et al., 1999), which is deemed to contribute, at least partly, to the interindividual heterogeneity in neurocognitive outcomes (see previous sections).
Still, even if peripheral blood lactate concentrations are associated with serum BDNF concentrations (Ferris et al., 2007; Schiffer et al., 2011), further studies will be required to investigate the dose–response relationship between exercise prescription and (serum) BDNF levels (Knaepen et al., 2010; Coelho et al., 2013; Huang et al., 2014). Since BDNF release is also influenced by several other non-modifiable (e.g., sex Trajkovska et al., 2007; Komulainen et al., 2010; Bus et al., 2011) or non-exercise-related modifiable factors (e.g., sleep or nutrition; Giese et al., 2013, 2014; Walsh et al., 2015; Schmitt et al., 2016) that are known to influence neuroplasticity in general (e.g., sleep, Meerlo et al., 2009; Raven et al., 2018; or nutrition, Greenwood and Parasuraman, 2010; Phillips, 2017; Poulose et al., 2017), these factors should be carefully monitored in further studies.
In addition, with regard to the optimal dose, it could be useful to gather markers of internal load that are directly related to the state of the central nervous system itself (e.g., brain activity during exercise) because differences in brain activity (e.g., measured by functional near-infrared spectroscopy) (i) allow distinguishing between responders and non-responders (Yamazaki et al., 2017), (ii) are sensitive to changes of exercise variables (e.g., exercise intensity) (Rooks et al., 2010; Giles et al., 2014; Tempest et al., 2014; Santos-Concejero et al., 2015, 2017; Takehara et al., 2017), (iii) are sensitive to demands posed by the cognitive task (Herff et al., 2013; Fishburn et al., 2014; Causse et al., 2017; Khaksari et al., 2019) or the motor task (Carius et al., 2016), (iv) and are associated with performance improvements in motor(–cognitive) tasks (Ono et al., 2014, 2015; Herold et al., 2017; Seidel et al., 2017). Hence, markers of internal load assessing activation of the central nervous system may serve to quantify “complexity” (defined as neurocognitive demands posed by the exercise), which is an important variable with regard to neurocognitive changes in response to acute physical exercises and physical training, too (Netz, 2019). However, while measuring brain activation during exercise offers great potential to understand exercise–cognition interaction in general and interindividual variability in particular, future research in this area is strongly needed before measures of brain activity can be used to guide exercise prescription.
Furthermore, we wish to stress that a traditional individualization of exercise prescription is perhaps necessary to answer basic research questions (e.g., Are the peripheral blood lactate release and changes in neurocognition a function of exercise intensity?) but that the individualization using an adapted exercise prescription may lead to further insights into exercise–cognition research (e.g., How to adapt exercise intensity to achieve a comparable change in the release of peripheral blood lactate across individuals and how this affects neurocognition?).
Conclusion and Further Remarks
In essence, this article aimed at providing a suggestion for a clearer definition of the dose in exercise–cognition research and presenting evidence in how interindividual variability in the dose might contribute to the interindividual heterogeneity in neurocognitive outcomes. We propose that the dose of an acute bout of physical exercises and/or physical training should be operationalized by a specific marker (or specific markers) of internal load. Modifying the exercise prescription by carefully adjusting the external load, a comparable dose can be achieved across individuals (see Figures 1A,B). Research is strongly encouraged to investigate in the future whether an exercise prescription inducing a comparable dose may lower the interindividual heterogeneity considering outcome variables on different levels of analysis (Stillman et al., 2016) and on different aspects of the brain (Stillman and Erickson, 2018; see Figure 1C). Finally, understanding how a comparable dose affects neurocognitive outcomes is an important step toward identifying what dose is optimal for achieving the greatest benefits with regard to neurocognitive outcomes in an individual.
Author Contributions
FH wrote and edited the manuscript. PM, TG, and NM reviewed and edited the drafted versions.
Conflict of Interest
The authors declare that the research was conducted in the absence of any commercial or financial relationships that could be construed as a potential conflict of interest.
References
Abe, K. (2012). Total daily physical activity and the risk of AD and cognitive decline in older adults. Neurology 79:1071; author reply 1071. doi: 10.1212/WNL.0b013e31826bd5cf
Ahtiainen, J. P., Walker, S., Peltonen, H., Holviala, J., Sillanpää, E., Karavirta, L., et al. (2016). Heterogeneity in resistance training-induced muscle strength and mass responses in men and women of different ages. Age 38:10. doi: 10.1007/s11357-015-9870-1
Aichberger, M. C., Busch, M. A., Reischies, F. M., Ströhle, A., Heinz, A., and Rapp, M. A. (2010). Effect of Physical Inactivity on Cognitive Performance after 2.5 Years of Follow-Up. GeroPsych 23, 7–15. doi: 10.1024/1662-9647/a000003
Ainsworth, B. E., Haskell, W. L., Whitt, M. C., Irwin, M. L., Swartz, A. M., Strath, S. J., et al. (2000). Compendium of physical activities. An update of activity codes and MET intensities. Med. Sci. Sports Exerc. 32, S498–S504.
Albinet, C. T., Mandrick, K., Bernard, P. L., Perrey, S., and Blain, H. (2014). Improved cerebral oxygenation response and executive performance as a function of cardiorespiratory fitness in older women: a fNIRS study. Front. Aging Neurosci. 6:273185. doi: 10.3389/fnagi.2014.00272
Arena, R., Myers, J., and Kaminsky, L. A. (2016). Revisiting age-predicted maximal heart rate. Can it be used as a valid measure of effort? Am. Heart J. 173, 49–56. doi: 10.1016/j.ahj.2015.12.006
Atkinson, G., and Batterham, A. M. (2015). True and false interindividual differences in the physiological response to an intervention. Exp. Physiol. 100, 577–588. doi: 10.1113/EP085070
Atkinson, G., and Nevill, A. M. (1998). Statistical methods for assessing measurement error (reliability) in variables relevant to sports medicine. Sports Med. 26, 217–238.
Atkinson, G., Williamson, P., and Batterham, A. M. (2019). Issues in the determination of “responders” and “non-responders” in physiological research. Exp. Physiol. 104, 1215–1225. doi: 10.1113/EP087712
Audiffren, M., and André, N. (2019). The exercise-cognition relationship: a virtuous circle. J. Sport Health Sci. 8, 339–347 doi: 10.1016/j.jshs.2019.03.001
Bagger, M., Petersen, P. H., and Pedersen, P. K. (2003). Biological variation in variables associated with exercise training. Int. J. Sports Med. 24, 433–440. doi: 10.1055/s-2003-41180
Bamman, M. M., Cooper, D. M., Booth, F. W., Chin, E. R., Neufer, P. D., Trappe, S., et al. (2014). Exercise biology and medicine: innovative research to improve global health. Mayo Clin. Proc. 89, 148–153. doi: 10.1016/j.mayocp.2013.11.013
Barha, C. K., Davis, J. C., Falck, R. S., Nagamatsu, L. S., and Liu-Ambrose, T. (2017a). Sex differences in exercise efficacy to improve cognition. A systematic review and meta-analysis of randomized controlled trials in older humans. Front. Neuroendocrinol. 46, 71–85. doi: 10.1016/j.yfrne.2017.04.002
Barha, C. K., Galea, L. A., Nagamatsu, L. S., Erickson, K. I., and Liu-Ambrose, T. (2017b). Personalising exercise recommendations for brain health. Considerations and future directions. Br. J. Sports Med. 51, 636–639. doi: 10.1136/bjsports-2016-096710
Barha, C. K., and Liu-Ambrose, T. (2018). Exercise and the aging brain: considerations for sex differences. BPL 3, 1–11. doi: 10.3233/BPL-1867
Barha, C. K., Liu-Ambrose, T., Best, J. R., Yaffe, K., and Rosano, C. (2019). Sex-dependent effect of the BDNF Val66Met polymorphism on executive functioning and processing speed in older adults: evidence from the health ABC study. Neurobiol. Aging 74, 161–170. doi: 10.1016/j.neurobiolaging.2018.10.021
Basso, J. C., and Suzuki, W. A. (2017). The effects of acute exercise on mood, cognition, neurophysiology, and neurochemical pathways. a review. BPL 2, 127–152. doi: 10.3233/BPL-160040
Bellotti, C., Calabria, E., Capelli, C., and Pogliaghi, S. (2013). Determination of maximal lactate steady state in healthy adults: can NIRS help? Med. Sci. Sports Exerc. 45, 1208–1216. doi: 10.1249/MSS.0b013e3182828ab2
Beneke, R., Leithäuser, R. M., and Ochental, O. (2011). Blood lactate diagnostics in exercise testing and training. Int. J. Sports Physiol. Perform. 6, 8–24.
Bherer, L., Erickson, K. I., and Liu-Ambrose, T. (2013). A review of the effects of physical activity and exercise on cognitive and brain functions in older adults. J. Aging Res. 2013:657508. doi: 10.1155/2013/657508
Bherer, L., Langeard, A., Kaushal, N., Vrinceanu, T., Desjardins-Crépeau, L., Langlois, F., et al. (2019). Physical exercise training effect and mediation through cardiorespiratory fitness on dual-task performances differ in younger-old and older-old adults. J. Gerontol. Ser. B Psychol. Sci. Soc. Sci. doi: 10.1093/geronb/gbz066 [Epub ahead of print].
Blair, S. N. (2009). Physical inactivity: the biggest public health problem of the 21st century. Br. J. Sports Med. 43, 1–2.
