- 1State Key Laboratory of Organic-Inorganic Composites, Beijing University of Chemical Technology, Beijing, China
- 2Key Laboratory of Biomedical Materials of Natural Macromolecules, Beijing University of Chemical Technology, Ministry of Education, Beijing, China
- 3Beijing Laboratory of Biomedical Materials, Beijing University of Chemical Technology, Beijing, China
- 4Institute of Emergent Elastomers, School of Materials Science and Engineering, South China University of Technology, Guangzhou, Guangdong, China
Developing eco-friendly polymeric plasticizers with excellent migration resistance is one of the research hotspots in the polyvinyl chloride (PVC) industry. A low-molecular-weight biobased polyester rubber (LMW-BPR) was synthesized from five biobased polyester monomers in a 100-L reactor and evaluated as a potential eco-friendly polymeric plasticizer for PVC. The obtained LMW-BPR is an amorphous polyester material with a low glass transition temperature of −48°C and a molecular weight of 22 kg/mol, which is lower than that of existing polyester rubber but higher than those of most polyester plasticizer commodities. Plasticized PVC composites with a total plasticizer content of 50 phr were prepared by using the mixture of LMW-BPR and di-isononyl cyclohexane-1,2-dicarboxylate (DINCH, an eco-friendly monomeric plasticizer commodity) as the plasticizer. The migration resistance test showed that the migration loss of plasticizer in plasticized PVC composite decreased significantly with the increase of LMW-BPR content. When the content of LMW-BPR reaches 30 phr, the plasticized PVC composites are almost nonmigratory. In addition, compared with PVC composite plasticized by pure DINCH, co-plasticized PVC composites containing LMW-BPR exhibit higher tensile strength and thermal stability, and their flexibility, low-temperature resistance and biocompatibility are also maintained at a similar level to the former. Overall, LMW-BPR is an effective eco-friendly polymeric plasticizer for PVC and also sustainable and scalable, thus it is worthy of wide application.
1 Introduction
Plasticizer is one of the largest polymer auxiliaries produced and consumed in the world, with a market volume of nearly 10 million metric tons, of which the soft polyvinyl chloride (PVC) industry accounts for about 90% (Ledniowska et al., 2022). PVC plasticizer commodities are varied, of which phthalates [e.g., di (2-ethylhexyl) phthalate-DEHP] are the most consumed, accounting for nearly 90% of the total. Unfortunately, some studies have shown that phthalates migrate easily and that phthalates migrating from PVC products can cause toxic effects on living organisms’ hypophysis cerebri, liver or genital system, and increase the incidence of some inflammatory diseases and even cancer (Agarwal et al., 1989; Dirven et al., 1990; Kolarik et al., 2008; Cao et al., 2019; Ito et al., 2019; Yu et al., 2019). Therefore, in recent years, some countries have enacted bills (e.g., the EU REACH Act) to prohibit the use of phthalates in some PVC products that are frequently contacted by humans, such as medical devices, food packaging or children’s toys. In this context, non-toxic and eco-friendly plasticizers such as di-isononyl cyclohexane-1,2-dicarboxylate (DINCH) and tri-octyl trimellitate (TOTM) have been widely used (Chiellini et al., 2013; Bourdeaux et al., 2016; Tortolano et al., 2018). However, the nature of small molecules makes these eco-friendly plasticizer commodities easy to migrate out of PVC products, which in turn affects the serviceability of the products and can cause contamination (Rahman and Brazel, 2004; Yu et al., 2008). Therefore, it is important to develop eco-friendly plasticizers that are resistant to migration (Jia et al., 2021; Ma et al., 2022; Han et al., 2024).
Substituting small molecular plasticizers with polymeric plasticizers has proven to be an effective strategy for inhibiting plasticizer migration in PVC products (Hakkarainen, 2008). Among the existing polymeric PVC plasticizers, nontoxic and eco-friendly polyester plasticizers are the most representative (Rusu et al., 2006; Lindström and Hakkarainen, 2007; Sin et al., 2012; Xie et al., 2014; Chuayjuljit et al., 2015; Zhao et al., 2015; Gao et al., 2018; Tan et al., 2019), with a history of use dating back to the 1940s (Rushton and Salomons, 1969). The high molecular weights of polyester plasticizer commodities (typically less than 10 kg/mol) give them excellent migration resistance, but they may still migrate in service in some harsh environments (Miao et al., 2017). Further molecular weight improvement may be an effective strategy to enhance the migration resistance of polyester plasticizers (Sun et al., 2019). However, for most semi-crystalline polyester plasticizers without additional modification, the macromolecular crystal region will affect the plasticizing efficiency and flexibility of soft PVC materials at high plasticizer filling volume, thus limiting their application scope (Lindstrom and Hakkarainen, 2006; Yin and Hakkarainen, 2011; Xu et al., 2020). To obtain excellent migration resistance while avoiding crystallization, developing high-molecular-weight amorphous polyester plasticizers may be an effective route.
