- 1Henan Airport Group Co, LTD, Zhengzhou, China
- 2School of Highway, Chang'an University, Xi’an, China
- 3Northwest Civil Aviation Airport Construction Group Co, LTD, Xi’an, China
The main objective of this study is to compare the differences between different gradation types of mixtures (stone matrix asphalt (SMA), large stone asphalt (LSA), stone asphalt concrete (SAC), and superior performing asphalt pavements (Superpave)) and conventional dense-graded mixtures (asphalt concrete (AC)) in terms of resistance to rutting, moisture damage, low-temperature cracking, and water permeability. The paper reports a study of the effect of different asphalt binders on the performance of asphalt mixtures. The experimental results demonstrated that the gradation types had a significant effect on the engineering performance of asphalt mixtures. SMA20, SAC20, and LSA30 exhibited lower rutting potential than AC20. In addition, SAC20 had a larger stripping ratio, while AC13 had a smaller stripping ratio. The stone matrix asphalt displayed the largest failure strain, while the SAC had the smallest, and Superpave ranged between SMA and SAC. SAC20 had the maximum permeability coefficient, followed by SMA20 and AC20, and Superpave20 had the smallest. The nominal maximum aggregate sizes significantly affected the resistance to permanent deformation, resistance to low-temperature cracking, and moisture sensitivity of the asphalt mixtures. Styrene–butadiene–styrene (SBS)-modified asphalt was found to significantly improve the performance of asphalt mixtures compared with ordinary asphalt.
1 Introduction
As trucks have become larger and operation frequency has increased in recent years, the wheel loads and tire pressures have exceeded the bearing capacity of traditional pavement. As a result, rutting, deformation, and fatigue cracking of flexible pavements are becoming more prevalent. Investigations have revealed that asphalt concrete does not have sufficient durability against heavy loads, which is affected by several factors such as properties of aggregates and asphalt, asphalt content, and environmental conditions (Izzo et al., 1997; Kandhal and Mallick, 2001; Ma et al., 2017; Ding et al., 2019; Zhu et al., 2020).
Road engineers and researchers are always looking for new solutions to address this problem. They have found that modification of asphalt binders can moderately improve the performance of hot mix asphalt (HMA). In particular, the introduction of polymers into the asphalt matrix increases its high-temperature viscosity, which contributes to the resistance of HMA to rutting during hot weather. Some specific types of polymer-modified asphalt are also able to reduce the stiffness of HMA at low-temperature. However, the investigations have confirmed that modification of asphalt alone cannot completely resolve serious rutting and cracking distress in pavements (Kandhal and Mallick, 2001; Mokhtari and Nejad, 2012).
Pavement engineering scholars have found that the properties and gradation types of mineral aggregates can significantly affect the mechanical strength (resilient modulus and tensile strength) and engineering performances (permanent deformation, low-temperature cracking, and moisture sensitivity) of hot mix asphalt mixtures (Sebaaly et al., 1997). Furthermore, it is beneficial to adjust the gradation composition of the aggregate to improve the performance of asphalt pavements while not changing the source and type of aggregate (Park et al., 2022). HMA mixtures are classified into five main categories based on gradation: stone matrix asphalt (SMA), stone asphalt concrete (SAC), large stone asphalt (LSA), superior performing asphalt pavements (Superpave), and conventional dense-graded asphalt concrete (AC) (Fang et al., 2019; Ferreira et al., 2020; Khasawneh and Alsheyab, 2020; Devulapalli et al., 2022).
SMA was developed in Germany in the 1960s. It is a deformation-resistant, durable paving material for heavily trafficked roads. SMA has been used as a durable asphalt pavement option for city streets and highways in Europe, Australia, the United States, and Canada. SMA has a high content of coarse aggregates that are interlocked to form a stone skeleton that resists permanent deformation. The stone skeleton is filled with a mastic consisting of asphalt and filler, into which fibers are incorporated to impart sufficient asphalt stability and inhibit the loss of binder during transport and placement. A typical SMA composition consists of 70%–80% coarse aggregate, 8%–12% filler, 6.0%–7.0% binder, and 0.3% fibers. Engineering practices in Europe have proven SMA to be more durable and resistant to rutting than dense-graded mixes (Mogawer and Stuart, 1994; Mokhtari and Nejad, 2012; Devulapalli et al., 2022).
Stone asphalt concrete (SAC) is a gap-graded HMA that is similar to a stone matrix asphalt (SMA) but tends to be more of a dense-graded mixture and is primarily designed to eliminate the requirement for expensive modified binders or fibers. Fairly good stone-to-stone contact is achieved by the structural skeleton formed by the coarser aggregates. The increased stone-to-stone contact is intended to provide greater rutting resistance. SAC mixes have a higher binder content than dense-graded mixes, thus providing a thicker asphalt film. The thicker asphalt film should resist moisture damage and aging better than conventional mixtures (Liu, 2011).
