- 1Department of Physics, Faculty of Sciences, International Islamic University, Islamabad, Pakistan
- 2Department of Statistics and Operations Research, College of Science, King Saud University, Riyadh, Saudi Arabia
- 3HITEC Colleges, HIT Taxila Cantt, Rawlpindi, Pakistan
- 4Institute of Space Technology, Islamabad, Pakistan
- 5Section of Mathematics, International Telematic University Uninettuno, Corso Vittorio Emanuele II, Roma, Italy
Polycrystalline compounds of lanthanum strontium manganite (La1-xSrxMnO3, LSMO) are widely used in electronic storage devices due to their minimal losses and electronic charge transport properties. In this study, we investigated how varying substitutions of Sr2+ for values of x = 0.0, 0.1, and 0.2 affected the tuning of the optical band gap and dielectric losses in La1-xSrxMnO3 nanoparticles. Synthesized samples were structurally analyzed via X-ray diffraction. A rhombohedral R
1 Introduction
In recent years, research motivated by the development of renewable energy resources has become a popular area for scientists. The use of natural energy resources creates a pollution-free environment and contributes less to climate change as compared to fossil fuels. Numerous materials are fabricated to convert these energy resources into useful electrical energy. For this reason, extensive research has been conducted on lanthanum-based ABO3-type perovskite oxide materials due to their diverse range of applications in many electrical and magnetic storage devices. In ABO3-type perovskite, A represents rare earth or alkaline earth positive cation metals and B denotes transition metal cations; their combined sum is 6, as in A3+B3+O3, A2+B4+O3, or A1+B5+O3. Lanthanum manganite (LaMnO3) nanoparticles exhibit unique optical and electrical properties and are widely used in many applications such as LiO2 batteries, supercapacitors, photocatalytic activity, and sustainable dye-synthesized solar cells (Hernández et al., 2015; Li et al., 2018; Shafi et al., 2018; Özkan et al., 2021). Existing studies have reported that high-specific-surface-area LaMnO3 nanoparticles are associated with very high catalytic activity in the oxygen reduction reaction (ORR) and oxygen evolution reaction (OER) due to the creation of oxygen vacancies in the Mn3+-O2--Mn4+ network (Li et al., 2018; Miao et al., 2020; Yan et al., 2020). The Jahn–Teller distortion in the octahedral symmetry of MnO6 due to substitution on either the A or the B site in the LaMnO3 system causes transitions from Mn3+ to Mn4+ ions, thereby enhancing the chemical stability, colossal magnetoresistance, nitrogen monoxide (NO) absorption capacity, and pseudocapacitive performance of perovskite materials (Shafi et al., 2018; Hu et al., 2019; Flores-Lasluisa et al., 2022). We can control particle size, electrical conductivity, optical band gap, and dielectric constant by partially substituting the divalent cation, such as Ca2+, Sr2+, Ba2+, or Mg2+ (Solopan et al., 2012; Zhong et al., 2013; Afify et al., 2021), with an La3+ ion on the A site in La1-xAxMnO3 perovskites.
Over the course of a few years, it has been observed that partially substituted lanthanum magnetite (La1-xAxMnO3) nanoparticles undergo electric polarization to determine the permittivity spectrum; based on this spectrum, this material is competitive in several applications due to its extremely high static dielectric constant (specifically, on the order of 105) (Assoudi et al., 2018; Mleiki et al., 2020). Doping with alkaline earth metals, such as Sr2+, enhances the material’s overall optical and dielectric characteristics. Tripathi et al. (Naseem Siddique et al., 2019) have reported that Sr reduces defects and improves the optical spectra of NiO nanoparticles. Du Huiling et al. (Arshad et al., 2020) revealed that dielectric losses are reduced upon Sr2+ doping in BST ceramics. Kandil et al. (Turky and Kandil, 2013) observed a significant increase in dielectric constant with the use of a higher Sr2+ molar ratio in BST nanoparticles. Transition metals, such as manganese (Mn), play a vital role in charge transport when it undergoes transition via partial replacement of Sr2+ (1.44 Å) with La3+ (1.36 Å) (Turky et al., 2016), improving electrical properties that are connected to dielectric losses in the desired material. Typically, tunneling and hooping processes can demonstrate the movement of these charge carriers (Hizi et al., 2022). In polycrystalline material, at high frequency, charge carriers move from one localized state to another across grain boundaries through a process known as hooping, which can reduce the defect density and contribute to an increase in the AC conductivity of the material (Mohanty et al., 2020a). It has been reported that, in an external electric field, charge carriers build up at grain boundaries and cause polarization in the material, thereby increasing the dielectric constant and overall electrical AC conductivity of doped material (Mohanty et al., 2020a; Mohanty et al., 2020b). Ali Omar Turky et al. prepared LSM nanoparticles for values of x = 0.2, 0.5, and 0.8 using precipitation (Turky et al., 2016) and citrate precursor (Turky et al., 2017) techniques, and reported observing increases in the optical band gap with a decrease in dielectric constant for higher values of x. According to Andreja Žužić et al. (Žužić et al., 2022), LSM nanoparticles prepared by the co-precipitation method possess higher electrical conductivity. Sandhya Suresh et al. (Sandhya et al., 2023) synthesized a series of LSM nanoparticles for values of x = 0.0, 0.3, 0.5, 0.7 by the combustion method and observed band gap increases with a decrease in the refractive index and dielectric losses. The main highlight of this work was the x = 0.7 sample; the authors noted a significant increase in dielectric constant with minimal dielectric losses, which may be attributable to large grain boundaries with comparatively more strain produced by Mn4+ ions on lattice sites. Extensive research has been conducted on LSM nanoparticles, as a result of which the research gap has been diminished; however, here we report a decrease in losses with band gap in a series of La1-xSrxMnO3 stable nanoparticles for x = 0.0, 0.1, 0.2, synthesized by the precipitation method.