Biobased polyester rubber (BPR), an innovative synthetic rubber that is both biosourced and biodegradable, not only has an amorphous polyester structure but also has an adjustable molecular weight (Zhang et al., 2021; Tang et al., 2022). In 2012, our laboratory developed and reported the first generation of BPR, namely poly (propanediol/butanediol/succinate/itaconate/sebacate) (Wei et al., 2012). Subsequently, a variety of high-performance BPR nanocomposites (Yang et al., 2012; Yang et al., 2013; Gao et al., 2016) and high-toughness polylactic acid/BPR composites (Kang et al., 2013) were developed by using high-molecular-weight BPR (HMW-BPR, M > 30 kg/mol). However, the development of application technology for low-molecular-weight BPR (LMW-BPR, M ≤ 30 kg/mol) is still blank. Herein, we synthesized LMW-BPR with a molecular weight of about 20 kg/mol as a high-molecular-weight amorphous plasticizer and blended it with DINCH in different ratios to evaluate its application potential as a migration-resistant and eco-friendly PVC plasticizer.
2 Materials and methods
2.1 Materials
Biobased 1,3-propanediol (≥99.5%, PDO) was provided by Suzhou Suzhen Bioengineering Co., Ltd. Biobased 1,4-butanediol (≥99.5%, BDO) was provided by Yuanli Chemical Group Co., Ltd. Biobased succinic acid (≥99.5%, SuA) was provided by Shandong Land Biological Technology Co., Ltd. Biobased sebacic acid (≥99.5%, SeA) was purchased from Shanghai Zhongshen Chemical Co., Ltd. Bioabsed itaconic acid (≥99.0%, IA) was purchased from Qingdao Langyatai Group Co., Ltd. Hydroquinone (≥99.0%), phosphorous acid (≥99.0%), and titanium (Ⅳ) n-butoxide (≥99%, TBT) were purchased from Alfa Aesar. PVC resin (S-70) was purchased from Formosa Group (Taiwan). Diisononyl 1,2-cyclohexane-dicarboxylate (DINCH) was purchased from BASF. Ca-Zn thermal stabilizer was purchased from Shandong Wantu Polymer Material Corporation Limited. Ethanol (AR) and petroleum ether (AR) were purchased from Beijing Chemical Plant. All chemicals were used as received without further purification.
2.2 Synthesis of low-molecular-weight biobased polyester rubber
The selected biobased polyester rubber (BPR) in this work is a fully biobased amorphous copolyester synthesized from biobased PDO, BDO, SuA, IA and SeA, and its synthesis pathway is the same as that reported in our previous work (Wei et al., 2012). However, to obtain a product suitable for use as a plasticizer, a low-molecular-weight BPR product was obtained by controlling the polycondensation time. The synthesis experiment was carried out on a 100-L esterification-polycondensation reactor in the following steps: (a) First, five stoichiometric biobased monomers, including PDO (10.8 kg), BDO (12.7 kg), SuA (19.1 kg), IA (3.3 kg) and SeA (14.0 kg), as well as the antioxidant phosphorous acid (0.01 wt% relative to all monomers) and the inhibitor hydroquinone (0.04 wt% relative to all monomers) were added into the reactor and esterified at 180°C for 2 h to obtain esterification product; (b) Then, the polycondensation reaction was performed at 220°C for 3 h under reduced pressure with TBT (0.05 wt% relative to all monomers) as catalyst; (c) Last, the polycondensation product was discharged and cooled to room temperature to obtain the target product, namely LMW-BPR.
2.3 Preparation of plasticized PVC sheets
In this work, using LMW-BPR and/or DINCH as the plasticizer, six plasticized PVC samples were prepared and named PVC/LMW-BPR-x, where x denotes the amount of LMW-BPR. As shown in Table 1, the formula for the preparation of PVC/LMW-BPR samples was demonstrated, and the preparation process was as follows: (a) First, the pre-mixed mixture containing PVC resin, mixed plasticizer and Ca-Zn stabilizer was added to a two-roll mill and processed at 160°C for 15 min; (b) Then, the cooled mixture was added to the mold and hot-pressed in a curing press for 5 min at 175°C and 10 MPa; (c) Last, the sample was transferred to a cold press and cold-pressed at 10 MPa for 5 min to obtain the target sheet with 2-mm thick.
2.4 Characterization
The molecular weight of the LMW-BPR plasticizer was determined by gel permeation chromatography (GPC) in tetrahydrofuran on a Waters Breeze instrument. Fourier transform infrared spectroscopy (FTIR) spectrum of LMW-BPR was recorded on a Bruker Tensor 27 spectrometer in the wavenumber ranging from 4,000 to 500 cm−1 at a resolution of 4 cm−1. Nuclear magnetic resonance (NMR) spectrum of LMW-BPR was recorded on a Bruker AV600 spectrometer, and the solvent was CDCl3. Differential scanning calorimetry (DSC) measurements were performed with a Mettler-Toledo DSC instrument under nitrogen. The testing process was as follows: (a) Heating from 25°C to 160°C and then keeping at 160°C for 5 min; (b) Cooling from 160°C to −100°C and then keeping at −100°C for 10 min; (c) Reheating from −100°C to 160°C. The rate of temperature change was 10 °C/min for all stages. Thermogravimetric analysis (TGA) measurements were carried out on a Mettler-Toledo TGA/DSC1 STARe system under nitrogen, and a heating rate of 10 °C/min was used. The low-temperature resistance test of plasticized PVC was carried out on a rubber/plastic low-temperature brittleness tester according to GB/T 1682-2014. Tensile test was carried out on a CMT 4104 Electrical Tensile Instrument according to GB/T 1040.2-2022, and a crosshead speed of 50 mm/min was used.