Large stone asphalt (LSA) is defined as an aggregate with a maximum size of more than 25 mm. The use of a large stone mixture could minimize or eliminate the rutting of heavy-duty asphalt pavement (Abdulshafi et al., 1999).
Superpave mixtures originated from a new mix design system proposed by the Strategic Highway Research Program (SHRP) that includes asphalt binder specifications for performance classification and a series of advanced mixture tests. In addition, the system focuses on binder characteristics, aggregate quality, and gradation. SHRP binder specifications are used to characterize asphalt properties related to rutting and cracking; however, the binder is only a relatively minor component of the HMA. Therefore, the quality and gradation of the aggregate are more important to the performance of the mixture. The SHRP gradation specification contains a restriction zone to avoid the bulge close to 0.6 mm in the gradation curve (the control point of the restriction zone is determined by the nominal maximum size of the aggregate). According to the Superpave specification, any gradation that passes above or below the restriction zone but within the relevant control points is expected to produce a good performance mixture (Kandhal and Cooley, 2001).
Dense-graded asphalt concrete (AC) is composed of aggregates, mineral powders, and asphalt binders, where the aggregate particles are continuously graded and interlocked. The void ratio of dense-graded asphalt concrete after compaction is less than 10% (Fang et al., 2019; Ferreira et al., 2020; Khasawneh and Alsheyab, 2020).
Many scholars have studied the influence of characteristics, gradation, and nominal maximum size of aggregate on the mechanical behavior and engineering performance of mixtures using methods based on different theories. Most findings indicated that the aggregate gradation has a significant impact on the resistance to rutting and permanent deformation of asphalt pavements (Kandhal and Mallick, 2001; Ferreira et al., 2020; Khasawneh and Alsheyab, 2020; Lira et al., 2021; Zhu et al., 2021; Devulapalli et al., 2022; Park et al., 2022).
However, previous studies have focused on a specific gradation type, such as dense-graded asphalt concrete (DGAC), stone matrix asphalt (SMA), and asphalt-treated base (ATB), and mainly vary the aggregate gradations by varying the proportion of different sizes of aggregates through different calculation methods (Zhu et al., 2021; Devulapalli et al., 2022). Little attention has been paid to the differences between the performance of mixtures composed of different gradation types and nominal maximum aggregate sizes when their properties, specifications, and sources are the same. In addition, the effect of different asphalt binders on the properties of asphalt mixtures consisting of different nominal maximum aggregate sizes (with the same gradation type) has not been clarified. Therefore, the main objectives of this study were to comparatively investigate the differences in terms of resistance to rutting, water damage, low-temperature cracking, and water permeability for different mixture gradation types (SMA, LSA, SAC, Superpave, and AC). The study also evaluated the effect of different asphalt binders on the mixture performance.
2 Materials and methods
2.1 Materials
Two types of binder were used in the study: a base asphalt with penetration ranging from 60 to 80 (labeled as Pen 60/80) and a styrene–butadiene–styrene (SBS)–polymer-modified asphalt binder (labeled as SBS MA) from KLMY Petroleum INC. The Pen 60/80 binder was used in this study to evaluate the effect of aggregate gradation and nominal maximum aggregate size on the pavement performance of the HMA mixture, and the SBS MA was used to investigate the effect of different binder types on the performance of the HMA mixture. The properties of binders are provided in Table 1.
To achieve the objectives of this study, the midpoint values of different gradation curves were selected as representative of different gradation types, where the nominal maximum aggregate size was selected as 20 mm (AC20, SMA20, SAC20, and Superpave20 (noted as SUPER20)). In addition, the nominal maximum aggregate size was set to 30 mm (i.e., LSA30), considering that the maximum nominal aggregate size of LSA mixtures should not be less than 25 mm. A group of dense-graded asphalt mixtures (AC13) was added to compare and analyze the effect of different asphalt binders and nominal maximum aggregate size on the mixture performance. The various gradation types are shown in Table 3.
2.2 Asphalt mixture preparation and tests
2.2.1 Asphalt mixture preparation
Engineering practice has confirmed that the Marshall volume design method has the advantages of simplicity and efficiency (MOT, 2011). To exclude the differences in mixes performance due to different preparation methods, the optimum asphalt content of the asphalt mixes in this study was determined using the standard Marshall method. It should be noted that the SMA20 mixture required the addition of SBS-modified asphalt with 0.4% (by weight of the mixture) fiber. Table 4 show the specific requirements (MOT, 2004) of the Marshall design method and the parameters of different asphalt mix volume indexes, respectively.