To avoid low conducting SrZrO3 phase formation, we utilized molar ratios of Sr ion of only 0.0, 0.1, and 0.2 in the La1-xSrxMnO3 lattice for relatively a high conducting phase. It has been reported that samples prepared via the precipitation technique have higher conductivity, recorded as 0.176 Ω−1∙cm−1 at room temperature for 20% Sr2+ concentration, which is comparable to the conductivity of silicon (Žužić et al., 2022). The hooping mechanism at the octahedral site in this La1-xSrxMnO3 system indicates that the tuning of optoelectrical properties, including optical band gap, refractive index, frequency-dependent dielectric constant, dielectric losses, and AC conductivity, is strongly influenced by degrees of substitution, lattice mismatch, and grain size.
2 Experimental details
2.1 Materials
Chemicals with purity levels of 99.99% were utilized in the experiment, including strontium chloride hexahydrate [SrCl2.6H2O], manganese acetate tetrahydrate [C4H6 MnO4.4H2O], and lanthanum (III) nitrate hexahydrate [La (NO3)3.6H2O]. As a precipitating agent, sodium hydroxide (NaOH) was also made available by Sigma-Aldrich. The chemicals were all utilized as procured directly from the supplier without any additional purification.
2.2 Sample preparation
To obtain LSMO nanoparticles for both undoped and Sr-doped samples, the co-precipitation method was utilized. Chemicals including strontium chloride hexahydrate [SrCl2.6H2O], manganese acetate tetrahydrate [C4H6 MnO4.4H2O], and lanthanum (III) nitrate hexahydrate [La (NO3)3.6H2O] were used in the experiment. These chemicals were dissolved in 300 mL of deionized water in the proportions of (1-x) (La (NO3)3.6H2O):x (SrCl2.6H2O): (C4H6 MnO4.4H2O) for (0.0 ≤ x ≤ 0⋅2), with a 5-g sample of each. The pH was adjusted to 8.5 through addition of 50 mL of a 1M NaOH solution progressively, with constant stirring, for 2 hours at 80 °C until black precipitates were obtained. The precipitates were cleaned of contaminants by centrifuging for 10 minutes; they were rinsed with distilled water each time. To obtain maximum yield, a filtration technique with Whatman filter paper was employed, and the precipitates were dried at 100oC for 5 h to obtain a pure solid phase. The final yield, LSMO powder, was annealed in a tube furnace for 10 h at 600oC. The same procedure was used to obtain Sr-doped specimens. The prepared samples were labeled La1-xSrxMnO3 for x = 0.0, 0.1, 0.2 nanoparticles.
2.3 Characterization techniques for LSMO powder
Advanced nanotechnology methods were utilized to characterize the atomic behavior of powder LSMO nanoparticles. A diffractometer system (XPERT-3 Malvern Panalytical) was utilized to investigate crystallographic manipulation over a wide angular region (50
3 Results and discussion
3.1 Structural properties
The X-ray diffraction (XRD) profiles of all prepared samples revealed their composition of pure La1-xSrxMnO3
To ensure sample phase purity, step scanning was performed at room temperature across the angular range 22.9° < 2 theta <68.6°. In Figure 1, peaks are indexed according to the electron density in the material at different diffraction angles.
The average crystallite size (D) was determined using Debye-Scherer’s equation:
where D represents the crystallite size, λ the wavelength of X-rays, θ the Bragg diffraction angle, and β the full width at half maximum (FWHM) of the diffraction peak (Wang et al., 2022; Ali Akhtar et al., 2023). The average crystallite size ranged between 15 and 20 nm, clarifying the crystallinity of the synthesized samples. The lattice constant obtained indicated anisotropic behavior with unit cell volume, which may be attributed to two main factors. First, the substitution of a relatively larger ion Sr2+ (1.44 Å) for coordination number 12 could have caused a portion of the Mn3+ ions to oxidize to Mn4+ ions, which have smaller ionic radii (Žužić et al., 2022). This oxidation could result in a contraction of the unit cell. Second, the existence of vacancies at oxygen sites might also have contributed to the reduction of electrostatic forces, leading to a decrease in unit cell volume. The lattice constants obtained for rhombohedrals with a hexagonal morphology (Manohar and Krishnamoorthi, 2017) are related to the unit cell volume given by Eq 2:
where a and c are lattice constants (Shkir et al., 2020a). It has been noticed that the presence of Sr2+ in host lattice La1-xSrxMnO3 decreases the dislocation density (defects per unit volume); this can be defined in terms of interims of crystallite size as:
The outcomes of Eq 3 (Chandekar et al., 2021b) confirmed that the crystallinity of the desired samples increased with a decrease in defects from 0.0044 to 0.0025, which makes them more suitable for storage applications. The macro strain, X-ray density, and specific surface area were also estimated to identify the influence of dopant atoms on their optoelectrical properties; this is discussed in later sections. Hence, Eqs. 4–6 were used to determine the micro-strain, X-ray density, and specific surface area expressed as follows:
and
In Eq 5,
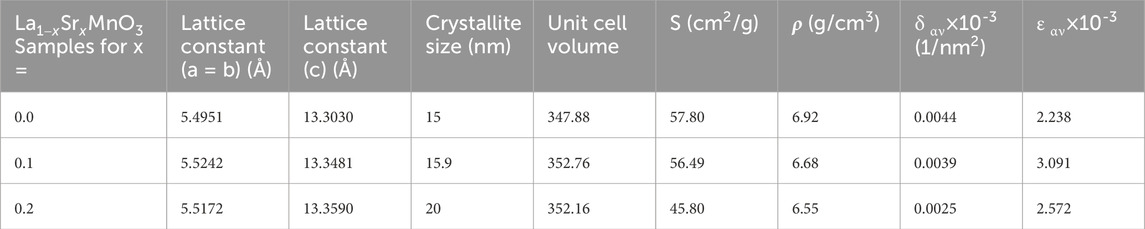
Table 1. Lattice constants, crystallite size, unit cell volume, specific surface area, X-ray density, dislocation density, and micro-strain for
3.2 Morphological studies
Field emission scanning electron microscopy (FESEM) was utilized to investigate the surface morphology of all prepared samples. No additional contaminants were observed in SEM images, as shown in Figure 2A–C. The XRD results exhibited strong agreement: with no extra phases, all LSMO-prepared nanoparticles for x = 0.0, 0.1, and 0.2 annealed at 600°C for 10 h confirmed the hexagonal-like morphology of the nanoparticles. A high potential of approximately 10.0 kV electrons was employed to capture images using backscattered electrons at resolutions of 500 nm and 200 nm, illustrated with histograms in Figure 2A–C. It can be seen that, in the case of 0.2 concentration of Sr2+ ion content, the particles were agglomerated faceted powders. A higher concentration of Sr2+ ion created more defects in the crystal structure via oxidization of Mn3+/Mn4+ transitions, leading to a more conductive nature. The average particle or grain size distribution is shown for all LSMO compounds, and the moderate ranges of particles in SEM images are displayed in histograms. The particle sizes of all reported samples are listed in Table 2.