Migration resistance test was carried out as follows: (a) First, the plasticized PVC sheet was cut into 10 mm × 10 mm × 2 mm specimen and the initial mass was recorded as m0; (b) Then, the specimen was fully immersed in ethanol or petroleum ether at 25°C for 96 h; (c) Last, the immersed specimen was taken out and dried to a constant mass, which was recorded as m1. The migration loss rate of plasticizers in PVC materials can calculated according to Eq. 1:
The cytotoxicity of plasticized PVC was evaluated by MTT [3-(4,5-dimethylthiazol-2-yl)-2,5-diphenyltetrazolium bromide] assay (Xue et al., 2015). The samples for test were cut into slices of similar size (3 cm × 1 cm × 2 mm) and sterilized by washing three times with a 75% (v/v) ethanol solution in sterilized water and exposed to Co60 for 15 min. After sterilization, the samples were incubated in Dulbecco’s modified Eagle’s medium (DMEM) at a proportion of 3 cm2/mL for 24 h at 37°C. The extract solution was then filtered (0.22 μm pore size) to eliminate any solid particles of the material. MC3T3-E1 cells were cultured in DMEM supplemented with 10% (v/v) fetal bovine serum (FBS) at a density of 4.0 × 104 cells/mL, and 100 μL of cell resuspension solution was pipetted into 96-well micrometre plates. After incubation at 37°C under 5% of CO2 atmosphere for 24 h, the medium was replaced by the previously prepared extracted dilutions, with the culture medium as blank control and dimethyl sulfoxide (DMSO) as negative control. After 24, 48, and 72 h of incubation with the samples, the morphologies of the cells in the plate were observed by using an inverted phase contrast microscope. The cells were treated with 20 μL/well MTT (5 mg/mL in PBS solution) and incubated for another 4 h at 37°C in a humidified atmosphere of 5% CO2. Then the culture medium was removed, and 200 μL/well of DMSO was added to dissolve the formed formazan crystals. After the plate was shaken for 15 min, the optical density (O.D.) was read on a multiwell microplate reader at 630 nm. The cytotoxicity for each membrane was tested by six averages of extract substrate. The viability percentage was expressed as the relative growth rate (RGR) by Eq. 2:
where O.D.Sample, O.D.DMSO and O.D.Control are the O.D. of the cells incubated in the samples’ extract solution, the DMSO, and the blank control.
3 Results and discussion
3.1 Structure and properties of LMW-BPR plasticizer
In this work, the LMW-BPR plasticizer was synthesized from biobased 1,3-propanediol, 1,4-butanediol, succinic acid, itaconic acid and sebacic acid in a 100-L reactor. The number average molecular weight of LMW-BPR measured by GPC is 22 kg/mol, which is smaller than that of BPR for rubber nanocomposites (>30 kg/mol), but significantly higher than that of most plasticizer commodities for PVC (<10 kg/mol). As shown in Figure 1A, the molecular structure of LMW-BPR is demonstrated, and the characteristic absorption peaks of each group it contains can be found in the FTIR spectrum shown in Figure 1B. The absorption peaks at 2,931 cm−1 and 2,859 cm−1 are assigned to the symmetrical and the antisymmetric stretching vibration of methylene (-CH2-). The intense absorption peak at 1732 cm−1 is associated with the stretching vibration of the carbonyl (C = O) in ester bond. The weak absorption peak at 1,645 cm−1 is assigned to the stretching vibration of the C = C group in itaconic acid unit. The strong absorption peak at 1,159 cm−1 is associated with the stretching vibration of C-O-C = O groups in ester bonds.
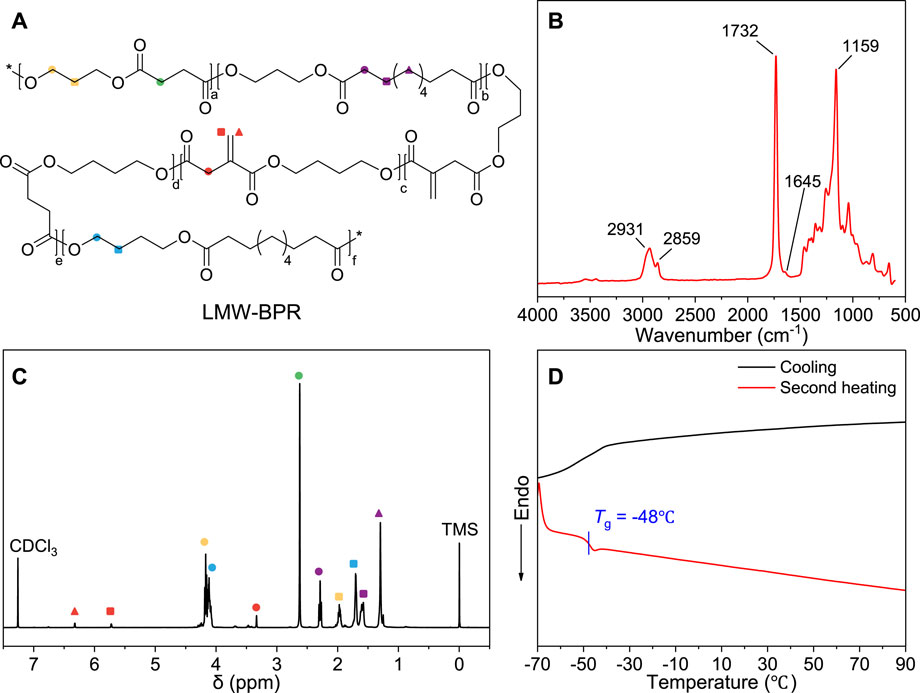
Figure 1. Structure and properties of LMW-BPR plasticizer. (A) Molecular structure; (B) FTIR spectrum; (C) 1H-NMR spectrum; (D) DSC curves.