Figure 1 shows that the dense-graded mixture AC and SUPER20 have higher stability and lower flow values than SMA20 and SAC20. As for AC mixtures, the optimum asphalt content decreased with the increase of nominal maximum aggregate size, which is mainly attributed to the fact that the finer the aggregate, the larger its specific surface area, the more asphalt content it adsorbs, and the thicker the asphalt film on the aggregate surface. The LSA30 mixture has the lowest voids filled with asphalt (VFA), which is related to a larger air void and lower asphalt content. Meanwhile, the AC13 mixture presented higher voids in mineral aggregate (VMA) value than the other mixtures, resulting in a higher asphalt content for the AC13 mixture.
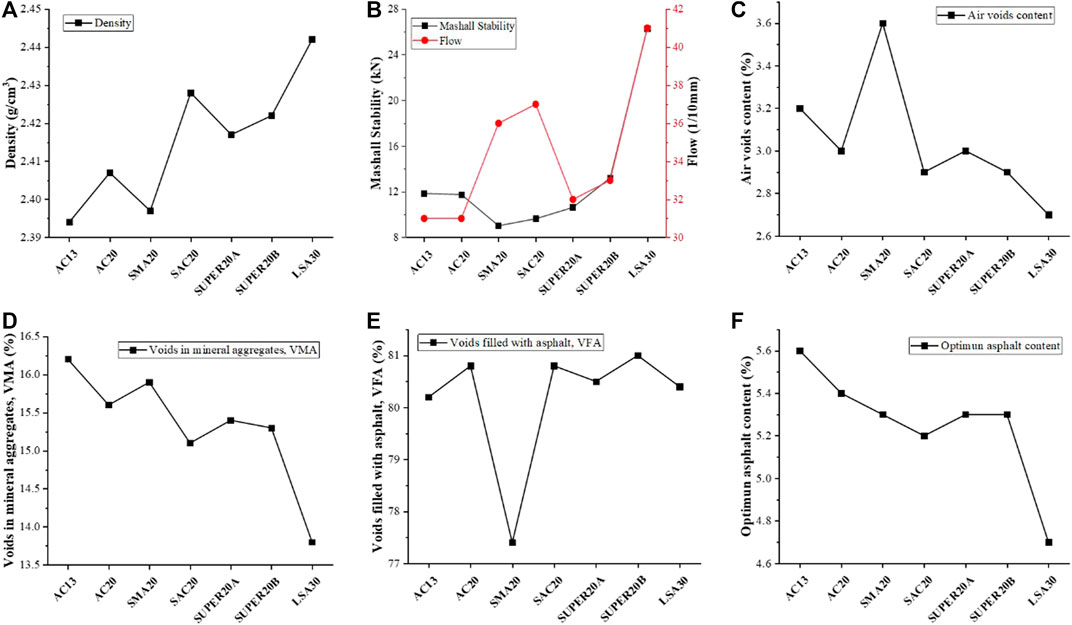
FIGURE 1. Volume parameters and optimum asphalt content of asphalt mixtures: (A) density, (B) mashall stability and flow, (C) air voids content, (D) voids in mineral aggregates, VMA (E) voids filled with asphalt, VFA, and (F) optimum asp halt content.
2.2.2 Asphalt mixture tests
Wheel tracking tests were conducted on all the mixtures based on JTG E20-2011 (MOT, 2011). The AC13, AC20, SMA20, SAC20, and SUPER20 mixture samples were prepared at 300 mm length, 300 mm width, and 50 mm thickness, while the samples of LSA30 were prepared at 300 mm length, 300 mm width, and 10 cm thickness. The test temperature was 60 °C with a tire pressure of 0.7 MPa and 1.44 MPa. Dynamic stability (DS) is used as an indicator of the high-temperature rutting resistance of asphalt mixtures. The larger the DS of the asphalt mixture, the better the mixture’s ability to resist high-temperature permanent deformation.
A moisture damage test was conducted on all the mixtures based on JHA3-7-4 (Japan Road Association, 1996). The test temperature was 60 °C in the water. Mixture samples (500 mm in length, 300 mm in width, and 50 mm in thickness) were tested for 6 h by the immersion water wheel tracking test. The stripping ratio (SA) can be calculated as follows:
where Sa represents the non-stripping area in cm2, and Sb is the stripping area in cm2. The larger the SA value, the better the resistance to water damage and the lower the water sensitivity of the asphalt mixture.
Three-point bending tests were carried out to evaluate the low-temperature cracking resistance performance of the asphalt mixture. In the study, samples with 250 mm length, 30 mm width, and 35 mm thickness were prepared, and the test was carried out at −10°C with a loading rate of 50 mm/min (MOT, 2011). Bending stress, failure strain, and stiffness were employed to characterize the low-temperature cracking resistance performance of the HMA mixture. Obviously, the higher the failure strain and bending stress, the better the low-temperature crack resistance of the asphalt mixture.