Blokland, G. A. M., McMahon, K. L., Hoffman, J., Zhu, G., Meredith, M., Martin, N. G., et al. (2008). Quantifying the heritability of task-related brain activation and performance during the N-back working memory task: a twin fMRI study. Biol. Psychol. 79, 70–79. doi: 10.1016/j.biopsycho.2008.03.006
Bonafiglia, J. T., Brennan, A. M., Ross, R., and Gurd, B. J. (2019a). An appraisal of the SDIR as an estimate of true individual differences in training responsiveness in parallel-arm exercise randomized controlled trials. Physiol. Rep. 7:e14163. doi: 10.14814/phy2.14163
Bonafiglia, J. T., Ross, R., and Gurd, B. J. (2019b). The application of repeated testing and monoexponential regressions to classify individual cardiorespiratory fitness responses to exercise training. Eur. J. Appl. Physiol. 119, 889–900. doi: 10.1007/s00421-019-04078-w
Bonafiglia, J. T., Nelms, M. W., Preobrazenski, N., LeBlanc, C., Robins, L., Lu, S., et al. (2018). Moving beyond threshold-based dichotomous classification to improve the accuracy in classifying non-responders. Physiol. Rep. 6:e13928. doi: 10.14814/phy2.13928
Bonafiglia, J. T., Rotundo, M. P., Whittall, J. P., Scribbans, T. D., Graham, R. B., and Gurd, B. J. (2016). Inter-individual variability in the adaptive responses to endurance and sprint interval training: a randomized crossover study. PLoS One 11:e0167790. doi: 10.1371/journal.pone.0167790
Booth, F. W., and Laye, M. J. (2010). The future: genes, physical activity and health. Acta Physiol. 199, 549–556. doi: 10.1111/j.1748-1716.2010.02117.x
Borges, N. R., and Driller, M. W. (2016). Wearable lactate threshold predicting device is valid and reliable in runners. J. Streng. Condit. Res. 30, 2212–2218. doi: 10.1519/JSC.0000000000001307
Bouchard, C. (2012). Genomic predictors of trainability. Exp. Physiol. 97, 347–352. doi: 10.1113/expphysiol.2011.058735
Bouchard, C. (2019). DNA sequence variations contribute to variability in fitness and trainability. Med. Sci. Sports Exerc. 51, 1781–1785. doi: 10.1249/MSS.0000000000001976
Bouchard, C., An, P., Rice, T., Skinner, J. S., Wilmore, J. H., Gagnon, J., et al. (1999). Familial aggregation of VO(2max) response to exercise training: results from the HERITAGE family study. J. Appl. Physiol. 87, 1003–1008. doi: 10.1152/jappl.1999.87.3.1003
Bouchard, C., Antunes-Correa, L. M., Ashley, E. A., Franklin, N., Hwang, P. M., Mattsson, C. M., et al. (2015). Personalized preventive medicine: genetics and the response to regular exercise in preventive interventions. Prog. Cardiovasc. Dis. 57, 337–346. doi: 10.1016/j.pcad.2014.08.005
Bouchard, C., Blair, S. N., Church, T. S., Earnest, C. P., Hagberg, J. M., Häkkinen, K., et al. (2012). Adverse metabolic response to regular exercise: is it a rare or common occurrence? PLoS One 7:e37887. doi: 10.1371/journal.pone.0037887
Bouchard, C., and Rankinen, T. (2001). Individual differences in response to regular physical activity. Med. Sci. Sports Exerc. 33, S446–S451. doi: 10.1097/00005768-200106001-00013
Bourdon, P. C., Cardinale, M., Murray, A., Gastin, P., Kellmann, M., Varley, M. C., et al. (2017). Monitoring athlete training loads. consensus statement. Int. J. Sports Physiol. Perform. 12, S2-161–S2-170. doi: 10.1123/IJSPP.2017-0208
Bowen, M. E. (2012). A prospective examination of the relationship between physical activity and dementia risk in later life. Am. J. Health Promot. 26, 333–340. doi: 10.4278/ajhp.110311-QUAN-115
Brigadski, T., and Leßmann, V. (2014). BDNF. A regulator of learning and memory processes with clinical potential. eNeuroforum 5, 1–11. doi: 10.1007/s13295-014-0053-9
Brooks, G. A. (2018). The Science and Translation of Lactate Shuttle Theory. Cell Metab. 27, 757–785. doi: 10.1016/j.cmet.2018.03.008
Brown, B. M., Castalanelli, N., Rainey-Smith, S. R., Doecke, J., Weinborn, M., Sohrabi, H. R., et al. (2019). Influence of BDNF Val66Met on the relationship between cardiorespiratory fitness and memory in cognitively normal older adults. Behav. Brain Res. 362, 103–108 doi: 10.1016/j.bbr.2019.01.013
Budde, H., Schwarz, R., Velasques, B., Ribeiro, P., Holzweg, M., Machado, S., et al. (2016). The need for differentiating between exercise, physical activity, and training. Autoimmun. Rev. 15, 110–111. doi: 10.1016/j.autrev.2015.09.004
Bueller, J. A., Aftab, M., Sen, S., Gomez-Hassan, D., Burmeister, M., and Zubieta, J.-K. (2006). BDNF Val66Met allele is associated with reduced hippocampal volume in healthy subjects. Biol. Psychiatry 59, 812–815. doi: 10.1016/j.biopsych.2005.09.022
Buford, T. W., and Pahor, M. (2012). Making preventive medicine more personalized. Implications for exercise-related research. Preven. Med. 55, 34–36. doi: 10.1016/j.ypmed.2012.05.001
Buford, T. W., Roberts, M. D., and Church, T. S. (2013). Toward exercise as personalized medicine. Sports Med. 43, 157–165. doi: 10.1007/s40279-013-0018-0
Bugg, J. M., Shah, K., Villareal, D. T., and Head, D. (2012). Cognitive and neural correlates of aerobic fitness in obese older adults. Exp. Aging Res. 38, 131–145. doi: 10.1080/0361073X.2012.659995
Burgess, D. J. (2017). The Research Doesn’t Always Apply. practical solutions to evidence-based training-load monitoring in elite team sports. Int. J. Sports Physiol. Perform. 12, S2136–S2141. doi: 10.1123/ijspp.2016-0608
Burley, S. D., Drain, J. R., Sampson, J. A., and Groeller, H. (2018). Positive, limited and negative responders: the variability in physical fitness adaptation to basic military training. J. Sci. Med. Sport 21, 1168–1172. doi: 10.1016/j.jsams.2018.06.018
Burnet, K., Kelsch, E., Zieff, G., Moore, J. B., and Stoner, L. (2018). How fitting is F.I.T.T.? A perspective on a transition from the sole use of frequency, intensity, time, and type in exercise prescription. Physiol. Behav. 199, 33–34. doi: 10.1016/j.physbeh.2018.11.007
Burns, J. M., Cronk, B. B., Anderson, H. S., Donnelly, J. E., Thomas, G. P., Harsha, A., et al. (2008). Cardiorespiratory fitness and brain atrophy in early Alzheimer disease. Neurology 71, 210–216. doi: 10.1212/01.wnl.0000317094.86209.cb
Bus, B. A. A., Molendijk, M. L., Penninx, B. J. W. H., Buitelaar, J. K., Kenis, G., Prickaerts, J., et al. (2011). Determinants of serum brain-derived neurotrophic factor. Psychoneuroendocrinology 36, 228–239. doi: 10.1016/j.psyneuen.2010.07.013
Cabral, D. F., Rice, J., Morris, T. P., Rundek, T., Pascual-Leone, A., and Gomes-Osman, J. (2019). Exercise for Brain Health: An Investigation into the Underlying Mechanisms Guided by Dose. Neurotherapeutics 16, 580–599. doi: 10.1007/s13311-019-00749-w
Campbell, K. L., Neil, S. E., and Winters-Stone, K. M. (2012). Review of exercise studies in breast cancer survivors: attention to principles of exercise training. Br. J. Sports Med. 46, 909–916. doi: 10.1136/bjsports-2010-082719
Canivet, A., Albinet, C. T., André, N., Pylouster, J., Rodríguez-Ballesteros, M., Kitzis, A., et al. (2015). Effects of BDNF polymorphism and physical activity on episodic memory in the elderly. A cross sectional study. Eur. Rev. Aging Phys. Act. 12:15. doi: 10.1186/s11556-015-0159-2
Canivet, A., Albinet, C. T., Rodríguez-Ballesteros, M., Chicherio, C., Fagot, D., André, N., et al. (2017). Interaction between BDNF Polymorphism and Physical Activity on Inhibitory Performance in the Elderly without Cognitive Impairment. Front. Hum. Neurosci. 11:4217. doi: 10.3389/fnhum.2017.00541
Carius, D., Andrä, C., Clauß, M., Ragert, P., Bunk, M., and Mehnert, J. (2016). Hemodynamic Response Alteration As a Function of Task Complexity and Expertise—An fNIRS Study in Jugglers. Front. Hum. Neurosci. 10:126. doi: 10.3389/fnhum.2016.00126
Carlsten, C., and Burke, W. (2006). Potential for genetics to promote public health: genetics research on smoking suggests caution about expectations. J. Am. Med. Assoc. 296, 2480–2482. doi: 10.1001/jama.296.20.2480
Caspersen, C. J., Powell, K. E., and Christenson, G. M. (1985). Physical activity, exercise, and physical fitness. Definitions and distinctions for health-related research. Publ. Health Rep. 100, 126–131.
Castalanelli, N., Weinborn, M., Gignac, G. E., Markovic, S., Rainey-Smith, S. R., Sohrabi, H. R., et al. (2019). Higher cardiorespiratory fitness is associated with better verbal generativity in community dwelling older adults. J. Aging Phys. Activ. doi: 10.1123/japa.2018-0382 [Epub ahead of print].
Causse, M., Chua, Z., Peysakhovich, V., Del Campo, N., and Matton, N. (2017). Mental workload and neural efficiency quantified in the prefrontal cortex using fNIRS. Sci. Rep. 7:5222. doi: 10.1038/s41598-017-05378-x
Chang, Y. K., Labban, J. D., Gapin, J. I., and Etnier, J. L. (2012a). The effects of acute exercise on cognitive performance: a meta-analysis. Brain Res. 1453, 87–101. doi: 10.1016/j.brainres.2012.02.068
Chang, Y.-K., Pan, C.-Y., Chen, F.-T., Tsai, C.-L., and Huang, C.-C. (2012b). Effect of resistance-exercise training on cognitive function in healthy older adults. A review. J. Aging Phys. Activ. 20, 497–517. doi: 10.1123/japa.20.4.497
Chmelo, E. A., Crotts, C. I., Newman, J. C., Brinkley, T. E., Lyles, M. F., Leng, X., et al. (2015). Heterogeneity of physical function responses to exercise training in older adults. J. Am. Geriatr. Soc. 63, 462–469. doi: 10.1111/jgs.13322
Chrzanowski-Smith, O. J., Piatrikova, E., Betts, J. A., Williams, S., and Gonzalez, J. T. (2019). Variability in exercise physiology: can capturing intra-individual variation help better understand true inter-individual responses? Eur. J. Sport Sci. doi: 10.1080/17461391.2019.1655100 [Epub ahead of print].
Church, T. S., Thomas, D. M., Tudor-Locke, C., Katzmarzyk, P. T., Earnest, C. P., Rodarte, R. Q., et al. (2011). Trends over 5 decades in U.S. occupation-related physical activity and their associations with obesity. PLoS One 6:e19657. doi: 10.1371/journal.pone.0019657
Churchward-Venne, T. A., Tieland, M., Verdijk, L. B., Leenders, M., Dirks, M. L., de Groot, L. C. P., et al. (2015). There are no nonresponders to resistance-type exercise training in older men and women. J. Am. Med. Direct. Assoc. 16, 400–411. doi: 10.1016/j.jamda.2015.01.071
Cobbold, C. (2018). Battle of the sexes: which is better for you, high- or low-intensity exercise? J. Sport Health Sci. 7, 429–432. doi: 10.1016/j.jshs.2018.05.004
Coelho, F. G., Gobbi, S., Andreatto, C. A., Corazza, D. I., Pedroso, R. V., and Santos-Galduroz, R. F. (2013). Physical exercise modulates peripheral levels of brain-derived neurotrophic factor (BDNF): a systematic review of experimental studies in the elderly. Arch. Gerontol. Geriatr. 56, 10–15. doi: 10.1016/j.archger.2012.06.003
Coggan, A. R., and Costill, D. L. (1984). Biological and technological variability of three anaerobic ergometer tests. Int. J. Sports Med. 5, 142–145. doi: 10.1055/s-2008-1025896
Colcombe, S. J., Erickson, K. I., Raz, N., Webb, A. G., Cohen, N. J., McAuley, E., et al. (2003). Aerobic fitness reduces brain tissue loss in aging humans. J. Gerontol. Ser. A Biol. Sci. Med. Sci. 58, 176–180.