The microstructure of LMW-BPR plasticizer was also further characterized through NMR. As shown in Figure 1C, the characteristic peaks of different hydrogen atoms in each monomer unit of LMW-BPR can be found in the 1H-NMR spectrum. The signals at 4.17 ppm and 1.98 ppm are respectively attributed to -O-CH2- and -O-CH2-CH2- in PDO unit. The signals at 4.12 ppm and 1.61 ppm are respectively attributed to -O-CH2- and -O-CH2-CH2- in BDO unit. The signal at 2.63 ppm belongs to the methylene (-CH2-COO-) in SuA unit. The signals at 6.32 ppm and 5.73 ppm are attributed to -CO-C (=CH2)-CH2- in IA unit, and the signal at 3.34 ppm belongs to -CO-C (=CH2)-CH2- in IA unit. The signals at 2.29 ppm, 1.71 ppm and 1.30 ppm correspond respectively to the three characteristic hydrogen atoms in the SeA unit.
The crystalline property of LMW-BPR was characterized by DSC. As shown in Figure 1D, LMW-BPR does not show any crystallization and melt transition during cooling and heating. This proves that it is an amorphous copolyester rubber. In addition, LMW-BPR also has a low glass transition temperature (Tg) and therefore has the potential to be used as a novel PVC plasticizer with good cold resistance. Overall, we obtained a novel fully biobased polyester plasticizer with the expected microstructure, low Tg and amorphous condensed structure.
3.2 Migration resistance of plasticizers in PVC materials
As shown in Figure 2, the migration loss rates of plasticizers in PVC materials after 96 h immersion in ethanol and petroleum ether at room temperature are demonstrated, respectively. The results showed that DINCH migrates in both solvent environments and has a higher migration loss rate in non-polar petroleum ether. However, there was no migration loss of LMW-BPR plasticizer in both solvent environments. This is mainly because LMW-BPR has a much higher molecular weight than DINCH and thus has higher migration resistance in the PVC matrix. When LMW-BPR was used in combination with DINCH to plasticize PVC, the migration loss rate of plasticizer (namely DINCH) decreased dramatically with the increase of LMW-BPR amount. When the amount of LMW-BPR was higher than 30 phr, there was almost no plasticizer loss in PVC materials. This indicates that the introduction of LMW-BPR can inhibit the migration of DINCH. The main reason is that the ester group in LMW-BPR can interact with that in DINCH, thus hindering the migration of DINCH from the PVC matrix. Overall, LMW-BPR is a novel plasticizer with excellent migration resistance and can inhibit the migration of the small-molecule DINCH.
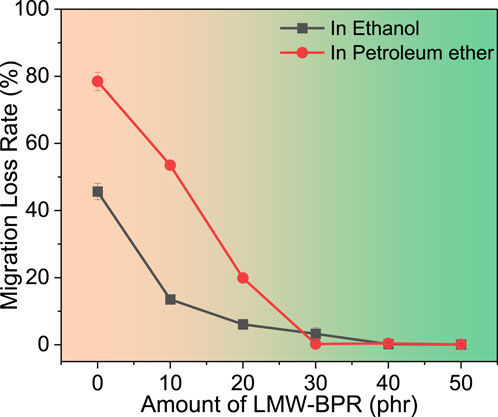
Figure 2. Migration loss rates of plasticizers in PVC materials after 96 h immersion in ethanol and petroleum ether.
3.3 Mechanical properties of PVC materials
As shown in Figure 3, the stress-strain curves of the plasticized PVC materials, as well as the line charts of their tensile strength and elongation at break as a function of LMW-BPR amount are demonstrated. The results showed that using LMW-BPR plasticizer to partially or completely replace DINCH for plasticizing PVC resin, the elongation at break of the resulting plasticized PVC materials remained at a high level (∼300%), which is comparable to that of PVC material plasticized by pure DINCH. This proves that LMW-BPR is a promising and efficient plasticizer for PVC. Note that the tensile strength of the plasticized PVC material gradually increased with increasing LMW-BPR substitution. The main reason for this is that the ester group content of LMW-BPR is higher than that of DINCH, and therefore the total interaction between the ester group and the chlorine atom in the plasticized PVC is enhanced with the increase of LMW-BPR substitution.
