- Department of Medical Biophysics and Medical Informatics, Third Faculty of Medicine, Charles University in Prague, Prague, Czechia
Working memory (WM) is the active retention and processing of information over a few seconds and is considered an essential component of cognitive function. The reduced WM capacity is a common feature in many diseases, such as schizophrenia, attention deficit hyperactivity disorder (ADHD), mild cognitive impairment (MCI), and Alzheimer's disease (AD). The theta-gamma neural code is an essential component of memory representations in the multi-item WM. A large body of studies have examined the association between cross-frequency coupling (CFC) across the cerebral cortices and WM performance; electrophysiological data together with the behavioral results showed the associations between CFC and WM performance. The oscillatory entrainment (sensory, non-invasive electrical/magnetic, and invasive electrical) remains the key method to investigate the causal relationship between CFC and WM. The frequency-tuned non-invasive brain stimulation is a promising way to improve WM performance in healthy and non-healthy patients with cognitive impairment. The WM performance is sensitive to the phase and rhythm of externally applied stimulations. CFC-transcranial-alternating current stimulation (CFC-tACS) is a recent approach in neuroscience that could alter cognitive outcomes. The studies that investigated (1) the association between CFC and WM and (2) the brain stimulation protocols that enhanced WM through modulating CFC by the means of the non-invasive brain stimulation techniques have been included in this review. In principle, this review can guide the researchers to identify the most prominent form of CFC associated with WM processing (e.g., theta/gamma phase-amplitude coupling), and to define the previously published studies that manipulate endogenous CFC externally to improve WM. This in turn will pave the path for future studies aimed at investigating the CFC-tACS effect on WM. The CFC-tACS protocols need to be thoroughly studied before they can be considered as therapeutic tools in patients with WM deficits.
Introduction to Brain Oscillations and Working Memory
The brain (neuronal) oscillations arise from the simultaneous interactions between the neuronal networks and are divided into five frequency bands: delta (0.5–3.5 Hz), theta (3.5–7 Hz), alpha (8–13 Hz), beta (18–25 Hz), and gamma (30–70 Hz) (Düzel et al., 2010; Başar, 2013; Merker, 2013; Luo and Guan, 2018). The brain oscillations can be detected by scalp electroencephalography (EEG) or directly in the cortex (Electrocorticography, ECoG, or intracranial EEG). Similarly, the neuronal oscillations originating from the sulci can be detected with scalp magnetoencephalography (MEG) (Marzetti et al., 2019; Andersen et al., 2020). The EEG frequency bands reflect the rhythmic and synchronized postsynaptic potentials that arise from the pyramidal neuronal assemblies (Jensen et al., 2014). Moreover, brain oscillations are predictive of information processing and are involved in selective communication and information transmission (Fries et al., 2001; Fries, 2015). The power of the oscillation bands and the coupling between the brain regions constantly change in response to task demands (Klimesch, 2018). For instance, gamma-band synchronization is triggered by stimuli and is essential for cortical computation (Fries, 2009). All the studies suggest an important role of neuronal oscillations in brain functions (Engel et al., 2001; Niebur, 2002; Buzsáki and Draguhn, 2004; Mann and Paulsen, 2005).
The neuronal oscillations play different roles in cognition/psychology: delta bands are associated with deep sleep and long-range coordination between the neuronal networks (Hiltunen et al., 2014; Leszczyński et al., 2015); theta bands are represented in shallow sleep, meditative states, coordination of memory encoding and maintenance (hippocampal theta), and long-range coordination of cognition (cortical theta) (Sederberg et al., 2003; Axmacher et al., 2010; Sauseng et al., 2010; Cohen, 2014); alpha bands are commonly associated with rest, relaxation, memory, and motor inhibition (Sauseng et al., 2009; Roux and Uhlhaas, 2014); beta bands are linked to awareness and attention (Egner and Gruzelier, 2004; Buschman and Miller, 2007). In contrast to delta, theta, and beta oscillations, the high-frequency gamma oscillations arise from the negative feedback between the GABAergic interneurons and pyramidal neurons; gamma oscillatory activities perform different computations and represent different information patterns (Fries et al., 2007; Jensen and Colgin, 2007).
Cross-Frequency Coupling
Cross-frequency coupling (CFC) is the interaction between the brain oscillations on different frequency bands (Jirsa and Müller, 2013; Sotero, 2016; Siebenhühner et al., 2020). From a theoretical perspective, there are four ways in which CFC can occur: phase-to-amplitude, power-to-power, phase-to-phase, and phase-to-frequency interactions (Jensen and Colgin, 2007; Helfrich et al., 2016). In power-to-power coupling: the changes in the power of the faster oscillations are correlated with the power changes in the lower frequency bands; in phase to phase coupling: phase-locking occurs between oscillations at different frequencies and their phase relationship remains constant; in phase to power coupling: the power of the fast oscillations is modulated by the phase of the slow oscillations (Schack et al., 2002; Bruns and Eckhorn, 2004; Lakatos et al., 2005; Mormann et al., 2005; Canolty et al., 2006). The phase-amplitude coupling (PAC) is a widely observed model of CFC in which the high-frequency amplitudes are modulated by the low-frequency phases (Canolty and Knight, 2010; Siems and Siegel, 2020). Abnormal CFCs have been reported by several studies conducted in patients with Parkinson's disease, Alzheimer's disease (AD), schizophrenia, mental disorders, and anxiety (Allen et al., 2011; De Hemptinne et al., 2013; Alegre, 2016; Lynn and Sponheim, 2016; Wang et al., 2017). The neural modulations/entrainments are classically divided into three approaches: sensory, non-invasive electrical/magnetic, and invasive electrical entrainment (Thut and Miniussi, 2009; Calderone et al., 2014; Herrmann et al., 2016; Hanslmayr et al., 2019).
Working Memory
Working memory (WM) is the active retention and manipulation of information over a few seconds and is considered an essential component of cognitive function (Aben et al., 2012; Cowan, 2014, 2017; Persuh et al., 2018). Although the storage capacity of WM is inherently limited (Fougnie et al., 2015), several studies have found that the WM capacity can be altered by training (Botvinick and Watanabe, 2007; MacOveanu et al., 2007; Edin et al., 2009). Neural activity in the prefrontal cortex and the strength of connectivity between the prefrontal and parietal cortices have been shown to be improved by training, as suggested by the studies in the humans and non-human primates (Klingberg et al., 2002; Jaeggi et al., 2008; Siegel et al., 2012; Constantinidis and Klingberg, 2016). Training has a primary benefit on tasks that are very similar to the training tasks and does not improve overall WM capacity (Hulme and Melby-Lervåg, 2012). From a theoretical perspective, the two terms have been used extensively to describe the temporal storage of information: Short-term memory (STM) and WM. STM is an essential component for holding motion, sensory, and cognitive information for a short interval of time. STM describes the process of passively maintaining the information over a short period of time, while the WM concept depicts the processes of maintaining and manipulating the information for a short period of time. Thus, information manipulation is the main difference between the two concepts (Aben et al., 2012; Cowan, 2017). Despite the differences between STM and WM, the two terms are still used interchangeably in the literature. It has been suggested that the two concepts represent the same cognitive process (Baddeley, 1992; Gathercole and Alloway, 2006; Unsworth and Engle, 2007; Klingberg, 2010; Nadel and Hardt, 2011). The tasks involving only item maintenance have often been used to test STM, such as word span, digit span, and delayed match-to-sample tasks, while the tasks involving item maintenance and manipulation have classically been used to test WM, such as n-back, computation span, mental control, and letter-number sequencing tasks (Engle et al., 1999; Kane et al., 2004; Ackerman et al., 2005; Conway et al., 2005; Colom et al., 2006). In addition, the mental arithmetic tasks have been considered as the primary tasks in WM assessment, since the solution of problems in these tasks activates the WM components (DeStefano and LeFevre, 2010). All the neuronal oscillations are important for the cognitive and memory processes, particularly theta (Klimesch, 1999; Gathercole et al., 2003; Kane et al., 2007; Hsieh and Ranganath, 2014) and gamma bands (Roberts et al., 2013; Roux and Uhlhaas, 2014). The causal relationship between the brain oscillations and memory processes can be tested by modulating the endogenous brain oscillations and assessing the behavioral effects of such modulation.
Several models have been proposed to illustrate the underlying mechanisms behind WM (Kamiński et al., 2011; Van Vugt et al., 2014; Vosskuhl et al., 2015; Wolinski et al., 2018; Sauseng et al., 2019). Two models were adopted; one model states that each memory item is translated into a fast and transient wave that can be detected electro-physiologically (gamma wave). Several individual gamma waves fit into a single theta cycle and the limited WM capacity can be explained by the finite number of gamma waves that can fit into a single theta cycle (Lisman and Idiart, 1995; Jensen and Lisman, 1996). Moreover, the WM capacity of seven items has been reported in the studies that used immediate verbal recall tasks (Gignac, 2015), while the studies that used rehearsal of verbal items, spatial, and visual tasks suggested the STM/WM capacity of four items (Cowan, 2001; Vogel et al., 2001). Theoretically, the STM/WM capacity can be improved by increasing the theta cycle length, or by increasing the gamma frequencies, which increases the number of gamma waves that fit within a given theta cycle (Kamiński et al., 2011). Contrary to the expectations, Malenínská et al. (2021) found no association between the theta/gamma ratio and performance on digit span task (Malenínská et al., 2021).