Colcombe, S. J., Erickson, K. I., Scalf, P. E., Kim, J. S., Prakash, R., McAuley, E., et al. (2006). Aerobic exercise training increases brain volume in aging humans. J. Gerontol. Ser. A Biol. Sci. Med. Sci. 61, 1166–1170.
Colcombe, S. J., Kramer, A. F., Erickson, K. I., Scalf, P., McAuley, E., Cohen, N. J., et al. (2004). Cardiovascular fitness, cortical plasticity, and aging. Proc. Natl. Acad. Sci. U.S.A. 101, 3316–3321. doi: 10.1073/pnas.0400266101
Colzato, L. S., Jongkees, B. J., Wit, M., de, van der Molen, M. J. W., and Steenbergen, L. (2018). Variable heart rate and a flexible mind. Higher resting-state heart rate variability predicts better task-switching. Cogn. Affect. Behav. Neurosci. 18, 730–738. doi: 10.3758/s13415-018-0600-x
Copeland, J. L., Clarke, J., and Dogra, S. (2015). Objectively measured and self-reported sedentary time in older Canadians. Prev. Med. Rep. 2, 90–95. doi: 10.1016/j.pmedr.2015.01.003
Correa Mesa, J. F., Cruz Martinez, L. E., Correa Morales, J. C., and Rojas Valencia, J. T. (2015). Maximum heart rate during exercise. reliability of the 220-age and Tanaka formulas in healthy young people at a moderate altitude. Rev. Fac. Med. 62, 579–585. doi: 10.15446/revfacmed.v62n4.44539
Cotman, C. W., Berchtold, N. C., and Christie, L.-A. (2007). Exercise builds brain health: key roles of growth factor cascades and inflammation. Trends Neurosci. 30, 464–472. doi: 10.1016/j.tins.2007.06.011
Dankel, S. J., and Loenneke, J. P. (2019). A method to stop analyzing random error and start analyzing differential responders to exercise. Sports Med. doi: 10.1007/s40279-019-01147-0 [Epub ahead of print].
Dao, E., Barha, C. K., Best, J. R., Hsiung, G.-Y., Tam, R., and Liu-Ambrose, T. (2019). The effect of aerobic exercise on white matter hyperintensity progression may vary by sex. Can. J. Aging 38, 236–244. doi: 10.1017/S0714980818000582
Davenport, M. H., Hogan, D. B., Eskes, G. A., Longman, R. S., and Poulin, M. J. (2012). Cerebrovascular reserve: the link between fitness and cognitive function? Exerc. Sport Sci. Rev. 40, 153–158. doi: 10.1097/JES.0b013e3182553430
Davidsen, P. K., Gallagher, I. J., Hartman, J. W., Tarnopolsky, M. A., Dela, F., Helge, J. W., et al. (2011). High responders to resistance exercise training demonstrate differential regulation of skeletal muscle microRNA expression. J. Appl. Physiol. 110, 309–317. doi: 10.1152/japplphysiol.00901.2010
Davidson, R. J., Jackson, D. C., and Kalin, N. H. (2000). Emotion, plasticity, context, and regulation: perspectives from affective neuroscience. Psychol. Bull. 126, 890–909. doi: 10.1037//0033-2909.126.6.890
Davidson, R. J., and McEwen, B. S. (2012). Social influences on neuroplasticity: stress and interventions to promote well-being. Nat. Neurosci. 15, 689–695. doi: 10.1038/nn.3093
Day, J. R., Rossiter, H. B., Coats, E. M., Skasick, A., and Whipp, B. J. (2003). The maximally attainable VO2 during exercise in humans. The peak vs. maximum issue. J. Appl. Physiol. 95, 1901–1907. doi: 10.1152/japplphysiol.00024.2003
de Bruijn, R. F., Schrijvers, E. M. C., de Groot, K. A., Witteman, J. C. M., Hofman, A., Franco, O. H., et al. (2013). The association between physical activity and dementia in an elderly population: the rotterdam study. Eur. J. Epidemiol. 28, 277–283. doi: 10.1007/s10654-013-9773-3
Del Coso, J., Hiam, D., Houweling, P., Pérez, L. M., Eynon, N., and Lucía, A. (2018). More than a ‘speed gene’: ACTN3 R577X genotype, trainability, muscle damage, and the risk for injuries. Eur. J. Appl. Physiol. 119, 49–60. doi: 10.1007/s00421-018-4010-0
Dennis, A., Thomas, A. G., Rawlings, N. B., Near, J., Nichols, T. E., Clare, S., et al. (2015). An ultra-high field magnetic resonance spectroscopy study of post exercise lactate, glutamate and glutamine change in the human brain. Front. Physiol. 6:351. doi: 10.3389/fphys.2015.00351
Dinoff, A., Herrmann, N., Swardfager, W., and Lanctôt, K. L. (2017). The effect of acute exercise on blood concentrations of brain-derived neurotrophic factor in healthy adults. A meta-analysis. Eur. J. Neurosci. 46, 1635–1646. doi: 10.1111/ejn.13603
Driller, M., Borges, N., and Plews, D. (2016). Evaluating a new wearable lactate threshold sensor in recreational to highly trained cyclists. Sports Eng. 19, 229–235. doi: 10.1007/s12283-016-0198-6
Dupuy, O., Gauthier, C. J., Fraser, S. A., Desjardins-Crepeau, L., Desjardins, M., Mekary, S., et al. (2015). Higher levels of cardiovascular fitness are associated with better executive function and prefrontal oxygenation in younger and older women. Front. Hum. Neurosci. 9:66. doi: 10.3389/fnhum.2015.00066
Erickson, K. I., Gildengers, A. G., and Butters, M. A. (2013). Physical activity and brain plasticity in late adulthood. Dialog. Clin. Neurosci. 15, 99–108.
Erickson, K. I., Hillman, C., Stillman, C. M., Ballard, R. M., Bloodgood, B., Conroy, D. E., et al. (2019). Physical activity, cognition, and brain outcomes: a review of the 2018 physical activity guidelines. Med. Sci. Sports Exerc. 51, 1242–1251. doi: 10.1249/MSS.0000000000001936
Erickson, K. I., Hillman, C. H., and Kramer, A. F. (2015). Physical activity, brain, and cognition. Curr. Opin. Behav. Sci. 4, 27–32. doi: 10.1016/j.cobeha.2015.01.005
Erickson, K. I., Kim, J. S., Suever, B. L., Voss, M. W., Francis, B. M., and Kramer, A. F. (2008). Genetic contributions to age-related decline in executive function: a 10-year longitudinal study of COMT and BDNF polymorphisms. Front. Hum. Neurosci. 2:11. doi: 10.3389/neuro.09.011.2008
Erickson, K. I., Oberlin, L., Gujral, S., Leckie, R. L., Weinstein, A. M., Hodzic, J. C., et al. (2014). Exercise as a way of capitalizing on neuroplasticity in late adulthood. Top. Geriatr. Rehabil. 30, 8–14. doi: 10.1097/TGR.0000000000000008
Erickson, K. I., Prakash, R. S., Voss, M. W., Chaddock, L., Heo, S., McLaren, M., et al. (2010). Brain-derived neurotrophic factor is associated with age-related decline in hippocampal volume. J. Neurosci. 30, 5368–5375. doi: 10.1523/JNEUROSCI.6251-09.2010
Erickson, K. I., Prakash, R. S., Voss, M. W., Chaddock, L., Hu, L., Morris, K. S., et al. (2009). Aerobic fitness is associated with hippocampal volume in elderly humans. Hippocampus 19, 1030–1039. doi: 10.1002/hipo.20547
Erickson, K. I., Voss, M. W., Prakash, R. S., Basak, C., Szabo, A., Chaddock, L., et al. (2011). Exercise training increases size of hippocampus and improves memory. Proc. Natl. Acad. Sci. U.S.A. 108, 3017–3022. doi: 10.1073/pnas.1015950108
Esco, M. R., Chamberlain, N., Flatt, A. A., Snarr, R. L., Bishop, P. A., and Williford, H. N. (2015). Cross-validation of age-predicted maximal heart rate equations among female collegiate athletes. J. Strength Cond. Res. 29, 3053–3059. doi: 10.1519/JSC.0000000000000978
Etnier, J. L., Drollette, E. S., and Slutsky, A. B. (2019). Physical activity and cognition: a narrative review of the evidence for older adults. Psychol. Sport Exerc. 42, 156–166. doi: 10.1016/j.psychsport.2018.12.006
Etnier, J. L., Nowell, P. M., Landers, D. M., and Sibley, B. A. (2006). A meta-regression to examine the relationship between aerobic fitness and cognitive performance. Brain Res. Rev. 52, 119–130. doi: 10.1016/j.brainresrev.2006.01.002
Falck, R. S., Davis, J. C., Best, J. R., Crockett, R. A., and Liu-Ambrose, T. (2019). Impact of exercise training on physical and cognitive function among older adults: a systematic review and meta-analysis. Neurobiol. Aging 79, 119–130. doi: 10.1016/j.neurobiolaging.2019.03.007
Falck, R. S., Davis, J. C., and Liu-Ambrose, T. (2016). What is the association between sedentary behaviour and cognitive function? A systematic review. Br. J. Sports Med. 51, 800–811. doi: 10.1136/bjsports-2015-095551
Faude, O., Hecksteden, A., Hammes, D., Schumacher, F., Besenius, E., Sperlich, B., et al. (2017). Reliability of time-to-exhaustion and selected psycho-physiological variables during constant-load cycling at the maximal lactate steady-state. Appl. Physiol. Nutr. Metab. 42, 142–147. doi: 10.1139/apnm-2016-0375
Ferris, L. T., Williams, J. S., and Shen, C. -L. (2007). The effect of acute exercise on serum brain-derived neurotrophic factor levels and cognitive function. Med. Sci. Sports Exerc. 39, 728–734. doi: 10.1249/mss.0b013e31802f04c7
Fishburn, F. A., Norr, M. E., Medvedev, A. V., and Vaidya, C. J. (2014). Sensitivity of fNIRS to cognitive state and load. Front. Hum. Neurosci. 8:76. doi: 10.3389/fnhum.2014.00076
Flück, M. (2018). Optimierung der muskuloskelettalen rehabilitation durch einbezug individueller faktoren der zellulären plastizität. Dtsch Z Sportmed. 2018, 283–284. doi: 10.5960/dzsm.2018.345
Fortune, J. M., Kelly, Á. M., Robertson, I. H., and Hussey, J. (2019). An investigation into the relationship between cardiorespiratory fitness, cognition and BDNF in young healthy males. Neurosci. Lett 704, 126–132. doi: 10.1016/j.neulet.2019.03.012
Freudenberger, P., Petrovic, K., Sen, A., Töglhofer, A. M., Fixa, A., Hofer, E., et al. (2016). Fitness and cognition in the elderly. The Austrian stroke prevention study. Neurology 86, 418–424. doi: 10.1212/WNL.0000000000002329
