- 1Queen Mary School, Jiangxi Medical College, Nanchang University, Nanchang, China
- 2Qingdao Hospital, University of Health and Rehabilitation Sciences (Qingdao Municipal Hospital), Qingdao, China
- 3Department of Infectious Diseases, The Second Affiliated Hospital of Nanchang University, Nanchang, China
Aggregation-induced emission (AIE) can exhibit different properties in different situations, such as non-emission and highly fluorescent in the dissolved state of the molecule and in the aggregate or solid state, respectively. This property of AIE is distinguished from aggregation-induced quenching (ACQ) or even the opposite. Combining the AIE phenomenon with different polymers yields different polymers with corresponding AIE properties. In this paper, the mechanism, synthesis, branching and application of AIE in the fields of optoelectronic functional materials, sensors, biology, and environment are reviewed. It is hoped that this review will stimulate more research on molecular aggregates and promote further cross-fertilisation and greater development in the disciplines of materials, chemistry and biomedicine.
1 Introduction
The study of molecular luminescence shows that the luminescence properties of individual molecules are determined by their molecular structure. Aggregation-induced quenching (ACQ), also known as concentration quenching, is related to the formation of aggregates, i.e., at higher concentrations, many organically luminous materials do not emit light. That is, fluorescence intensity decreases with increasing concentration (Mei et al., 2015). In 2001, academician Tang et al. (2001), Luo et al. (2001), Hong et al. (2009), Hong et al. (2011) and his team accidentally discovered a completely opposite phenomenon of luminescence (Figures 1, 2, 3). When a non-luminescent thiole derivative exist in the form of a single molecule in solution, the fluorescence intensified gradually with the drying of the solvent. On this basis, the research group carried out a series of in-depth exploration and research, and a completely new concept was proposed, namely, aggregation-inducedemission (Tang et al., 2009; Wang et al., 2017). For example, a dye with a multi-rotor structure of hexaphenylthiarorole (HPS) does not fluoresce when it is in a dispersed state in a good solvent, but it fluoresces strongly when it is present in a concentrated state in an undesirable solvent, and this special phenomenon has further stimulated the scientific community’s enthusiasm for the study of this phenomenon. Due to its different properties from traditional dye aggregation quenching, AIE dyes can be applied in different concentration environments, which solves the difficulties and limitations faced by traditional dyes in practical applications, and has great research value and significance (Zhao et al., 2010; Leung et al., 2014; Chen et al., 2015; Song et al., 2016; Yan et al., 2016). In recent years, the concept of AIE has been introduced into macromolecules, and a variety of AIE polymers have been designed and synthesized (Chen et al., 2003; Ma et al., 2019). Compared with small molecule AIE fluorophores, AIE polymers have the advantages of easy modification and good processing performance, which can meet the different needs of different fields. In addition, the AIE polymer backbone or side chain can better limit the rotation and vibration of the AIE molecules, so the non-radiative transitions can be better suppressed to enhance fluorescence. Compared with traditional fluorescent polymers, AIE polymers are expected to exhibit unique properties and significant advantages in practical applications due to their high luminescence efficiency in aggregate and solid-state luminescence efficiency, large Stokes shift, and excellent photostability.
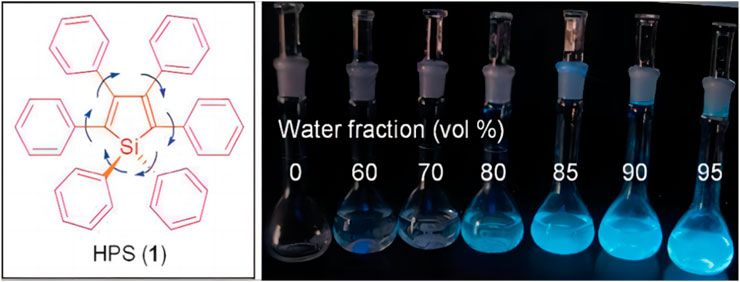
Figure 1. (Left) Structural chemical formula of HPS. (Right) Picture of HPS solution at different water contents (Hong et al., 2009).
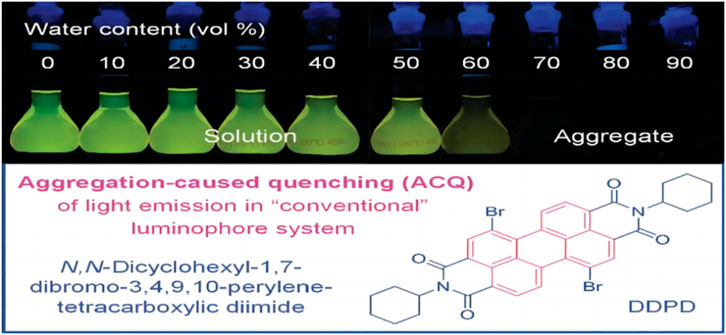
Figure 2. Fluorescence images of DDPD solutions/suspensions at different water contents (Hong et al., 2011).
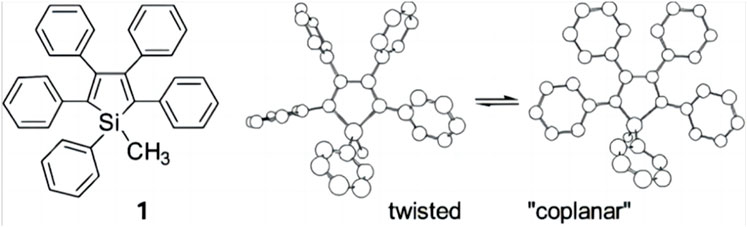
Figure 3. Molecular structure and conformational transition of 1-methyl-1, 2,3,4,5-pentaphenylthiarrole (Luo et al., 2001).
2 Mechanism and synthesis method of AIE
2.1 Mechanism of AIE
Since the discovery of this phenomenon in the 19th century, academician Benzhong Tang’s research group began to study AIE phenomenon in diverse AIE systems. They realized that the active movement of AIE luminaires (AIEgens) in the excitation molecule could lead to accelerated non-radiative decay of excitons, which further led to the extinguishment of exciton emission in the dissolved state. Such intramolecular rotation is confined to the aggregated state, resulting in a reduction in non-radiative attenuation and an increase luminescence (Leung et al., 2014; Zhang X. et al., 2019). They suggested that intramolecular rotation restriction (RIR) was the mechanism of AIE. However, a subsequent study found that the RIR mechanism did not work for all AIEgens. To explain these new rotorless AIE systems, they propose a supplement to the new RIRs, namely, Limiting Intramolecular Vibrations (RIVs), as intramolecular vibrations are included among the causes of non-radiative decay. (Tseng et al., 2012; Zhao et al., 2019; Yang et al., 2013; Zhou et al., 2016). Leung et al. (2014) reported a broadeningof the AIE mechanism through analysis of 10, 10′,11, 11′-tetrahydro-5,5′-bidibenzo [a,d] (Tang et al., 2009) annulenylidene (THBDBA),and5,5′-bidibenzo [a,d] (Tang et al., 2009) annulenylidene (BDBA). Analyses of the computational QM/MM model reveal that the novel mechanism behind the AIE of THBDBA and BDBA is the restriction of intramolecular vibration (RIV). A more generalized mechanistic understanding of AIE results by combining RIR and RIV into the principle of restriction of intramolecular motions (RIM). (Figures 4, 5).
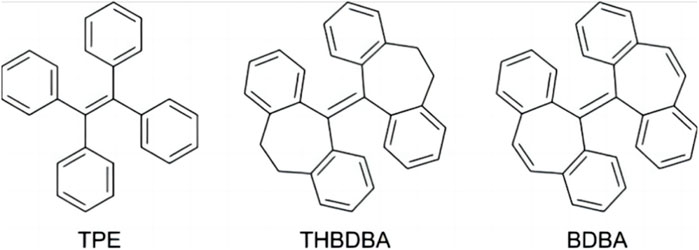
Figure 4. Molecular structures of tetraphenylethene (TPE), 10,10′,11,11′- tetrahydro-5,5′-bidibenzo [a,d] (Tang et al., 2009)annulenylidene (THBDBA), and 5,5′- bidibenzo [a,d] (Tang et al., 2009)annulenylidene (BDBA) (Leung et al., 2014).
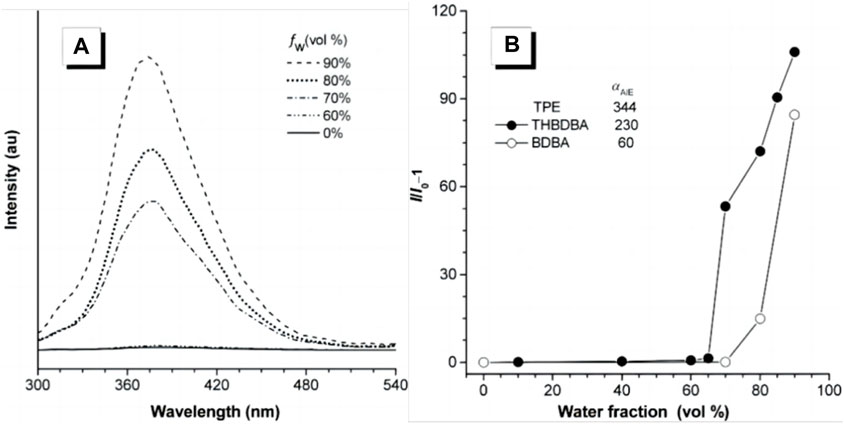
Figure 5. (A) The photoluminescence (PL) spectra of THBDBA in THF and THF/water mixtures with increasing water fractions (fw) to 90%. (B) Change in PL intensity of THBDBA and BDBA at 377 nm versus water fraction in THF/water mixtures. Inset: αAIE values of TPE, THBDBA, and BDBA. Dye concentration: 20 mm; excitation wavelength: 280 nm (Leung et al., 2014).
2.2 Synthesis method of AIE
The synthesis methods of AIE polymers are mainly divided into: one-component polymerisation, two-component polymerisation and multi-component polymerisation. In other words, they can be divided into direct polymerisation and post-functionalisation methods (Mei et al., 2015; Zhan et al., 2017). Of these, there are four pathways for synthesis by direct polymerisation: 1) Direct polymerisation of monomers containing AIE; 2) Co-polymerisation of AIE-containing monomers with other monomers; 3) The polymerisation is initiated by an initiator containing AIE; 4) AIE polymers are synthesised by linking AIE-containing monomers through polycondensation reactions. 5) Taking the AIE-free active precursor, the AIE structure is generated in the polymer backbone by polymerisation or copolymerisation. Post-functionalization methods synthesize AIE polymers by backbone, side chain, or cross-linking reactions, as well as polymers prepared by inactive precursors or other molecular modifications.
2.2.1 One-component polymerization
One-component polymerisation is a polymerisation reaction based on a single monomer and is characterised by the fact that the polymer is prepared without the need for cumbersome, stringent stoichiometric balances. There are several methods for preparing AIE polymers by single-component polymerisation, such as free radical polymerisation and translocation polymerisation. Yang et al. (2009) prepared polymers containing side chains of AIE units by free radical polymerisation of olefinic monomers with AIE properties. The polymerisation reaction is based on the free radical polymerisation of carbazole-substituted triphenylene monomers via azobisisobutyronitrile (AZBN) to build AIE polymers. Due to the twisted structure of the monomer, the polymer is well dispersed in good organic solvents and is aggregated in poor solvent water, showing significant fluorescence enhancement properties. In addition, the high glass transition and thermal decomposition temperatures of the polymers indicate their potential for use in the preparation of organic light-emitting diode devices and fluorescent sensors.