A simple statistical relation was utilized to calculate average particle size from the corresponding histograms:
3.3 Compositional analysis
Energy-dispersive X-ray (EDX) spectra offer proof of the stoichiometry of the nominal composition. Quantitative energy-dispersive X-ray spectroscopy was used to determine the chemical composition of the synthetic La1-xSrxMnO3 (x = 0.0, 0.1, 0.2) samples, as shown in Figure 3A–C.
The elements La, Sr, Mn, and O were present in the synthetic samples, according to the EDX spectrum. Figure 3A–C illustrates the presence of peaks for lanthanum, manganese, oxygen, and strontium, illustrating the phase purity and elemental composition. There was no additional peak for impurities.
The initial values utilized during synthesis and the atomic weight percentages of elements used during synthesis as obtained from the EDX spectrum were in good accord. The weights and atomic percentages of the samples are shown in Table 3. Nanoparticles that were created had an excess of oxygen and a nearly stoichiometric composition (Jadhav et al., 2015).
3.4 FTIR analysis
Using IRTracer-100, functional groups located in prepared samples in the 400–4,000 cm-1 range were identified. The Fourier transform infrared (FTIR) spectroscopy spectra of each sample are shown in Figure 4. Six oxygen atoms were positioned around one Mn atom in the MnO6 structure, which had perfect octahedral symmetry. IR activity was present in only two of the six vibrational modes. These IR band positions matched well with those reported in the literature (Hassan et al., 2020). The bending modes of Mn-O around 465 and 477 cm-1 can be attributed to a change in the Mn-O-Mn bond angle, as observed, whereas stretching vibrations in Mn-O-Mn can be ascribed to the wavenumber between 690 and 706 cm-1. Bending and stretching vibrations may be due to a change in bond angle and internal motion or a change in the length of the Mn-O-Mn molecule.
Transmission peaks of Mn-O-Mn stretching are shifted toward a lower wave number, while the bending vibrations of Mn-O-Mn are displaced to a higher one, according to the energy relation represented by Eq 7. The change in the bond length and angle under the influence of ionic defects in the crystal lattice brought on by the substitution ratio Sr2+/La3+ leads to a transition from Mn3+ to the smaller Mn4+ ion.
where c represents the photon’s speed, E the photon’s energy, h Planck’s constant, and
3.5 Optical measurements
The influence of Sr2+ on the optical and charge transport properties of La1-xSrxMnO3 for x = 0.0, 0.1, and 0.2 nanostructures can be discussed in terms of optical band gap and refractive index. The absorption spectrum of LSMO nanoparticles in an ultraviolet (UV) region (200-340) nm is shown in Figure 5. All samples possessed a good absorption edge in the UV range, which can be ascribed to the inorganic nature of LSMO compound; typically, direct and forbidden transitions are responsible for such weak absorption peaks around 280 nm (Naseem Siddique et al., 2019). It can be observed that, as a result of increasing Sr2+ content from 0.1 to 0.2, a notable shift in
This variation in absorption edge as a result of different values of x, attributed to the alteration in the optical band gap (Eg) as a function of the diameter of a particle or grain size, can be expressed in terms of the Brass effective model (Hassan et al., 2020).
Here, me, mh, and R represent the effective masses of the electrons, holes, and diameter of particle size, respectively, while
where
where A represents absorbance and d denotes the cuvette’s thickness, usually taken as 1 cm. To obtain the values of absorption edges for direct transitions, we plotted the graph (
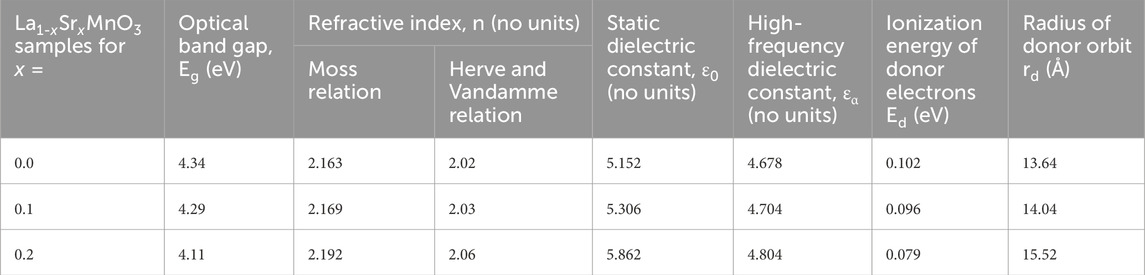
Table 4. The optical band gap, refractive index, static dielectric constant, high-frequency dielectric constant, ionization energy, and radius of the donor atom for La1-xSrxMnO3 (x = 0.0, 0.1, and 0.2) nanoparticles.