Frewen, J., Finucane, C., Savva, G. M., Boyle, G., Coen, R. F., and Kenny, R. A. (2013). Cognitive function is associated with impaired heart rate variability in ageing adults: the Irish longitudinal study on ageing wave one results. Clin. Auton. Res. 23, 313–323. doi: 10.1007/s10286-013-0214-x
Friedman, N. P., Miyake, A., Young, S. E., DeFries, J. C., Corley, R. P., and Hewitt, J. K. (2008). Individual differences in executive functions are almost entirely genetic in origin. J. Exp. Psychol. Gen. 137, 201–225. doi: 10.1037/0096-3445.137.2.201
Gallen, C. L., and D’Esposito, M. (2019). Brain modularity: a biomarker of intervention-related plasticity. Trends Cogn. Sci. 23, 293–304. doi: 10.1016/j.tics.2019.01.014
Garber, C. E., Blissmer, B., Deschenes, M. R., Franklin, B. A., Lamonte, M. J., Lee, I.-M., et al. (2011). American College of Sports Medicine position stand. Quantity and quality of exercise for developing and maintaining cardiorespiratory, musculoskeletal, and neuromotor fitness in apparently healthy adults: guidance for prescribing exercise. Med. Sci. Sports Exerc. 43, 1334–1359. doi: 10.1249/MSS.0b013e318213fefb
Gass, G. C., McLellan, T. M., and Gass, E. M. (1991). Effects of prolonged exercise at a similar percentage of maximal oxygen consumption in trained and untrained subjects. Eur. J. Appl. Physiol. 63, 430–435. doi: 10.1007/BF00868074
Gellish, R. L., Goslin, B. R., Olson, R. E., McDonald, A., Russi, G. D., and Moudgil, V. K. (2007). Longitudinal modeling of the relationship between age and maximal heart rate. Med. Sci. Sports Exerc. 39, 822–829. doi: 10.1097/mss.0b013e31803349c6
Giese, M., Unternaehrer, E., Brand, S., Calabrese, P., Holsboer-Trachsler, E., and Eckert, A. (2013). The interplay of stress and sleep impacts BDNF level. PLoS One 8:e76050. doi: 10.1371/journal.pone.0076050
Giese, M., Unternährer, E., Hüttig, H., Beck, J., Brand, S., Calabrese, P., et al. (2014). BDNF: an indicator of insomnia? Mol. Psychiatry 19, 151–152. doi: 10.1038/mp.2013.10
Giles, G. E., Brunye, T. T., Eddy, M. D., Mahoney, C. R., Gagnon, S. A., Taylor, H. A., et al. (2014). Acute exercise increases oxygenated and deoxygenated hemoglobin in the prefrontal cortex. NeuroReport 25, 1320–1325. doi: 10.1097/WNR.0000000000000266
Gillie, B. L., Vasey, M. W., and Thayer, J. F. (2014). Heart rate variability predicts control over memory retrieval. Psychol. Sci. 25, 458–465. doi: 10.1177/0956797613508789
Goldberg, T. E., and Weinberger, D. R. (2004). Genes and the parsing of cognitive processes. Trends Cogn. Sci. 8, 325–335. doi: 10.1016/j.tics.2004.05.011
Grande, G., Vanacore, N., Maggiore, L., Cucumo, V., Ghiretti, R., Galimberti, D., et al. (2014). Physical activity reduces the risk of dementia in mild cognitive impairment subjects. A cohort study. J. Alzheimers Dis. 39, 833–839. doi: 10.3233/JAD-131808
Greenham, G., Buckley, J. D., Garrett, J., Eston, R., and Norton, K. (2018). Biomarkers of physiological responses to periods of intensified, non-resistance-based exercise training in well-trained male athletes: a systematic review and meta-analysis. Sports Med. 48, 2517–2548. doi: 10.1007/s40279-018-0969-2
Greenwood, P. M., and Parasuraman, R. (2010). Neuronal and cognitive plasticity. A neurocognitive framework for ameliorating cognitive aging. Front. Aging Neurosci. 2:150. doi: 10.3389/fnagi.2010.00150
Gronwald, T., Alves, A. C. D. B., Murillo-Rodríguez, E., Latini, A., Schuette, J., and Budde, H. (2019a). Standardization of exercise intensity and consideration of a dose-response is essential. Commentary on “Exercise-linked FNDC5/irisin rescues synaptic plasticity and memory defects in Alzheimer’s models”, by Lourenco et al., published 2019 in nature medicine. J. Sport Health Sci. 8, 353–354. doi: 10.1016/j.jshs.2019.03.006
Gronwald, T., Hoos, O., and Hottenrott, K. (2019b). Effects of a short-term cycling interval session and active recovery on non-linear dynamics of cardiac autonomic activity in endurance trained cyclists. JCM 8:194. doi: 10.3390/jcm8020194
Gronwald, T., Hoos, O., and Hottenrott, K. (2019c). Effects of acute normobaric hypoxia on non-linear dynamics of cardiac autonomic activity during constant workload cycling exercise. Front. Physiol. 10:865. doi: 10.3389/fphys.2019.00999
Gronwald, T., Hoos, O., Ludyga, S., and Hottenrott, K. (2018a). Non-linear dynamics of heart rate variability during incremental cycling exercise. Res. Sports Med. 59, 1–11. doi: 10.1080/15438627.2018.1502182
Gronwald, T., Velasques, B., Ribeiro, P., Machado, S., Murillo-Rodríguez, E., Ludyga, S., et al. (2018b). Increasing exercise’s effect on mental health: exercise intensity does matter. Proc. Natl. Acad. Sci. U.S.A. 115, E11890–E11891. doi: 10.1073/pnas.1818161115
Gronwald, T., Schulze, S., Ludyga, S., and Hottenrott, K. (2016). Evaluierung individueller Trainingsvorgaben auf Basis der Herzfrequenzvariabilität für ein Lauftraining im Freizeit- und Gesundheitssport. Praxis 105, 1065–1070. doi: 10.1024/1661-8157/a002458
Halson, S. L. (2014). Monitoring training load to understand fatigue in athletes. Sports Med. 44(Suppl. 2), S139–S147. doi: 10.1007/s40279-014-0253-z
Hamer, M., and Chida, Y. (2009). Physical activity and risk of neurodegenerative disease: a systematic review of prospective evidence. Psychol. Med. 39, 3–11. doi: 10.1017/S0033291708003681
Hansen, A. L., Johnsen, B. H., and Thayer, J. F. (2003). Vagal influence on working memory and attention. Int. J. Psychophysiol. 48, 263–274. doi: 10.1016/S0167-8760(03)00073-4
Hashimoto, T., Tsukamoto, H., Takenaka, S., Olesen, N. D., Petersen, L. G., Sørensen, H., et al. (2017). Maintained exercise-enhanced brain executive function related to cerebral lactate metabolism in men. FASEB J. 32, 1417–1427. doi: 10.1096/fj.201700381RR
Hayes, S. M., Forman, D. E., and Verfaellie, M. (2016). Cardiorespiratory fitness is associated with cognitive performance in older but not younger adults. J. Gerontol. Ser. B Psychol. Sci. Soc. Sci. 71, 474–482. doi: 10.1093/geronb/gbu167
Hecksteden, A., Kraushaar, J., Scharhag-Rosenberger, F., Theisen, D., Senn, S., and Meyer, T. (2015). Individual response to exercise training - a statistical perspective. J. Appl. Physiol. 118, 1450–1459. doi: 10.1152/japplphysiol.00714.2014
Hecksteden, A., Pitsch, W., Rosenberger, F., and Meyer, T. (2018). Repeated testing for the assessment of individual response to exercise training. J. Appl. Physiol. 124, 1567–1579. doi: 10.1152/japplphysiol.00896.2017
Heisz, J. J., Clark, I. B., Bonin, K., Paolucci, E. M., Michalski, B., Becker, S., et al. (2017). The effects of physical exercise and cognitive training on memory and neurotrophic factors. J. Cogn. Neurosci. 29, 1895–1907. doi: 10.1162/jocn_a_01164
Herff, C., Heger, D., Fortmann, O., Hennrich, J., Putze, F., and Schultz, T. (2013). Mental workload during n-back task-quantified in the prefrontal cortex using fNIRS. Front. Hum. Neurosci. 7:935. doi: 10.3389/fnhum.2013.00935
Herold, F., Hamacher, D., Schega, L., and Müller, N. G. (2018a). Thinking while moving or moving while thinking – concepts of motor-cognitive training for cognitive performance enhancement. Front. Aging Neurosci. 10, 1–11. doi: 10.3389/fnagi.2018.00228
Herold, F., Wiegel, P., Scholkmann, F., and Müller, N. G. (2018b). Applications of functional near-infrared spectroscopy (fnirs) neuroimaging in exercise?cognition science: a systematic, methodology-focused review. J. Clin. Med. 7:466. doi: 10.3390/jcm7120466
Herold, F., Orlowski, K., Börmel, S., and Müller, N. G. (2017). Cortical activation during balancing on a balance board. Hum. Move. Sci. 51, 51–58. doi: 10.1016/j.humov.2016.11.002
Herold, F., Törpel, A., Schega, L., and Müller, N. G. (2019). Functional and/or structural brain changes in response to resistance exercises and resistance training lead to cognitive improvements – a systematic review. Eur. Rev. Aging Phys. Act. 16:10. doi: 10.1186/s11556-019-0217-2
Hillman, C. H., Erickson, K. I., and Kramer, A. F. (2008). Be smart, exercise your heart. Exercise effects on brain and cognition. Nat. Rev. Neurosci. 9, 58–65. doi: 10.1038/nrn2298
Hofmann, P., and Tschakert, G. (2010). Special needs to prescribe exercise intensity for scientific studies. Cardiol. Res. Pract. 2011:209302. doi: 10.4061/2011/209302
Honea, R. A., Thomas, G. P., Harsha, A., Anderson, H. S., Donnelly, J. E., Brooks, W. M., et al. (2009). Cardiorespiratory fitness and preserved medial temporal lobe volume in Alzheimer disease. Alzheimer Dis. Assoc. Disord. 23, 188–197. doi: 10.1097/WAD.0b013e31819cb8a2
Horwitz, R. I., Cullen, M. R., Abell, J., and Christian, J. B. (2013). Medicine. (De)personalized medicine. Science 339, 1155–1156. doi: 10.1126/science.1234106
Hötting, K., and Röder, B. (2013). Beneficial effects of physical exercise on neuroplasticity and cognition. Neurosci. Biobehav. Rev. 37, 2243–2257. doi: 10.1016/j.neubiorev.2013.04.005
Howley, E. T. (2001). Type of activity. Resistance, aerobic and leisure versus occupational physical activity. Med. Sci. Sports Exerc. 33, S364–S369; discussion S419–S420.