Gu et al. (2013) prepared AIE polymers by atom transfer radical polymerisation. Due to the presence of electron-pushing diethylamino groups and electron-pulling 1,8-naphthylimides in the monomers, the polymers were found to be very reactive in THF-ethanol solvent mixtures. As the mass content of ethanol increases, it first shows the intramolecular charge transfer effect, and when the ethanol content is greater than 60%, it shows the AIE property, and when the ethanol content is 99%, the fluorescence intensity is 2.83 times higher than that of THF solution (Figure 6).
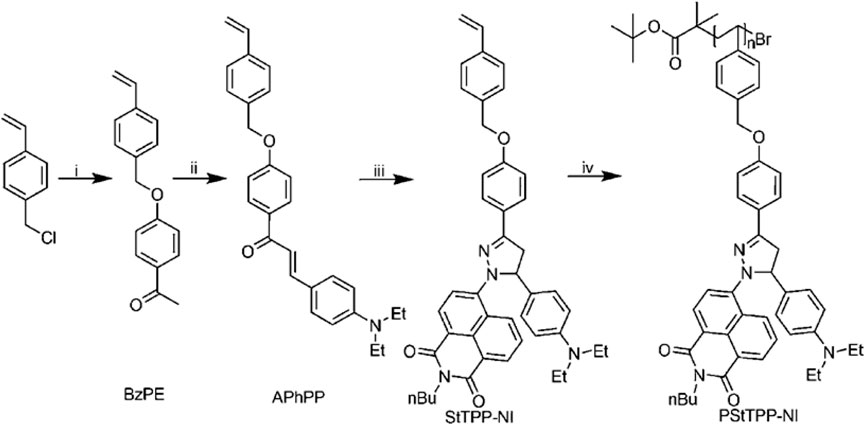
Figure 6. Synthesis of AIE polymer by one-component polymerization (Gu et al., 2013).
Chen et al. (2003) prepared polymers containing side chains of AIE units by translocation polymerisation of alkynyl monomers with AIE properties. AIE polymers with high relative molecular mass and high yield (80%) were prepared by polymerisation using silica polyacetylene monomer as monomer. Due to the distorted structure of the silane molecules, the polymers are non-fluorescent in the dispersed state, and exhibit significant AIE characteristics in poor solvents or low temperatures.
2.2.2 Two-component polymerization
Two-component polymerisation is a strategy for preparing polymers by linking two difunctional monomers. It is the more traditional and popular method for the preparation of AIE polymers, which involves a number of classical polymerisation reactions such as click polymerisation, free radical polymerisation, polycondensation, ring-opening polymerisation, etc. (Hu et al., 2014).
An early reaction is called azide-alkyne click polymerization (AACP), which builds linear and hyperbranched polymers that can have AIE properties. Qin et al. (2009) synthesised for the first time tetraphenylethylene-containing AIE polymers with good solubility, high relative molecular mass and good thermal stability using Cu(Ⅰ)-catalyzed AACP. In order to overcome the problem of metal residues in click polymerisation, various metal-free spontaneous polymerisations such as mercaptoalkynyl polymerisation, aminoalkynyl polymerisation and hydroxyalkynyl polymerisation have been developed.
Free radical polymerisation is a commonly used method for the synthesis of AIE polymers from AIE reactive monomers. Forinstance, Wan et al. (2018) used EtAmPy with AIE properties as a primer to synthesize end-group-functionalized polymers with AIE properties by atom transfer radical polymerization (Figure 7). In addition, Tanaka et al. (2015) designed and synthesised boron diimide AIE monomers with different substituents, and produced AIE polymers with high relative molecular mass and scintillation ability by polycondensation reaction based on Suzuki coupling (Figure 8).
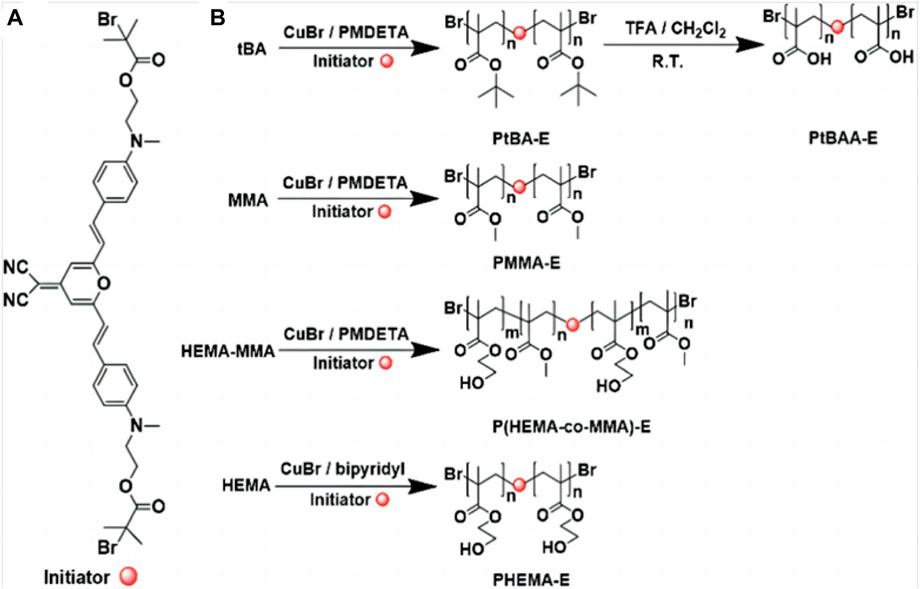
Figure 7. (A) Structure of initiator EtAmPy and (B) synthetic routes to the polymers (Wan et al., 2018).
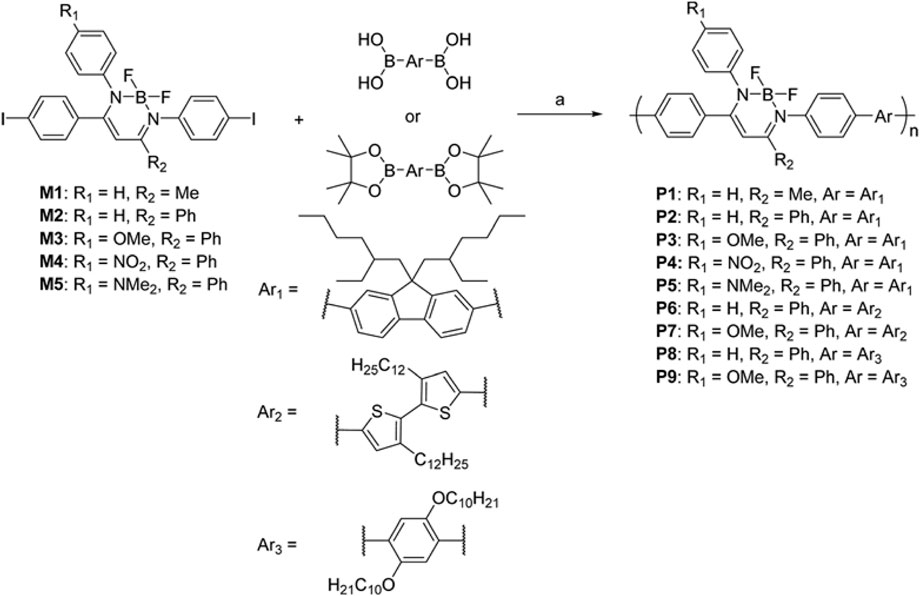
Figure 8. Methods of synthesis of polymers (Tanaka et al., 2015).
2.2.3 Multi-component polymerisation
Multi-component polymerisation is a unique polymerisation reaction based on a multi-component reaction involving three or more monomers. They are characterised by high atom economy, mild reaction conditions, simple operating procedures and a variety of structures. Song et al. (2018) successfully synthesised AIE polymers by Ag2WO4-catalyzed multicomponent polymerisation of CO2, thioglycodiyne-containing and alkyl dihalides under mild reaction conditions at atmospheric pressure. The polymer has an absolute fluorescence quantum yield of 61% in the solid state, which is a significant improvement over the previous one (Figure 9).
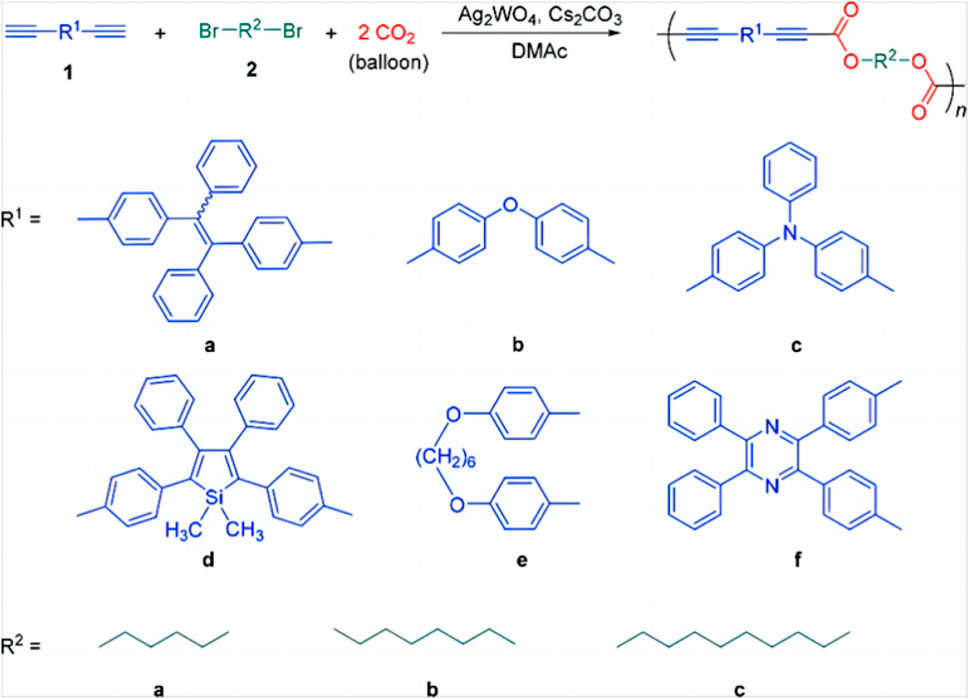
Figure 9. Preparation of AIE polymer by multi-component. Polymerization (Song et al., 2018).
2.2.4 Other examples of direct aggregation and post-functionalization
AIE active conjugated polymers are generally prepared by polycondensation reaction. Dai et al. (2015) synthesized organoboron conjugated polymers via palladiumcatalyzed Suzuki coupling reaction of 2,7-dioxaborolanefluorene and using Pd(PPh3)4 as the catalyst. A rotatable phenyl group was incorporated onto the nitrogen atom of the boron ketoiminate derivative, endowing the final polymers with AIE property. High molecular weight astrophic or cross-linked AIE polymers are synthesized by reacting with molecules containing multiple reactive groups. Ma et al. (2015), Wang et al. (2015) used water-soluble star-shaped polyethylene oxide (PEO) or branched polyethylenimine (PEI) to cross-link anhydride or epoxy groups containing polymers to yield the final AIE polymers. He et al. (2020) synthesized a series of multifunctional hyperbranched polyesters (β-aminoacrylates) by spontaneous aminoalkyne bond polymerization using esterification-activated triyne and diamine as monomers. As a result, high-quality HB-PAAs with high average molecular weight (Mw up to 18,290), soluble and heat-stable were obtained, with a yield of up to 99%. When the introduction of an AIE-active TPE group into the hb-PAA backbone can make it exhibit typical AIE characteristics.