The narrowing of the energy band gap is caused by the small numbers of voids/defects present in a crystal lattice, according to Kamlesh V. Chandekar et al. (Kamlesh et al., 2021a). In the present series of LSMO, results obtained from XRD analysis showed that dislocation density (defects per unit volume) decreased from 0.0044 to 0.0025, correlated with an increase in crystallite size from 15 to 20 nm, as doping concentration of Sr rose from 0.0 to 0.2, which means these stable LSMO nanoparticles have great potential for used as solar cells due to their lower transparency; we calculate their degree of transparency in the upcoming discussion. The elevation of the Fermi level was the result of introducing donor states with Strontium ion content; this also discussed, along with evidence of how much energy is required for the transition to the conduction band and additionally the radius of unoccupied or donor states, which helps us to understand their band structure. Generally, at a low value of x, all states are nondegenerate; whenever we introduce donor states via an extra holding charge carrier, i.e., Sr2+, the effect is to raise the Fermi level—not much in the case of degenerate states (Turky et al., 2017), but near the conduction band, this suppresses the optical band gap. The ionization energy required for the donor atom is given by Eq. 11 with some modifications (Matsuura, 2006):
where
The refractive index is an important parameter in the context of optical measurements that measures the degree of transparency as light passes through the sample material and can be expressed both by Moss and by Herve and Vandamme’s relation, as in Eqs 12, 13:
and
Here, A and B are numerical constants with respective ionization energies of 13.6 eV and 3.4 eV. From the data obtained for LSMO nanoparticles based on both relations, it can be noted that there is not much difference in the values of the refractive index. The trend in Table 4 shows that the refractive index increased as we raised the strontium content for x = 0.0 to 0.2. For the higher values of the x, the refractive index decreases, as reported by Sandhya Suresh et al. (Sandhya et al., 2023) in 2023, which means that, at low values, LSMO is more optically dense as compared to higher values. As explained earlier in XRD analysis, more defects/voids were observed with higher values of Sr in the crystal lattice, which causes more instability. According to Sandhya Suresh et al., (Sandhya et al., 2023), static and high-frequency dielectric constants play a significant role in optoelectronic devices, which can be expressed as:
where Eg and n represent the optical band gap and refractive index, respectively; these parameters will be discussed in the following section, and values are additionally presented in Table 4.
3.6 Frequency-dependent dielectric properties
In the preceding section, we have attempted to establish several critical relations correlated with optical behavior, mainly the static and high-frequency dielectric constant. In the present discussion, we extend this momentum to explain how the electric dipoles of La1-xSrxMnO3 for x = 0.0, 0.1, and 0.2 were altered in the presence of varying AC electric fields. To establish the dielectric spectrum, pellets measuring 12.7 mm in diameter with thickness 1.5 mm were made by crushing nanopowder at 10 tons for 10 min, such that these pellets served as electrodes, and samples were taken as the dielectric medium (Shah et al., 2012). An impedance analyzer (1 kHz–2 MHz) was utilized to record frequency-dependent dielectric responses at room temperature, including the dielectric constant
where t represents the thickness of the sample,
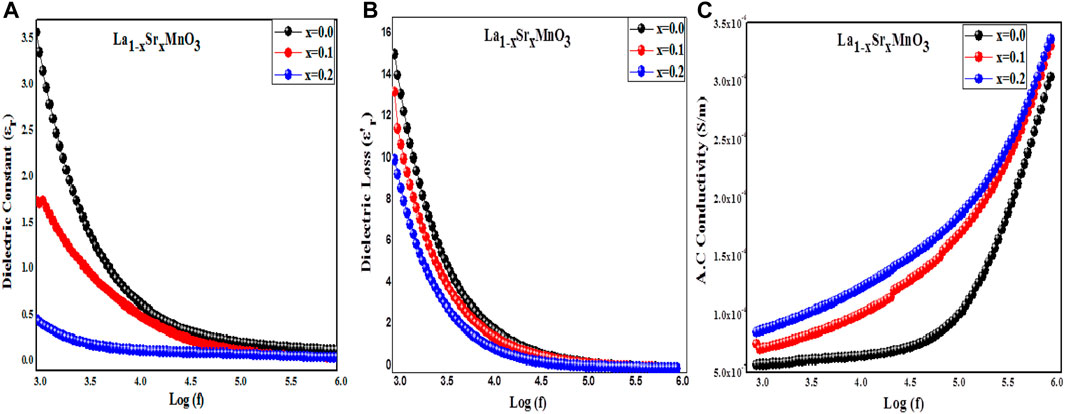
Figure 7. (A–C): The dielectric constant, dielectric loss, and (A, C) conductivity of La1-xSrxMnO3 (x = 0.0, 0.1, and 0.2).