Huang, T., Larsen, K. T., Ried-Larsen, M., Moller, N. C., and Andersen, L. B. (2014). The effects of physical activity and exercise on brain-derived neurotrophic factor in healthy humans: a review. Scand. J. Med. Sci. Sports 24, 1–10. doi: 10.1111/sms.12069
Hubal, M. J., Gordish-Dressman, H., Thompson, P. D., Price, T. B., Hoffman, E. P., Angelopoulos, T. J., et al. (2005). Variability in muscle size and strength gain after unilateral resistance training. Med. Sci. Sports Exerc. 37, 964–972.
Hwang, J., Brothers, R. M., Castelli, D. M., Glowacki, E. M., Chen, Y. T., Salinas, M. M., et al. (2016). Acute high-intensity exercise-induced cognitive enhancement and brain-derived neurotrophic factor in young, healthy adults. Neurosci. Lett. 630, 247–253. doi: 10.1016/j.neulet.2016.07.033
Hyodo, K., Dan, I., Kyutoku, Y., Suwabe, K., Byun, K., Ochi, G., et al. (2016). The association between aerobic fitness and cognitive function in older men mediated by frontal lateralization. NeuroImage 125, 291–300. doi: 10.1016/j.neuroimage.2015.09.062
Impellizzeri, F. M., Marcora, S. M., and Coutts, A. J. (2019). Internal and external training load: 15 years on. Int. J. Sports Physiol. Perform. 14, 270–273. doi: 10.1123/ijspp.2018-0935
Jiménez-Maldonado, A., Rentería, I., García-Suárez, P. C., Moncada-Jiménez, J., and Freire-Royes, L. F. (2018). The Impact of high-intensity interval training on brain derived neurotrophic factor in brain: a mini-review. Front. Neurosci. 12:839. doi: 10.3389/fnins.2018.00839
Jones, N., Kiely, J., Suraci, B., Collins, D., de Lorenzo, D., Pickering, C., et al. (2016). A genetic-based algorithm for personalized resistance-training. Biol. Sport 33, 117–126. doi: 10.5604/20831862.1198210
Joyner, M. J. (2019). Limits to the evidence that dna sequence differences contribute to variability in fitness and trainability. Med. Sci. Sports Exerc. 51, 1786–1789. doi: 10.1249/MSS.0000000000001977
Joyner, M. J., and Lundby, C. (2018). Concepts about V⋅O2max and trainability are context dependent. Exerc. Sport Sci. Rev. 46, 138–143. doi: 10.1249/JES.0000000000000150
Karavirta, L., Häkkinen, K., Kauhanen, A., Arija-Blázquez, A., Sillanpää, E., Rinkinen, N., et al. (2011). Individual responses to combined endurance and strength training in older adults. Med. Sci. Sports Exerc. 43, 484–490. doi: 10.1249/MSS.0b013e3181f1bf0d
Karvonen, J., and Vuorimaa, T. (1988). Heart rate and exercise intensity during sports activities. Pract. Appl. Sports Med. 5, 303–311. doi: 10.2165/00007256-198805050-00002
Katch, V., Weltman, A., Sady, S., and Freedson, P. (1978). Validity of the relative percent concept for equating training intensity. Eur. J. Appl. Physiol. 39, 219–227. doi: 10.1007/BF00421445
Katch, V. L., Sady, S. S., and Freedson, P. (1982). Biological variability in maximum aerobic power. Med. Sci. Sports Exerc. 14, 21–25
Kemppainen, J., Aalto, S., Fujimoto, T., Kalliokoski, K. K., Långsjö, J., Oikonen, V., et al. (2005). High intensity exercise decreases global brain glucose uptake in humans. J. Physiol. 568, 323–332. doi: 10.1113/jphysiol.2005.091355
Khaksari, K., Condy, E., Millerhagen, J. B., Anderson, A. A., Dashtestani, H., and Gandjbakhche, A. H. (2019). Effects of Performance and task duration on mental workload during working memory task. Photonics 6, 94. doi: 10.3390/photonics6030094
Knaepen, K., Goekint, M., Heyman, E. M., and Meeusen, R. (2010). Neuroplasticity - exercise-induced response of peripheral brain-derived neurotrophic factor: a systematic review of experimental studies in human subjects. Sports Med. 40, 765–801. doi: 10.2165/11534530-000000000-00000
Kohane, I. S. (2009). The twin questions of personalized medicine: who are you and whom do you most resemble? Genome Med. 1:4. doi: 10.1186/gm4
Komulainen, P., Kivipelto, M., Lakka, T. A., Savonen, K., Hassinen, M., Kiviniemi, V., et al. (2010). Exercise, fitness and cognition – A randomised controlled trial in older individuals. The DR’s EXTRA study. Eur. Geriatr. Med. 1, 266–272. doi: 10.1016/j.eurger.2010.08.001
Kramer, A. F., Hahn, S., Cohen, N. J., Banich, M. T., McAuley, E., Harrison, C. R., et al. (1999). Ageing, fitness and neurocognitive function. Nature 400, 418–419. doi: 10.1038/22682
Ku, P. -W., Liu, Y. -T., Lo, M. -K., Chen, L. -J., and Stubbs, B. (2017). Higher levels of objectively measured sedentary behavior is associated with worse cognitive ability. Two-year follow-up study in community-dwelling older adults. Exp. Gerontol. 99, 110–114. doi: 10.1016/j.exger.2017.09.014
Laurin, D., Verreault, R., Lindsay, J., MacPherson, K., and Rockwood, K. (2001). Physical activity and risk of cognitive impairment and dementia in elderly persons. Arch. Neurol. 58, 498–504. doi: 10.1001/archneur.58.3.498
Leckie, R. L., Oberlin, L. E., Voss, M. W., Prakash, R. S., Szabo-Reed, A., Chaddock-Heyman, L., et al. (2014). BDNF mediates improvements in executive function following a 1-year exercise intervention. Front. Hum. Neurosci. 8:985. doi: 10.3389/fnhum.2014.00985
Lee, J. K., Koh, A. C., Koh, S. X., Liu, G. J., Nio, A. Q., and Fan, P. W. (2014). Neck cooling and cognitive performance following exercise-induced hyperthermia. Eur. J. Appl. Physiol. 114, 375–384. doi: 10.1007/s00421-013-2774-9
Leifer, E. S., Brawner, C. A., Fleg, J. L., Kraus, W. E., Whellan, D. J., Piña, I. L., et al. (2014). Are there negative responders to exercise training among heart failure patients? Med. Sci. Sports Exerc. 46, 219–224. doi: 10.1249/MSS.0b013e3182a44164
Lightfoot, J. T. (2008). Commentary on viewpoint: Perspective on the future use of genomics in exercise prescription. J. Appl. Physiol. 104, 1249. doi: 10.1152/japplphysiol.00014.2008
Lindheimer, J. B., Szabo, A., Raglin, J. S., and Beedie, C. (2019). Advancing the understanding of placebo effects in psychological outcomes of exercise: lessons learned and future directions. Eur. J. Sport Sci. doi: 10.1080/17461391.2019.1632937 [Epub ahead of print].
Liu-Ambrose, T., Barha, C. K., and Best, J. R. (2018). Physical activity for brain health in older adults. Appl. Physiol. Nutr. Metab. 43, 1105–1112. doi: 10.1139/apnm-2018-0260
Loprinzi, P. D., and Frith, E. (2018). The role of sex in memory function: considerations and recommendations in the context of exercise. J. Clin. Med. 7:132. doi: 10.3390/jcm7060132
Lucas, S. J. E., Cotter, J. D., Brassard, P., and Bailey, D. M. (2015). High-intensity interval exercise and cerebrovascular health: curiosity, cause, and consequence. J. Cereb. Blood Flow Metab. 35, 902–911. doi: 10.1038/jcbfm.2015.49
Ludyga, S., Gronwald, T., and Hottenrott, K. (2015). Effects of high vs. low cadence training on cyclists’ brain cortical activity during exercise. J. Sci. Med. Sport 19, 342–7. doi: 10.1016/j.jsams.2015.04.003
Ludyga, S., Gronwald, T., and Hottenrott, K. (2016). The athlete’s brain: cross-sectional evidence for neural efficiency during cycling exercise. Neural Plast. 2016:4583674. doi: 10.1155/2016/4583674
Mann, T., Lamberts, R. P., and Lambert, M. I. (2013). Methods of prescribing relative exercise intensity. Physiol. Pract. Consider. Sports Med. 43, 613–625. doi: 10.1007/s40279-013-0045-x
Mann, T. N., Lamberts, R. P., and Lambert, M. I. (2014). High responders and low responders: factors associated with individual variation in response to standardized training. Sports Med. 44, 1113–1124. doi: 10.1007/s40279-014-0197-3
Mattson, M. P. (2012). Evolutionary aspects of human exercise—born to run purposefully. Ageing Res. Rev. 11, 347–352. doi: 10.1016/j.arr.2012.01.007
McClearn, G. E. (1997). Substantial genetic influence on cognitive abilities in twins 80 or more years old. Science 276, 1560–1563. doi: 10.1126/science.276.5318.1560
McLaren, S. J., Macpherson, T. W., Coutts, A. J., Hurst, C., Spears, I. R., and Weston, M. (2018). The relationships between internal and external measures of training load and intensity in team sports. A Meta-Analysis. Sports Med. 48, 641–658. doi: 10.1007/s40279-017-0830-z
McMorris, T., and Hale, B. J. (2015). Is there an acute exercise-induced physiological/biochemical threshold which triggers increased speed of cognitive functioning? A meta-analytic investigation. J. Sport Health Sci. 4, 4–13. doi: 10.1016/j.jshs.2014.08.003
McMorris, T., Turner, A., Hale, B. J., and Sproule, J. (2016). “Beyond the catecholamines hypothesis for an acute exercise–cognition interaction,” in Exercise-Cognition Interaction, ed T. McMorris (San Diego, CA:Elsevier), 65–103.
T. McMorris (ed.) (2016a). “Chronic exercise and cognition in humans,” in Exercise-Cognition Interaction (New York, NY:Elsevier), 167–186.
McMorris, T. (2016b). Developing the catecholamines hypothesis for the acute exercise-cognition interaction in humans: lessons from animal studies. Physiol. Behav. 165, 291–299. doi: 10.1016/j.physbeh.2016.08.011
T. McMorris (ed.) (2016c). “Exercise–cognition interaction: state of the art and future research,” in Exercise-Cognition Interaction (New York, NY:Elsevier), 459–481.
T. McMorris, (ed.) (2016d). “History of research into the acute exercise–cognition interaction,” in Exercise-Cognition Interaction (New York, NY:Elsevier), 1–28.
McSween, M. -P., Coombes, J. S., MacKay, C. P., Rodriguez, A. D., Erickson, K. I., Copland, D. A., et al. (2018). The immediate effects of acute aerobic exercise on cognition in healthy older adults: a systematic review. Sports Med. 49, 67–82. doi: 10.1007/s40279-018-01039-9
Meerlo, P., Mistlberger, R. E., Jacobs, B. L., Heller, H. C., and McGinty, D. (2009). New neurons in the adult brain: the role of sleep and consequences of sleep loss. Sleep Med. Rev. 13, 187–194. doi: 10.1016/j.smrv.2008.07.004
Meyer, T., Gabriel, H. H., and Kindermann, W. (1999). Is determination of exercise intensities as percentages of VO2max or HRmax adequate? Med. Sci. Sports Exerc. 31, 1342–1345.