3 Research branches of AIE
Over the past few decades, a series of new branches of AIE have been evolved based on the key breakthroughs in AIE research, such as clusterization-triggered emission (CTE), crystal-induced emission (CIE), room temperature phosphorescence (RTP), aggregation induced delayed fluorescence (AIDF), mechanoluminescence (ML), aggregation induced generation of reactive oxygen species (AIG-ROS) and so on. In this part, the research branches of AIE are summarized.
3.1 Clusterization-triggered emission (CTE)
Traditional luminescence groups are generally composed of aromatic groups, etc. With the continuous development of AIEgens research, it has been found that many non-conjugated polymers and natural products that do not contain aromatic building groups also demonstrate AIE properties (Ye et al., 2017). The CTE mechanism is proposed in view of the presence of electron-rich subunits in polymers, the aggregation emission when linking clusters, the overlap of different clusters with subsequent electron clouds, and the conformational strengthening of molecules. In these clusters, conjugation is extended by steric electron interactions, that is, the overlap of π and lone pair (n) electrons between cyanogroups, while ossifying the molecular conformation, thus providing significant emission upon irradiation. Therefore, those photophysical processes that differ from the general laws can be explained by CTE (Zhou et al., 2016). The proposal and study of CTE mechanism further improves the interpretation of AIE characteristics expressed by non-conjugated polymers and natural products that do not contain aromatic building groups. With the continuous development and deepening of CIE system, some other unconventional luminaries have been designed and discovered by using CIE mechanism. For example, polyacrylonitrile (PAN) is a cyano-based polymer that emits little light in dilute dimethylformamide (DMF) solution, while its solid powder glows visible blue when exposed to ultraviolet light. Polymethacrylate (N-hydroxysuccinimide) ester (PNHSMA) without aromatic structure also emits almost no light in dilute solution, but becomes highly luminous in concentrated solution and solid powder (Bin et al., 2016). Starch and cellulose contain a lot of isolated oxygen atoms, which do not produce visible light in solution. While in the solid state, these electron-rich oxygen atoms can be pushed into a star cluster, leading to a highly overlapped electron clouds by interactions across space, and subsequent conjugated and rigid conformations which contribute to emission. As a result, these materials can emit visible light when excited (Gong et al., 2013; He et al., 2018).
3.2 Crystal-induced emission (CIE)
Since many advanced optoelectronic devices work as thin films or crystals, organic light-emitting devices with strong solid-state emission are very important (Guo et al., 2018). In addition, crystallization is conducive to charge transfer in organic semiconductors and to the generation of OLETs and organic lasers (Zhao et al., 2017; Zhao et al., 2018). However, for conventional luminaries, crystallization is undesirable because strong p-p interactions in the crystals usually lead to a change in emission wavelength, resulting in reduced emission intensity (Zheng et al., 2016; Yamaguchi et al., 2017). In order to avoid luminescent crystallization, various chemical modification and physical doping strategies are used, which lead to high cost and complex equipment (Parashchuk et al., 2018; Liu D. et al., 2019). It has been found that some molecules behave differently in different states, and do not exhibit emission in solution and amorphous solids, but emit significantly in crystalline states., such as (4-biphenylyl) phenyldibenzofulvene (BpPDBF) (Dong et al., 2006). This phenomenon was called crystal induced emission (CIE). Further studies have shown that there are a variety of intermolecular forces that interact with each other and few strong p-p interactions among molecules in CIE crystals, which is not only conducive to heighten the molecular conformation, but also avert emission quenching through p-p interactions (Chen et al., 2017; Yamaguchi et al., 2017). Therefore, the presence of an energy available conical intersection (CI) or minimum bandgap of the excited state will result in a redshift and attenuation of the emission (Li and Blancafort, 2013; Gao et al., 2017). However, in the crystal form, the CI and excited state minima are energetically inaccessible due to conformational rigidity. The CIE phenomenon indicates that active intramolecular motion may still exist even in amorphous solids and affect the photophysical properties of luminogen.
3.3 Room-temperature phosphorescence (RTP)
Room temperature phosphorescent (RTP) organic luminescence has attracted a lot of attention due to its relatively long-lived triple excitation state, which has important applications in emergency lighting, anti-counterfeiting, and high-sensitivity bioimaging. However, the weak spin-orbits coupled (SOC) of most organic molecules give rise to inefficient and low speed than the radiative decay of three-state excitons compared to inorganic and organometallic phosphorescent compounds. Typical examples have halogen bonds (Bolton et al., 2014), and n-π transition (Zhao et al., 2016) that promote the singlet-triolet intersystem crossing (ISC) and simultaneously reduce the nonradiative decay of the three-state exciton. Taking BDBF as an example, the planar molecule BPBF emits light in the crystalline state but not in the amorphous state (Gong et al., 2013). Crystallization can also inhibit the significant phosphorescence induced by the non-radiative attenuating exciton triad (Gong et al., 2013). Since non-radiative attenuation of crystal-induced conformational stiffness can effectively block molecules, which allows better management of the ISC process, CIP essentially implements the simple design of RTP.
3.4 Aggregation-induced delayed fluorescence (AIDF)
Fluorescence and phosphorescence can be modulated by suppressing non-radiative attenuation and ISC processes at the aggregation level. What’s more, more importantly, delayed fluorescence torsion system crossover is achieved by exciton excitation by singlet and triplet states (Duan et al., 2018). TADF emitters are highly device-efficient phosphorescent materials, and almost all of them are dispersed into the host matrix to inhibit concentration quenching or exciton annihilation. Therefore, in order to ensure the efficient of TADF-based OLEDs, the precise control of doping process is usually required. In addition, the electroluminescence (EL) efficiency of many doped OLEDs at high brightness still shows a serious roll-over phenomenon Which becomes an obstacle that needs to be overcome (Furue et al., 2016; Bryce, 2017). In the aggregated state, isomers usually have distorted structures, strong emission and weak intermolecular p-p interactions. Therefore, pure AIEgens films can alleviate emission quenching and exciton annihilation and are suitable for fabrication of undoped OLEDs devices.
3.5 Mechanoluminescence (ML)
In addition to photoluminescence, mechanluminescence (ML), which converts mechanical energy into visible light, is another emission phenomenon.ML phenomenon has been discovered since 1605, but its luminescence mechanism has not been clearly defined. In general, the controversy in ML phenomena is centered on how the discharge occurs (Zink et al., 1976; Chandra et al., 2013). The luminous causes of ML include gas discharge, energy transfer and the coexistence of both. The theory of electron bombardment proposed by Zink et al. and Chandra et al. seems to hold for most organic or inorganic crystal breaks in ML (Chandra et al., 2012). They proposed that when the electrons are bombarded, the material dissolves to form a new surface with an energy of +5 eV and a wavelength of 250 nm. Therefore, ML materials with typical luminescence centers can be excited with the energy transferred from Which the crystals are bombarded with electrons, while there is no consensus on the luminescence mechanism of ML materials without typical luminescence centers. Nevertheless, from the perspective of cluster luminescence, Their emission appears to be excited by cluster excitons that emit light or are doped with impurities (Zhang et al., 2020).
3.6 Aggregation induced generation of reactive oxygen species (AIG-ROS)
The radiant decay of excited states includes fluorescence, phosphorescence and delayed fluorescence. In addition, excitons may undergo inactivation pathways such as internal conversion or electron transfer to O2, such as heat generation or reactive oxygen species (ROS) (Bin Chen et al., 2017; Zhou et al., 2017). Due to their planar structure and extended conjugated P system, traditional phosphor compounds are susceptible to aggregation under physiological conditions. Therefore, due to the acceleration of exciton non-radiative attenuation by strong p-p superposition, the production efficiency of ISC and ROS is reduced. They show high ROS production efficiency and low LOS production efficiency. (Gu et al., 2018; Zhang C. et al., 2019). AIEgens in the polymeric state exhibited a higher ROS production efficiency, while the lower ROS production efficiency in the solution state (Sturala et al., 2017). The low ROS generation in solution state is due to the non-radiative attenuation accelerated by the active intramolecular motion, resulting in low ISC efficiency and triplet instability. So non-radiative attenuation is effectively inhibited, and ISC efficiency and triplet exciton stability are improved, thus producing high ROS efficiency. (Xu et al., 2017) (Table 1).
4 Applications of AIE
AIE materials can make full use of the natural aggregation of molecules and inhibit the non-radiative dissipation of excited states by limiting intramolecular motion, and have been widely used in various frontier fields such as optoelectronic devices, chemical sensing, and biological detection and imaging due to their high luminescence efficiency of aggregate states (Fan et al., 2024; Zheng, 2021; Hou, 2024; Tang, 2024; Ma et al., 2022; Xue et al., 2023; Lee et al., 2023; Xu et al., 2023; Zhang E. et al., 2023; Yuan et al., 2022; Zalmi et al., 2022; Gu et al., 2023; Sun et al., 2023; Ye et al., 2023).
4.1 Optoelectronic functional materials
The application of traditional ACQ organic functional materials is greatly restricted due to their low photoelectric conversion efficiency, slow response time and poor molecular flexibility, as well as the fact that they are often used in aggregated or thin film environments. To compensate for the shortcomings of ACQ, researchers have had to develop novel materials. The development of AIE materials has led to the gradual resolution of this drawback and has become a luminescent material with potential application value.
4.1.1 Electroluminescent device (ELD)
Conjugated polymers usually show luminescent properties once they are made into light-emitting diode (LED) devices (Zhao et al., 2012; Liu et al., 2016; Wei et al., 2016). Tan (Tan et al., 2017) et al. designed and synthesised novel blue light-emitting materials TPE-4Br and TPE-3Br using tetraphenylene (TPE). And as the light-emitting layer of the organic light-emitting diode device, using the intrinsic exciton luminescence of the material and its electroluminescent complex luminescence, the ideal white light electroluminescence can be obtained (Figure 10). Zhang H. et al. (2019) prepared six AIE-HLCT blue light molecules using phenanthrene imidazole (PPI), cyano and TPE motifs. The TPE motif makes the material exhibit obvious AIE properties, resulting in high fluorescence quantum efficiency in both solid-state environments, resulting in undoped sky blue OLEDs (maximum brightness, EQE, CE, PE 31 070 cd·m−2, 7. 16%, 18. 46 cd·A−1 and, 16. 32 lm· W−1), the electrogenerated exciton utilization rate is 48%, and the efficiency roll-off is low.