Vosskuhl et al. (2015) artificially slowed theta frequency to increase the number of gamma waves per single theta cycle and found that the verbal STM capacity was improved compared with the sham stimulation (Vosskuhl et al., 2015). In another study, Wolinski et al. (2018) examined the effect of transcranial alternating current stimulation (tACS) administered at a slow theta (4 Hz), a fast theta frequency (7 Hz), and in a placebo condition over the right parietal cortex while performing visuospatial WM task. They found that tACS administered at 4 Hz had a positive effect on the WM performance, while tACS administered at 7 Hz had a detrimental effect (Wolinski et al., 2018).
In contrast to the first model which assumes that each gamma wave represents a single memory item, the second model assumes that each memory item is encoded by the entire gamma burst (Herman et al., 2013; Van Vugt et al., 2014). After a certain period of time, the memory items need to be refreshed through the new gamma bursts. This reactivation occurs after a few theta cycles, which could explain the limited WM capacity (Van Vugt et al., 2014). Based on this model, a slowing down theta cycle means that fewer memory items could be activated in a given period of time. Thus, one might expect a decrease rather than an increase in the WM capacity. However, the increase in the WM capacity reported by Vosskuhl et al. (2015) and Wolinski et al. (2018) could mean that the gain in memory fidelity due to the greater activation with the longer gamma burst displaces the memory decay resulting from the slowing down theta cycles (Vosskuhl et al., 2015; Wolinski et al., 2018). These two models can be used to predict the increase or decrease in the WM capacity at a given tACS frequency. Thus, based on these models one could design a brain stimulation protocol to boost WM (e.g., theta/gamma CFC tACS).
Differences in the WM capacity between individuals result in variations in several skills, such as attention, academic performance, and non-verbal reasoning ability (Gathercole et al., 2003; Kane et al., 2007). The reduced WM capacity is a common feature in many diseases, such as schizophrenia, stroke, traumatic brain injury, attention deficit hyperactivity disorder (ADHD), mild cognitive impairment (MCI), and AD (Baddeley et al., 1991; Gagnon and Belleville, 2011; Constantinidis and Klingberg, 2016). In addition, abnormal PACs have been associated with diseases, such as AD, epilepsy, mental disorders, and Parkinson's disease (Salimpour and Anderson, 2019). Taken together, this information sheds light on the possible role of CFC in WM and its potential role as a therapeutic target in such diseases. Improving the WM performance is a challenge and a hot topic in clinical practice, especially in patients with AD, MCI, etc., any progress in this area is beneficial. WM can be manipulated/modulated by various approaches, and non-invasive brain stimulation with an electric or magnetic field is one of them. Over the past two decades, there has been a long list of studies reporting the effects of frequency-tuned tACS, and transcranial magnetic stimulation (TMS) on WM (Jaušovec et al., 2014; Hoy et al., 2015, 2016; Chander et al., 2016; Feurra et al., 2016; Alekseichuk et al., 2017; Papazova et al., 2020). It is important to emphasize that there are different forms of tACS, some forms target specific individual frequency bands, such as theta, gamma, and beta (theta-tACS, gamma-tACS, beta-tACS, etc.), and CFC-tACS form; where tACS can modulate the interaction between the two frequency bands, such as theta and gamma (e.g., theta/gamma 6 Hz, 80 Hz peak-CFC-tACS, where gamma bursts at 80 Hz were nested into the peak of theta cycles at a frequency of 6 Hz, which is so-called peak-coupled tACS). Since the topic of this review is CFC of neuronal oscillations and WM in adult humans, the studies that have investigated the effect of non-invasive brain stimulation on CFC (e.g., CFC-tACS), as well as the studies that have investigated the association between CFC of neuronal oscillations and WM in adult humans, are thoroughly discussed in this review and listed in Table 1.
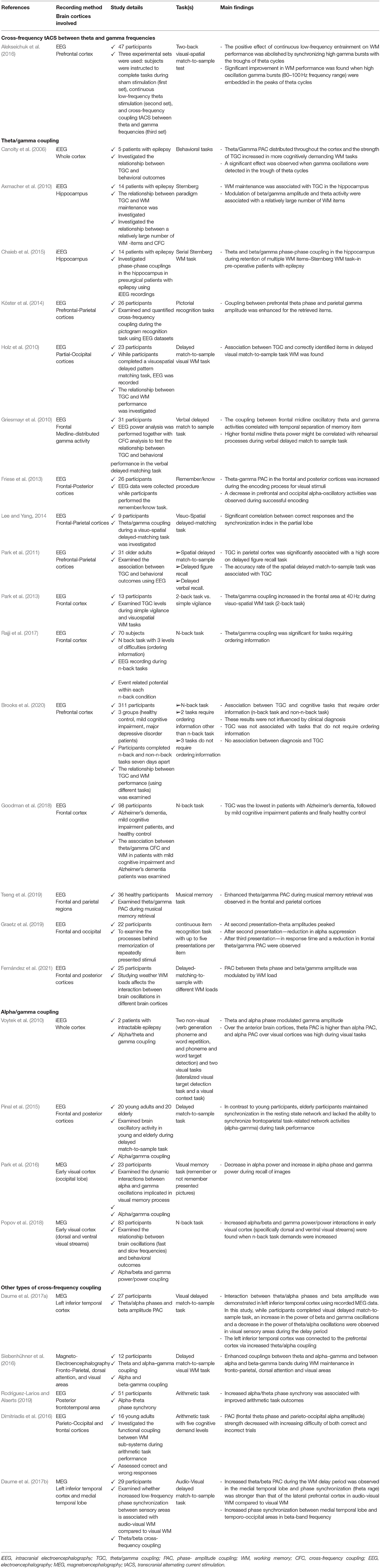
Table 1. Studies that investigated the association between the cross-frequency coupling (CFC) over different brain cortices and working memory (WM) performance.
Theta-Gamma Neural Code and WM
The relationship between the brain oscillations and WM capacity has been investigated in the different brain regions (parietal, frontal, occipital regions, hippocampus…etc.), and several studies have focused on the association between CFC, particularly theta-gamma coupling (TGC), and WM performance (Schack et al., 2002; Demiralp et al., 2007; Mizuhara and Yamaguchi, 2011; Bahramisharif et al., 2018; Biel et al., 2021). Theta/gamma PAC is found in the hippocampus and other brain structures (Maris et al., 2011; Belluscio et al., 2012; van der Meij et al., 2012; Colgin, 2015) and provides a code for representing and maintaining the multiple WM items-theta/gamma neural codes (Axmacher et al., 2010; Lisman and Jensen, 2013). The theta/gamma neural code hypothesis posits that the conserved memory items are registered via theta-nested gamma cycles in the sensory regions. Accordingly, the theta-gamma neural code coordinates communication between the different brain cortices during memory and sensory processes (Lisman and Jensen, 2013) and is specifically correlated to the WM requirements (Park et al., 2013).
Several studies have used intracranial EEG data in patients with epilepsy along with the behavioral outcomes to demonstrate the association between theta/gamma CFC across different brain regions and WM performance (Canolty et al., 2006; Rizzuto et al., 2006; Axmacher et al., 2010; Freunberger et al., 2011; Chuderski, 2016; Chai et al., 2018). The results of these studies can be summarized as follows: theta/gamma PAC distributed across the cortex and the strength of TGC increased with more cognitively demanding WM tasks. Moreover, a significant effect was observed when the gamma oscillations were detected in the trough of theta cycles (Canolty et al., 2006). In general, the theta troughs and peaks have different functions: WM retrieval occurs during the peaks, whereas WM encoding occurs during the troughs (Rizzuto et al., 2006). Axmacher et al. found that the WM maintenance is associated with the TGC in the hippocampus and the modulation of beta/gamma amplitude and theta activity were associated with a relatively large number of WM items (Axmacher et al., 2010). On the other hand, Chaieb et al. observed theta and beta/gamma phase-phase coupling in the hippocampus during the maintenance of multiple WM items (Sternberg WM task) in pre-surgical patients with epilepsy (Chaieb et al., 2015). All these studies are summarized in Table 1.
Cross-frequency coupling supports the organization of brain rhythms and is present during a range of cognitive functions. However, little is known about whether and how long-range CFC across the distant brain regions subserves WM. Here we report that theta–slow gamma coupling between the hippocampus and medial prefrontal cortex (mPFC) is augmented in a genetic mouse model of cognitive dysfunction. This increased CFC is observed specifically when the mice successfully perform a spatial WM task. In wild-type mice, increasing task difficulty by introducing a long delay or by optogenetically interfering with encoding, also increases the theta–gamma coupling during correct trials. Finally, the epochs of high hippocampal theta–prefrontal slow gamma coupling are associated with the increased synchronization of neurons within the mPFC. These findings suggest that the enhancement of theta–slow gamma coupling reflects a compensatory mechanism to maintain spatial WM performance in the setting of increased difficulty. The association between WM and theta-gamma PAC in the frontal, parietal, occipital, and posterior cortices has been reported in several studies using the different tasks (pictorial recognition tasks, delayed match-to-sample visual WM task, verbal delayed match to sample task, delayed figure recall, n-back task, etc.) to demonstrate the association of interest by using the EEG recordings (Griesmayr et al., 2010; Holz et al., 2010; Park et al., 2011; Friese et al., 2013; Köster et al., 2014; Lee and Yang, 2014; Graetz et al., 2019; Tseng et al., 2019; Fernández et al., 2021). The lowest level of TGC coupling was found in the patients with Alzheimer's dementia, followed by the patients with MCI and finally healthy controls (Goodman et al., 2018). All the studies showed modulations in the theta-gamma CFC related to correctly identified/retrieved items. Besides that, a decrease in the prefrontal and occipital alpha oscillatory activities was observed by Friese et al. (2013). The details of the studies are summarized in Table 1. The combined effect of WM training and transcranial direct current stimulation (tDCS) on the behavioral changes was investigated using the EEG features and cognitive task scores, and it was found that an increase in PAC between the prefrontal theta oscillations and temporo-parietal gamma oscillations was associated with the improvement in behavioral task scores, and more prominent improvement was found when the gamma waves coincided near the theta peaks (Jones et al., 2020).