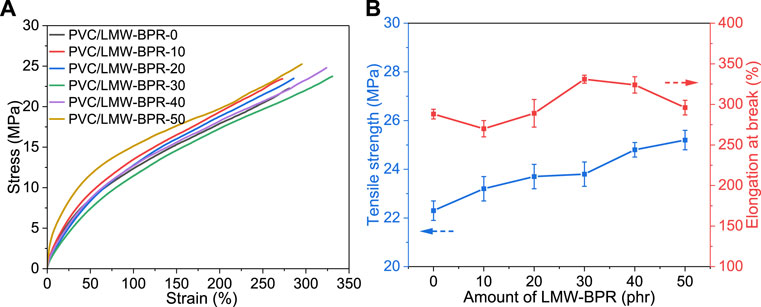
Figure 3. Mechanical properties of plasticized PVC materials: (A) Stress versus strain curves; (B) Line charts of tensile strength and elongation at break.
3.4 Thermal properties of PVC materials
Figure 4A shows the DSC curves of PVC materials. The Tg of plasticized PVC was significantly lower than that of pure PVC resin. This proves that the introduction of LMW-BPR and DINCH played a good plasticizing effect. As mentioned above, both the density of ester groups and the total interaction between ester groups and chlorine atoms in the plasticized PVC materials gradually increased with the increase of LMW-BPR content. This leads to a weakening of the molecular chain motility in the plasticized PVC material, which in turn leads to a gradual increase in the Tg of the material. As shown in Figure 4B, when the content of LMW-BPR is low (≤30 phr), the plasticized PVC material has a low embrittlement temperature (<−50°C), i.e. shows excellent low-temperature resistance. When the content of LMW-BPR is too high, the interaction between the molecular chains in the plasticized PVC material is too strong, which in turn leads to the deterioration of low-temperature impact performance. Note that all plasticized PVC materials have an embrittlement temperature below −40°C and therefore have the potential to be used in low-temperature fields.
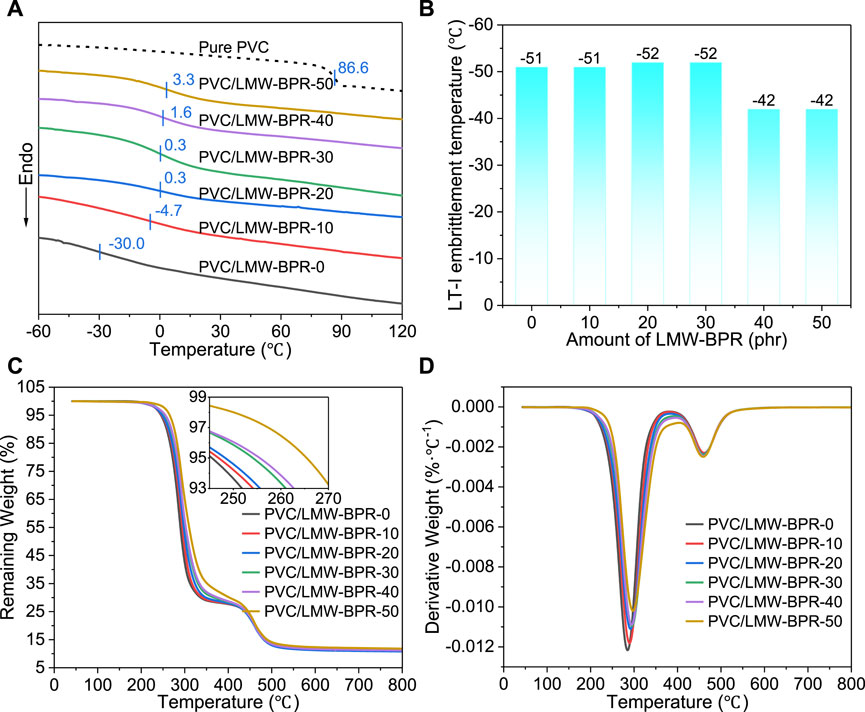
Figure 4. Thermal properties of plasticized PVC materials: (A) DSC curves; (B) Bar chats of embrittlement temperatures; (C) TG thermograms; (D) DTG thermograms.
Figures 4C, D show the thermogravimetric (TG) and derivative thermogravimetric (DTG) thermograms of plasticized PVC materials, respectively. All PVC samples exhibit a two-stage thermal weight loss, the first corresponding to the elimination reaction of the chlorine atoms and the second to the thermal cleavage of the molecular backbone. With the increase of LMW-BPR content, the onset decomposition temperature at 5% of weight loss (Td, 5%) and the maximum decomposition temperature of the first stage (Td, max1) of plasticized PVC materials gradually increased. The main reason is that the interaction between ester groups and chlorine atoms in plasticized PVC increases with the increase of LMW-BPR content, which will inhibit the elimination of chlorine atoms to a certain extent. Overall, plasticized PVC materials with good low-temperature resistance and high thermal stability can be prepared by using LMW-BPR as a plasticizer.
3.5 Biocompatibility of PVC materials
In vitro cytotoxicity test is one of the important methods to characterize the biocompatibility of materials. To evaluate the biocompatibility of plasticized PVC materials, we tested the relative growth rate (RGR) values of MC3T3 cells cultured in the extracts of different plasticized PVC materials at different times by using MTT assay. As shown in Figure 5, all plasticized PVC materials have RGR values above 75%, which corresponds to cytotoxicity grade 1 or 0, and thus are qualified biocompatible materials. Overall, LMW-BPR has comparable biocompatibility with the eco-friendly plasticizer DINCH, and is a promising, non-toxic and eco-friendly plasticizer.