The permeability test was conducted according to JIS A 1218–1990 (Japan Road Association, 1996). Test conditions were 150 kPa (24 h) and 500 kPa (24 h) for water pressure and 20 °C for temperature.
3 Experimental results and discussions
3.1 Wheel tracking tests
The effect of gradation types on the rutting resistance of asphalt mixtures with different tire pressures is illustrated in Figure 2. The experimental results indicated that different gradation types of mixtures exhibited distinct high-temperature rutting resistance potential.
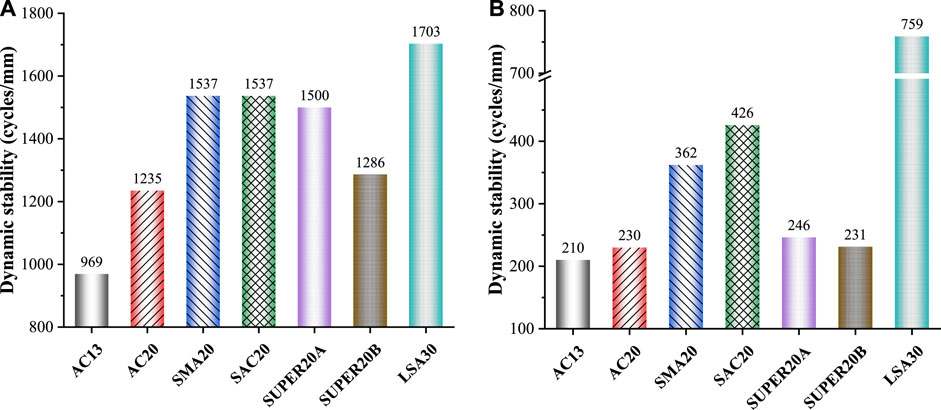
FIGURE 2. Dynamic stability versus gradation types under different tire pressures: (A) 0.7 MPa and (B) 1.44 MPa.
When the nominal maximum aggregate size was set to 20 mm and the tire pressure was 0.7 MPa, as shown in Figure 2A, the best rutting resistance (maximum dynamic stability) was obtained for both the SMA and SAC mixtures, which was mainly attributed to the large amount of coarse aggregates in the gradation forming a stable skeletal structure, thus improving the resistance to permanent deformation (rutting) of the asphalt mixture (Devulapalli et al., 2022). The dynamic stability of SUPER20A is slightly greater than that of SUPER20B, which was due to the larger proportion of coarse aggregate in the former. AC20 has the least dynamic stability because, on one hand, the higher fraction of fine aggregate in the gradation absorbed more free asphalt, and on the other hand, the synthetic gradation of coarse and fine aggregates formed a suspended dense structure in the asphalt mixture. The LSA30 mixture has the largest nominal maximum aggregate size and the highest coarse aggregate content. Meanwhile, the aggregates can contact and embed each other to achieve greater structural stability, so its mixture has the best rutting resistance.
A comparative analysis of the dynamic stability of AC13 and AC20 mixtures revealed that the high-temperature rutting resistance of the mixture was positively proportional to the nominal maximum aggregate size. In other words, the dynamic stability of AC20 was significantly higher than that of AC13 because the former contained more large-size aggregates and formed a certain skeletal structure that was able to resist the potential permanent deformation (Fang et al., 2019).
The tire pressure was elevated to 1.44 MPa, as shown in Figure 2B. Similar to Figure 2A, the LSA30 mixture has the largest dynamic stability, followed closely by SAC20, SMA20, SUPER20, and AC20, and the smallest was AC13. It should be noted that the dynamic stability of SAC20 was slightly superior to that of SMA20 at higher tire pressure, although contact or an aggregate skeleton had been achieved for both gradations. This outcome is mainly because the internal void content of the SAC20 mixture (2.9%) was lower than that of the SMA20 mixture (3.6%), so the former has less space for the mixture to deform at high tire pressure. Thus, the SAC20 mixture presented better anti-rutting performance.
The aforementioned analysis revealed that aggregate gradation significantly affected the high-temperature rutting resistance of the mixture, and the more coarse aggregate content in the gradation and the larger the nominal maximum particle size, the better the high-temperature resistance of the mixture to permanent deformation.
3.2 Wheel tracking tests under immersion in water
The results of wheel tracking tests of mixtures with different gradation types under water immersion are presented in Figure 3.