The theta/gamma PAC strength is influenced by the oscillatory activities of other frequency bands; alpha amplitude influenced TGC in the two intracranial electroencephalography (iEEG) experiments that used different WM tasks (Leszczyński et al., 2015). Sauseng et al. found that repetitive TMS at alpha frequency suppressed distracting information and could influence the STM capacity (Sauseng et al., 2009). On the other hand, delta-alpha coupling influenced TGC and supported switching between the WM modes in the hippocampal region (Leszczyński et al., 2015).
Alpha-Gamma Coupling and WM
The default mode network (DMN) arises from the medial prefrontal cortex, the posterior cingulate cortex, and the inferior parietal cortex (Buckner et al., 2008). It is associated with episodic memory and self-referential thinking (Buckner et al., 2008; Knyazev et al., 2011; Weiler et al., 2014). The activity of DMN is higher during the resting state as compared with the task-performing state (Buckner et al., 2008). Indeed, an association between the DMN and WM networks has been suggested where DMN nodes could be activated during the memory phases (Hahn et al., 2007; Vilberg and Rugg, 2008; Daselaar et al., 2009). Moreover, the activity of DMN was mediated by alpha and beta oscillations, and the connectivity between some DMN parts is correlated to the alpha oscillatory activities (Hacker et al., 2017; Tang et al., 2017). A decrease in DMN functional connectivity and alpha power has been detected in patients with AD (Jeong, 2004; Zhang et al., 2009). In contrast to the young adults, old adults maintained synchronization in the resting state network and lacked the ability to synchronize the frontoparietal task-related network activities during the task performance (Pinal et al., 2015).
The electrophysiological studies have shown that when the content of WM changes from multiple items to distinct visual or spatial information, the oscillatory theta activities are replaced by alpha ones (Fries et al., 2001; Sauseng et al., 2005). Moreover, the studies examining the brain oscillations during the WM delay period found that the theta oscillatory activities occurred predominantly in the tasks that required sequential multiple WM item coding, while the alpha-oscillatory activities occurred in the tasks that required retention of visual or spatial information presented simultaneously (Roux and Uhlhaas, 2014). CFC between the parieto-occipital alpha activity and topographically distributed gamma activity is involved in prioritizing different visual representations in WM and deficits in the prefrontal cortex disrupt this process (Davoudi et al., 2021).
Alpha/gamma coupling has been demonstrated during visual WM maintenance in patients with epilepsy (Voytek et al., 2010). Park et al. used MEG data from healthy participants who were asked to recall the displayed images and found a reduction in the alpha power and an enhancement of alpha/gamma PAC during the process of recalling the images (Park et al., 2016). Moreover, alpha/beta and gamma power in the early visual cortex (in the dorsal and ventral visual streams) were modulated in response to the demands of the n-back task (Popov et al., 2018). The details of the studies are summarized in Table 1. Presumably, the theta and alpha activities are localized across different EEG recording sites. This assumption is supported by electrocorticography recordings, which showed that the alpha/gamma coupling was detected in the parietal–occipital cortices during the tasks requiring visual information processing, whereas theta/gamma PAC was predominantly observed in the frontotemporal regions during non-visual tasks (Voytek et al., 2010).
Other Types of CFC and WM
In principle, PAC can be generated in many ways; one source of the low-frequency band can be coupled to one or more sources of high-frequency bands. Thus, PAC is a general phenomenon and is not restricted to the theta/gamma frequencies. WM affects PAC in different ways; as WM could increase the PAC strength in some patterns and decrease it in others (Maris et al., 2011). CFC between the neural oscillations other than theta/gamma and alpha/gamma has been demonstrated during the WM maintenance as follows: (1) enhanced coupling between the theta/alpha phases and amplitude of beta has been demonstrated in temporal cortex during the visual delayed match-to-sample task (Daume et al., 2017a), (2) improved beta-theta PAC in the medial temporal lobe during the WM delay period (audio-visual delayed match to sample task) and enhanced phase synchronization between the medial temporal lobe and temporo-occipital areas in the beta band frequency range (Daume et al., 2017b), (3) improved the couplings between theta and alpha–gamma and between alpha and beta-gamma bands during the WM maintenance (delayed match-to-sample visual WM task) in the frontoparietal, dorsal, and visual areas (Siebenhühner et al., 2016), (4) increased alpha/theta phase synchrony was associated with the improved arithmetic task outcomes in the posterior and frontoparietal regions (Rodriguez-Larios and Alaerts, 2019), (5) the PAC (frontal theta phase and parieto-occipital alpha amplitude) strength decreased with increasing difficulty of both the correct and incorrect responses to the arithmetic tasks (Dimitriadis et al., 2016). The details of the studies are summarized in Table 1.
Frequency-Tuned Non-invasive Stimulation CFC and WM
The non-invasive brain stimulation methods are a potent way to study the causal relationship between brain activities and behavioral outcomes. These methods could causally modulate the behavior using electric or magnetic fields (Walsh and Cowey, 2000; Miniussi and Ruzzoli, 2013). Several studies have investigated the effect of frequency-tuned tACS and TMS on the WM performance with mixed results (Jaušovec et al., 2014; Hoy et al., 2015; Chander et al., 2016; Alekseichuk et al., 2017; Kuhnke et al., 2017; Sreeraj et al., 2017; Borghini et al., 2018; Wolinski et al., 2018; Jones et al., 2019; Beynel et al., 2020; Kehler et al., 2020; Papazova et al., 2020) and others. In the context of WM and CFC, a study was conducted to demonstrate the direct causality between the CFC and WM performance, the interaction between theta and gamma bands in the prefrontal cortex was externally modulated by CFC tACS protocol (Alekseichuk et al., 2016). The volunteers were instructed to perform the tasks during the stimulation and were assigned into three groups: sham stimulation (first group), continuous single-frequency theta stimulation (second group), and CFC tACS between theta and gamma frequencies (third group). Based on the behavioral and EEG data, the positive effect of continuous low-frequency entrainment on the WM performance was canceled out by the synchronization of high gamma bursts with the troughs of theta cycles (Alekseichuk et al., 2016). In contrast, a significant improvement in the WM performance was found when high oscillations gamma bursts (80–100-Hz frequency range) were embedded in the peaks of theta cycles (Alekseichuk et al., 2016).
Discussion
Overall, this review reports the association between the CFC and WM performance, almost all the studies showed a relationship between the CFC in different brain regions and WM performance, especially WM maintenance. Theta/gamma PAC is the most commonly reported CFC model in this context. The causal role of oscillations in memory processes can be realized by modulating endogenous oscillations and precisely determining the behavioral effects of such modulation. Entrainment of oscillations can be achieved by various methods and non-invasive brain stimulation is one of them. Non-invasive brain stimulation is a robust tool to establish a causal relationship between the neuronal oscillations at the mesoscopic scale and their role in cognition (Romei et al., 2011). The disadvantages of non-invasive brain stimulation methods are (1) high inter-subject variability (López-Alonso et al., 2014); (2) weak and inconsistent results of different studies (Ziemann and Siebner, 2015). (30) inconsistent long-lasting aftereffects (Veniero et al., 2015). Frequency-tuned non-invasive stimulation is a recent approach in neuroscience, in which the frequency of transcranially applied electromagnetic currents is matched to the ongoing oscillatory components with the aim of altering the behavior (Veniero et al., 2015; Albouy et al., 2018). WM can be manipulated/modulated by various approaches, and frequency-tuned non-invasive brain stimulation with an electric or magnetic field is one of them. Over the past two decades, there has been a long list of studies reporting the effects of tACS and TMS on WM (Jaušovec and Jaušovec, 2014; Hoy et al., 2015, 2016; Alekseichuk et al., 2016; Chander et al., 2016; Feurra et al., 2016; Kuhnke et al., 2017; Jones et al., 2019; Beynel et al., 2020; Kehler et al., 2020) and others. In general, theta-tACS improved the WM outcomes in the majority of studies, whereas the effect of gamma-tACS, alpha-tACS, etc., on WM appears inconsistent. This is partly due to the heterogeneity of the experimental setups and stimulation sites (frontal, parietal, and occipital) used in these studies. The CFC-tACS protocols have been investigated in several domains (learning, WM, and verbal-long term memory) (Alekseichuk et al., 2016; Lara et al., 2018; Turi et al., 2020; Riddle et al., 2021) and have recently gained popularity among the researchers. In principle, the peak-coupled tACS (gamma bursts nested into theta peaks) protocols mimic the endogenous theta-gamma CFC phase specificity needed for cognitive control (Smith et al., 2015). Thus, one might expect that the peak-coupled theta-gamma tACS would improve cognitive functions compared with the sham stimulation. In the context of WM, the causal role of CFC was demonstrated by Alekseichuk et al. (2016) in which exogenously applied theta and gamma CFC tACS were adjusted to the intrinsic continuous theta and repetitive gamma waves in the prefrontal cortices of healthy participants. Interestingly, theta-gamma tACS boosted working memory more than theta-tACS alone, and the effect was more pronounced when the gamma bursts (in the range of 80–100 Hz) were over the peak of the theta cycles (peak-coupled tACS). Alekseichuk et al. (2016) and Turi et al. (2020) investigated the effect of theta/gamma CFC tACS protocols aimed at the stimulating frontal and cingulate cortices on Go/NoGo monetary reward-based and punishment-based instrumental learning task outcomes. They used different theta/gamma CFC tACS protocols, in contrast to the results of (Alekseichuk et al., 2016) This study showed no consistent reinforcement effect of peak-coupled tACS, whereas trough-coupled tACS (gamma bursts were nested into theta troughs) impaired cognitive control (Alekseichuk et al., 2016; Turi et al., 2020). Furthermore, Riddle et al. found that the delta/beta peak-coupled tACS (beta oscillations in the prefrontal cortex were nested into the peak of delta cycles in the prefrontal cortex) and theta/gamma peak-coupled tACS (gamma bursts in the parietal-occipital were nested into the peak of theta cycles in the prefrontal cortex) modulated the cognitive task outcomes (Riddle et al., 2021). Additionally, Lara et al. examined the effect of theta/gamma CFC tACS during the verbal long-term memory encoding, the results of this study were consistent with those of Lara et al. (2018). Thus, the effect of CFC-tACS on the brain function outcomes could vary depending on the domain tested (WM, learning, long-term memory, etc.), brain regions activated, and experimental setups used. In general, the WM performance is sensitive to the phase and rhythm of externally applied tACS (Alekseichuk et al., 2016), as well as to the area of stimulation (brain cortices) and the experimental setups. In the context of WM and CFC, Alekseichuk et al. (2016) study opens the way for the promising research on frequency tuned non-invasive brain stimulation protocols to modulate the CFC activities instead of only modulating the narrow banded oscillatory activities. Furthermore, future studies should investigate the effect of CFC-tACS on WM in the healthy participants and in the patients with WM deficits as the two groups differ substantially in their susceptibility to tACS effect (Hoy et al., 2015, 2016; Dallmer-Zerbe et al., 2020).