Montero, D., and Lundby, C. (2017). Refuting the myth of non-response to exercise training: ‘non-responders’ do respond to higher dose of training. J. Physiol. 595, 3377–3387. doi: 10.1113/JP273480
Moreau, D., and Chou, E. (2019). The acute effect of high-intensity exercise on executive function: a meta-analysis. Perspect. Psychol. Sci. 14, 734–764. doi: 10.1177/1745691619850568
Müller, P., Rehfeld, K., Schmicker, M., and Müller, N. (2018). P52. Future directions for physical exercise as personalized medicine. Clin. Neurophysiol. 129:e88. doi: 10.1016/j.clinph.2018.04.689
Müller, P., Schmicker, M., and Müller, N. G. (2017). Präventionsstrategien gegen Demenz [Preventive strategies for dementia]. Zeitschrift für Gerontol. Geriatr. 50(Suppl. 2), 89–95. doi: 10.1007/s00391-017-1202-x
Nes, B. M., Janszky, I., Wisløff, U., Støylen, A., and Karlsen, T. (2013). Age-predicted maximal heart rate in healthy subjects. The HUNT fitness study. Scand. J. Med. Sci. Sports 23, 697–704. doi: 10.1111/j.1600-0838.2012.01445.x
Netz, Y. (2019). Is there a preferred mode of exercise for cognition enhancement in older age?—a narrative review. Front. Med. 6:1. doi: 10.3389/fmed.2019.00057
Northey, J. M., Cherbuin, N., Pumpa, K. L., Smee, D. J., and Rattray, B. (2017). Exercise interventions for cognitive function in adults older than 50. A systematic review with meta-analysis. Br. J. Sports Med. 52, 154–160. doi: 10.1136/bjsports-2016-096587
Ono, Y., Noah, J. A., Zhang, X., Nomoto, Y., Suzuki, T., Shimada, S., et al. (2015). Motor learning and modulation of prefrontal cortex. An fNIRS assessment. J. Neural Eng. 12:66004. doi: 10.1088/1741-2560/12/6/066004
Ono, Y., Nomoto, Y., Tanaka, S., Sato, K., Shimada, S., Tachibana, A., et al. (2014). Frontotemporal oxyhemoglobin dynamics predict performance accuracy of dance simulation gameplay: temporal characteristics of top-down and bottom-up cortical activities. NeuroImage 85(Pt 11), 461–470. doi: 10.1016/j.neuroimage.2013.05.071
Owen, N., Sparling, P. B., Healy, G. N., Dunstan, D. W., and Matthews, C. E. (2010). Sedentary behavior. Emerging evidence for a new health risk. Mayo Clin. Proc. 85, 1138–1141. doi: 10.4065/mcp.2010.0444
Paillard, T. (2015). Preventive effects of regular physical exercise against cognitive decline and the risk of dementia with age advancement. Sports Med. Open 1:4. doi: 10.1186/s40798-015-0016-x
Paillard-Borg, S., Fratiglioni, L., Winblad, B., and Wang, H. -X. (2009). Leisure activities in late life in relation to dementia risk. Principal component analysis. Dement. Geriatr. Cogn. Disord. 28, 136–144. doi: 10.1159/000235576
Peck, R. W. (2018). Precision medicine is not just genomics: the right dose for every patient. Annu. Rev. Pharmacol. Toxicol. 58, 105–122. doi: 10.1146/annurev-pharmtox-010617-052446
Pedersen, B. K. (2019). Physical activity and muscle-brain crosstalk. Nat. Rev. Endocrinol. 15, 383–392. doi: 10.1038/s41574-019-0174-x
Pentikäinen, H., Savonen, K., Ngandu, T., Solomon, A., Komulainen, P., Paajanen, T., et al. (2019). Cardiorespiratory fitness and cognition: longitudinal associations in the FINGER study. J. Alzheimers Dis. 68, 961–968. doi: 10.3233/JAD-180897
Pescatello, L. S. (2008). Commentary on viewpoint: perspective on the future use of genomics in exercise prescription. J. Appl. Physiol. 104:1247. doi: 10.1152/japplphysiol.01312.2007
Phillips, C. (2017). Lifestyle modulators of neuroplasticity: how physical activity, mental engagement, and diet promote cognitive health during aging. Neural Plast. 2017:3589271. doi: 10.1155/2017/3589271
Pickering, C., and Kiely, J. (2017a). ACTN3: more than just a gene for speed. Front. Physiol. 8:1080. doi: 10.3389/fphys.2017.01080
Pickering, C., and Kiely, J. (2017b). Exercise genetics: seeking clarity from noise. BMJ Open Sport Exerc. Med. 3:e000309. doi: 10.1136/bmjsem-2017-000309
Pickering, C., and Kiely, J. (2017c). Understanding personalized training responses: can genetic assessment help? Open Sports Sci. J. 10, 191–213. doi: 10.2174/1875399X01710010191
Pickering, C., and Kiely, J. (2018a). ACTN3, morbidity, and healthy aging. Front. Genet. 9:15. doi: 10.3389/fgene.2018.00015
Pickering, C., and Kiely, J. (2018b). Do non-responders to exercise exist—and if so, what should we do about them? Sports Med. 49, 1–7. doi: 10.1007/s40279-018-01041-1
Pickering, C., and Kiely, J. (2019a). Exercise response efficiency: a novel way to enhance population health? Lifestyle Genom. 11, 129–135. doi: 10.1159/000501206
Pickering, C., and Kiely, J. (2019b). The development of a personalised training framework: implementation of emerging technologies for performance. JFMK 4:25. doi: 10.3390/jfmk4020025
Plews, D. J., Laursen, P. B., Stanley, J., Kilding, A. E., and Buchheit, M. (2013). Training adaptation and heart rate variability in elite endurance athletes: opening the door to effective monitoring. Sports Med. 43, 773–781. doi: 10.1007/s40279-013-0071-8
Pontifex, M. B., McGowan, A. L., Chandler, M. C., Gwizdala, K. L., Parks, A. C., Fenn, K., et al. (2018). A primer on investigating the after effects of acute bouts of physical activity on cognition. Psychol. Sport Exerc. 40, 1–22. doi: 10.1016/j.psychsport.2018.08.015
Poulose, S. M., Miller, M. G., Scott, T., and Shukitt-Hale, B. (2017). Nutritional factors affecting adult neurogenesis and cognitive function. Adv. Nutr. 8, 804–811. doi: 10.3945/an.117.016261
Proia, P., Di Liegro, C. M., Schiera, G., Fricano, A., and Di Liegro, I. (2016). Lactate as a metabolite and a regulator in the central nervous system. Int. J. Mol. Sci. 17:1450. doi: 10.3390/ijms17091450
Quistorff, B., Secher, N. H., and van Lieshout, J. J. (2008). Lactate fuels the human brain during exercise. FASEB J. 22, 3443–3449. doi: 10.1096/fj.08-106104
Raichlen, D. A., and Alexander, G. E. (2017). Adaptive capacity: an evolutionary neuroscience model linking exercise, cognition, and brain health. Trends Neurosci. 40, 408–421. doi: 10.1016/j.tins.2017.05.001
Ratamess, N., Alvar, B. A., Evetoch, T. K., Housh, T. J., Kibler, W. B., Kraemer, W. J., et al. (2009). American college of sports medicine position stand. Progression models in resistance training for healthy adults. Med. Sci. Sports Exerc. 41, 687–708. doi: 10.1249/MSS.0b013e3181915670
Ravaglia, G., Forti, P., Lucicesare, A., Pisacane, N., Rietti, E., Bianchin, M., et al. (2008). Physical activity and dementia risk in the elderly: findings from a prospective Italian study. Neurology 70, 1786–1794. doi: 10.1212/01.wnl.0000296276.50595.8
Raven, F., van der Zee, E. A., Meerlo, P., and Havekes, R. (2018). The role of sleep in regulating structural plasticity and synaptic strength: implications for memory and cognitive function. Sleep Med. Rev. 39, 3–11. doi: 10.1016/j.smrv.2017.05.002
Riske, L., Thomas, R. K., Baker, G. B., and Dursun, S. M. (2017). Lactate in the brain: an update on its relevance to brain energy, neurons, glia and panic disorder. Ther. Adv. Psychopharmacol. 7, 85–89. doi: 10.1177/2045125316675579
Robergs, R. A., and Landwehr, R. (2002). The surprising history of the “HRmax=220-age” equiation. J. Exerc. Physiol. 5, 1–10
Rooks, C. R., Thom, N. J., McCully, K. K., and Dishman, R. K. (2010). Effects of incremental exercise on cerebral oxygenation measured by near-infrared spectroscopy. A systematic review. Prog. Neurobiol. 92, 134–150. doi: 10.1016/j.pneurobio.2010.06.002
Ross, R., Goodpaster, B. H., Koch, L. G., Sarzynski, M. A., Kohrt, W. M., Johannsen, N. M., et al. (2019). Precision exercise medicine: understanding exercise response variability. Br. J. Sports Med. 53, 1141–1153 doi: 10.1136/bjsports-2018-100328
Ross, R., de Lannoy, L., and Stotz, P. J. (2015). Separate effects of intensity and amount of exercise on interindividual cardiorespiratory fitness response. Mayo Clin. Proc. 90, 1506–1514. doi: 10.1016/j.mayocp.2015.07.024
Rovio, S., Kåreholt, I., Helkala, E. -L., Viitanen, M., Winblad, B., Tuomilehto, J., et al. (2005). Leisure-time physical activity at midlife and the risk of dementia and Alzheimer’s disease. Lancet Neurol. 4, 705–711. doi: 10.1016/S1474-4422(05)70198-8
Sanders, L. M. J., Hortobágyi, T., La Bastide-van Gemert, S., van der Zee, E. A., and van, Heuvelen, M. J. G. (2019). Dose-response relationship between exercise and cognitive function in older adults with and without cognitive impairment: a systematic review and meta-analysis. PLoS One 14:e0210036. doi: 10.1371/journal.pone.0210036
Santos-Concejero, J., Billaut, F., Grobler, L., Oliván, J., Noakes, T. D., and Tucker, R. (2015). Maintained cerebral oxygenation during maximal self-paced exercise in elite Kenyan runners. J. Appl. Physiol. 118, 156–162. doi: 10.1152/japplphysiol.00909.2014
Santos-Concejero, J., Billaut, F., Grobler, L., Olivan, J., Noakes, T. D., and Tucker, R. (2017). Brain oxygenation declines in elite Kenyan runners during a maximal interval training session. Eur. J. Appl. Physiol. 117(5):1017–1024. doi: 10.1007/s00421-017-3590-4
Sarzynski, M. A., Rankinen, T., Earnest, C. P., Leon, A. S., Rao, D. C., Skinner, J. S., et al. (2013). Measured maximal heart rates compared to commonly used age-based prediction equations in the heritage family study. Am. J. Hum. Biol. 25, 695–701. doi: 10.1002/ajhb.22431
Scharhag-Rosenberger, F., Meyer, T., Gässler, N., Faude, O., and Kindermann, W. (2010). Exercise at given percentages of VO2max. Heterogeneous metabolic responses between individuals. J. Sci. Med. Sport 13, 74–79. doi: 10.1016/j.jsams.2008.12.626
Scharhag-Rosenberger, F., Walitzek, S., Kindermann, W., and Meyer, T. (2012). Differences in adaptations to 1 year of aerobic endurance training: individual patterns of nonresponse. Scand. J. Med. Sci. Sports 22, 113–118. doi: 10.1111/j.1600-0838.2010.01139.x
Scheuer, J., and Tipton, C. M. (1977). Cardiovascular adaptations to physical training. Annu. Rev. Physiol. 39, 221–251. doi: 10.1146/annurev.ph.39.030177.001253
Schiffer, T., Schulte, S., Sperlich, B., Achtzehn, S., Fricke, H., and Struder, H. K. (2011). Lactate infusion at rest increases BDNF blood concentration in humans. Neurosci. Lett. 488, 234–237. doi: 10.1016/j.neulet.2010.11.035
Schmitt, K., Holsboer-Trachsler, E., and Eckert, A. (2016). BDNF in sleep, insomnia, and sleep deprivation. Ann. Med. 48, 42–51. doi: 10.3109/07853890.2015.1131327
Seidel, O., Carius, D., Kenville, R., and Ragert, P. (2017). Motor learning in a complex balance task and associated neuroplasticity. A comparison between endurance athletes and non-athletes. J. Neurophysiol. 118, 1849–1860. doi: 10.1152/jn.00419.2017
Shargal, E., Kislev-Cohen, R., Zigel, L., Epstein, S., Pilz-Burstein, R., and Tenenbaum, G. (2015). Age-related maximal heart rate: examination and refinement of prediction equations. J. Sports Med. Phys. Fitness 55, 1207–1218.