When the nominal maximum aggregate size was fixed at 20 mm, the order of stripping ratio of the asphalt mixture was as follows: SAC > SUPER > AC > SMA. It is generally believed that the thicker the asphalt film wrapped around the aggregate, the lower the moisture damage suffered by the mixture. Obviously, the higher coarse aggregate content and relatively low specific surface area in SAC20 resulted in less asphalt coating and reduced asphalt film thickness, which led to a larger stripping ratio. Compared to the SAC20 mixture, the proportion of coarse aggregate in the SUPER20 mixture was slightly lower, while the SMA mixture showed the best resistance to moisture damage due to the presence of fibers, which fixed more asphalt and formed a thicker asphalt film (Devulapalli et al., 2022). Despite the higher fine aggregate content and the greater asphalt film thickness in the AC20 mixture, its dense suspension structure had difficulty supporting the repeated operation of the test wheel and exhibited a lower level of moisture damage. The stripping ratio dramatically increased when a larger size aggregate such as LSA30 was employed, which was mainly attributed to the greater void content and coarse aggregate percentage within the mixture. The stripping ratio of the AC13 mixture was minimal due to the lesser void ratio.
Comparing AC13 and AC20, it was found that the stripping ratio of the mixture increased with the increasing nominal maximum aggregate size. In other words, the larger the nominal maximum aggregate size, the higher the moisture damage and the worse the water stability. In a word, the aggregate gradation types and nominal maximum aggregate sizes significantly affected the resistance of the mixture to moisture damage.
3.3 Three-point bending tests
Figure 4 plots the results of beam bending tests of mixtures with different gradation types at −10 °C. Normally, the higher the failure stress and failure strain and the lower the fracture stiffness of asphalt mixtures, the better its low-temperature cracking resistance.
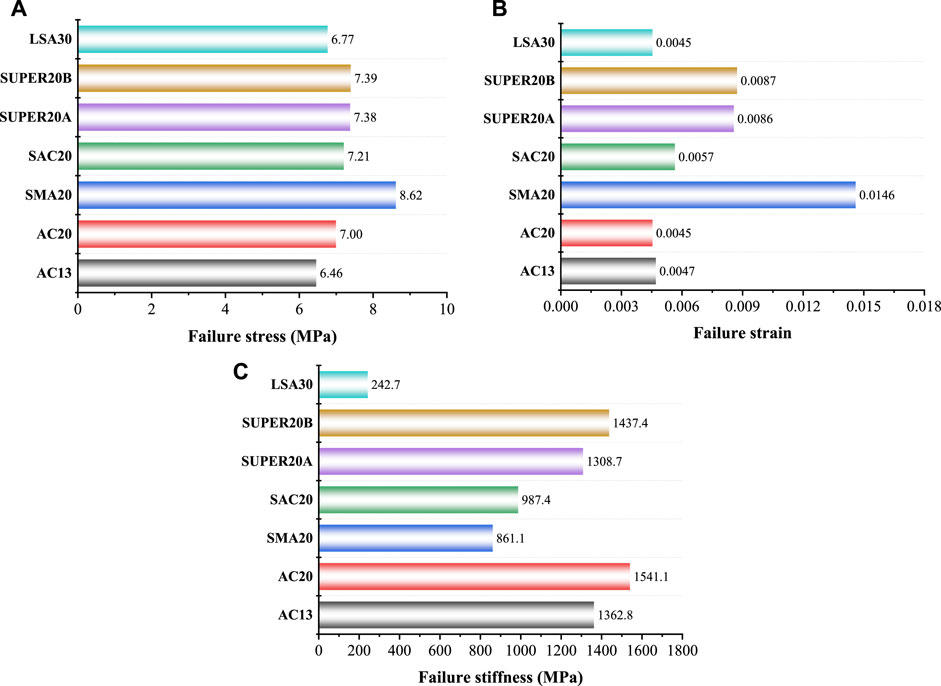
FIGURE 4. Low-temperature cracking parameters versus gradation types: (A) failure stress, (B) failure strain, and (C) failure stiffness.
It can be seen from Figure 4A that when the nominal maximum aggregate size was constant, the failure stresses of mixtures with different gradation types were quite similar (except for the SMA mixture). Even if the nominal maximum particle size was adjusted to 13 mm or 30 mm, the failure stresses of mixtures with different gradation types were almost the same. In other words, the effect of gradation type on the low-temperature failure stresses of the mixtures was not significant, which might be explained by the synergistic capability of the embedded structure of coarse aggregate and the asphalt mastic to resist the bending and tensile loads.