In summary, the association between CFC and WM has been demonstrated in many studies. The effect of CFC tACS on the WM outcomes needs to be comprehensively studied using different brain regions involved in the WM processing and different experimental setups to achieve a consistent effect that is associated with the acceptable behavioral improvement and minimal tACS-induced side effects. Innovative approaches to validate the tACS effects in realistic settings are needed before CFC-tACS can modulate everyday cognitive performance and be used as a promising therapeutic tool.
Author Contributions
MA conducted the literature search and the summaries of previous published studies. MA and WA wrote the first and the final version of the manuscript. EK contributed to the progress of the manuscript and provided guidance with expert perspective. All authors contributed and approved the final version of the manuscript.
Conflict of Interest
The authors declare that the research was conducted in the absence of any commercial or financial relationships that could be construed as a potential conflict of interest.
Publisher's Note
All claims expressed in this article are solely those of the authors and do not necessarily represent those of their affiliated organizations, or those of the publisher, the editors and the reviewers. Any product that may be evaluated in this article, or claim that may be made by its manufacturer, is not guaranteed or endorsed by the publisher.
Abbreviations
WM, working memory; ADHD, attention deficit hyperactivity disorder; MCI, mild cognitive impairment; AD, Alzheimer's disease; CFC, cross frequency coupling; PAC, phase amplitude coupling; EEG, electroencephalography; MEG, magnetoencephalography; iEEG, intracranial electroencephalography; STM, short term memory; tACS, transcranial alternating current stimulation; tDCS, transcranial direct current stimulation; TMS, transcranial magnetic stimulation; TGC, theta gamma coupling; DMN, default mode network.
References
Aben, B., Stapert, S., and Blokland, A. (2012). About the distinction between working memory and short-term memory. Front. Psychol. 3:301. doi: 10.3389/fpsyg.2012.00301
Ackerman, P. L., Beier, M. E., and Boyle, M. O. (2005). Working memory and intelligence: the same or different constructs? Psychol. Bull. 131, 30–60. doi: 10.1037/0033-2909.131.1.30
Albouy, P., Baillet, S., and Zatorre, R. J. (2018). Driving working memory with frequency-tuned noninvasive brain stimulation. Ann. N. Y. Acad. Sci. 1423, 126–137. doi: 10.1111/nyas.13664
Alegre, M. (2016). Cross-frequency coupling in the pathophysiology of Parkinson's disease. Clin. Neurophysiol. 127:e29. doi: 10.1016/j.clinph.2015.11.087
Alekseichuk, I., Pabel, S. C., Antal, A., and Paulus, W. (2017). Intrahemispheric theta rhythm desynchronization impairs working memory. Restor. Neurol. Neurosci. 35, 147–158. doi: 10.3233/RNN-160714
Alekseichuk, I., Turi, Z., Amador de Lara, G., Antal, A., and Paulus, W. (2016). Spatial working memory in humans depends on theta and high gamma synchronization in the prefrontal cortex. Curr. Biol. 26, 1513–1521. doi: 10.1016/j.cub.2016.04.035
Allen, E. A., Liu, J., Kiehl, K. A., Gelernter, J., Pearlson, G. D., Perrone-Bizzozero, N. I., et al. (2011). Components of cross-frequency modulation in health and disease. Front. Syst. Neurosci. 5:59. doi: 10.3389/fnsys.2011.00059
Andersen, L. M., Jerbi, K., and Dalal, S. S. (2020). Can EEG and MEG detect signals from the human cerebellum? Neuroimage 215:116817. doi: 10.1016/j.neuroimage.2020.116817
Axmacher, N., Henseler, M. M., Jensen, O., Weinreich, I., Elger, C. E., and Fell, J. (2010). Cross-frequency coupling supports multi-item working memory in the human hippocampus. Proc. Natl. Acad. Sci. U.S.A. 107, 3228–3233. doi: 10.1073/pnas.0911531107
Baddeley, A. D., Bressi, S., Della Sala, S., Logie, R., and Spinnler, H. (1991). The decline of working memory in alzheimer's disease: a longitudinal study. Brain 114, 2521–2542. doi: 10.1093/brain/114.6.2521
Bahramisharif, A., Jensen, O., Jacobs, J., and Lisman, J. (2018). Serial representation of items during working memory maintenance at letter-selective cortical sites. PLoS Biol. 16:e2003805. doi: 10.1371/journal.pbio.2003805
Başar, E. (2013). Brain oscillations in neuropsychiatric disease. Dialogues Clin. Neurosci. 15, 291–300. doi: 10.31887/DCNS.2013.15.3/ebasar
Belluscio, M. A., Mizuseki, K., Schmidt, R., Kempter, R., and Buzsáki, G. (2012). Cross-frequency phase-phase coupling between theta and gamma oscillations in the hippocampus. J. Neurosci. 32, 423–435. doi: 10.1523/JNEUROSCI.4122-11.2012
Beynel, L., Davis, S. W., Crowell, C. A., Dannhauer, M., Lim, W., Palmer, H., et al. (2020). Site-specific effects of online rTMS during a working memory task in healthy older adults. Brain Sci. 10:255. doi: 10.3390/BRAINSCI10050255
Biel, A. L., Minarik, T., and Sauseng, P. (2021). EEG cross-frequency phase synchronization as an index of memory matching in visual search. Neuroimage 235:117971. doi: 10.1016/j.neuroimage.2021.117971
Borghini, G., Candini, M., Filannino, C., Hussain, M., Walsh, V., Romei, V., et al. (2018). Alpha oscillations are causally linked to inhibitory abilities in ageing. J. Neurosci. 38, 4418–4429. doi: 10.1523/JNEUROSCI.1285-17.2018
Botvinick, M., and Watanabe, T. (2007). From numerosity to ordinal rank: A gain-field model of serial order representation in cortical working memory. J. Neurosci. 27, 8636–8642. doi: 10.1523/JNEUROSCI.2110-07.2007
Brooks, H., Goodman, M. S., Bowie, C. R., Zomorrodi, R., Blumberger, D. M., Butters, M. A., et al. (2020). Theta–gamma coupling and ordering information: a stable brain–behavior relationship across cognitive tasks and clinical conditions. Neuropsychopharmacology 45, 2038–2047. doi: 10.1038/s41386-020-0759-z
Bruns, A., and Eckhorn, R. (2004). Task-related coupling from high- to low-frequency signals among visual cortical areas in human subdural recordings. Int. J. Psychophysiol. 51, 97–116. doi: 10.1016/j.ijpsycho.2003.07.001
Buckner, R. L., Andrews-Hanna, J. R., and Schacter, D. L. (2008). The brain's default network: anatomy, function, and relevance to disease. Ann. N. Y. Acad. Sci. 1124, 1–38. doi: 10.1196/annals.1440.011
Buschman, T. J., and Miller, E. K. (2007). Top-down versus bottom-up control of attention in the prefrontal and posterior parietal cortices. Science 315, 1860–1864. doi: 10.1126/science.1138071
Buzsáki, G., and Draguhn, A. (2004). Neuronal olscillations in cortical networks. Science 304, 1926–1929. doi: 10.1126/science.1099745
Calderone, D. J., Lakatos, P., Butler, P. D., and Castellanos, F. X. (2014). Entrainment of neural oscillations as a modifiable substrate of attention. Trends Cogn. Sci. 18, 300–309. doi: 10.1016/j.tics.2014.02.005
Canolty, R. T., Edwards, E., Dalal, S. S., Soltani, M., Nagarajan, S. S., Kirsch, H. E., et al. (2006). High gamma power is phase-locked to theta oscillations in human neocortex. Science 313, 1626–1628. doi: 10.1126/science.1128115
Canolty, R. T., and Knight, R. T. (2010). The functional role of cross-frequency coupling. Trends Cogn. Sci. 14, 506–515. doi: 10.1016/j.tics.2010.09.001
Chai, W. J., Abd Hamid, A. I., and Abdullah, J. M. (2018). Working memory from the psychological and neurosciences perspectives: a review. Front. Psychol. 9, 401. doi: 10.3389/fpsyg.2018.00401
Chaieb, L., Leszczynski, M., Axmacher, N., Höhne, M., Elger, C. E., and Fell, J. (2015). Theta-gamma phase-phase coupling during working memory maintenance in the human hippocampus. Cogn. Neurosci. 6, 149–157. doi: 10.1080/17588928.2015.1058254