Silva, V. A., Bottaro, M., Justino, M. A., Ribeiro, M. M., Lima, R. M., and Oliveira, R. J. (2007). Maximum heart rate in brazilian elderly women: comparing measured and predicted values. Arq. Bras. Cardiol. 88, 147–162. doi: 10.1590/S0066-782X2007000300010
Skurvydas, A., Brazaitis, M., Kamandulis, S., and Sipaviciene, S. (2010). Muscle damaging exercise affects isometric force fluctuation as well as intraindividual variability of cognitive function. J. Mot. Behav. 42, 179–186. doi: 10.1080/00222891003751835
Smiley-Oyen, A. L., Lowry, K. A., Francois, S. J., Kohut, M. L., and Ekkekakis, P. (2008). Exercise, fitness, and neurocognitive function in older adults: the “selective improvement” and “cardiovascular fitness” hypotheses. Ann. Behav. Med. 36, 280–291. doi: 10.1007/s12160-008-9064-5
Sobol, N. A., Dall, C. H., Høgh, P., Hoffmann, K., Frederiksen, K. S., Vogel, A., et al. (2018). Change in fitness and the relation to change in cognition and neuropsychiatric symptoms after aerobic exercise in patients with mild Alzheimer’s disease. J. Alzheimers Dis. 65, 137–145. doi: 10.3233/JAD-180253
Sobral-Monteiro-Junior, R., Maillot, P., Gatica-Rojas, V., Ávila, W. R. M., de Paula, A. M. B., Guimarães, A. L. S., et al. (2019). Is the “lactormone” a key-factor for exercise-related neuroplasticity? A hypothesis based on an alternative lactate neurobiological pathway. Med. Hypotheses 123, 63–66. doi: 10.1016/j.mehy.2018.12.013
Soligard, T., Schwellnus, M., Alonso, J. -M., Bahr, R., Clarsen, B., Dijkstra, H. P., et al. (2016). How much is too much? (Part 1) international olympic committee consensus statement on load in sport and risk of injury. Br. J. Sports Med. 50, 1030–1041. doi: 10.1136/bjsports-2016-096581
Solomon, T. P. J. (2018). Sources of inter-individual variability in the therapeutic response of blood glucose control to exercise in type 2 diabetes: going beyond exercise dose. Front. Physiol. 9:896. doi: 10.3389/fphys.2018.00896
Sparks, L. M. (2017). Exercise training response heterogeneity: physiological and molecular insights. Diabetologia 60, 2329–2336. doi: 10.1007/s00125-017-4461-6
Spiering, B. A., Kraemer, W. J., Anderson, J. M., Armstrong, L. E., Nindl, B. C., Volek, J. S., et al. (2008). Resistance exercise biology: manipulation of resistance exercise programme variables determines the responses of cellular and molecular signalling pathways. Sports Med. 38, 527–540. doi: 10.2165/00007256-200838070-00001
Stern, Y., MacKay-Brandt, A., Lee, S., McKinley, P., McIntyre, K., Razlighi, Q., et al. (2019). Effect of aerobic exercise on cognition in younger adults: a randomized clinical trial. Neurology 92, e905–e916. doi: 10.1212/WNL.0000000000007003
Stillman, C. M., Cohen, J., Lehman, M. E., and Erickson, K. I. (2016). Mediators of physical activity on neurocognitive function: a review at multiple levels of analysis. Front. Hum. Neurosci. 10:626. doi: 10.3389/fnhum.2016.00626
Stillman, C. M., and Erickson, K. I. (2018). Physical activity as a model for health neuroscience. Ann. N. Y. Acad. Sci. 1428, 103–111. doi: 10.1111/nyas.13669
Stillman, C. M., Uyar, F., Huang, H., Grove, G. A., Watt, J. C., Wollam, M. E., et al. (2018). Cardiorespiratory fitness is associated with enhanced hippocampal functional connectivity in healthy young adults. Hippocampus 28, 239–247. doi: 10.1002/hipo.22827
Stimpson, N. J., Davison, G., and Javadi, A.-H. (2018). Joggin’ the Noggin. Towards a physiological understanding of exercise-induced cognitive benefits. Neurosci. Biobehav. Rev. 88, 177–186. doi: 10.1016/j.neubiorev.2018.03.018
Stone, M., Plisk, S., and Collins, D. (2002). Training principles: evaluation of modes and methods of resistance training—a coaching perspective. Sports Biomech. 1, 79–103. doi: 10.1080/14763140208522788
Ströhlein, J. K., van den Bongard, F., Barthel, T., and Reinsberger, C. (2017). Dose-response-relationship between physical activity and cognition in elderly. Dtsch. Z. Sportmed. 2017, 234–242. doi: 10.5960/dzsm.2017.300
Strzelczyk, T. A., Quigg, R. J., Pfeifer, P. B., Parker, M. A., and Greenland, P. (2001). Accuracy of estimating exercise prescription intensity in patients with left ventricular systolic dysfunction. J. Cardiopulm. Rehabil. 21, 158–163.
Suwabe, K., Byun, K., Hyodo, K., Reagh, Z. M., Roberts, J. M., Matsushita, A., et al. (2018). Reply to Gronwald et al. Exercise intensity does indeed matter; maximal oxygen uptake is the gold-standard indicator. Proc. Natl. Acad. Sci. U.S.A. 115, E11892–E11893. doi: 10.1073/pnas.1818247115
Suwabe, K., Hyodo, K., Byun, K., Ochi, G., Fukuie, T., Shimizu, T., et al. (2017). Aerobic fitness associates with mnemonic discrimination as a mediator of physical activity effects. Evidence for memory flexibility in young adults. Sci. Rep. 7:5140. doi: 10.1038/s41598-017-04850-y
Swinton, P. A., Hemingway, B. S., Saunders, B., Gualano, B., and Dolan, E. (2018). A statistical framework to interpret individual response to intervention: paving the way for personalized nutrition and exercise prescription. Front. Nutr. 5:41. doi: 10.3389/fnut.2018.00041
Szabo, A. N., McAuley, E., Erickson, K. I., Voss, M., Prakash, R. S., Mailey, E. L., et al. (2011). Cardiorespiratory fitness, hippocampal volume, and frequency of forgetting in older adults. Neuropsychology 25, 545–553. doi: 10.1037/a0022733
Taher, M., Leen, W. G., Wevers, R. A., and Willemsen, M. A. (2016). Lactate and its many faces. Eur. J. Paediatr. Neurol. 20, 3–10. doi: 10.1016/j.ejpn.2015.09.008
Tait, J. L., Duckham, R. L., Milte, C. M., Main, L. C., and Daly, R. M. (2017). Influence of sequential vs. simultaneous dual-task exercise training on cognitive function in older adults. Front. Aging Neurosci. 9:368. doi: 10.3389/fnagi.2017.00368
Takehara, N., Tsubaki, A., Yamazaki, Y., Kanaya, C., Sato, D., Morishita, S., et al. (2017). Changes in oxyhemoglobin concentration in the prefrontal cortex and primary motor cortex during low- and moderate-intensity exercise on a cycle ergometer. Adv. Exp. Med. Biol. 977, 241–247. doi: 10.1007/978-3-319-55231-6_33
Tan, Z. S., Spartano, N. L., Beiser, A. S., DeCarli, C., Auerbach, S. H., Vasan, R. S., et al. (2017). Physical activity, brain volume, and dementia risk. The framingham study. J. Gerontol. A Biol. Sci. Med. Sci. 72, 789–795. doi: 10.1093/gerona/glw130
Tanaka, H., Monahan, K. D., and Seals, D. R. (2001). Age-predicted maximal heart rate revisited. J. Am. Coll. Cardiol. 37, 153–156. doi: 10.1016/S0735-1097(00)01054-8
Tempest, G. D., Eston, R. G., and Parfitt, G. (2014). Prefrontal cortex haemodynamics and affective responses during exercise. A multi-channel near infrared spectroscopy study. PLoS One 9:e95924. doi: 10.1371/journal.pone.0095924
Thayer, J. F., Åhs, F., Fredrikson, M., Sollers, J. J., and Wager, T. D. (2012). A meta-analysis of heart rate variability and neuroimaging studies. Implications for heart rate variability as a marker of stress and health. Neurosci. Biobehav. Rev. 36, 747–756. doi: 10.1016/j.neubiorev.2011.11.009
Thompson, P. M., Cannon, T. D., Narr, K. L., van Erp, T., Poutanen, V. P., Huttunen, M., et al. (2001). Genetic influences on brain structure. Nat. Neurosci. 4, 1253–1258. doi: 10.1038/nn758
Timmons, J. A. (2011). Variability in training-induced skeletal muscle adaptation. J. Appl. Physiol. 110, 846–853. doi: 10.1152/japplphysiol.00934.2010
Timmons, J. A., Knudsen, S., Rankinen, T., Koch, L. G., Sarzynski, M., Jensen, T., et al. (2010). Using molecular classification to predict gains in maximal aerobic capacity following endurance exercise training in humans. J. Appl. Physiol. 108, 1487–1496. doi: 10.1152/japplphysiol.01295.2009
Toga, A. W., and Thompson, P. M. (2005). Genetics of brain structure and intelligence. Annu. Rev. Neurosci. 28, 1–23. doi: 10.1146/annurev.neuro.28.061604.135655
Törpel, A., Herold, F., Hamacher, D., Müller, N. G., and Schega, L. (2018). Strengthening the brain—is resistance training with blood flow restriction an effective strategy for cognitive improvement? J. Clin. Med. 7:E377. doi: 10.3390/jcm7100337
Trajkovska, V., Marcussen, A. B., Vinberg, M., Hartvig, P., Aznar, S., and Knudsen, G. M. (2007). Measurements of brain-derived neurotrophic factor. Methodological aspects and demographical data. Brain Res. Bull. 73, 143–149. doi: 10.1016/j.brainresbull.2007.03.009
Tsukamoto, H., Suga, T., Takenaka, S., Tanaka, D., Takeuchi, T., Hamaoka, T., et al. (2016). Repeated high-intensity interval exercise shortens the positive effect on executive function during post-exercise recovery in healthy young males. Physiol. Behav. 160, 26–34. doi: 10.1016/j.physbeh.2016.03.029