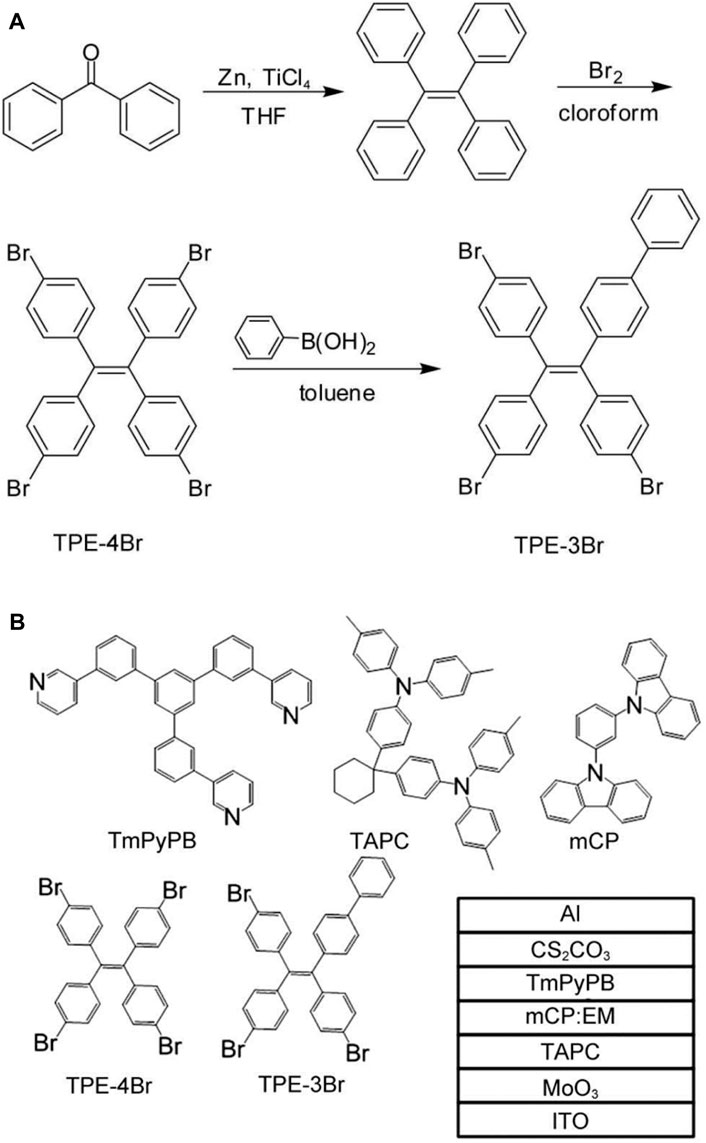
Figure 10. (A) Synthetic routes of TPE-4Br and TPE-3Br. (Tan et al., 2017), (B) Chemical structures of organic materials and simplified configuration of OLED devices (Tan et al., 2017).
4.1.2 Stimulus-responsive materials
AIE polymers can respond accordingly to stimuli from different elements in their surroundings such as heat and light (Wu et al., 2012; Yin et al., 2013; Kuo and Hong, 2024) and can therefore be used to design stimulus-responsive materials. Zhang et al. (2018) synthesised a series of AIE supramolecular polymer gels by coordinated multilevel hierarchical self-assembly of host-guest interactions. It can be converted into a gel with a higher concentration than the original, which show AIE properties due to the restriction of intramolecular movement of tetraphenylethylene in the three-dimensional polymer network. Using the kinetic nature of ligand bonding and subject-guest interactions, this polymer can exhibit a gel-sol transition, as well as an “on-off” of fluorescence under different stimuli (e.g., temperature, competing guest molecules, halides, etc.) (Figure 11). Zhao et al. (2021) reported a novel light-responsive luminescent material (PRL) with reversible conformational and fluorescence switching properties in the solid state. The luminescence properties of TPE-4N can be reversibly controlled by rapid light triggering and thermal annealing. The luminescence properties of TPE-4N can be reversibly controlled by rapid light triggering and thermal annealing. Since light stimulation is non-contact and non-destructive, it has the advantages of high contrast, fast response, good persistence, and reversibility.
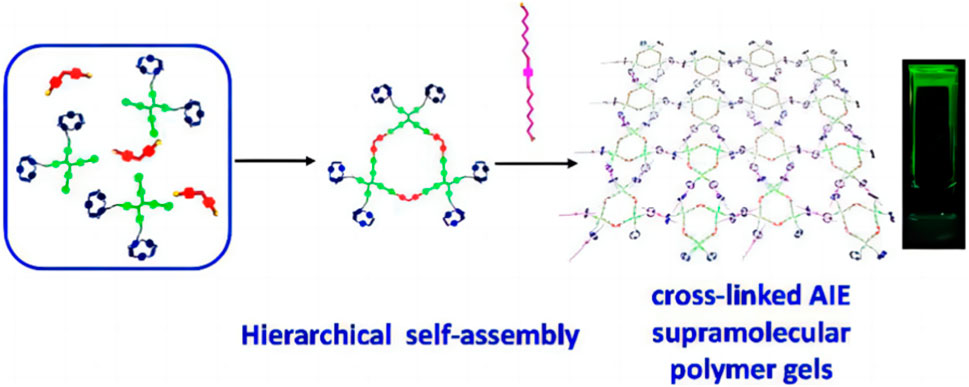
Figure 11. AIE supramolecular polymer gel capable of responding to external stimulation (Zhang et al., 2018).
4.2 Sensor applications
Sensing refers to the use of a special system, i.e., a sensor or probe, to detect specific entities, such as matter and energy. Sensors have been applied to various fields such as medicine, industry, environmental protection and control, so the development of sensors is of great significance (Wang et al., 2014; Gupta, 2019; Jiang et al., 2021; Yan et al., 2022; Ye et al., 2023).
4.2.1 Chemical sensors
Carbon dioxide gas can be detected and quantified using the AIE molecule. Liu et al. (2010), dissolved 1,1,2,3,4,5-Hexaphenylsilole (HPS) in an amine solvent (e.g., dipropylamine, DPA) to obtain a solution with no fluorescence emission. When carbon dioxide gas is drummed into this solution, it reacts with the dipropylamine in it to form a carbamate ionic liquid (CIL). The HPS molecule begins to emit fluorescence because the restricted intramolecular rotation (RIR) process is triggered. The intensity of fluorescence varies depending on the number of ions produced as carbon dioxide enters the liquid. The more carbon dioxide, the more ions produced, the stronger the fluorescence, and vice versa. This method allows for accurate analysis of carbon dioxide levels, such as prediction of volcanic eruptions, as well as environmental safety testing (Figure 12). Cuc et al. (2021) reported a novel multi-stimulus-responsive fluorescent supramolecular polymers Poly (TPE-DBC)/FL-DBA and TPE-DBC/FL-DBA. After the coordination of the supramolecular polymer with Al3+ ions, the TPE-DBC/FL-DBA-Al3+ and Poly (TPE-DBC)/FL-DBA-Al3+ supramolecular host and guest systems were obtained, respectively. The supramolecular polymers exhibited high sensitivity and selectivity for Al3+, and the green-emitting guest FL-DBA-Al3+ was chelated by FL-DBA and Al3+, and exhibited strong green fluorescence emission behavior, with LODs of 50.61 and 38.59 nmol/L, respectively. Therefore, this study successfully synthesized a ratiometric fluorescence host-guest sensor material, which provides a new method for the detection of Al3+.
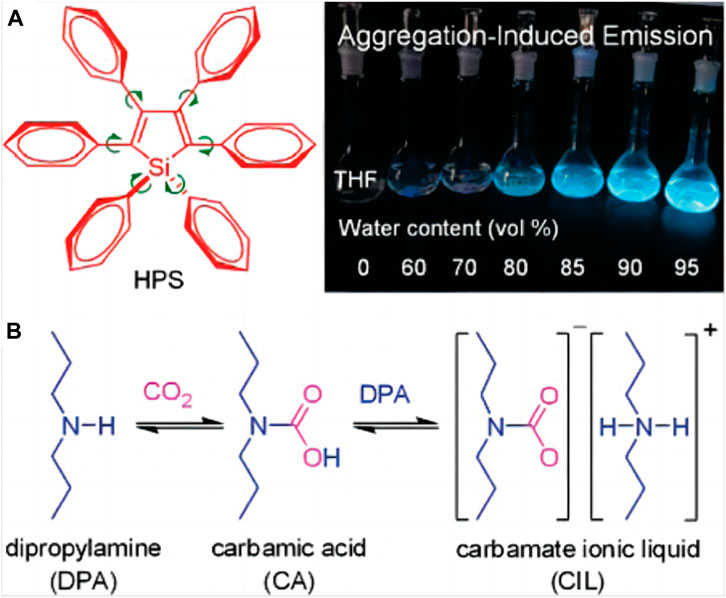
Figure 12. (A) Fluorescence images at different water contents. (B) Synthesis of CIL (Liu et al., 2010).
4.2.2 Biosensor
Sensors made of AIE-type polymers have high sensitivity, fast response and amplification effects, and will not interfere with the application process due to aggregation-induced quenching. Liu et al. (2011) and others invented a new concept of “light-up” biosensor for highly selective detection of D-glucose in the aqueous phase. The luminescence of TPE-centred diboronic acid molecules can be greatly enhanced when oligomers are formed with D-glucose molecules, due to the fact that the formation of oligomers results in the restriction of the intramolecular rotation of the aromatic ring of the TPE. Conversely, using probes to bind to other sugars, such as D-fructose, D-galactose, and D-mannose, yields no enhanced fluorescence due to the inability of these sugars to form oligomers with this fluorophore (Figure 13). Lu et al. (2020) reported for the first time the application of Ru(II) complexes with AIECL activity and their non-selective RNA probes. Dichlorobis (1,10-phenanthroline)ruthenium (II) (Ru2 (phen)2Cl2) was found to exhibit significant AIE and AIECL properties in a mixed solution of H2O-MeCN. When the moisture content is as high as 70%, its AIE strength and AIECL intensity peak, which are 5.7 and 120 times higher than those in pure MeCN, respectively. The intensity of ECL in this system is significantly affected by the type of nucleic acid in solution. This study has opened up a way to identify various nucleic acids, and is of great significance in many research fields such as biosensors and bioimaging probes.
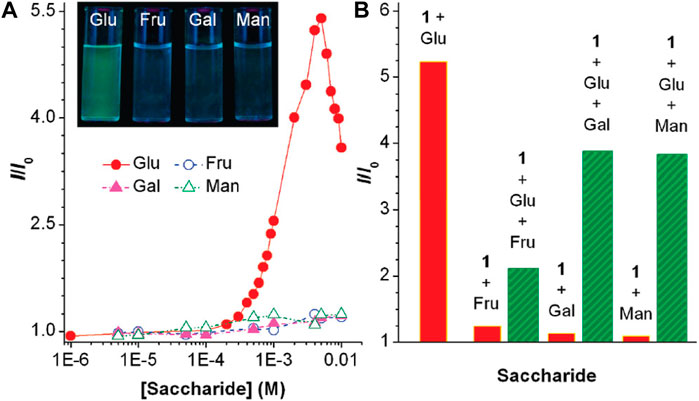
Figure 13. (A) Change in FL intensity at different sugar concentrations (B) FL response of 1 (50 μM) in the presence of interferences (Liu et al., 2011).
4.3 Biomonitoring, imaging and therapeutic applications
AIE polymers use the spontaneous aggregation process to inhibit non-radiative transitions, exhibiting synergistic amplification effects, showing advantages in bioimaging applications with high signal-to-noise ratios. At the same time, the ease of modification and versatility of AIE polymers make them responsive and specific to changes in the microenvironment, so they have high potential for development in biological applications (Dai et al., 2019; Zheng et al., 2020).