Chander, B. S., Witkowski, M., Braun, C., Robinson, S. E., Born, J., Cohen, L. G., et al. (2016). tACS phase locking of frontal midline theta oscillations disrupts working memory performance. Front. Cell. Neurosci. 10:120. doi: 10.3389/fncel.2016.00120
Chuderski, A. (2016). Fluid intelligence and the cross-frequency coupling of neuronal oscillations. Span. J. Psychol. 19:E91. doi: 10.1017/sjp.2016.86
Cohen, M. X. (2014). A neural microcircuit for cognitive conflict detection and signaling. Trends Neurosci. 37, 480–490. doi: 10.1016/j.tins.2014.06.004
Colgin, L. L. (2015). Theta-gamma coupling in the entorhinal-hippocampal system. Curr. Opin. Neurobiol. 31, 45–50. doi: 10.1016/j.conb.2014.08.001
Colom, R., Shih, P. C., Flores-Mendoza, C., and Quiroga, M. Á. (2006). The real relationship between short-term memory and working memory. Memory 14, 804–813. doi: 10.1080/09658210600680020
Constantinidis, C., and Klingberg, T. (2016). The neuroscience of working memory capacity and training. Nat. Rev. Neurosci. 17, 438–449. doi: 10.1038/nrn.2016.43
Conway, A. R. A., Kane, M. J., Bunting, M. F., Hambrick, D. Z., Wilhelm, O., and Engle, R. W. (2005). Working memory span tasks: A methodological review and user's guide. Psychon. Bull. Rev. 12, 769–786. doi: 10.3758/BF03196772
Cowan, N. (2001). The magical number 4 in short-term memory: a reconsideration of mental storage capacity. Behav. Brain Sci. 24, 87–114. doi: 10.1017/S0140525X01003922
Cowan, N. (2014). Working memory underpins cognitive development, learning, and education. Educ. Psychol. Rev. 26, 197–223. doi: 10.1007/s10648-013-9246-y
Cowan, N. (2017). The many faces of working memory and short-term storage. Psychon. Bull. Rev. 24, 1158–1170. doi: 10.3758/s13423-016-1191-6
Dallmer-Zerbe, I., Popp, F., Lam, A. P., Philipsen, A., and Herrmann, C. S. (2020). Transcranial alternating current stimulation (tACS) as a tool to modulate p300 amplitude in attention deficit hyperactivity disorder (ADHD): preliminary findings. Brain Topogr. 33, 191–207. doi: 10.1007/s10548-020-00752-x
Daselaar, S. M., Prince, S. E., Dennis, N. A., Hayes, S. M., Kim, H., and Cabeza, R. (2009). Posterior midline and ventral parietal activity is associated with retrieval success and encoding failure. Front. Hum. Neurosci. 3:2009. doi: 10.3389/neuro.09.013.2009
Daume, J., Graetz, S., Gruber, T., Engel, A. K., and Friese, U. (2017b). Cognitive control during audiovisual working memory engages frontotemporal theta-band interactions. Sci. Rep. 7:12585. doi: 10.1038/s41598-017-12511-3
Daume, J., Gruber, T., Engel, A. K., and Friese, U. (2017a). Phase-amplitude coupling and long-range phase synchronization reveal frontotemporal interactions during visual working memory. J. Neurosci. 37, 313–322. doi: 10.1523/JNEUROSCI.2130-16.2017
Davoudi, S., Dezfouli, M. P., Knight, R. T., Daliri, M. R., and Johnson, E. L. (2021). Prefrontal lesions disrupt posterior alpha–gamma coordination of visual working memory representations. J. Cogn. Neurosci. 33, 1798–1810. doi: 10.1162/jocn_a_01715
De Hemptinne, C., Ryapolova-Webb, E. S., Air, E. L., Garcia, P. A., Miller, K. J., Ojemann, J. G., et al. (2013). Exaggerated phase-amplitude coupling in the primary motor cortex in Parkinson disease. Proc. Natl. Acad. Sci. U.S.A. 110, 4780–4785. doi: 10.1073/pnas.1214546110
Demiralp, T., Bayraktaroglu, Z., Lenz, D., Junge, S., Busch, N. A., Maess, B., et al. (2007). Gamma amplitudes are coupled to theta phase in human EEG during visual perception. Int. J. Psychophysiol. 64, 24–30. doi: 10.1016/j.ijpsycho.2006.07.005
DeStefano, D., and LeFevre, J. (2010). The role of working memory in mental arithmetic. Cogn. Psychol. 16, 353–386. doi: 10.1080/09541440244000328
Dimitriadis, S. I., Sun, Y., Thakor, N. V., and Bezerianos, A. (2016). Causal Interactions between frontalθ – parieto-occipitalα2 predict performance on a mental arithmetic task. Front. Hum. Neurosci. 10:454. doi: 10.3389/fnhum.2016.00454
Düzel, E., Penny, W. D., and Burgess, N. (2010). Brain oscillations and memory. Curr. Opin. Neurobiol. 20, 143–149. doi: 10.1016/j.conb.2010.01.004
Edin, F., Klingberg, T., Johansson, P., McNab, F., Tegnér, J., and Compte, A. (2009). Mechanism for top-down control of working memory capacity. Proc. Natl. Acad. Sci. U.S.A. 106, 6802–6807. doi: 10.1073/pnas.0901894106
Egner, T., and Gruzelier, J. H. (2004). EEG Biofeedback of low beta band components: frequency-specific effects on variables of attention and event-related brain potentials. Clin. Neurophysiol. 115, 131–139. doi: 10.1016/S1388-2457(03)00353-5
Engel, A. K., Fries, P., and Singer, W. (2001). Dynamic predictions: Oscillations and synchrony in top–down processing. Nat. Rev. Neurosci. 2, 704–716. doi: 10.1038/35094565
Engle, R. W., Tuholski, S. W., Laughlin, J. E., and Conway, A. R. A. (1999). Working memory, short-term memory, and general fluid intelligence: a latent-variable approach. J. Exp. Psychol. Gen. 128, 309–331. doi: 10.1037/0096-3445.128.3.309
Fernández, A., Pinal, D., Díaz, F., and Zurrón, M. (2021). Working memory load modulates oscillatory activity and the distribution of fast frequencies across frontal theta phase during working memory maintenance. Neurobiol. Learn. Mem. 183, 107476. doi: 10.1016/j.nlm.2021.107476
Feurra, M., Galli, G., Pavone, E. F., Rossi, A., and Rossi, S. (2016). Frequency-specific insight into short-term memory capacity. J. Neurophysiol. 116, 153–158. doi: 10.1152/jn.01080.2015
Fougnie, D., Zughni, S., Godwin, D., and Marois, R. (2015). Working memory storage is intrinsically domain specific. J. Exp. Psychol. Gen. 144, 30–47. doi: 10.1037/a0038211
Freunberger, R., Werkle-Bergner, M., Griesmayr, B., Lindenberger, U., and Klimesch, W. (2011). Brain oscillatory correlates of working memory constraints. Brain Res. 1375, 93–102. doi: 10.1016/j.brainres.2010.12.048
Fries, P. (2009). Neuronal gamma-band synchronization as a fundamental process in cortical computation. Annu. Rev. Neurosci. 32, 209–224. doi: 10.1146/annurev.neuro.051508.135603
Fries, P. (2015). Rhythms for cognition: communication through coherence. Neuron 88, 220–235. doi: 10.1016/j.neuron.2015.09.034
Fries, P., Nikolić, D., and Singer, W. (2007). The gamma cycle. Trends Neurosci. 30, 309–316. doi: 10.1016/j.tins.2007.05.005
Fries, P., Reynolds, J. H., Rorie, A. E., and Desimone, R. (2001). Modulation of oscillatory neuronal synchronization by selective visual attention. Science 291, 1560–1563. doi: 10.1126/science.1055465
Friese, U., Köster, M., Hassler, U., Martens, U., Trujillo-Barreto, N., and Gruber, T. (2013). Successful memory encoding is associated with increased cross-frequency coupling between frontal theta and posterior gamma oscillations in human scalp-recorded EEG. Neuroimage 66, 642–647. doi: 10.1016/j.neuroimage.2012.11.002
Gagnon, L. G., and Belleville, S. (2011). Working memory in mild cognitive impairment and Alzheimer's disease: contribution of forgetting and predictive value of complex span tasks. Neuropsychology 25, 226–236. doi: 10.1037/a0020919
Gathercole, S., Brown, L., and Pickering, S. (2003). Working memory assessments at school entry as longitudinal predictors of National Curriculum attainment levels. Educ. Child Psychol. 20, 109–122. Available at: https://research-information.bris.ac.uk/en/publications/working-memory-assessments-at-school-entry-as-longitudinal-predic (accessed February 5, 2021).