van Hall, G., Stromstad, M., Rasmussen, P., Jans, O., Zaar, M., Gam, C., et al. (2009). Blood lactate is an important energy source for the human brain. J. Cereb. Blood Flow Metab. 29, 1121–1129. doi: 10.1038/jcbfm.2009.35
Vanrenterghem, J., Nedergaard, N. J., Robinson, M. A., and Drust, B. (2017). Training load monitoring in team sports. A novel framework separating physiological and biomechanical load-adaptation pathways. Sports Med. 47, 2135–2142. doi: 10.1007/s40279-017-0714-2
Verstynen, T. D., Lynch, B., Miller, D. L., Voss, M. W., Prakash, R. S., Chaddock, L., et al. (2012). Caudate nucleus volume mediates the link between cardiorespiratory fitness and cognitive flexibility in older adults. J. Aging Res. 2012:939285. doi: 10.1155/2012/939285
Vesterinen, V., Häkkinen, K., Hynynen, E., Mikkola, J., Hokka, L., and Nummela, A. (2013). Heart rate variability in prediction of individual adaptation to endurance training in recreational endurance runners. Scan. J. Med. Sci. Sports 23, 171–180. doi: 10.1111/j.1600-0838.2011.01365.x
Vesterinen, V., Nummela, A., Heikura, I., Laine, T., Hynynen, E., Botella, J., et al. (2016). Individual endurance training prescription with heart rate variability. Med. Sci. Sports Exerc. 48, 1347–1354. doi: 10.1249/MSS.0000000000000910
Vidoni, E. D., Honea, R. A., Billinger, S. A., Swerdlow, R. H., and Burns, J. M. (2012). Cardiorespiratory fitness is associated with atrophy in Alzheimer’s and aging over 2 years. Neurobiol. Aging 33, 1624–1632. doi: 10.1016/j.neurobiolaging.2011.03.016
Voelcker-Rehage, C., Godde, B., and Staudinger, U. M. (2011). Cardiovascular and coordination training differentially improve cognitive performance and neural processing in older adults. Front. Hum. Neurosci. 5:26. doi: 10.3389/fnhum.2011.00026
Voils, C. I., Chang, Y., Crandell, J., Leeman, J., Sandelowski, M., and Maciejewski, M. L. (2012). Informing the dosing of interventions in randomized trials. Contemp. Clin. Trials 33, 1225–1230. doi: 10.1016/j.cct.2012.07.011
Voisin, S., Jacques, M., Lucia, A., Bishop, D. J., and Eynon, N. (2019). Statistical considerations for exercise protocols aimed at measuring trainability. Exerc. Sport Sci. Rev. 47, 37–45. doi: 10.1249/JES.0000000000000176
Vollaard, N. B. J., Constantin-Teodosiu, D., Fredriksson, K., Rooyackers, O., Jansson, E., Greenhaff, P. L., et al. (2009). Systematic analysis of adaptations in aerobic capacity and submaximal energy metabolism provides a unique insight into determinants of human aerobic performance. J. Appl. Physiol. 106, 1479–1486. doi: 10.1152/japplphysiol.91453.2008
Voss, M. W. (2016). “The chronic exercise–cognition interaction,” in Exercise-Cognition Interaction ed. T. McMorris (Elsevier: San Diego, CA), 187–209.
Voss, M. W., Erickson, K. I., Prakash, R. S., Chaddock, L., Kim, J. S., Alves, H., et al. (2013a). Neurobiological markers of exercise-related brain plasticity in older adults. Brain Behav. Immun. 28, 90–99. doi: 10.1016/j.bbi.2012.10.021
Voss, M. W., Vivar, C., Kramer, A. F., and van Praag, H. (2013b). Bridging animal and human models of exercise-induced brain plasticity. Trends Cogn. Sci. 17, 525–544. doi: 10.1016/j.tics.2013.08.001
Voss, M. W., Nagamatsu, L. S., Liu-Ambrose, T., and Kramer, A. F. (2011). Exercise, brain, and cognition across the life span. J. Appl. Physiol. 111, 1505–1513. doi: 10.1152/japplphysiol.00210.2011
Wallace, L. K., Slattery, K. M., and Coutts, A. J. (2009). The ecological validity and application of the session-RPE method for quantifying training loads in swimming. J. Strength Cond. Res. 23, 33–38. doi: 10.1519/JSC.0b013e3181874512
Walsh, J. J., Edgett, B. A., Tschakovsky, M. E., and Gurd, B. J. (2015). Fasting and exercise differentially regulate BDNF mRNA expression in human skeletal muscle. Appl. Physiol. Nutr. Metab. 40, 96–98. doi: 10.1139/apnm-2014-0290
Wang, L., Yoshikawa, T., Hara, T., Nakao, H., Suzuki, T., and Fujimoto, S. (2006). Which common NIRS variable reflects muscle estimated lactate threshold most closely? Appl. Physiol. Nutr. Metab. 31, 612–620. doi: 10.1139/h06-069
Wasfy, M. M., and Baggish, A. L. (2016). Exercise dose in clinical practice. Circulation 133, 2297–2313. doi: 10.1161/CIRCULATIONAHA.116.018093
Weatherwax, R. M., Harris, N. K., Kilding, A. E., and Dalleck, L. C. (2016). The incidence of training responsiveness to cardiorespiratory fitness and cardiometabolic measurements following individualized and standardized exercise prescription: study protocol for a randomized controlled trial. Trials 17:601. doi: 10.1186/s13063-016-1735-0
Weltman, A., Snead, D., Seip, R., Schurrer, R., Weltman, J., Rutt, R., et al. (1990). Percentages of maximal heart rate, heart rate reserve and VO2max for determining endurance training intensity in male runners. Int. J. Sports Med. 11, 218–222. doi: 10.1055/s-2007-1024795
Weltman, A., Weltman, J., Rutt, R., Seip, R., Levine, S., Snead, D., et al. (1989). Percentages of maximal heart rate, heart rate reserve, and VO2peak for determining endurance training intensity in sedentary women. Int. J. Sports Med. 10, 212–216. doi: 10.1055/s-2007-1024903
Wengaard, E., Kristoffersen, M., Harris, A., and Gundersen, H. (2017). Cardiorespiratory fitness is associated with selective attention in healthy male high-school students. Front. Hum. Neurosci. 11:330. doi: 10.3389/fnhum.2017.00330
Williams, C. J., Gurd, B. J., Bonafiglia, J. T., Voisin, S., Li, Z., Harvey, N., et al. (2019). A multi-center comparison of o2peak trainability between interval training and moderate intensity continuous training. Front. Physiol. 10:480. doi: 10.3389/fphys.2019.00019
Williamson, P. J., Atkinson, G., and Batterham, A. M. (2017). Inter-individual responses of maximal oxygen uptake to exercise training: a critical review. Sports Med. 47, 1501–1513. doi: 10.1007/s40279-017-0680-8
Wilson, G. C., Mavros, Y., Tajouri, L., and Singh, M. F. (2019). The role of genetic profile in functional performance adaptations to exercise training or physical activity: a systematic review of the literature. J. Aging Phys. Act. 27, 594–616. doi: 10.1123/japa.2018-0155
Winters-Stone, K. M., Neil, S. E., and Campbell, K. L. (2014). Attention to principles of exercise training: a review of exercise studies for survivors of cancers other than breast. Br. J. Sports Med. 48, 987–995. doi: 10.1136/bjsports-2012-091732
Xu, G., Mao, Z., Ye, Y., and Lv, K. (2011). Relationship between muscle oxygenation by NIRS and blood lactate. J. Phys. Conf. Ser. 277:12042. doi: 10.1088/1742-6596/277/1/012042
Yamazaki, Y., Sato, D., Yamashiro, K., Tsubaki, A., Takehara, N., Uetake, Y., et al. (2018). Inter-individual differences in working memory improvement after acute mild and moderate aerobic exercise. PLoS One 13:e0210053. doi: 10.1371/journal.pone.0210053
Yamazaki, Y., Sato, D., Yamashiro, K., Tsubaki, A., Yamaguchi, Y., Takehara, N., et al. (2017). Inter-individual differences in exercise-induced spatial working memory improvement. A near-infrared spectroscopy study. Adv. Exp. Med. Biol. 977, 81–88. doi: 10.1007/978-3-319-55231-6_12
Zeki, Al Hazzouri, A., Haan, M. N., Deng, Y., Neuhaus, J., and Yaffe, K. (2014). Reduced heart rate variability is associated with worse cognitive performance in elderly Mexican Americans. Hypertension 63, 181–187. doi: 10.1161/HYPERTENSIONAHA.113.01888
Zhu, N., Suarez-Lopez, J. R., Sidney, S., Sternfeld, B., Schreiner, P. J., Carnethon, M. R., et al. (2010). Longitudinal examination of age-predicted symptom-limited exercise maximum HR. Med. Sci. Sports Exerc. 42, 1519–1527. doi: 10.1249/MSS.0b013e3181cf8242
Keywords: physical activity, cognition, personalized training, personalized medicine, neuroplasticity, neuroprotection
Citation: Herold F, Müller P, Gronwald T and Müller NG (2019) Dose–Response Matters! – A Perspective on the Exercise Prescription in Exercise–Cognition Research. Front. Psychol. 10:2338. doi: 10.3389/fpsyg.2019.02338
Received: 29 April 2019; Accepted: 01 October 2019;
Published: 01 November 2019.
Edited by:
Ana Bengoetxea, Université Libre de Bruxelles, BelgiumReviewed by:
Jon Irazusta, University of the Basque Country, SpainJosé Augusto Marinho Alves, Polytechnic Institute of Santarém, Portugal
Copyright © 2019 Herold, Müller, Gronwald and Müller. This is an open-access article distributed under the terms of the Creative Commons Attribution License (CC BY). The use, distribution or reproduction in other forums is permitted, provided the original author(s) and the copyright owner(s) are credited and that the original publication in this journal is cited, in accordance with accepted academic practice. No use, distribution or reproduction is permitted which does not comply with these terms.
*Correspondence: Fabian Herold, ZmFiaWFuLmhlcm9sZEBkem5lLmRl