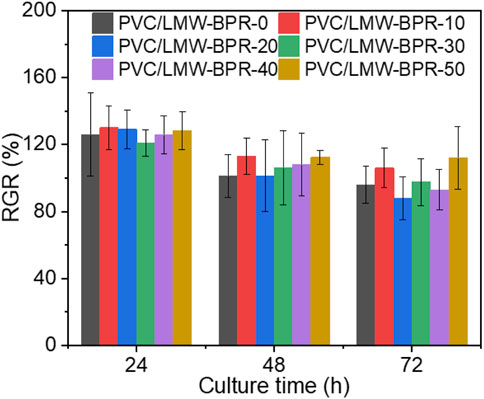
Figure 5. RGR values of MC3T3 cells cultured in the extracts of different plasticized PVC materials for different times.
4 Conclusion
In this paper, LMW-BPR with Mn of 22 kg/mol and Tg of −48°C was synthesized by melting polycondensation in a 100-L esterification-polycondensation reactor from five biobased polyester monomers, namely 1,3-propanediol, 1,4-butanediol, succinic acid, itaconic acid and sebacic acid. Subsequently, plasticized PVC composites with a total plasticizer content of 50 phr were prepared by using a mixture of LMW-BPR and DINCH as the plasticizer. Due to its high molecular weight, which is lower than that of existing polyester rubber but higher than that of most polyester plasticizer commodities, LMW-BPR does not migrate out of plasticized PVC composite and also can inhibit the migration of small molecules of DINCH. Migration resistance tests show that when the content of LMW-BPR reaches 30 phr, the plasticized PVC composites are almost nonmigratory. With the increase of LMW-BPR content, the tensile strength and the thermal stability of plasticized PVC composite increase, which can be attributed to the existence of strong interaction between abundant in-chain ester bonds in LMW-BPR and chlorine atoms in the PVC matrix. In addition, plasticized PVC containing LMW-BPR exhibited comparable plasticizing efficiency, flexibility, low-temperature resistance and biocompatibility with pure DINCH plasticized PVC. Overall, in this work, we have not only developed an efficient, sustainable and easily scalable PVC plasticizer, which is worthy of wide application, but also broadened the application range of BPR.
Data availability statement
The original contributions presented in the study are included in the article/Supplementary Material, further inquiries can be directed to the corresponding authors.
Author contributions
QZ: Writing–original draft, Writing–review and editing. JS: Writing–original draft. ZY: Writing–original draft. XD: Writing–review and editing. ZW: Writing–review and editing. LZ: Writing–review and editing.
Funding
The author(s) declare that financial support was received for the research, authorship, and/or publication of this article. This work was financially supported by the National Natural Science Foundation of China, Basic Science Center Program (51988102); the National Natural Science Foundation of China (52073011); the Innovative Research Groups (51221002 and 51521062); and the Postdoctoral Science Foundation of Beijing University of Chemical Technology (CMSE202202).
Conflict of interest
The authors declare that the research was conducted in the absence of any commercial or financial relationships that could be construed as a potential conflict of interest.
Publisher’s note
All claims expressed in this article are solely those of the authors and do not necessarily represent those of their affiliated organizations, or those of the publisher, the editors and the reviewers. Any product that may be evaluated in this article, or claim that may be made by its manufacturer, is not guaranteed or endorsed by the publisher.
References
Agarwal, D. K., Lawrence, W. H., Turner, J. E., and Autian, J. (1989). Effects of parenteral di-(2-ethylhexyl)phthalate (DEHP) on gonadal biochemistry, pathology, and reproductive performance of mice. J. Toxicol. Environ. health 26, 39–59. doi:10.1080/15287398909531232
Bourdeaux, D., Yessaad, M., Chennell, P., Larbre, V., Eljezi, T., Bernard, L., et al. (2016). Analysis of PVC plasticizers in medical devices and infused solutions by GC-MS. J. Pharm. Biomed. Analysis 118, 206–213. doi:10.1016/j.jpba.2015.10.034
Cao, Y., Li, L., Shen, K. H., and Liu, J. G. (2019). Disease burden attributable to endocrine-disrupting chemicals exposure in China: a case study of phthalates. Sci. Total Environ. 662, 615–621. doi:10.1016/j.scitotenv.2019.01.255
Chiellini, F., Ferri, M., Morelli, A., Dipaola, L., and Latini, G. (2013). Perspectives on alternatives to phthalate plasticized poly(vinyl chloride) in medical devices applications. Prog. Polym. Sci. 38, 1067–1088. doi:10.1016/j.progpolymsci.2013.03.001
Chuayjuljit, S., Siraprapoj, C., and Boonmahitthisud, A. (2015). Effects of poly(butylene succinate) and ultrafine wollastonite on the physical properties of plasticized poly(vinyl chloride). J. Vinyl & Addit. Technol. 21, 220–227. doi:10.1002/vnl.21386