Gathercole, S. E., and Alloway, T. P. (2006). Practitioner review: Short-term and working memory impairments in neurodevelopmental disorders: diagnosis and remedial support. J. Child Psychol. Psychiatry Allied Discip. 47, 4–15. doi: 10.1111/j.1469-7610.2005.01446.x
Gignac, G. E. (2015). The magical numbers 7 and 4 are resistant to the flynn effect: no evidence for increases in forward or backward recall across 85 years of data. Intelligence 48, 85–95. doi: 10.1016/j.intell.2014.11.001
Goodman, M. S., Kumar, S., Zomorrodi, R., Ghazala, Z., Cheam, A. S. M., Barr, M. S., et al. (2018). Theta-Gamma coupling and working memory in Alzheimer's dementia and mild cognitive impairment. Front. Aging Neurosci. 10:101. doi: 10.3389/fnagi.2018.00101
Graetz, S., Daume, J., Friese, U., and Gruber, T. (2019). Alterations in oscillatory cortical activity indicate changes in mnemonic processing during continuous item recognition. Exp. Brain Res. 237, 573–583. doi: 10.1007/s00221-018-5439-4
Griesmayr, B., Gruber, W. R., Klimesch, W., and Sauseng, P. (2010). Human frontal midline theta and its synchronization to gamma during a verbal delayed match to sample task. Neurobiol. Learn. Mem. 93, 208–215. doi: 10.1016/j.nlm.2009.09.013
Hacker, C. D., Snyder, A. Z., Pahwa, M., Corbetta, M., and Leuthardt, E. C. (2017). Frequency-specific electrophysiologic correlates of resting state fMRI networks. Neuroimage 149, 446–457. doi: 10.1016/j.neuroimage.2017.01.054
Hahn, B., Ross, T. J., and Stein, E. A. (2007). Cingulate activation increases dynamically with response speed under stimulus unpredictability. Cereb. Cortex 17, 1664–1671. doi: 10.1093/cercor/bhl075
Hanslmayr, S., Axmacher, N., and Inman, C. S. (2019). Modulating human memory via entrainment of brain oscillations. Trends Neurosci. 42, 485–499. doi: 10.1016/j.tins.2019.04.004
Helfrich, R. F., Herrmann, C. S., Engel, A. K., and Schneider, T. R. (2016). Different coupling modes mediate cortical cross-frequency interactions. Neuroimage 140, 76–82. doi: 10.1016/j.neuroimage.2015.11.035
Herman, P. A., Lundqvist, M., and Lansner, A. (2013). Nested theta to gamma oscillations and precise spatiotemporal firing during memory retrieval in a simulated attractor network. Brain Res. 1536, 68–87. doi: 10.1016/j.brainres.2013.08.002
Herrmann, C. S., Strüber, D., Helfrich, R. F., and Engel, A. K. (2016). EEG oscillations: from correlation to causality. Int. J. Psychophysiol. 103, 12–21. doi: 10.1016/j.ijpsycho.2015.02.003
Hiltunen, T., Kantola, J., Elseoud, A. A., Lepola, P., Suominen, K., Starck, T., et al. (2014). Infra-slow EEG fluctuations are correlated with resting-state network dynamics in fMRI. J. Neurosci. 34, 356–362. doi: 10.1523/JNEUROSCI.0276-13.2014
Holz, E. M., Glennon, M., Prendergast, K., and Sauseng, P. (2010). Theta-gamma phase synchronization during memory matching in visual working memory. Neuroimage 52, 326–335. doi: 10.1016/j.neuroimage.2010.04.003
Hoy, K. E., Bailey, N., Arnold, S., Windsor, K., John, J., Daskalakis, Z. J., et al. (2015). The effect of γ-tACS on working memory performance in healthy controls. Brain Cogn. 101, 51–56. doi: 10.1016/j.bandc.2015.11.002
Hoy, K. E., Whitty, D., Bailey, N., and Fitzgerald, P. B. (2016). Preliminary investigation of the effects of γ -tACS on working memory in schizophrenia. J. Neural Transm. 123, 1205–1212. doi: 10.1007/s00702-016-1554-1
Hsieh, L. T., and Ranganath, C. (2014). Frontal midline theta oscillations during working memory maintenance and episodic encoding and retrieval. Neuroimage 85, 721–729. doi: 10.1016/j.neuroimage.2013.08.003
Hulme, C., and Melby-Lervåg, M. (2012). Current evidence does not support the claims made for CogMed working memory training. J. Appl. Res. Mem. Cogn. 1, 197–200. doi: 10.1016/j.jarmac.2012.06.006
Jaeggi, S. M., Buschkuehl, M., Jonides, J., and Perrig, W. J. (2008). Improving fluid intelligence with training on working memory. Proc. Natl. Acad. Sci. U.S.A. 105, 6829–6833. doi: 10.1073/pnas.0801268105
Jaušovec, N., and Jaušovec, K. (2014). Increasing working memory capacity with theta transcranial alternating current stimulation (tACS). Biol. Psychol. 96, 42–47. doi: 10.1016/j.biopsycho.2013.11.006
Jaušovec, N., Jaušovec, K., and Pahor, A. (2014). The influence of theta transcranial alternating current stimulation (tACS) on working memory storage and processing functions. Acta Psychol. 146, 1–6. doi: 10.1016/j.actpsy.2013.11.011
Jensen, O., and Colgin, L. L. (2007). Cross-frequency coupling between neuronal oscillations. Trends Cogn. Sci. 11, 267–269. doi: 10.1016/j.tics.2007.05.003
Jensen, O., and Lisman, J. E. (1996). Novel lists of 7±2 known items can be reliably stored in an oscillatory short-term memory network: interaction with long-term memory. Learn. Mem. 3, 257–263. doi: 10.1101/lm.3.2-3.257
Jensen, O., Spaak, E., and Zumer, J. M. (2014). “Human brain oscillations: From physiological mechanisms to analysis and cognition,” in Magnetoencephalography: From Signals to Dynamic Cortical Networks, eds S. Supek and C. J. Aine (Berlin: Springer-Verlag), 359–403. doi: 10.1007/978-3-642-33045-2_17
Jeong, J. (2004). EEG dynamics in patients with Alzheimer's disease. Clin. Neurophysiol. 115, 1490–1505. doi: 10.1016/j.clinph.2004.01.001
Jirsa, V., and Müller, V. (2013). Cross-frequency coupling in real and virtual brain networks. Front. Comput. Neurosci. 7:78. doi: 10.3389/fncom.2013.00078
Jones, K. T., Arciniega, H., and Berryhill, M. E. (2019). Replacing tDCS with theta tACS provides selective, but not general WM benefits. Brain Res. 1720:146324. doi: 10.1016/j.brainres.2019.146324
Jones, K. T., Johnson, E. L., and Berryhill, M. E. (2020). Frontoparietal theta-gamma interactions track working memory enhancement with training and tDCS. Neuroimage 211:116615. doi: 10.1016/j.neuroimage.2020.116615
Kamiński, J., Brzezicka, A., and Wróbel, A. (2011). Short-term memory capacity (7±2) predicted by theta to gamma cycle length ratio. Neurobiol. Learn. Mem. 95, 19–23. doi: 10.1016/j.nlm.2010.10.001
Kane, M. J., Brown, L. H., McVay, J. C., Silvia, P. J., Myin-Germeys, I., and Kwapil, T. R. (2007). For whom the mind wanders, and when: an experience-sampling study of working memory and executive control in daily life. Psychol. Sci. 18, 614–621. doi: 10.1111/j.1467-9280.2007.01948.x
Kane, M. J., Tuholski, S. W., Hambrick, D. Z., Wilhelm, O., Payne, T. W., and Engle, R. W. (2004). The generality of working memory capacity: a latent-variable approach to verbal and visuospatial memory span and reasoning. J. Exp. Psychol. Gen. 133, 189–217. doi: 10.1037/0096-3445.133.2.189
Kehler, L., Francisco, C. O., Uehara, M. A., and Moussavi, Z. (2020). “The effect of transcranial alternating current stimulation (tACS) on cognitive function in older adults with dementia,” in Proceedings of the Annual International Conference of the IEEE Engineering in Medicine and Biology Society, EMBS (Institute of Electrical and Electronics Engineers Inc.), 3649–3653. doi: 10.1109/EMBC44109.2020.9175903
Klimesch, W. (1999). EEG alpha and theta oscillations reflect cognitive and memory performance: a review and analysis. Brain Res. Rev. 29, 169–195. doi: 10.1016/S0165-0173(98)00056-3
Klimesch, W. (2018). The frequency architecture of brain and brain body oscillations: an analysis. Eur. J. Neurosci. 48, 2431–2453. doi: 10.1111/ejn.14192
Klingberg, T. (2010). Training and plasticity of working memory. Trends Cogn. Sci. 14, 317–324. doi: 10.1016/j.tics.2010.05.002
Klingberg, T., Forssberg, H., and Westerberg, H. (2002). Training of working memory in children with ADHD. J. Clin. Exp. Neuropsychol. 24, 781–791. doi: 10.1076/jcen.24.6.781.8395
Knyazev, G. G., Slobodskoj-Plusnin, J. Y., Bocharov, A. V., and Pylkova, L. V. (2011). The default mode network and EEG alpha oscillations: an independent component analysis. Brain Res. 1402, 67–79. doi: 10.1016/j.brainres.2011.05.052
Köster, M., Friese, U., Schöne, B., Trujillo-Barreto, N., and Gruber, T. (2014). Theta-gamma coupling during episodic retrieval in the human EEG. Brain Res. 1577, 57–68. doi: 10.1016/j.brainres.2014.06.028