4.3.1 Biomonitoring applications
Researchers have used fluorescent probes to achieve direct visualisation of lesion sites and cells (Tian et al., 2019).Although traditional fluorescent molecules have been heavily developed and widely used, their fluorescence tends to diminish or burst at high concentrations, thus limiting their practical applications (Ding et al., 2013; Mei et al., 2018).Therefore, the design of fluorescent probes using AIE molecules for the detection of biological targets and imaging of biological samples has become a current research hotspot. Peptides are usually composed of 20 natural amino acids and some non-canonical amino acids, which play very important roles in biological functions (Zhang et al., 2021), such as tissue targeting (Lee et al., 2010; Araste et al., 2018; Wang and Hu, 2019), membrane penetration (Green et al., 1989; Lundberg and Langel, 2003; Temsamani and Vidal, 2004; Lehto et al., 2016; Nakayama et al., 2018), endosome escape (Midoux and Monsigny, 1999; Brogden, 2005; Lo and Wang, 2008; Schellinger et al., 2013), cellular transport (Carnevale et al., 2018), and nuclear localisation (Robbins et al., 1991; Xu et al., 2016). Modifying these short peptides with different functions as functional modules in AIE-based fluorescent probes (MPAPs) can make the fluorescent probes have good biocompatibility, excellent biodegradability, good bioactivity and rich diversity, etc (Yang et al., 2020).The introduction of peptides also improves the hydrophilicity of AIE molecules and they also favour their application in biomedical fields. In order to accurately target the target cells and locate in the nucleus for long-lasting and low-toxicity tracing of tumour cells, Cheng et al. (2017) designed and synthesized a “T” probe, TCNTP (Figures 14, 15). The probe was obtained by covalently linking a functional peptide (TCNT) and a fluorescent imaging agent (PyTPE) via a cuprous-catalysed azide and alkyne click reaction. TCNT consists of four modules, includes targeted cyclic peptide (cNGR) with high affinity for aminopeptidase N (CD13), cell-penetrating peptide (CPP), nuclear localisation signal peptide (NLS) and targeted peptide (RGD) that selectively interacts with integrin αv β3. Assisted by functional peptides, the TCNTP probe selectively targeted the nuclei of A375 cells overexpressing both integrin αv β3 and CD13 and showed intense yellow fluorescence. The experimental results show that the modular TCNTP probe can not only precisely target specific cell lines and transport biomolecules to the nucleus, but also achieve long-lasting tracking transport.
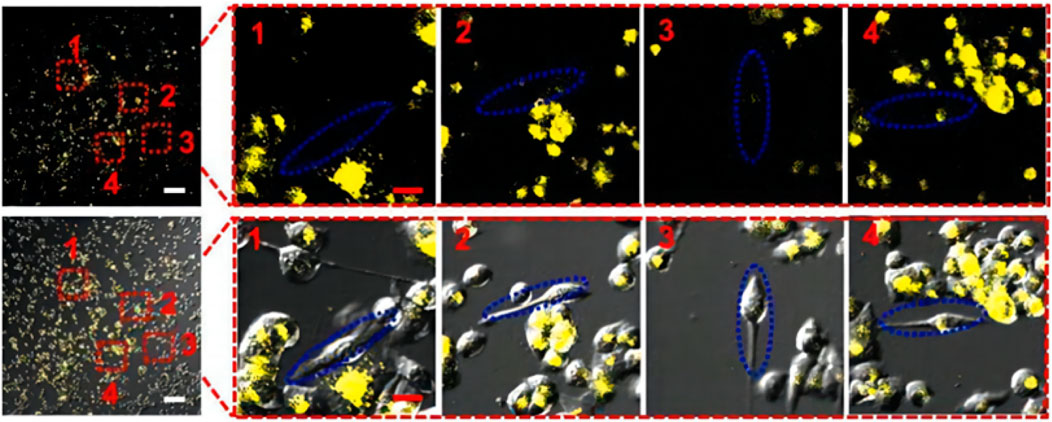
Figure 14. A375 cells and unstained MDAMB231 cells (blue markings) (Cheng et al., 2017).
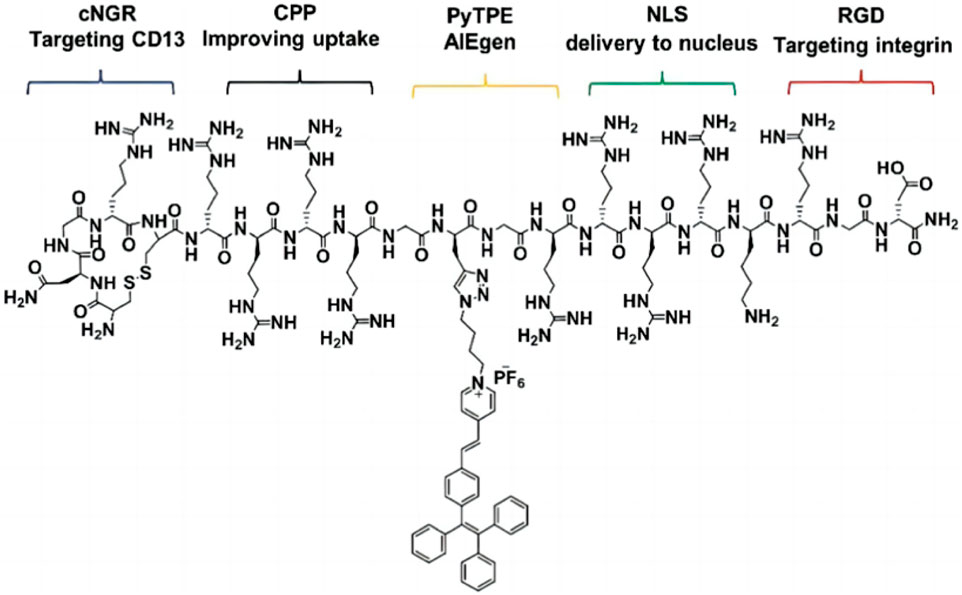
Figure 15. The chemical structures of TCNTP (Cheng et al., 2017).
4.3.2 Bio-imaging applications
AIE polymer probes are characterised by their tunable emission wavelength (from UV to NIR), tunable size (from tens of nanometres to hundreds of nanometres), high fluorescence intensity, good photostability, good aqueous dispersion and low cytotoxicity. It has been successfully applied to in vitro/in vivo imaging. Lu et al. (2012) synthesised a series of novel amphiphilic poly-N-(2-hydroxypropyl) methacrylamide based poly-N-(2-hydroxypropyl) methacrylamide through the free-radical polymerisation reaction of a monomer/hydrophobic fluorophore with AIE properties and N-(2-hydroxypropyl) methacrylamide. copolymer AIE fluorophores. The experiments show that the aggregation of AIE fluorophores in the micelle core contributes to the stronger aggregation of AIE segments, resulting in higher quantum efficiency.The polymer has good water solubility, allowing hydrophobic AIE materials to be applied under biological conditions. The polymer was endocytosed by 2 experimental cell lines [human glioblastoma cerebri and human oesophageal precancerous cells (CP-A)] and distributed into the cytoplasm. The polymer was not cytotoxic to either of the 2 cell lines at a polymer mass concentration of 1 mg/mL (Figures 16, 17).
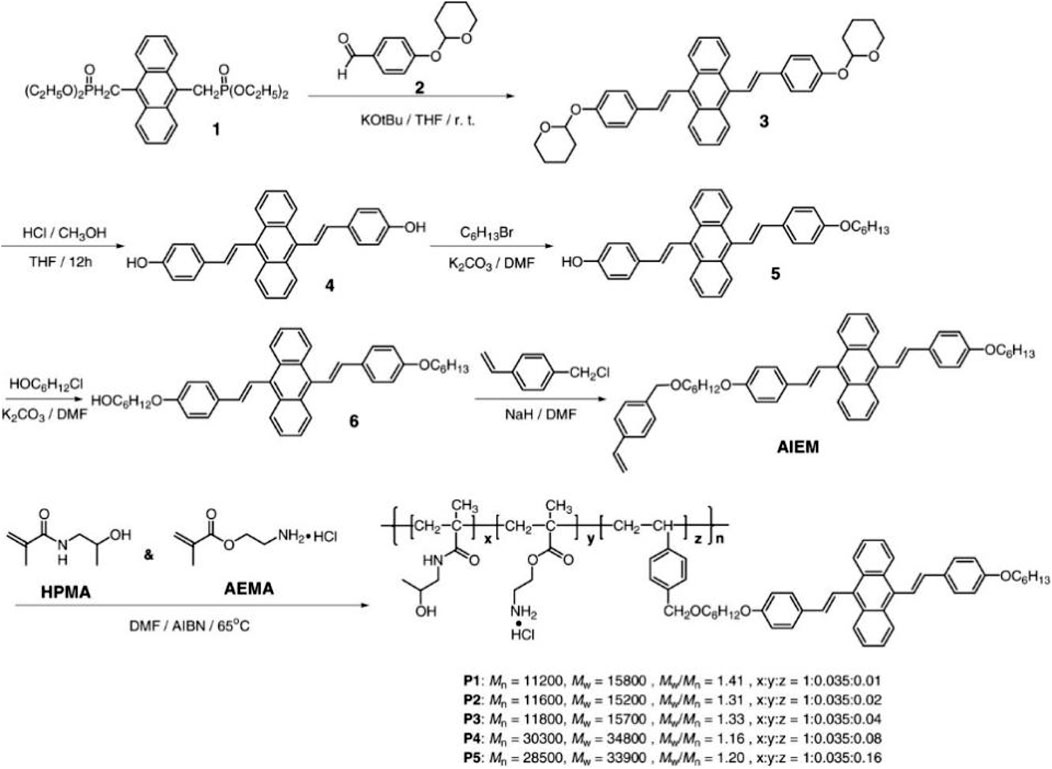
Figure 16. Synthesis method of AIE monomer and copolymer (Lu et al., 2012).
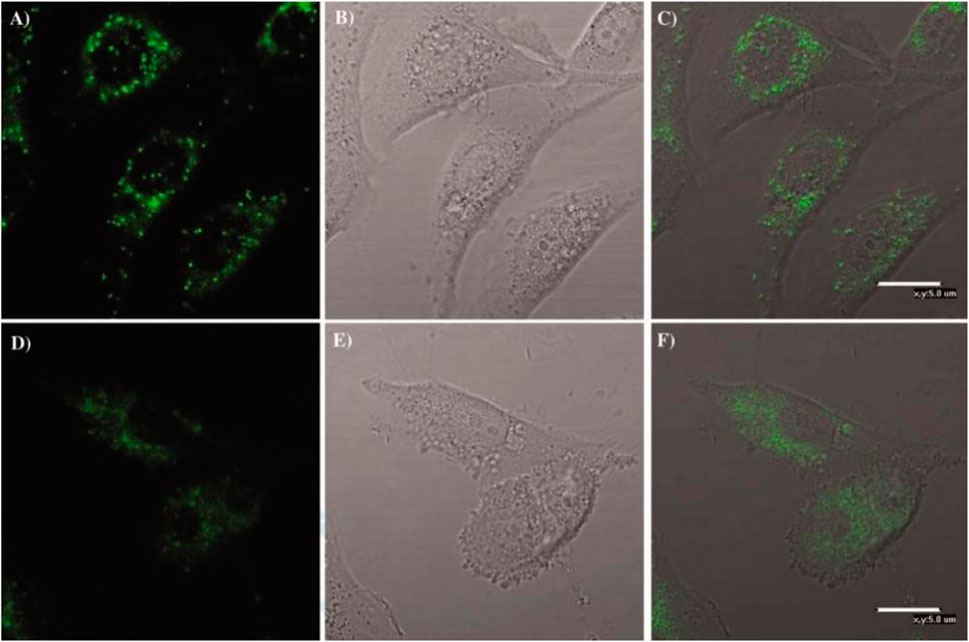
Figure 17. Amphiphilic AIE polymers that can be applied to the imaging of biological cells. Fluorescence image (A.D). Brightfield image (B.E). (A) superimposes (B–D) superimposes (E, F) (Lu et al., 2012).