Dirven, H. A., Van Den Broek, P. H., and Jongeneelen, F. J. (1990). Effect of di(2-ethylhexyl)phthalate on enzyme activity levels in liver and serum of rats. Toxicology 65, 199–207. doi:10.1016/0300-483x(90)90089-y
Gao, C. H., Zhang, X. H., Sun, J. W., Yuan, Z., Han, S. J., Liu, Y. T., et al. (2018). Poly(hexane succinate) plasticizer designed for poly(vinyl chloride) with a high efficiency, nontoxicity, and improved migration resistance. J. Appl. Polym. Sci. 135. doi:10.1002/app.46388
Gao, S., Wang, R., Fang, B., Kang, H., Mao, L., and Zhang, L. (2016). Preparation and properties of a novel bio-based and non-crystalline engineering elastomer with high low-temperature and oil resistance. J. Appl. Polym. Sci. 133, 42855. doi:10.1002/app.42855
Hakkarainen, M. (2008). Migration of monomeric and polymeric PVC plasticizers. Chromatogr. Sustain. Polym. Mater., 159–185. doi:10.1007/12_2008_140
Han, Y., Weng, Y., and Zhang, C. (2024). Development of biobased plasticizers with synergistic effects of plasticization, thermal stabilization, and migration resistance: a review. J. Vinyl Addit. Technol. 30, 26–43. doi:10.1002/vnl.22048
Ito, Y., Kamijima, M., and Nakajima, T. (2019). Di(2-ethylhexyl) phthalate-induced toxicity and peroxisome proliferator-activated receptor alpha: a review. Environ. Health Prev. Med. 24, 47. doi:10.1186/s12199-019-0802-z
Jia, P., Ma, Y., Song, F., Liu, C., Hu, L., and Zhou, Y. (2021). Renewable atom-efficient dendrimer-like acetate: from toxic tung oil to non-toxic plasticizers. Mater. Today Chem. 21, 100518. doi:10.1016/j.mtchem.2021.100518
Kang, H., Qiao, B., Wang, R., Wang, Z., Zhang, L. Q., Ma, J., et al. (2013). Employing a novel bioelastomer to toughen polylactide. Polymer 54, 2450–2458. doi:10.1016/j.polymer.2013.02.053
Kolarik, B., Naydenov, K., Larsson, M., Bornehag, C. G., and Sundell, J. (2008). The association between phthalates in dust and allergic diseases among Bulgarian children. Environ. Health Perspect. 116, 98–103. doi:10.1289/ehp.10498
Ledniowska, K., Nosal-Kovalenko, H., Janik, W., Krasuska, A., Stanczyk, D., Sabura, E., et al. (2022). Effective, environmentally friendly PVC plasticizers based on succinic acid. Polymers 14, 1295. doi:10.3390/polym14071295
Lindstrom, A., and Hakkarainen, M. (2006). Environmentally friendly plasticizers for poly(vinyl chloride) - improved mechanical properties and compatibility by using branched poly(butylene adipate) as a polymeric plasticizer. J. Appl. Polym. Sci. 100, 2180–2188. doi:10.1002/app.23633
Lindström, A., and Hakkarainen, M. (2007). Designed chain architecture for enhanced migration resistance and property preservation in poly (vinyl chloride)/polyester blends. Biomacromolecules 8, 1187–1194. doi:10.1021/bm070001k
Ma, Y., Bei, Y., Zhang, M., Song, F., Hu, F., Kou, Z., et al. (2022). Synthesis of woody oil-based plasticizer via solvent-free Diels-Alder reaction and its biodegradability. Industrial Crops Prod. 188, 115646. doi:10.1016/j.indcrop.2022.115646
Miao, H. Y., Zhao, H., and Jiang, P. P. (2017). Poly(vinyl chloride) films plasticized with novel poly-nadic-anhydride polyester plasticizers. J. Vinyl & Addit. Technol. 23, 321–328. doi:10.1002/vnl.21510
Rahman, M., and Brazel, C. S. (2004). The plasticizer market: an assessment of traditional plasticizers and research trends to meet new challenges. Prog. Polym. Sci. 29, 1223–1248. doi:10.1016/j.progpolymsci.2004.10.001
Rushton, B. M., and Salomons, N. S. (1969). An investigation of factors relating to the exudation of polyester plasticizers from poly(vinyl chloride). J. Appl. Polym. Sci. 13, 2341–2358. doi:10.1002/app.1969.070131110
Rusu, M., Ursu, M., and Rusu, D. (2006). Poly(vinyl chloride) and poly(e-caprolactone) blends for medical use. J. Thermoplast. Compos. Mater. 19, 173–190. doi:10.1177/0892705706056463
Sin, M. C., Tan, I. K. P., Annuar, M. S. M., and Gan, S. N. (2012). Thermal behaviour and thermodegradation kinetics of poly (vinyl chloride) plasticized with polymeric and oligomeric medium-chain-length poly (3-hydroxyalkanoates). Polym. Degrad. Stab. 97, 2118–2127. doi:10.1016/j.polymdegradstab.2012.08.020
Sun, Z., Choi, B., Feng, A., Moad, G., and Thang, S. H. (2019). Nonmigratory poly (vinyl chloride)-block-polycaprolactone plasticizers and compatibilizers prepared by sequential RAFT and ring-opening polymerization (RAFT-T-ROP). Macromolecules 52, 1746–1756. doi:10.1021/acs.macromol.8b02146