Kuhnke, P., Meyer, L., Friederici, A. D., and Hartwigsen, G. (2017). Left posterior inferior frontal gyrus is causally involved in reordering during sentence processing. Neuroimage 148, 254–263. doi: 10.1016/j.neuroimage.2017.01.013
Lakatos, P., Shah, A. S., Knuth, K. H., Ulbert, I., Karmos, G., and Schroeder, C. E. (2005). An oscillatory hierarchy controlling neuronal excitability and stimulus processing in the auditory cortex. J. Neurophysiol. 94, 1904–1911. doi: 10.1152/jn.00263.2005
Lara, G. A., Alekseichuk, I., Turi, Z., Lehr, A., Antal, A., and Paulus, W. (2018). Perturbation of theta-gamma coupling at the temporal lobe hinders verbal declarative memory. Brain Stimul. 11, 509–517. doi: 10.1016/j.brs.2017.12.007
Lee, Y. Y., and Yang, C. Y. (2014). Utilizing the extent of theta–gamma synchronization to estimate visuospatial memory ability. Australas. Phys. Eng. Sci. Med. 37, 665–672. doi: 10.1007/s13246-014-0299-0
Leszczyński, M., Fell, J., and Axmacher, N. (2015). Rhythmic working memory activation in the human hippocampus. Cell Rep. 13, 1272–1282. doi: 10.1016/j.celrep.2015.09.081
Lisman, J. E., and Idiart, M. A. P. (1995). Storage of 7 ± 2 short-term memories in oscillatory subcycles. Science 267, 1512–1515. doi: 10.1126/science.7878473
Lisman, J. E., and Jensen, O. (2013). The theta-gamma neural code. Neuron 77, 1002–1016. doi: 10.1016/j.neuron.2013.03.007
López-Alonso, V., Cheeran, B., Río-Rodríguez, D., and Fernández-Del-Olmo, M. (2014). Inter-individual variability in response to non-invasive brain stimulation paradigms. Brain Stimul. 7, 372–380. doi: 10.1016/j.brs.2014.02.004
Luo, W., and Guan, J.-S. (2018). Do brain oscillations orchestrate memory? Brain Sci. Adv. 4, 16–33. doi: 10.26599/BSA.2018.9050008
Lynn, P. A., and Sponheim, S. R. (2016). Disturbed theta and gamma coupling as a potential mechanism for visuospatial working memory dysfunction in people with schizophrenia. Neuropsychiatr. Electrophysiol. 2, 1–30. doi: 10.1186/s40810-016-0022-3
MacOveanu, J., Klingberg, T., and Tegnér, J. (2007). Neuronal firing rates account for distractor effects on mnemonic accuracy in a visuo-spatial working memory task. Biol. Cybern. 96, 407–419. doi: 10.1007/s00422-006-0139-8
Malenínská, K., Rudolfová, V., Šulcová, K., Koudelka, V., Brunovský, M., Horáček, J., et al. (2021). Is short-term memory capacity (7±2) really predicted by theta to gamma cycle length ratio? Behav. Brain Res. 414:113465. doi: 10.1016/j.bbr.2021.113465
Mann, E. O., and Paulsen, O. (2005). Mechanisms underlying gamma ('40 Hz') network oscillations in the hippocampus - a mini-review. Prog. Biophys. Mol. Biol. 87, 67–76. doi: 10.1016/j.pbiomolbio.2004.06.004
Maris, E., van Vugt, M., and Kahana, M. (2011). Spatially distributed patterns of oscillatory coupling between high-frequency amplitudes and low-frequency phases in human iEEG. Neuroimage 54, 836–850. doi: 10.1016/j.neuroimage.2010.09.029
Marzetti, L., Basti, A., Chella, F., D'Andrea, A., Syrjälä, J., and Pizzella, V. (2019). Brain functional connectivity through phase coupling of neuronal oscillations: a perspective from magnetoencephalography. Front. Neurosci. 13:964. doi: 10.3389/fnins.2019.00964
Merker, B. (2013). Cortical gamma oscillations: the functional key is activation, not cognition. Neurosci. Biobehav. Rev. 37, 401–417. doi: 10.1016/j.neubiorev.2013.01.013
Miniussi, C., and Ruzzoli, M. (2013). Transcranial stimulation and cognition. Handb. Clin. Neurol. 116, 739–750. doi: 10.1016/B978-0-444-53497-2.00056-5
Mizuhara, H., and Yamaguchi, Y. (2011). Neuronal ensemble for visual working memory via interplay of slow and fast oscillations. Eur. J. Neurosci. 33, 1925–1934. doi: 10.1111/j.1460-9568.2011.07681.x
Mormann, F., Fell, J., Axmacher, N., Weber, B., Lehnertz, K., Elger, C. E., et al. (2005). Phase/amplitude reset and theta–gamma interaction in the human medial temporal lobe during a continuous word recognition memory task. Hippocampus 15, 890–900. doi: 10.1002/hipo.20117
Nadel, L., and Hardt, O. (2011). Update on memory systems and processes. Neuropsychopharmacology 36, 251–273. doi: 10.1038/npp.2010.169
Niebur, E. (2002). Electrophysiological correlates of synchronous neural activity and attention: a short review. BioSystems 67, 157–166. doi: 10.1016/S0303-2647(02)00102-8
Papazova, I., Strube, W., Hoffmann, L., Schwippel, T., Padberg, F., Palm, U., et al. (2020). T54 effects of gamma transcranial alternating current stimulation to the left dorsolateral prefrontal cortex on working memory in schizophrenia patients. Schizophr. Bull. 46, S251–S252. doi: 10.1093/schbul/sbaa029.614
Park, H., Lee, D. S., Kang, E., Kang, H., Hahm, J., Kim, J. S., et al. (2016). Formation of visual memories controlled by gamma power phase-locked to alpha oscillations. Sci. Rep. 6:28092. doi: 10.1038/srep28092
Park, J. Y., Jhung, K., Lee, J., and An, S. K. (2013). Theta-gamma coupling during a working memory task as compared to a simple vigilance task. Neurosci. Lett. 532, 39–43. doi: 10.1016/j.neulet.2012.10.061
Park, J. Y., Lee, Y. R., and Lee, J. (2011). The relationship between theta-gamma coupling and spatial memory ability in older adults. Neurosci. Lett. 498, 37–41. doi: 10.1016/j.neulet.2011.04.056
Persuh, M., Larock, E., and Berger, J. (2018). Working memory and consciousness: the current state of play. Front. Hum. Neurosci. 12:78. doi: 10.3389/fnhum.2018.00078
Pinal, D., Zurrón, M., Díaz, F., and Sauseng, P. (2015). Stuck in default mode: inefficient cross-frequency synchronization may lead to age-related short-term memory decline. Neurobiol. Aging 36, 1611–1618. doi: 10.1016/j.neurobiolaging.2015.01.009
Popov, T., Jensen, O., and Schoffelen, J. M. (2018). Dorsal and ventral cortices are coupled by cross-frequency interactions during working memory. Neuroimage 178, 277–286. doi: 10.1016/j.neuroimage.2018.05.054
Rajji, T. K., Zomorrodi, R., Barr, M. S., Blumberger, D. M., Mulsant, B. H., and Daskalakis, Z. J. (2017). Ordering information in working memory and modulation of gamma by theta oscillations in humans. Cereb. Cortex 27, 1482–1490. doi: 10.1093/cercor/bhv326
Riddle, J., McFerren, A., and Frohlich, F. (2021). Causal role of cross-frequency coupling in distinct components of cognitive control. Prog. Neurobiol. 202:102033. doi: 10.1016/j.pneurobio.2021.102033
Rizzuto, D. S., Madsen, J. R., Bromfield, E. B., Schulze-Bonhage, A., and Kahana, M. J. (2006). Human neocortical oscillations exhibit theta phase differences between encoding and retrieval. Neuroimage 31, 1352–1358. doi: 10.1016/j.neuroimage.2006.01.009
Roberts, B. M., Hsieh, L. T., and Ranganath, C. (2013). Oscillatory activity during maintenance of spatial and temporal information in working memory. Neuropsychologia 51, 349–357. doi: 10.1016/j.neuropsychologia.2012.10.009
Rodriguez-Larios, J., and Alaerts, K. (2019). Tracking transient changes in the neural frequency architecture: harmonic relationships between theta and alpha peaks facilitate cognitive performance. J. Neurosci. 39, 6291–6298. doi: 10.1523/JNEUROSCI.2919-18.2019
Romei, V., Driver, J., Schyns, P. G., and Thut, G. (2011). Rhythmic TMS over parietal cortex links distinct brain frequencies to global versus local visual processing. Curr. Biol. 21, 334–337. doi: 10.1016/j.cub.2011.01.035
Roux, F., and Uhlhaas, P. J. (2014). Working memory and neural oscillations: Alpha-gamma versus theta-gamma codes for distinct WM information? Trends Cogn. Sci. 18, 16–25. doi: 10.1016/j.tics.2013.10.010
Salimpour, Y., and Anderson, W. S. (2019). Cross-Frequency coupling based neuromodulation for treating neurological disorders. Front. Neurosci. 13:125. doi: 10.3389/fnins.2019.00125
Sauseng, P., Griesmayr, B., Freunberger, R., and Klimesch, W. (2010). Control mechanisms in working memory: a possible function of EEG theta oscillations. Neurosci. Biobehav. Rev. 34, 1015–1022. doi: 10.1016/j.neubiorev.2009.12.006