In addition Seo et al. (2016) performed signal enhancement by designing and constructing nanoparticle imaging probes (with a size of about 26 nm) with unique photonic characteristics, to wit: 1) Peroxide is an energy donor material that produces electron excitation energy that can be used in response to inflammation to produce H2O2; 2) Conjugated polymers with low bandgap and bright NIR emission show AIE properties; 3) Photons across the energy gap transfer the resulting excitation energy to the emitter for effective excitation. Its energy-relaying nano-integration in water showed a 50-fold enhanced sensing signal compared to a dissolved mixture of AIE polymer and peroxalate in tetrahydrofuran. In addition to detecting H2O2 down to 10–9 mol/L, the enhanced chemiluminescence has a considerable tissue penetration depth (>12 mm) under ex vivo conditions, enabling deep imaging of mice with peritonitis in the model (Figure 18).
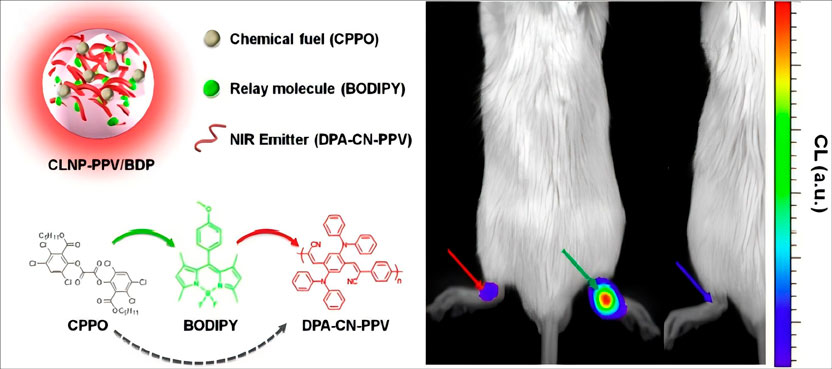
Figure 18. AIE nanoparticles capable of detecting bio-marker H2O2 (Seo et al., 2016).
4.3.3 Applications of biotherapy
Using the host-guest method, Liu et al. (2021) used a carboxylate-modified column (Luo et al., 2001) aryl hydrocarbon H as the supramolecular host and a fluorophore G with tetrastyrene nucleus with AIE properties as the guest to obtain the host-guest complexes H⊃G. They found that the fluorescence of H ⊃ G was much stronger than that of free G (Figure 19), and then prepared SNPs with high emissive properties from H ⊃ G by a nanoprecipitation method. The SNPs have good photostability and can be used for live cell imaging. It is important to note that SNPs are capable of forming drug delivery systems together with doxorubicin (DOX) and are self-imaging.The fluorescence of both SNPs and DOX, mediated by FRET and ACQ effects, was lost due to the energy transfer relay (ETR) effect. In acidic microenvironments, DOX-loaded SNPs@DOX fluoresce, allowing for the detection of drug release. It is important to note that the application of this material can greatly exert the anti-cancer efficacy of loaded drugs, which shows great potential in drug delivery systems for the purpose of imaging guidance.
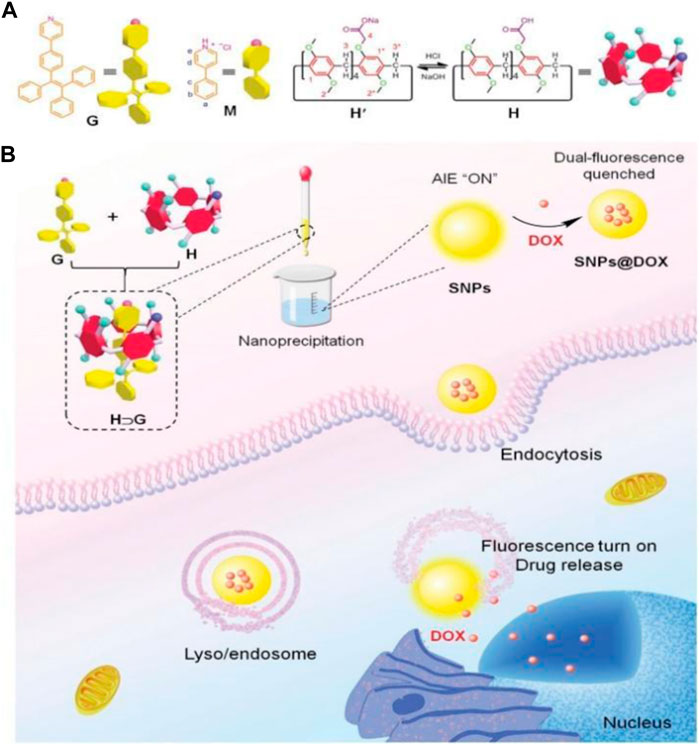
Figure 19. (A) Chemical structure of G, M, and H. (B) Schematic diagram of the use of SNPs as drug nanocarriers and imaging-guided drug delivery (Liu et al., 2021).
4.4 Other applications
4.4.1 Anti-counterfeiting applications
Huang et al. (2019) designed novel tetraphenylene (TPE) derivatives integrating aggregation-induced emission (AIE), polymorphic mechanical discolouration and self-recovering photochromism. The molecule is susceptible to grinding, heating and vapour fuming and exhibits corresponding emission colour shifts. Heated powders or single crystals exhibit reversible photochromism. Under ultraviolet light, it will take on a bright red color, but it will return to its original appearance soon after leaving ultraviolet light. Together, this reversible polymorphism, high contrast and fast-responding mechanical and photochromic properties provide a dual enhancement of multimodal assurance for advanced anti-counterfeiting. Zhang T. T. et al. (2023) developed two novel dual-mode orthogonal tunable fluorescence systems A and B using a supramolecular host-guest approach. When 0.2 equiv. of CB (Leung et al., 2014) is added to the guest molecular solution of the fluorescent dye peryldimide (PDI-C6)@-7-hydroxycoumarin, System A produces blue, yellow, and white fluorescence under a UV lamp at 25°C, 365 nm.When quinine sulfate at 0.4 or 0.5 equiv. is added to a solution of PDI-C6 (10 μm) @CB (Leung et al., 2014) (20 μm) molecules, wash B can be used to distinguish white luminescent materials. The light-emitting system has potential application value in the fields of anti-counterfeiting, 3D printing and so on.
4.4.2 Explosive detection applications
Zhu et al. (2022) synthesised helical mesoporous organic-inorganic hybrid silica nanofibres by co-condensation of 1,2-bis (triethoxysilyl) ethane with tetra-phenylene-containing (TPE) triethoxysilane, a polymer that emits blue fluorescence.The pore channels of the hybridised silica are arranged in a hexagonal shape. Layered mesopores were identified on the nanofibre surface. As the weight ratio of TPE increases, the fluorescence quantum yield also increases. These hybrid silica exhibit a sensitive fluorescence burst response to nitroaromatic explosive picric acids and can be used for explosives detection. Li et al. (2020) reported a novel AIE ultra-sensitive fluorescence sensor based on a tripod naphthalimide derivative (NQ). The sensor is used for the sensitive detection of picric acid (PA), an explosive analogue in the model, with high sensitivity and selectivity, with a minimum detection limit (LOD) of 4.73 × 10−8 mol/L. In addition, under natural light and ultraviolet irradiation, the identification of PA by S-TNQ solid powder can be visually observed, which has potential application value in the field of explosives detection.
4.4.3 Solar cell applications
Li et al. (2019) prepared bi-anchored phenothiazine core sensitisers (YL1-YL4) with tetraphenylethylene (TPE) entities as N-substituents for dye-sensitised solar cells (DSSC). The YL4-based DSSC exhibited optimal solar-electric conversion efficiencies of up to 9.79% and 10.87% without and with CDCA co-adsorbent, respectively. These figures are better than the values obtained from previous designs. YL Dye features a double layer of masking to effectively block dark current: an internal mask to prevent anchoring of the skeleton and an external mask to prevent clogged TPE units. The plugged TPE also enhances the dye stability of the TiO2 surface. The device achieves a maximum cellular efficiency of 27.54% at 187 lux and a minimum of 24.98% at 1025 lux and 26.81% at 597 lux under the Philips T5 lamp. This shows great potential for the Internet of Things (IoT) and the value of research in solar cells. Hu et al. (2021) developed a two-donor supramolecular artificial LHS (TPE-containing metal-ring compound L-3 and DSA-dinitrile-containing guest L-4) through metal-ligand coordination interaction and host-guest interaction. The material exhibits higher energy transfer efficiency and antenna effect, and exhibits good capture ability for ultraviolet and blue visible light. Compared with single-donor LHS, the energy transfer efficiency is about 0.29 times more (361 nm excitation) and the antenna effect is about 1.9 times more (415 nm excitation). Therefore, this study provides a unique strategy for harnessing solar energy through artificial LHS.
4.4.4 Environmental science applications
In 2020, Feng et al. (2020) designed and synthesized an AIE active formate oligomer (OU) gelling agent by a simple “one-pot” method, which self-assembled into a supramolecular gel (OUG) through hydrogen bonding, π-π stacking, and van der Waals interaction. Due to the dynamic characteristics of these non-covalent bonds, OUG shows stimulus responses to a variety of factors. For example, Fe3+,HSO4− and F− exhibit highly sensitive and reversible response characteristics, and the lowest detection limit is in the range of 5.89 × 10−9 −8.17 × 10−8 mol/L. What’s more, OUG can absorb up to 97.5% Fe3+ from the aqueous environment.OUG acts as a reversible fluorescence sensor switch through competitive cation-π and cation-anion interactions. This material is simple to synthesize, low cost and highly sensitive, and has great application potential in sensing and preventing heavy metal ion pollution.
5 Summary and prospect
The AIE study highlights the importance of polymeric and molecular science for the development of materials. Over the past years, much has been achieved in AIE research, including the understanding of its mechanism, structure-performance relationships, and applications.Although supramolecular luminescent materials have developed rapidly in recent years, there are still some problems that need to be studied urgently: 1) A variety of non-covalent interactions in most supramolecular assembly systems can interact with each other and coordinate with each other, which can greatly affect the photophysical properties of supramolecular assemblies. 2) The stability of general supramolecular luminescent materials is relatively poor, which is both an advantage and a disadvantage, and the balance should be grasped according to the actual situation; 3) The non-covalent bond construction of supramolecular materials is easily degraded, so it is key to prepare supramolecular luminescent materials with low biotoxicity, high biocompatibility and good water solubility through structural modification, so as to further promote their applications in the fields of drug delivery, biosensing and imaging. As a new class of materials for polymeric scientific research, AIE continues to integrate with materials, biology, energy, environment and other research fields, breathing new life into these areas. With more researchers beginning to work in this domain, more and more AIE products will be used in all aspects of life, becoming an indispensable part.