For the frequency range of 1 kHz–2 MHz, Figure 7B displays the frequency response of the dielectric loss for ambient temperature La1-xSrxMnO3 nanoparticles (x = 0.0, 0.1, and 0.2), showing the dissipation of energy caused by defects/voids or dislocation density present in the prepared nanoparticles (Kamlesh et al., 2021b). In the region of low frequency, 3.0 log(f) to 5.0 log(f), an increment in the dielectric loss is indicated; this steadily decreases with increasing frequency (>5.0 log(f)), describing the polar nature of the samples. The
where E is the electric field vector,
Here
Figure 7C shows the AC conductivity of La1-xSrxMnO3 (x = 0.0, 0.1, and 0.2) perovskite nanoparticles as a function of frequency. The presence of frequency-dependent and -independent regions observed in this curve is due to the polar nature of dielectrics. In the region of low (3.0 log(f) to 4.0 log(f)) frequency, the fact that AC conductivity is lower may be attributed to high resistive grain boundaries that resist tunneling of charge carriers. Ahmad Gholizadeh et al. (Ahmad and Beyranvand, 2020) reported in their work that interfacial polarization is the reason for this low-conductivity behavior. Similarly, at high (>4.0 log(f)) frequencies, a significant increase in the conductivity was observed as a result of the smaller numbers of grains present in the reported samples (Amini and Ahmad, 2020). A notable relaxation was observed in pure samples, which might be due to the availability of fewer charge carriers as compared to doped samples, which exhibited relatively more conduction. The AC conductivity of the sample can be related to leakage current or angular frequency, as follows:
where
The flat response of the sample in the low-frequency range may be attributed to the DC component of conductivity. Low-frequency ion accumulation and low conductivity are the results of the electric field’s delayed periodic reversal. Johnscher’s universal power law (Chandekar et al., 2020b) provides the clearest description of frequency-dependent AC conductivity. The use of Sr2+ as a dopant plays a vital role in enhancing the AC conductivity of LSMO nanocrystals by introducing donor states with an excess of polarons; these reduce the defects/inhomogeneity and also the porosity of the material (Sandhya et al., 2023). Similarly, Ahmad Gholizadeh et al. (Amini and Ahmad, 2020) utilized different dopants in MFe12O19 (M = Ba, Pb, Sr) and examined frequency-dependent AC conductivity; they found that the strontium-doped sample exhibited higher conductivity due to highly conductive grains present in the sample. Eq. 20, which reflects the conduction process in La1-xSrxMnO3, attributes the defects in the host lattice caused by the partial replacement of the Sr2+ ion to charge transport, with hopping charged carriers over disordered arrangements:
where
4 Conclusion
1. In this study, optoelectrical properties as a function of different (0
2. Structural studies revealed a rhombohedral (R
3. Morphological and compositional analysis showed that the prepared samples had polygonal or hexagonal-like structures, with average particle or grain size estimated from SEM images in the range of 87–119 nm. Based on the EDX spectra, the purity or molar ratio of each composition in the compound was confirmed; additionally, bonds in the fingerprint region of 400–1,500 cm-1 were confirmed by FTIR analysis.
4 The optoelectrical properties of un-doped and Sr-doped samples indicated that the elevation of donor states near the conduction band suppressed the optical band gap from 4.34 eV to 4.11 eV, which was correlated with the refractive index. Moss’s relation showed increases in refractive index from 2.163 to 2.192, which means that the samples became more optically dense with higher Sr content. The static and frequency-dependent dielectric constant increased with an increase in Sr content, which means that the reported samples have the potential to serve in energy storage devices and high-frequency transmission cables due to their minimal dielectric losses. Finally, AC conductivity increased with an excess of polarons in the heavy-doped sample.
Based on the above points, we conclude that LSMO nanoparticles with low Sr content are more stable and represent a promising material for application in solid oxide fuel cells (SOFCs) and electronic storage devices.
Data availability statement
The original contributions presented in the study are included in the article/Supplementary material, further inquiries can be directed to the corresponding authors.
Author contributions
AA: Conceptualization, Data curation, Formal Analysis, Investigation, Methodology, Project administration, Resources, Software, Validation, Writing–original draft, Writing–review and editing. WS: Conceptualization, Methodology, Supervision, Writing–review and editing, Data curation, Formal Analysis, Investigation. ZU: Formal Analysis, Investigation, Writing–review and editing. SM: Conceptualization, Data curation, Investigation, Writing–review and editing. MR: Formal Analysis, Investigation, Methodology, Writing–review and editing. SA: Writing–review and editing, Data curation, Methodology, Validation, Visualization, Software. NI: Investigation, Writing–review and editing. HA: Writing–review and editing, Data curation, Validation, Visualization, Software.
Funding
The author(s) declare that financial support was received for the research, authorship, and/or publication of this article. This Project is funded by King Saud University, Riyadh, Saudi Arabia.
Conflict of interest
The authors declare that the research was conducted in the absence of any commercial or financial relationships that could be construed as a potential conflict of interest.
Publisher’s note
All claims expressed in this article are solely those of the authors and do not necessarily represent those of their affiliated organizations, or those of the publisher, the editors and the reviewers. Any product that may be evaluated in this article, or claim that may be made by its manufacturer, is not guaranteed or endorsed by the publisher.