Tan, J. H., Liu, B. W., Fu, Q. H., Wang, L. W., Xin, J. N., and Zhu, X. B. (2019). Role of the oxethyl unit in the structure of vegetable oil-based plasticizer for PVC: an efficient strategy to enhance compatibility and plasticization. Polymers 11, 779. doi:10.3390/polym11050779
Tang, S., Li, J., Wang, R., Zhang, J., Lu, Y., Hu, G.-H., et al. (2022). Current trends in bio-based elastomer materials. SusMat 2, 2–33. doi:10.1002/sus2.45
Tortolano, L., Matmati, H., Bourhis, M., Manerlax, K., Lemare, F., Saunier, J., et al. (2018). DinCH and ESBO actual migration from PVC infusion tubings used in an oncopediatric unit. J. Appl. Polym. Sci. 135, 8. doi:10.1002/app.46649
Wei, T., Lei, L., Kang, H., Qiao, B., Wang, Z., Zhang, L., et al. (2012). Tough bio-based elastomer nanocomposites with high performance for engineering applications. Adv. Eng. Mater. 14, 112–118. doi:10.1002/adem.201100162
Xie, T. T., Gao, C. H., Wang, C. X., Shen, S. E., and Wu, Y. M. (2014). Application of poly(butylenes 2-methylsuccinate) as migration resistant plasticizer for poly(vinyl chloride). Polymer-Plastics Technol. Eng. 53, 465–471. doi:10.1080/03602559.2013.845207
Xu, Y., Xiong, Y., and Guo, S. (2020). Effect of liquid plasticizers on crystallization of PCL in soft PVC/PCL/plasticizer blends. J. Appl. Polym. Sci. 137, 48803. doi:10.1002/app.48803
Xue, J., Niu, Y., Gong, M., Shi, R., Chen, D., Zhang, L., et al. (2015). Electrospun microfiber membranes embedded with drug-loaded clay nanotubes for sustained antimicrobial protection. ACS Nano 9, 1600–1612. doi:10.1021/nn506255e
Yang, D., Tian, M., Dong, Y., Kang, H. L., Gong, D. L., and Zhang, L. Q. (2013). A high-performance dielectric elastomer consisting of bio-based polyester elastomer and titanium dioxide powder. J. Appl. Phys. 114, 154104. doi:10.1063/1.4824805
Yang, D., Tian, M., Kang, H., Dong, Y. C., Liu, H. L., Yu, Y. C., et al. (2012). New polyester dielectric elastomer with large actuated strain at low electric field. Mater. Lett. 76, 229–232. doi:10.1016/j.matlet.2012.02.084
Yin, B., and Hakkarainen, M. (2011). Oligomeric isosorbide esters as alternative renewable resource plasticizers for PVC. J. Appl. Polym. Sci. 119, 2400–2407. doi:10.1002/app.32913
Yu, B. Y., Chung, J. W., and Kwak, S. Y. (2008). Reduced migration from flexible poly(vinyl chloride) of a plasticizer containing β-cyclodextrin derivative. Environ. Sci. Technol. 42, 7522–7527. doi:10.1021/es800895x
Yu, L., Li, H.-X., Guo, J.-Y., Huang, Y.-Q., Wang, H., Talukder, M., et al. (2019). Di (2-ethyl hexyl) phthalate (DEHP)-induced spleen toxicity in quail (Coturnix japonica) via disturbing Nrf2-mediated defense response. Environ. Pollut. 251, 984–989. doi:10.1016/j.envpol.2019.05.061
Zhang, Q., Song, M., Xu, Y., Wang, W., Wang, Z., and Zhang, L. (2021). Bio-based polyesters: recent progress and future prospects. Prog. Polym. Sci. 120, 101430. doi:10.1016/j.progpolymsci.2021.101430
Keywords: polyester plasticizer, polyvinyl chloride, biobased polyester rubber, migration resistance, low-temperature resistance
Citation: Zhang Q, Sun J, Yao Z, Ding X, Wang Z and Zhang L (2024) Synthesis and performance evaluation of low-molecular-weight biobased polyester rubber as a novel eco-friendly polymeric plasticizer for polyvinyl chloride. Front. Mater. 11:1406469. doi: 10.3389/fmats.2024.1406469
Received: 25 March 2024; Accepted: 04 July 2024;
Published: 29 July 2024.
Edited by:
Yasir Nawab, National Textile University, PakistanReviewed by:
Puyou Jia, Chinese Academy of Forestry, ChinaJunheng Zhang, South-Central University for Nationalities, China
Copyright © 2024 Zhang, Sun, Yao, Ding, Wang and Zhang. This is an open-access article distributed under the terms of the Creative Commons Attribution License (CC BY). The use, distribution or reproduction in other forums is permitted, provided the original author(s) and the copyright owner(s) are credited and that the original publication in this journal is cited, in accordance with accepted academic practice. No use, distribution or reproduction is permitted which does not comply with these terms.
*Correspondence: Zhao Wang, d2FuZ3poYW9jbEBzY3V0LmVkdS5jbg==; Xuejia Ding, ZGluZ3hqQG1haWwuYnVjdC5lZHUuY24=