Figure 4B confirms that the effect of aggregate gradation type on the failure strain of the mixture was more pronounced. Specifically, when the nominal maximum aggregate size was retained at 20 mm, the SMA20 mixture presented the maximum failure strain, while the failure strains of SAC20 and AC20 mixtures were smaller, and that of the SUPER20 mixture was between SMA20 and SAC20. This phenomenon can be explained by the fact that the SMA mixture has a thicker effective asphalt film compared with AC20, SAC20, and SUPER20. The LSA30 mixture has the lowest low-temperature failure strain, which was associated with a lower asphalt content. In addition, when the same dense-graded mixture, such as AC, was employed, the failure strain of the mixture decreased with increasing nominal maximum aggregate size. In other words, the mixture with a higher percentage of coarse aggregates (AC20) experienced worse resistance to low-temperature cracking, owing to the lesser amount of asphalt and the thinner effective asphalt film.
It can be seen from Figure 4C that the variation of the failure stiffness of the mixture was almost opposite to the failure strain at constant nominal maximum aggregate size. The failure stiffness of the SMA20 mixture was less than that of SAC20, SUPER20, and AC20. It is worth noting that the failure stiffness of SUPER20 was not between SMA20 and SAC20 as expected. This is mainly because the failure stiffness of the mixtures was determined by the combination of failure stress and failure strain. The failure stiffness of the AC20 mixture was slightly higher than that of the AC13 mixture.
The aforementioned analysis suggested that the aggregate gradation type and nominal maximum aggregate size would significantly affect the failure strain of the mixture, but the mechanism of its effect on the failure stress and failure stiffness has yet to be clarified.
3.4 Water permeability test
Figure 5 shows the permeability coefficients for the different gradation types of mixtures. For the mixture with a nominal maximum aggregate size of 20 mm, SAC20 had a much higher permeability coefficient than the other types of mixtures. AC20 and SUPER20 obtained a smaller permeability coefficient, while SMA20 was between SUPER20 and AC20. This phenomenon would be interpreted as follows: SAC20 contained more coarse aggregates and formed a structural skeleton through stone-to-stone contact. Therefore, more air voids may have been generated between the structural skeleton during the hot mix asphalt mixture forming process, which eventually led to the flow of water through the voids and yielded a larger water permeability coefficient. AC20 has a suspended dense structure with fewer internal voids, while SMA20 and SUPER20 have a thicker asphalt film, which makes it difficult for water to penetrate through the mixture. For the LSA30 mixture, more coarse aggregates formed the skeleton and created more voids that could not be filled with a small amount of asphalt; thus, it had the largest permeability coefficient.
Furthermore, when the aggregate gradation type was the same, such as AC, increasing the nominal maximum aggregate size would significantly enlarge the water permeability coefficient of the mixture because as the particle size increased, the internal voids of the mixture gradually increased, and water penetrated more easily. Consequently, both the type of aggregate gradation and the nominal maximum aggregate size affected the permeability performance of the mixture.
3.5 The effect of asphalt binders
Figure 6 presents the effect of different asphalt binders on the high-temperature rutting resistance and low-temperature cracking resistance of the mixtures. Figure 6A shows that the SBS-modified asphalt imparted greater dynamic stability to the mixture when the aggregate gradation was the same (AC). For AC13, the dynamic stability of the mixture increased sharply from 969 to 6,300, an increase of 550%, when the asphalt binder was adjusted from ordinary base asphalt (Pen 60/80) to SBS-modified asphalt (SBS MA). This change also was applicable to AC20.
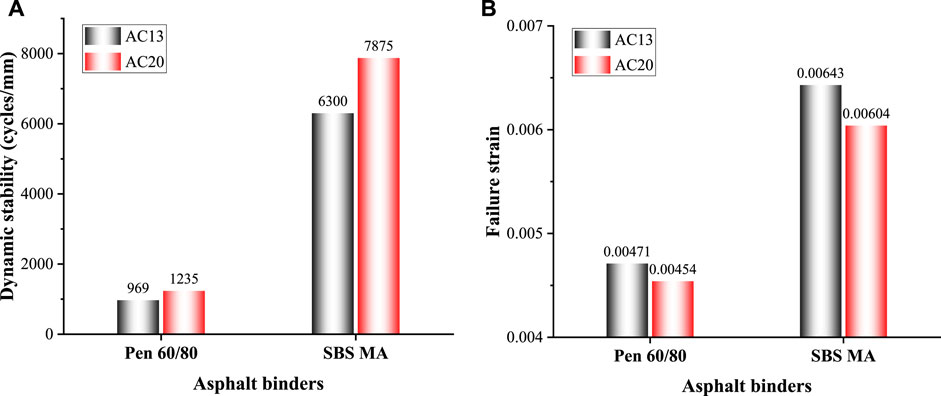
FIGURE 6. Rutting and cracking resistance of mixtures with different asphalt binders: (A) dynamic stability and (B) failure strain.
Figure 6B demonstrates that the SBS-modified asphalt was also able to significantly improve the low-temperature failure strain of the mixture, thus enhancing the low-temperature cracking resistance of the mixture. Similarly, the failure strain of the AC13 mixture increased rapidly from 0.00471 to 0.00643, an enhancement of nearly 37%, as the asphalt binder was changed to SBS-modified asphalt.