References
Afify, M. S., El Faham, M. M., Eldemerdash, U., El Rouby, W. M., and El-Dek, S. (2021). Room temperature ferromagnetism in Ag doped LaMnO3 nanoparticles. J. alloys Compd. 861 (2021), 158570. doi:10.1016/j.jallcom.2020.158570
Ahmad, G., and Beyranvand, M. (2020). Investigation on the structural, magnetic, dielectric and impedance analysis of Mg0.3-xBaxCu0.2Zn0.5Fe2O4 nanoparticles. Phys. B 584, 412079. doi:10.1016/j.physb.2020.412079
Ali, A., Shah, W. H., Safeen, A., Ali, L., Tufail, M., Ullah, Z., et al. (2023a). Effect of Ca doping on the arbitrary canting of magnetic exchange interactions in La1-xCaxMnO3 nanoparticles. Front. Mater.10 (2023), 1117793. doi:10.3389/fmats.2023.1117793
Ali, A. H., Ahmed, A. M., Abdelhamied, M. M., Abdel-Khaliek, A. A., Khalik, S. A. E., Abass, S. M., et al. (2023b). Synthesis of lead-free Cu/CuFeO 2/CZTS thin film as a novel photocatalytic hydrogen generator from wastewater and solar cell applications. doi:10.21203/rs.3.rs-3304698/v1
Amini, M., and Ahmad, G. (2020). Shape control and associated magnetic and dielectric properties of MFe12O19 (M = Ba, Pb, Sr) hexaferrites. J. Phys. Chem. Solids 147, 109660. doi:10.1016/j.jpcs.2020.109660
Arshad, M., Huiling, D., Sufyan, J. M., Maqsood, A., Ashraf, I., Hussain, S., et al. (2020). Fabrication, structure, and frequency-dependent electrical and dielectric properties of Sr doped BaTiO3 ceramics. Ceram. Int. 46 (2), 2238–2246. doi:10.1016/j.ceramint.2019.09.208
Assoudi, N., Hzez, W., Dhahri, R., Walha, I., Rahmouni, H., Khirouni, K., et al. (2018). Physical properties of Ag/Ca doped Lantanium manganite. J. Mater. Sci. Mater. Electron. 29, 20113–20121. doi:10.1007/s10854-018-0143-5
Ben, A., Alkallas, F. H., Chandekar, K. V., Kumar, A., Ubaidullah, M., Shkir, M., et al. (2023). Facile low temperature development of Ag-doped PbS nanoparticles for optoelectronic applications. Mater. Chem. Phys. 297, 127299. doi:10.1016/j.matchemphys.2023.127299
Chandekar, K. V., Khan, A., Alshahrani, T., Shkir, M., Kumar, A., El-Toni, A. M., et al. (2020a). Novel rare earth Dy doping impact on physical properties of PbI2 nanostructures synthesized by microwave route for optoelectronics. Mater. Charact. 170, 110688. doi:10.1016/j.matchar.2020.110688
Chandekar, K. V., Shkir, M., Khan, A., and AlFaify, S. (2020b). An in-depth study on physical properties of facilely synthesized Dy@CdS NPs through microwave route for optoelectronic technology. Mater. Sci. Semicond. Process. 118, 105184. doi:10.1016/j.mssp.2020.105184
Chandekar, K. V., Shkir, M., Khan, A., Sayed, M., Alotaibi, N., Alshahrani, T., et al. (2021b). Significant and systematic impact of yttrium doping on physical properties of nickel oxide nanoparticles for optoelectronics applications. J. Mater. Res. Technol. 15, 2584–2600. e260. doi:10.1016/j.jmrt.2021.09.072
Eghdami, F., and Ahmad, G. (2023). A correlation between microstructural and impedance properties of MnFe2-Co O4 nanoparticles. Phys. B 650, 414551. doi:10.1016/j.physb.2022.414551
Flores-Lasluisa, J. X., Huerta, F., Cazorla-Amorós, D., and Morallón, E. (2022). Manganese oxides/LaMnO3 perovskite materials and their application in the oxygen reduction reaction. Energy 247 (2022), 123456. doi:10.1016/j.energy.2022.123456
Gholizadeh, A., and Banihashemi, V. (2021). Effects of Ca–Gd co-substitution on the structural, magnetic, and dielectric properties of M-type strontium hexaferrit. doi:10.1111/jace.1919
Gholizadeh, A., and Tajabor, N. (2010). Influence of N2- and Ar-ambient annealing on the physical properties of SnO2:Co transparent conducting films. Mater. Sci. Semicond. process. 13 (3), 162–166. doi:10.1016/j.mssp.2010.10.004
Gouider Trabelsi, A. B., Chandekar, K. V., Alkallas, F., Ashraf, I., Hakami, J., Shkir, M., et al. (2022). A comprehensive study on Co-doped CdS nanostructured films fit for optoelectronic applications. J. Mater. Res. Technol. 21, 3982–4001. e4001. doi:10.1016/j.jmrt.2022.11.002
Hassan, A. A. S., Khan, W., Husain, S., Dhiman, P., and Singh, M. (2020). Investigation of structural, optical, electrical, and magnetic properties of Fe-doped La0. 7Sr0. 3MnO3 manganites. Int. J. Appl. Ceram. Technol. 17 (5), 2430–2438. doi:10.1111/ijac.13540
Hernández, E., Sagredo, V., and Delgado, G. E. (2015). Synthesis and magnetic characterization of LaMnO3 nanoparticles. Rev. Mex. física 61 (3), 166–169.