Author contributions
YP: Writing–original draft. LL: Writing–original draft. XC: Writing–original draft. JZ: Writing–review and editing. CL: Writing–review and editing.
Funding
The author(s) declare that no financial support was received for the research, authorship, and/or publication of this article.
Conflict of interest
The authors declare that the research was conducted in the absence of any commercial or financial relationships that could be construed as a potential conflict of interest.
Publisher’s note
All claims expressed in this article are solely those of the authors and do not necessarily represent those of their affiliated organizations, or those of the publisher, the editors and the reviewers. Any product that may be evaluated in this article, or claim that may be made by its manufacturer, is not guaranteed or endorsed by the publisher.
References
Araste, F., Abnous, K., Hashemi, M., Taghdisi, S. M., Ramezani, M., and Alibolandi, M. (2018). Peptide-based targeted therapeutics: focus on cancer treatment. J. Control. Release 292, 141–162. doi:10.1016/j.jconrel.2018.11.004
Bin, X., Luo, W., Yuan, W., and Zhang, Y. (2016). Clustering-triggered emission of poly(n-hydroxysuccinimide methacrylate). Acta Chimi Sin. 74, 935–941. doi:10.6023/A16080423
Bin Chen, B., Li, R. S., Liu, M. L., Zhang, H. Z., and Huang, C. Z. (2017). Self-exothermic reaction prompted synthesis of single-layered graphene quantum dots at room temperature. Chem. Commun. 53, 4958–4961. doi:10.1039/C7CC00546F
Bolton, O., Lee, D., Jung, J., and Kim, J. (2014). Tuning the photophysical properties of metal-free room temperature organic phosphors via compositional variations in bromobenzaldehyde/dibromobenzene mixed crystals. Chem. Mater. 26, 6644–6649. doi:10.1021/cm503678r
Brogden, K. A. (2005). Antimicrobial peptides: pore formers or metabolic inhibitors in bacteria? Nat. Rev. Microbiol. 3, 238–250. doi:10.1038/nrmicro1098
Bryce, M. R. (2017). Aggregation-induced delayed fluorescence (AIDF) materials: a new break-through for nondoped OLEDs. Sci. China Chem. 60, 1561–1562. doi:10.1007/s11426-017-9161-4
Carnevale, K. J. F., Muroski, M. E., Vakil, P. N., Foley, M. E., Laufersky, G., Kenworthy, R., et al. (2018). Selective uptake into drug resistant mammalian cancer by cell penetrating peptide-mediated delivery. Bioconjug Chem. 29, 3273–3284. doi:10.1021/acs.bioconjchem.8b00429
Chandra, B. P., Chandra, V. K., and Jha, P. (2013). Models for intrinsic and extrinsic fracto-mechanoluminescence of solids. J. Lumin 135, 139–153. doi:10.1016/J.JLUMIN.2012.10.009
Chandra, B. P., Chandra, V. K., Jha, P., Patel, R., Shende, S. K., Thaker, S., et al. (2012). Fracto-mechanoluminescence and mechanics of fracture of solids. J. Lumin 132, 2012–2022. doi:10.1016/J.JLUMIN.2012.03.001
Chen, D., Zhan, J., Zhang, M., Zhang, J., Tao, J., Tang, D., et al. (2015). A fluorescent supramolecular polymer with aggregation induced emission (AIE) properties formed by crown ether-based host-guest interactions. Polym. Chem. 6, 25–29. doi:10.1039/c4py01206b
Chen, J., Xie, Z., Lam, J. W. Y., Law, C. C. W., and Tang, B. Z. (2003). Silole-containing polyacetylenes. Synthesis, thermal stability, light emission, nanodimensional aggregation, and restricted intramolecular rotation. Macromolecules 36, 1108–1117. doi:10.1021/ma0213504
Chen, T., Yang, S., Chai, J., Song, Y., Fan, J., Rao, B., et al. (2017). Crystallization-induced emission enhancement: a novel fluorescent Au-Ag bimetallic nanocluster with precise atomic structure. Sci. Adv. 3, e1700956. doi:10.1126/sciadv.1700956
Cheng, Y., Sun, C. L., Ou, X. W., Liu, B. F., Lou, X. D., and Xia, F. (2017). Dual-targeted peptide-conjugated multifunctional fluorescent probe with AIEgen for efficient nucleus-specific imaging and long-term tracing of cancer cells. Chem. Sci. 8, 4571–4578. doi:10.1039/c7sc00402h
Cuc, T. T. K., Nhien, P. Q., Khang, T. M., Chen, H.-Y., Wu, C.-H., Hue, B.-T. B., et al. (2021). Controllable FRET behaviors of supramolecular host-guest systems as ratiometric aluminum ion sensors manipulated by tetraphenylethylene-functionalized macrocyclic host donor and multistimuli-responsive fluorescein-based guest acceptor. ACS Appl. Mater Interfaces 13, 20662–20680. doi:10.1021/acsami.1c02994
Dai, C., Yang, D., Fu, X., Chen, Q., Zhu, C., Cheng, Y., et al. (2015). A study on tunable AIE (AIEE) of boron ketoiminate-based conjugated polymers for live cell imaging. Polym. Chem. 6, 5070–5076. doi:10.1039/c5py00733j
Dai, Y., Wu, D., Lin, S., Ma, X., Zhang, X., and Xia, F. (2019). pH-responsive polymeric micelles with tunable aggregation-induced emission and controllable drug release. J. Nanoparticle Res. 21, 5. doi:10.1007/s11051-018-4447-4
Ding, D., Li, K., Liu, B., and Tang, B. Z. (2013). Bioprobes based on AIE fluorogens. Acc. Chem. Res. 46, 2441–2453. doi:10.1021/ar3003464
Dong, Y., Lam, J. W. Y., Qin, A., Li, Z., Sun, J., Sung, H. H. Y., et al. (2006). Switching the light emission of (4-biphenylyl)phenyldibenzofulvene by morphological modulation: crystallization-induced emission enhancement. Chem. Commun. 7, 40–42. doi:10.1039/B613157C
Duan, Y. C., Wen, L. L., Gao, Y., Wu, Y., Zhao, L., Geng, Y., et al. (2018). Fluorescence, phosphorescence, or delayed fluorescence? - a theoretical exploration on the reason why a series of similar organic molecules exhibit different luminescence types. J. Phys. Chem. C 122, 23091–23101. doi:10.1021/ACS.JPCC.8B06533
Fan, M., Xu, Z., Jiang, Y., Zhang, Y., and Xu, G. (2024) “Method for screening aggregation-induced luminescent molecule based on machine learning for use in biomedicine fluorescence imaging,” in Involves screening AIE molecule whose wavelength meets preset requirement according to predicted wavelength information of each AIE molecule.
Feng, Y. L., Jiang, N., Zhu, D. X., Su, Z. M., and Bryce, M. R. (2020). Supramolecular oligourethane gel as a highly selective fluorescent “on-off-on” sensor for ions. J. Mater Chem. C Mater 8, 11540–11545. doi:10.1039/d0tc02381g
Furue, R., Nishimoto, T., Park, I. S., Lee, J., and Yasuda, T. (2016). Aggregation induced delayed fluorescence based on donor/acceptor-tethered janus carborane triads: unique photophysical properties of nondoped OLEDs. Angew. Chem. 55, 7171–7175. doi:10.1002/anie.201603232
Gao, Y. J., Chang, X. P., Liu, X. Y., Li, Q. S., Cui, G., and Thiel, W. (2017). Excited-state decay paths in tetraphenylethene derivatives. J. Phys. Chem. A 121, 2572–2579. doi:10.1021/acs.jpca.7b00197
Gong, Y., Tan, Y., Li, H., Zhang, Y., Yuan, W., Zhang, Y., et al. (2013). Crystallization-induced phosphorescence of benzils at room temperature. Sci. China Chem. 56, 1183–1186. doi:10.1007/s11426-013-4930-9
Green, M., Ishino, M., and Loewenstein, P. M. (1989). Mutational analysis of HIV-1 Tat minimal domain peptides - identification of trans-dominant mutants that suppress HIV-Ltr-driven gene-expression. Cell. 58, 215–223. doi:10.1016/0092-8674(89)90417-0
Gu, N., Sun, Z., Song, L., Du, P., and Xiong, J. (2023). Aggregation-induced emission materials for high-efficiency perovskite solar cells. Chemphyschem 24, e202200919. doi:10.1002/cphc.202200919
Gu, P. Y., Lu, C. J., Hu, Z. J., Li, N. J., Zhao, T. T., Xu, Q. F., et al. (2013). The AIEE effect and two-photon absorption (TPA) enhancement induced by polymerization: synthesis of a monomer with ICT and AIE effects and its homopolymer by ATRP and a study of their photophysical properties. J. Mater Chem. C Mater 1, 2599–2606. doi:10.1039/c3tc00738c
Gu, X., Zhang, X., Ma, H., Jia, S., Zhang, P., Zhao, Y., et al. (2018). Corannulene-incorporated AIE nanodots with highly suppressed nonradiative decay for boosted cancer phototheranostics in vivo. Adv. Mater. 30, 1801065. doi:10.1002/ADMA.201801065
Guo, J., Zhao, Z., and Tang, B. Z. (2018). Purely organic materials with aggregation-induced delayed fluorescence for efficient nondoped OLEDs. Adv. Opt. Mater 6, 1800264. doi:10.1002/ADOM.201800264
Gupta, A. (2019). Aggregation-induced emission: a tool for sensitive detection of amines. ChemistrySelect 4, 12848–12860. doi:10.1002/slct.201903248
He, B., Zhang, J., Wang, J., Wu, Y., Qin, A., and Tang, B. Z. (2020). Preparation of multifunctional hyperbranched poly(β-aminoacrylate)s by spontaneous amino-yne click polymerization. Macromolecules 53, 5248–5254. doi:10.1021/acs.macromol.0c00813
He, Z., Ke, C., and Tang, B. Z. (2018). Journey of aggregation-induced emission research. ACS Omega 3, 3267–3277. doi:10.1021/acsomega.8b00062
Hong, Y., Lam, J. W. Y., and Tang, B. Z. (2011). Aggregation-induced emission. Chem. Soc. Rev. 40, 5361–5388. doi:10.1039/c1cs15113d
Hong, Y. N., Lam, J. W. Y., and Tang, B. Z. (2009). Aggregation-induced emission: phenomenon, mechanism and applications. Chem. Commun., 4332–4353. doi:10.1039/b904665h
Hou, Y. (2024). New aggregation-induced emission-type double D-pi-A structure fluorescent dye i.e. in (4-((E)-2-(4-((E)-2-(pyridin-4-yl)ethenyl)phenyl)ethenyl)pyridine containing compound) useful in the technical field of organic luminescent materials.