The aforementioned variations in mixture performance confirmed that SBS-modified asphalt significantly improved the high- and low-temperature performance of the mixtures. In particular, SBS MA enhanced the high-temperature rutting resistance of the dense-graded mixes more significantly, which was mainly attributed to the excellent elasticity and flexibility of the polymer SBS. When the temperature was high, the viscosity of the SBS-modified asphalt was much higher than that of the conventional base asphalt, which effectively prevented the asphalt from flowing. In addition, the excellent elasticity of SBS enabled the asphalt mixture to deform and recover moderately after wheel loading, reducing the amount of permanent deformation and thus improving the high-temperature rutting resistance of the mixture. At the same time, the excellent flexibility of SBS-modified asphalt allowed it to withstand greater bending and tensile deformation at low-temperature, thus improving the low-temperature cracking resistance of the mixture.
4 Conclusion
On the basis of laboratory test results and analysis, the following conclusions were drawn.
• Dense-graded mixtures AC20 and SUPER20 exhibited greater stability and smaller flow values than SMA20 and SAC20. When the gradation type was fixed, the optimum asphalt content of the mixture decreased with increasing nominal maximum aggregate size.
• Wheel tracking tests confirmed that better high-temperature rutting resistance was obtained for SMA20 and SAC20, followed by SUPER20 and AC20. For the AC mixtures, the rutting resistance of the asphalt mixtures increased with the increase in nominal maximum aggregate size.
• Wheel tracking tests with water immersion showed that SAC20 possessed a larger stripping ratio, while AC20 had less moisture damage. LSA30 and AC13 yielded the largest and smallest stripping ratios, respectively. The moisture sensitivity of the mixtures increased with increasing nominal maximum aggregate size when the gradation types were the same.
• The three-point bending test illustrated that SMA20 obtained the maximum low-temperature failure strain (best low-temperature cracking resistance). SAC20 had the least failure strain, while SUPER20 was between SMA20 and SAC20. LSA30 had the worst low-temperature performance. For dense-graded mixtures, the low-temperature performance decreased with increasing nominal maximum aggregate size.
• The permeability tests showed that when the nominal maximum aggregate size was the same, the permeability coefficient was the highest for SAC20, followed by SMA20 and AC20, and the lowest for SUPER20. For the AC mixtures, the permeability coefficient increased with increasing nominal maximum aggregate size.
• Compared with the common base asphalt (Pen 60/80), SBS-modified asphalt improved both the high-temperature rutting resistance and low-temperature cracking resistance of dense-graded asphalt mixtures. In particular, it was more effective in enhancing the high-temperature performance of the mixtures.
Data availability statement
The original contributions presented in the study are included in the article/supplementary material; further inquiries can be directed to the corresponding author.
Author contributions
TJ: acquisition of data; PH and JL: revised the manuscript critically for important intellectual content; HS and KY: analysis of data; HL: drafted the manuscript; DW and RK: acquisition of data.
Conflict of interest
Authors TJ, HS and RK were employed by Henan Airport Group Co, LTD. Authors KY and DW were employed by Northwest Civil Aviation Airport Construction Group Co, LTD.
The remaining authors declare that the research was conducted in the absence of any commercial or financial relationships that could be construed as a potential conflict of interest.
Publisher’s note
All claims expressed in this article are solely those of the authors and do not necessarily represent those of their affiliated organizations, or those of the publisher, the editors, and the reviewers. Any product that may be evaluated in this article, or claim that may be made by its manufacturer, is not guaranteed or endorsed by the publisher.
References
Abdulshafi, O. A., Fitch, M. G., Kedzierski, B., and Powers, D. B. (1999). Laboratory optimization of asphalt concrete intermediate course mixes to improve flexible pavement performance. Transp. Res. Rec. 1681 (1), 69–75. doi:10.3141/1681-09
Devulapalli, L., Sarang, G., and Kothandaraman, S. (2022). Characteristics of aggregate gradation, drain down and stabilizing agents in stone matrix asphalt mixtures: A state of art review. Journal of traffic and transportation engineering. English Edition.