Sauseng, P., Klimesch, W., Doppelmayr, M., Pecherstorfer, T., Freunberger, R., and Hanslmayr, S. (2005). EEG alpha synchronization and functional coupling during top-down processing in a working memory task. Hum. Brain Mapp. 26, 148–155. doi: 10.1002/hbm.20150
Sauseng, P., Klimesch, W., Heise, K. F., Gruber, W. R., Holz, E., Karim, A. A., et al. (2009). Brain oscillatory substrates of visual short-term memory capacity. Curr. Biol. 19, 1846–1852. doi: 10.1016/j.cub.2009.08.062
Sauseng, P., Peylo, C., Biel, A. L., Friedrich, E. V. C., and Romberg-Taylor, C. (2019). Does cross-frequency phase coupling of oscillatory brain activity contribute to a better understanding of visual working memory? Br. J. Psychol. 110, 245–255. doi: 10.1111/bjop.12340
Schack, B., Vath, N., Petsche, H., Geissler, H. G., and Möller, E. (2002). Phase-coupling of theta-gamma EEG rhythms during short-term memory processing. Int. J. Psychophysiol. 44, 143–163. doi: 10.1016/S0167-8760(01)00199-4
Sederberg, P. B., Kahana, M. J., Howard, M. W., Donner, E. J., and Madsen, J. R. (2003). Theta and gamma oscillations during encoding predict subsequent recall. J. Neurosci. 23, 10809–10814. doi: 10.1523/JNEUROSCI.23-34-10809.2003
Siebenhühner, F., Wang, S. H., Arnulfo, G., Lampinen, A., Nobili, L., Palva, J. M., et al. (2020). Genuine cross-frequency coupling networks in human resting-state electrophysiological recordings. PLoS Biol. 18:e3000685. doi: 10.1371/journal.pbio.3000685
Siebenhühner, F., Wang, S. H., Palva, J. M., and Palva, S. (2016). Cross-frequency synchronization connects networks of fast and slow oscillations during visual working memory maintenance. Elife 5:e36. doi: 10.7554/eLife.13451.036
Siegel, M., Donner, T. H., and Engel, A. K. (2012). Spectral fingerprints of large-scale neuronal interactions. Nat. Rev. Neurosci. 13, 121–134. doi: 10.1038/nrn3137
Siems, M., and Siegel, M. (2020). Dissociated neuronal phase- and amplitude-coupling patterns in the human brain. Neuroimage 209:116538. doi: 10.1016/j.neuroimage.2020.116538
Smith, E. H., Banks, G. P., Mikell, C. B., Cash, S. S., Patel, S. R., Eskandar, E. N., et al. (2015). Frequency-Dependent representation of reinforcement-related information in the human medial and lateral prefrontal cortex. J. Neurosci. 35:15827. doi: 10.1523/JNEUROSCI.1864-15.2015
Sotero, R. C. (2016). Topology, cross-frequency, and same-frequency band interactions shape the generation of phase-amplitude coupling in a neural mass model of a cortical column. PLoS Comput. Biol. 12:1005180. doi: 10.1371/journal.pcbi.1005180
Sreeraj, V. S., Shanbhag, V., Nawani, H., Shivakumar, V., Damodharan, D., Bose, A., et al. (2017). Feasibility of online neuromodulation using transcranial alternating current stimulation in schizophrenia. Indian J. Psychol. Med. 39:92. doi: 10.4103/0253-7176.198937
Tang, W., Liu, H., Douw, L., Kramer, M. A., Eden, U. T., Hämäläinen, M. S., et al. (2017). Dynamic connectivity modulates local activity in the core regions of the default-mode network. Proc. Natl. Acad. Sci. U.S.A. 114, 9713–9718. doi: 10.1073/pnas.1702027114
Thut, G., and Miniussi, C. (2009). New insights into rhythmic brain activity from TMS-EEG studies. Trends Cogn. Sci. 13, 182–189. doi: 10.1016/j.tics.2009.01.004
Tseng, Y.-L., Liu, H.-H., Liou, M., Tsai, A. C., Chien, V. S. C., Shyu, S.-T., et al. (2019). Lingering sound: event-related phase-amplitude coupling and phase-locking in fronto-temporo-parietal functional networks during memory retrieval of music melodies. Front. Hum. Neurosci. 13:150. doi: 10.3389/fnhum.2019.00150
Turi, Z., Mittner, M., Lehr, A., Bürger, H., Antal, A., and Paulus, W. (2020). θ-γ cross-frequency transcranial alternating current stimulation over the trough impairs cognitive control. eNeuro 7, 1–12. doi: 10.1523/ENEURO.0126-20.2020
Unsworth, N., and Engle, R. W. (2007). On the division of short-term and working memory: an examination of simple and complex span and their relation to higher order abilities. Psychol. Bull. 133, 1038–1066. doi: 10.1037/0033-2909.133.6.1038
van der Meij, R., Kahana, M., and Maris, E. (2012). Phase-amplitude coupling in human electrocorticography is spatially distributed and phase diverse. J. Neurosci. 32, 111–123. doi: 10.1523/JNEUROSCI.4816-11.2012
Van Vugt, M. K., Chakravarthi, R., and Lachaux, J.-P. (2014). For whom the bell tolls: periodic reactivation of sensory cortex in the gamma band as a substrate of visual working memory maintenance. Front. Hum. Neurosci. 8:696. doi: 10.3389/fnhum.2014.00696
Veniero, D., Vossen, A., Gross, J., and Thut, G. (2015). Lasting EEG/MEG aftereffects of rhythmic transcranial brain stimulation: level of control over oscillatory network activity. Front. Cell. Neurosci. 9:477. doi: 10.3389/fncel.2015.00477
Vilberg, K. L., and Rugg, M. D. (2008). Memory retrieval and the parietal cortex: a review of evidence from a dual-process perspective. Neuropsychologia 46, 1787–1799. doi: 10.1016/j.neuropsychologia.2008.01.004
Vogel, E. K., Woodman, G. F., and Luck, S. J. (2001). Storage of features, conjunctions, and objects in visual working memory. J. Exp. Psychol. Hum. Percept. Perform. 27, 92–114. doi: 10.1037/0096-1523.27.1.92
Vosskuhl, J., Huster, R. J., and Herrmann, C. S. (2015). Increase in short-term memory capacity induced by down-regulating individual theta frequency via transcranial alternating current stimulation. Front. Hum. Neurosci. 9:257. doi: 10.3389/fnhum.2015.00257
Voytek, B., Canolty, R. T., Shestyuk, A., Crone, N. E., Parvizi, J., and Knight, R. T. (2010). Shifts in gamma phase-amplitude coupling frequency from theta to alpha over posterior cortex during visual tasks. Front. Hum. Neurosci. 4:191. doi: 10.3389/fnhum.2010.00191
Walsh, V., and Cowey, A. (2000). Transcranial magnetic stimulation and cognitive neuroscience. Nat. Rev. Neurosci. 1, 73–80. doi: 10.1038/35036239
Wang, J., Fang, Y., Wang, X., Yang, H., Yu, X., and Wang, H. (2017). Enhanced gamma activity and cross-frequency interaction of resting-state electroencephalographic oscillations in patients with Alzheimer's disease. Front. Aging Neurosci. 9:243. doi: 10.3389/fnagi.2017.00243
Weiler, M., Teixeira, C. V. L., Nogueira, M. H., De Campos, B. M., Damasceno, B. P., Cendes, F., et al. (2014). Differences and the relationship in default mode network intrinsic activity and functional connectivity in mild alzheimer's disease and amnestic mild cognitive impairment. Brain Connect. 4, 567–574. doi: 10.1089/brain.2014.0234
Wolinski, N., Cooper, N. R., Sauseng, P., and Romei, V. (2018). The speed of parietal theta frequency drives visuospatial working memory capacity. PLoS Biol. 16:e2005348. doi: 10.1371/journal.pbio.2005348
Zhang, H. Y., Wang, S. J., Xing, J., Liu, B., Ma, Z. L., Yang, M., et al. (2009). Detection of PCC functional connectivity characteristics in resting-state fMRI in mild Alzheimer's disease. Behav. Brain Res. 197, 103–108. doi: 10.1016/j.bbr.2008.08.012
Keywords: cross-frequency coupling, neuronal oscillations, phase-amplitude coupling, theta-gamma coupling, working memory
Citation: Abubaker M, Al Qasem W and Kvašňák E (2021) Working Memory and Cross-Frequency Coupling of Neuronal Oscillations. Front. Psychol. 12:756661. doi: 10.3389/fpsyg.2021.756661
Received: 10 August 2021; Accepted: 14 September 2021;
Published: 21 October 2021.
Edited by:
Robert West, DePauw University, United StatesReviewed by:
Kira Bailey, Ohio Wesleyan University, United StatesAshley Jean Scolaro, Central College, United States
Copyright © 2021 Abubaker, Al Qasem and Kvašňák. This is an open-access article distributed under the terms of the Creative Commons Attribution License (CC BY). The use, distribution or reproduction in other forums is permitted, provided the original author(s) and the copyright owner(s) are credited and that the original publication in this journal is cited, in accordance with accepted academic practice. No use, distribution or reproduction is permitted which does not comply with these terms.
*Correspondence: Mohammed Abubaker, mohammad.abubaker@lf3.cuni.cz