Hizi, W., Gassoumi, M., Rahmouni, H., Guesmi, A., Ben Hamadi, N., and Dhahri, E. (2022). Effect of sintering temperature and polarization on the dielectric and electrical properties of La0. 9Sr0. 1MnO3 manganite in alternating current. Materials 15 (10), 3683. doi:10.3390/ma15103683
Hu, J., Zhang, L., Lu, B., Wang, X., and Huang, H. (2019). LaMnO3 nanoparticles supported on N doped porous carbon as efficient photocatalyst. Vacuum 159, 59–68. doi:10.1016/j.vacuum.2018.10.021
Jadhav, S. V., Nikam, D., Khot, V., Mali, S., Hong, C., and Pawar, S. (2015). PVA and PEG functionalised LSMO nanoparticles for magnetic fluid hyperthermia application. Mater. Charact. 102, 209–220. doi:10.1016/j.matchar.2015.03.001
Jheng-Yun, L., Hong-Kai, C., Cheng-Che, H., and Jian-Zhang, C. (2023). LiMn2O4 Li-ion hybrid supercapacitors processed by nitrogen atmospheric-pressure plasma jet. Ceram. Int. 49, 11067–11107. doi:10.1016/j.ceramint.2022.11.303
Kamlesh, V. C., Alshahrani, T., Ben Gouider Trabelsi, A., Alkallas, F. H., Shkir, M., and AlFaify, S. (2021a). Novel rare earth yttrium doping effect on physical properties of PbS nanostructures: facile synthesis and characterization. J. Mater Sci. 56, 4763–4781. doi:10.1007/s10853-020-05539-w
Kamlesh, V. C., Shkir, M., Palanivel, B., Ahmad, Z., Algarni, H., and AlFaify, S. (2021b). Comparative study of Pr-doped and undoped PbS nanostructures facilely synthesized for optoelectronic applications. Solid State Sci. 122, 106773. doi:10.1016/j.solidstatesciences.2021.106773
Li, C., Yu, Z., Liu, H., and Chen, K. (2018). High surface area LaMnO3 nanoparticles enhancing electrochemical catalytic activity for rechargeable lithium-air batteries. J. Phys. Chem. Solids 113, 151–156. doi:10.1016/j.jpcs.2017.10.039
Manohar, A., and Krishnamoorthi, C. (2017). Structural, optical, dielectric and magnetic properties of CaFe2O4 nanocrystals prepared by solvothermal reflux method. J. Alloys Compd. 722, 818–827. doi:10.1016/j.jallcom.2017.06.145
Manohar, A., Krishnamoorthi, C., Naidu, K. C. B., and Pavithra, C. (2019). Dielectric, magnetic hyperthermia, and photocatalytic properties of ZnFe 2 O 4 nanoparticles synthesized by solvothermal reflux method. Appl. Phys. A 125, 477–510. doi:10.1007/s00339-019-2760-0
Matsuura, H. (2006). Influence of excited states of a deep substitutional dopant on majority-carrier concentration in semiconductors. Phys. Rev. B 74, 245216. doi:10.1103/PhysRevB.74.245216
McBride, K., Cook, J., Gray, S., Felton, S., Stella, L., and Poulidi, D. (2016). Evaluation of La 1− x Sr x MnO 3 (0≤ x< 0.4) synthesised via a modified sol–gel method as mediators for magnetic fluid hyperthermia. CrystEngComm 18 (3), 407–416. doi:10.1039/c5ce01890k
Miao, He, Wu, X., Chen, B., Wang, Q., Wang, F., Wang, J., et al. (2020). A-site deficient/excessive effects of LaMnO3 perovskite as bifunctional oxygen catalyst for zinc-air batteries. Electrochimica Acta 333, 135566. doi:10.1016/j.electacta.2019.135566
Mleiki, A., Khlifi, A., Rahmouni, H., Guermazi, N., Khirouni, K., Hlil, E., et al. (2020). Magnetic and dielectric properties of Ba-lacunar La0. 5Eu0. 2Ba0. 3MnO3 manganites synthesized using sol-gel method under different sintering temperatures. J. Magnetism Magnetic Mater. 502, 166571. doi:10.1016/j.jmmm.2020.166571
Mohanty, D., Mallick, P., Biswal, S., Behera, B., Mohapatra, R. K., Behera, A., et al. (2020b). Investigation of structural, dielectric and electrical properties of ZnFe2O4 composite. Mater. Today Proc. 33, 4971–4975. doi:10.1016/j.matpr.2020.02.827
Mohanty, D., Satpathy, S. K., Behera, B., and Mohapatra, R. K. (2020a). Dielectric and frequency dependent transport properties in magnesium doped CuFe2O4 composite. Mater. Today Proc. 33 (2020), 5226–5231. doi:10.1016/j.matpr.2020.02.944
Mojahed, M., Dizaji, H. R., and Gholizadeh, A. (2022). Structural, magnetic, and dielectric properties of Ni/Zn co-substituted CuFe2O4 nanoparticles. Phys. B 646, 414337. doi:10.1016/j.physb.2022.414337
Naseem Siddique, M., Ahmed, A., and Tripathi, P. (2019). Enhanced optical properties of pure and Sr doped NiO nanostructures: a comprehensive study. Optik 185, 599–608. doi:10.1016/j.ijleo.2019.03.150
Özkan, Ç., Türk, A., and Celik, E. (2021). Synthesis and characterizations of LaMnO3 perovskite powders using sol–gel method. J. Mater. Sci. Mater. Electron. 32 (11), 15544–15562. doi:10.1007/s10854-021-06104-0
Sandhya, S., Vindhya, P. S., Devika, S., and Kavitha, V. T. (2023). structural, optical and dielectric properties of nanostructured La1− xSrxMnO3 perovskites. Mater. Today Commun. 36, 106657. doi:10.1016/j.mtcomm.2023.106657
Shafi, P. M., Joseph, N., Thirumurugan, A., and Bose, A. C. (2018). Enhanced electrochemical performances of agglomeration-free LaMnO3 perovskite nanoparticles and achieving high energy and power densities with symmetric supercapacitor design. Chem. Eng. J. 338, 147–156. doi:10.1016/j.cej.2018.01.022
Shah, W. H., Safeen, K., and Rehman, G. (2012). Effects of divalent alkaline earth ions on the magnetic and transport features of La0. 65A0. 35Mn0. 95Fe0. 05O3 (A= Ca, Sr, Pb, Ba) compounds. Curr. Appl. Phys. 12 (3), 742–747. doi:10.1016/j.cap.2011.10.015