Ding, Xunhao, Chen, Luchuan, Ma, Tao, Ma, Hai Xia, Gu, Linhao, Chen, Tian, et al. (2019). Laboratory investigation of the recycled asphalt concrete with stable crumb rubber asphalt binder. Constr. Build. Mater. 203, 552–557. doi:10.1016/j.conbuildmat.2019.01.114
Fang, M., Park, D., Singuranayo, J. L., Chen, H., and Li, Y. (2019). Aggregate gradation theory, design and its impact on asphalt pavement performance: A review. Int. J. Pavement Eng. 20 (12), 1408–1424. doi:10.1080/10298436.2018.1430365
Ferreira, J. L., Babadopulos, L. F., Bastos, J. B., and Soares, J. B. (2020). A tool to design rutting resistant asphalt mixes through aggregate gradation selection. Constr. Build. Mater. 236, 117531. doi:10.1016/j.conbuildmat.2019.117531
Izzo, R. P., Button, J. W., and Tahmoressi, M. (1997). Comparison of coarse matrix high binder and dense-graded hot-mix asphalt. Transp. Res. Rec. 1590 (1), 108–117. doi:10.3141/1590-13
Kandhal, P., and Cooley, L. (2001). The restricted zone in the Superpave aggregate gradation specification. National Cooperative Highway Research Program NCHRP Report 464. Washington, DC: Transportation Research Board, National Research Council.
Kandhal, P. S., and Mallick, R. B. (2001). Effect of mix gradation on rutting potential of dense-graded asphalt mixtures. Transp. Res. Rec. 1767 (1), 146–151. doi:10.3141/1767-18
Khasawneh, M. A., and Alsheyab, M. A. (2020). Effect of nominal maximum aggregate size and aggregate gradation on the surface frictional properties of hot mix asphalt mixtures. Constr. Build. Mater. 244, 118355. doi:10.1016/j.conbuildmat.2020.118355
Lira, B., Ekblad, J., and Lundström, R. (2021). Evaluation of asphalt rutting based on mixture aggregate gradation. Road Mater. Pavement Des. 22 (5), 1160–1177. doi:10.1080/14680629.2019.1683061
Liu, H. Y. (2011). Analysis of aggregate blending of stone asphalt concrete. J. Wuhan Univ. Technol. 23, 43–47. (In Chinese).
Ma, Tao, Wang, Hao, Zhao, Yongli, Huang, Xiaoming, and Wang, Siqi (2017). Laboratory investigation of crumb rubber modified asphalt binder and mixtures with warmmix additives. Int. J. Civ. Eng. 15 (2), 185–194. doi:10.1007/s40999-016-0040-3
MOT, (2011). JTG E20-2011, standard test methods of bitumen and bituminous mixtures for highway engineering. PR China (Beijing). (In Chinese).
MOT, (2004). Technical specifications for construction of highway asphalt pavements. PR China (Beijing). (In Chines).
Mogawer, W. S., and Stuart, K. D. (1994). Evaluation of stone matrix asphalt versus dense-graded mixtures. Washington DC: Transportation Research Record, Transportation Research Board.
Mokhtari, A., and Nejad, F. M. (2012). Mechanistic approach for fiber and polymer modified SMA mixtures. Constr. Build. Mater. 36, 381–390. doi:10.1016/j.conbuildmat.2012.05.032
Park, B., Cocconcelli, C., and Chun, S. (2022). Gradation characteristics-based interlayer mixture design method for enhanced rutting resistance of asphalt pavements. Can. J. Civ. Eng. 49 (4), 607–616. doi:10.1139/cjce-2021-0078
Sebaaly, P. E., Ridolfi, D., Gangavaram, R. S., and Epps, J. A. (1997). Selecting most desirable hot-mix asphalt mixtures. Transp. Res. Rec. 1590 (1), 99–107. doi:10.3141/1590-12
Zhu, F., Han, J., Zhang, S., and Dong, W. (2021). A new method for the aggregate proportion calculation and gradation optimization of asphalt-treated base (atb-25). Math. Problems Eng. 2021, 1–9. doi:10.1155/2021/9709070
Keywords: asphalt mixture, gradation, SMA, LSA, SAC, Superpave, characteristics
Citation: Ji T, Hao P, She H, Yang K, Li H, Wang D, Kang R and Liu J (2023) Durability behavior of asphalt mixtures in regard to material properties and gradation type. Front. Mater. 10:1151479. doi: 10.3389/fmats.2023.1151479
Received: 26 January 2023; Accepted: 15 February 2023;
Published: 03 March 2023.
Edited by:
Zhanping You, Michigan Technological University, United StatesReviewed by:
Hui Yao, Beijing University of Technology, ChinaTao Ma, Southeast University, China
Ke Zhang, Fuyang Normal University, China
Copyright © 2023 Ji, Hao, She, Yang, Li, Wang, Kang and Liu. This is an open-access article distributed under the terms of the Creative Commons Attribution License (CC BY). The use, distribution or reproduction in other forums is permitted, provided the original author(s) and the copyright owner(s) are credited and that the original publication in this journal is cited, in accordance with accepted academic practice. No use, distribution or reproduction is permitted which does not comply with these terms.
*Correspondence: Peiwen Hao, c3VwZXJwYXZlQDE2My5jb20=