Shkir, M., Chandekar, K. V., Alshahrani, T., Kumar, A., Khan, A., and AlFaify, S. (2020b). An impact of La doping content on key physical properties of PbS spherical nanoparticles facilely synthesized via low temperature chemical route. Eur. Phys. J. Plus 135, 816. doi:10.1140/epjp/s13360-020-00740-x
Shkir, M., Chandekar, K. V., Khan, A., Alshahrani, T., Mohamed El-Toni, A., Sayed, M., et al. (2021a). Tailoring the structure-morphology-vibrational-optical-dielectric and electrical characteristics of Ce@NiO NPs produced by facile combustion route for optoelectronics. Mater. Sci. Semicond. Process. 126, 105647. doi:10.1016/j.mssp.2020.105647
Shkir, M., Khan, Z. R., Chandekar, K. V., Alshahrani, T., Kumar, A., and AlFaify, S. (2020a). A facile microwave synthesis of Cr-doped CdS QDs and investigation of their physical properties for optoelectronic applications. Appl. Nanosci. 10, 3973–3985. doi:10.1007/s13204-020-01505-9
Singh, K. P., and Prasad, A. (2015). Effects of Sr2+ doping on the electrical properties of (Bi0. 5Na0. 5) 0.94 Ba0. 06TiO3 ceramics. Process. Appl. Ceram. 9 (1), 33–42. doi:10.2298/pac1501033s
Solopan, S. O., V’yunov, O., Belous, A., Polek, T., and Tovstolytkin, A. (2012). Effect of nanoparticles agglomeration on electrical properties of La1− xAxMnO3 (A= Sr, Ba) nanopowder and ceramic solid solutions. Solid state Sci. 14 (4), 501–505. doi:10.1016/j.solidstatesciences.2012.01.030
Song, G., Zhang, Y., Li, S., Yang, J., Bai, W., and Tang, X. (2021). Dielectric relaxation behavior of BTO/LSMO heterojunction. Nanomaterials 11 (5), 1109. doi:10.3390/nano11051109
Sun, X., Li, X., Hou, S., Huang, C., Zou, J., Li, M., et al. (2014). The effect of Mg doping on the dielectric and tunable properties of Pb0. 3Sr0. 7TiO3 thin films prepared by sol–gel method. Appl. Phys. A 114 (3), 777–783. doi:10.1007/s00339-013-7645-z
Tarnack, M., Czaderna-Lekka, A., Wojnarowska, Z., Kaminski, K., and Paluch, M. (2023). Nature of dielectric response of phenyl alcohols. J. Phys. Chem. B 127, 6191–6196. doi:10.1021/acs.jpcb.3c02335
Turky, A. O., and Kandil, A. T. (2013). Optical and electrical properties of Ba1−x Sr x TiO3 nanopowders at different Sr2+ ion content. J. Mater Sci. Mater Electron 24, 3284–3291. doi:10.1007/s10854-013-1244-9
Turky, A. O., Rashad, M. M., Hassan, A. M., Elnaggar, E. M., and Bechelany, M. (2016). Tailoring optical, magnetic and electric behavior of lanthanum strontium manganite La 1− x Sr x MnO 3 (LSM) nanopowders prepared via a co-precipitation method with different Sr 2+ ion contents. RSC Adv. 6 (22), 17980–17986. doi:10.1039/c5ra27461c
Turky, A. O., Rashad, M. M., Hassan, A. M., Elnaggar, E. M., and Bechelany, M. (2017). Optical, electrical and magnetic properties of lanthanum strontium manganite La 1− x Sr x MnO 3 synthesized through the citrate combustion method. Phys. Chem. Chem. Phys. 19 (9), 6878–6886. doi:10.1039/c6cp07333f
Wang, H., Ling, F., Li, Y., Wu, D., Qi, L., Liang, Z., et al. (2022). Effect of V doping on the electrical transport and magnetoresistance properties of La0. 825Sr0. 175MnO3 ceramics. J. Sol-Gel Sci. Technol. 103 (3), 799–806. doi:10.1007/s10971-022-05870-5
Yan, Z., Liu, H., Hao, Z., Meng, Yu, Chen, X., and Chen, J. (2020). Electrodeposition of (hydro) oxides for an oxygen evolution electrode. Chem. Sci. 11, 10614–10625. doi:10.1039/d0sc01532f
Zhong, W., Au, C.-T., and Du, Y.-W. (2013). Review of magnetocaloric effect in perovskite-type oxides. Chin. Phys. B 22, 057501, 057501. doi:10.1088/1674-1056/22/5/057501
Zhoua, H., Kea, J., Xua, D., and Liub, J. (2023). MnWO4 nanorods embedded into amorphous MoS microsheets in 2D/1D MoS/MnWO4 S–scheme heterojunction for visible-light photocatalytic water oxidation. J. Mater. Sci. Technol. 136, 169–179. doi:10.1016/j.jmst.2022.07.021
Keywords: LSMO nanoparticles, co-precipitation, optical band gap, dielectric constant, dielectric loss, ac conductivity
Citation: Ali A, Shah WH, Ullah Z, Malik S, Rauf M, Askar S, Imran N and Ahmad H (2024) Narrowing of band gap and decrease in dielectric loss in La1-xSrxMnO3 for x = 0.0, 0.1, and 0.2 manganite nanoparticles. Front. Mater. 11:1369122. doi: 10.3389/fmats.2024.1369122
Received: 11 January 2024; Accepted: 20 February 2024;
Published: 26 March 2024.
Edited by:
Ehsan Nazarzadeh Zare, Damghan University, IranCopyright © 2024 Ali, Shah, Ullah, Malik, Rauf, Askar, Imran and Ahmad. This is an open-access article distributed under the terms of the Creative Commons Attribution License (CC BY). The use, distribution or reproduction in other forums is permitted, provided the original author(s) and the copyright owner(s) are credited and that the original publication in this journal is cited, in accordance with accepted academic practice. No use, distribution or reproduction is permitted which does not comply with these terms.
*Correspondence: Wiqar H. Shah, d2lxYXIuaHVzc2FpbkBpaXUuZWR1LnBr; Akhtar Ali, YWtodGFyaGl0ZWNAZ21haWwuY29t; Sameh Askar, c2Fza2FyQGtzdS5lZHUuc2E=