Hu, R., Leung, N. L. C., and Tang, B. Z. (2014). AIE macromolecules: syntheses, structures and functionalities. Chem. Soc. Rev. 43, 4494–4562. doi:10.1039/C4CS00044G
Hu, Y.-X., Jia, P.-P., Zhang, C.-W., Xu, X.-D., Niu, Y., Zhao, X., et al. (2021). A supramolecular dual-donor artificial light-harvesting system with efficient visible light-harvesting capacity. Org. Chem. Front. 8, 5250–5257. doi:10.1039/d1qo00771h
Huang, G., Xia, Q., Huang, W., Tian, J., He, Z., Li, B. S., et al. (2019). Multiple anti-counterfeiting guarantees from a simple tetraphenylethylene derivative - high-contrasted and multi-state mechanochromism and photochromism. Angew. Chem. Int. Ed. Engl. 58, 17814–17819. doi:10.1002/anie.201910530
Huang, J., Nie, H., Zeng, J., Zhuang, Z., Gan, S., Cai, Y., et al. (2017). Highly efficient nondoped OLEDs with negligible efficiency roll-off fabricated from aggregation-induced delayed fluorescence luminogens. Angew. Chem. Int. Ed. 56, 12971–12976. doi:10.1002/ANIE.201706752
Jiang, X., Yu, Z., Ma, C., Wang, D., Wu, Y., Shi, C., et al. (2021). Aggregation-induced emission molecule microwire-based specific organic vapor detector through structural modification. ACS Appl. Mater Interfaces 13, 12501–12508. doi:10.1021/acsami.0c22975
Kuo, S. W., and Hong, J. L. (2024). Tetraphenylthiophene-functionalized poly(N -isopropylacrylamide): probing LCST with aggregation-induced emission.
Lee, M. M. S., Yu, E. Y. Y., Yan, D., Chau, J. H. C., Wu, Q., Lam, J. W. Y., et al. (2023). The role of structural hydrophobicity on cationic amphiphilic aggregation-induced emission photosensitizer-bacterial interaction and photodynamic efficiency. ACS Nano 17, 17004–17020. doi:10.1021/acsnano.3c04266
Lee, S., Xie, J., and Chen, X. Y. (2010). Peptide-based probes for targeted molecular imaging. Biochemistry 49, 1364–1376. doi:10.1021/bi901135x
Lehto, T., Ezzat, K., Wood, M. J. A., and EL Andaloussi, S. (2016). Peptides for nucleic acid delivery. Adv. Drug Deliv. Rev. 106, 172–182. doi:10.1016/j.addr.2016.06.008
Leung, N. L. C., Xie, N., Yuan, W., Liu, Y., Wu, Q., Peng, Q., et al. (2014). Restriction of intramolecular motions: the general mechanism behind aggregation-induced emission. Chem. – A Eur. J. 20, 15349–15353. doi:10.1002/CHEM.201403811
Li, C. T., Kuo, Y. L., Kumar, C. P., Huang, P. T., and Lin, J. T. (2019). Tetraphenylethylene tethered phenothiazine-based double-anchored sensitizers for high performance dye-sensitized solar cells. J. Mater Chem. A Mater 7, 23225–23233. doi:10.1039/c9ta09025h
Li, Q., and Blancafort, L. (2013). A conical intersection model to explain aggregation induced emission in diphenyl dibenzofulvene. Chem. Commun. 49, 5966–5968. doi:10.1039/C3CC41730A
Li, Y.-J., Zhang, Y.-F., Zhang, Y.-M., Wang, Z.-H., Yang, H.-L., Yao, H., et al. (2020). Tripodal naphthalimide assembled novel AIE supramolecular fluorescent sensor for rapid and selective detection of picric acid. Dyes Pigments 181, 108563. doi:10.1016/j.dyepig.2020.108563
Liu, B., Nie, H., Zhou, X., Hu, S., Luo, D., Gao, D., et al. (2016). Manipulation of charge and exciton distribution based on blue aggregation-induced emission fluorophors: a novel concept to achieve high-performance hybrid white organic light-emitting diodes. Adv. Funct. Mater 26, 776–783. doi:10.1002/adfm.201503368
Liu, D., Sun, K., Zhao, G., Wei, J., Duan, J., Xia, M., et al. (2019a). Spatial separation of a TADF sensitizer and fluorescent emitter with a core-dendron system to block the energy loss in deep blue organic light-emitting diodes. J. Mater Chem. C Mater 7, 11005–11013. doi:10.1039/C9TC03334C
Liu, D. H., Du, J. S., Qi, S. L., Li, M. Y., Wang, J. F., Liu, M. H., et al. (2021). Supramolecular nanoparticles constructed from pillar[5]arene-based host-guest complexation with enhanced aggregation-induced emission for imaging-guided drug delivery. Mater Chem. Front. 5, 1418–1427. doi:10.1039/d0qm00974a
Liu, Y., Deng, C., Tang, L., Qin, A., Hu, R., Sun, J. Z., et al. (2011). Specific detection of d-glucose by a tetraphenylethene-based fluorescent sensor. J. Am. Chem. Soc. 133, 660–663. doi:10.1021/ja107086y
Liu, Y., Tang, Y. H., Barashkov, N. N., Irgibaeva, I. S., Lam, J. W. Y., Hu, R. R., et al. (2010). Fluorescent chemosensor for detection and quantitation of carbon dioxide gas. J. Am. Chem. Soc. 132, 13951–13953. doi:10.1021/ja103947j
Liu, Z., Zou, H., Zhao, Z., Zhang, P., Shan, G. G., Kwok, R. T. K., et al. (2019b). Tuning organelle specificity and photodynamic therapy efficiency by molecular function design. ACS Nano 13, 11283–11293. doi:10.1021/acsnano.9b04430
Lo, S. L., and Wang, S. (2008). An endosomolytic Tat peptide produced by incorporation of histidine and cysteine residues as a nonviral vector for DNA transfection. Biomaterials 29, 2408–2414. doi:10.1016/j.biomaterials.2008.01.031
Lu, H. G., Su, F. Y., Mei, Q., Zhou, X. F., Tian, Y. Q., Tian, W. J., et al. (2012). A series of poly[N-(2-hydroxypropyl)methacrylamide] copolymers with anthracene-derived fluorophores showing aggregation-induced emission properties for bioimaging. J. Polym. Sci. Part A-Polymer Chem. 50, 890–899. doi:10.1002/pola.25841
Lu, L., Zhang, L., Miao, W., Wang, X., and Guo, G. (2020). Aggregation-induced electrochemiluminescence of the dichlorobis(1,10-phenanthroline)ruthenium(II) (Ru(phen)2Cl2)/Tri-n-propylamine (TPrA) system in H2O-MeCN mixtures for identification of nucleic acids. Anal. Chem. 92, 9613–9619. doi:10.1021/acs.analchem.0c00799
Lundberg, P., and Langel, Ü. (2003). A brief introduction to cell-penetrating peptides. J. Mol. Recognit. 16, 227–233. doi:10.1002/jmr.630
Luo, J. D., Xie, Z. L., Lam, J. W. Y., Cheng, L., Chen, H. Y., Qiu, C. F., et al. (2001). Aggregation-induced emission of 1-methyl-1,2,3,4,5-pentaphenylsilole. Chem. Commun., 1740–1741. doi:10.1039/b105159h
Ma, C., Zhang, X., Wang, K., Zhang, X., Zhou, Y., Liu, H., et al. (2015). A biocompatible cross-linked fluorescent polymer prepared via ring-opening PEGylation of 4-arm PEG-amine, itaconic anhydride, and an AIE monomer. Polym. Chem. 6, 3634–3640. doi:10.1039/c5py00111k
Ma, H., Yang, M., Zhang, S., Yin, P., Wang, T., Yang, Y., et al. (2019). Two aggregation-induced emission (AIE)-active reaction-type probes: for real-time detecting and imaging of superoxide anions. ANALYST 144, 536–542. doi:10.1039/c8an01811a
Ma, J., Gu, Y., Ma, D., Lu, W., and Qiu, J. (2022). Insights into AIE materials: a focus on biomedical applications of fluorescence. Front. Chem. 10, 985578. doi:10.3389/fchem.2022.985578
Mei, J., Huang, Y., and Tian, H. (2018). Progress and trends in AIE-based bioprobes: a brief overview. ACS Appl. Mater Interfaces 10, 12217–12261. doi:10.1021/acsami.7b14343
Mei, J., Leung, N. L. C., Kwok, R. T. K., Lam, J. W. Y., and Tang, B. Z. (2015). Aggregation-induced emission: together we shine, united we soar. Chem. Rev 115, 11718–940. doi:10.1021/acs.chemrev.5b00263
Midoux, P., and Monsigny, M. (1999). Efficient gene transfer by histidylated polylysine/pDNA complexes. Bioconjug. Chem. 10, 406–11. doi:10.1021/bc9801070
Nakayama, N., Takaoka, S., Ota, M., Takagaki, K., and Sano, K. I. (2018). Effect of the aspect ratio of coiled-coil protein carriers on cellular uptake. Langmuir 34, 14286–14293. doi:10.1021/acs.langmuir.8b02616
Parashchuk, O. D., Mannanov, A. A., Konstantinov, V. G., Dominskiy, D. I., Surin, N. M., Borshchev, O. V., et al. (2018). Molecular self-doping controls luminescence of pure organic single crystals. Adv. Funct. Mater 28, 1800116. doi:10.1002/ADFM.201800116
Qin, A. J., Lam, J. W. Y., Tang, L., Jim, C. K. W., Zhao, H., Sun, J. Z., et al. (2009). Polytriazoles with aggregation-induced emission characteristics: synthesis by click polymerization and application as explosive chemosensors. Macromolecules 42, 1421–1424. doi:10.1021/ma8024706
Robbins, J., Dilworth, S. M., Laskey, R. A., and Dingwall, C. (1991). Two interdependent basic domains in nucleoplasmin nuclear targeting sequence: identification of a class of bipartite nuclear targeting sequence. Cell 64, 615–623. doi:10.1016/0092-8674(91)90245-T
Schellinger, J. G., Pahang, J. A., Johnson, R. N., Chu, D. S. H., Sellers, D. L., Maris, D. O., et al. (2013). Melittin-grafted HPMA-oligolysine based copolymers for gene delivery. Biomaterials 34, 2318–2326. doi:10.1016/j.biomaterials.2012.09.072
Seo, Y. H., Singh, A., Cho, H. J., Kim, Y., Heo, J., Lim, C. K., et al. (2016). Rational design for enhancing inflammation-responsive in vivo chemiluminescence via nanophotonic energy relay to near-infrared AIE-active conjugated polymer. Biomaterials 84, 111–118. doi:10.1016/j.biomaterials.2016.01.038
Song, B., He, B., Qin, A., and Tang, B. Z. (2018). Direct polymerization of carbon dioxide, diynes, and alkyl dihalides under mild reaction conditions. Am. Chem. Soc. 51, 42–48. doi:10.1021/acs.macromol.7b02109
Song, Z., Zhang, W., Jiang, M., Sung, H. H. Y., Kwok, R. T. K., Nie, H., et al. (2016). Synthesis of imidazole-based AIEgens with wide color tunability and exploration of their biological applications. Adv. Funct. Mater 26, 824–832. doi:10.1002/adfm.201503788
Sturala, J., Etherington, M. K., Bismillah, A. N., Higginbotham, H. F., Trewby, W., Aguilar, J. A., et al. (2017). Excited-state aromatic interactions in the aggregation-induced emission of molecular rotors. J. Am. Chem. Soc. 139, 17882–17889. doi:10.1021/jacs.7b08570
Sun, X. M., Liu, J., Li, Z. H., Fu, Y. P., Huang, T. T., Tang, Z. D., et al. (2023). Structure-property relationship on aggregation-induced emission properties of simple azine-based AIEgens and its application in metal ions detection. Chin. Chem. Lett. 34, 107792. doi:10.1016/j.cclet.2022.107792
Tan, K. M., Yan, M. N., Wang, Y. N., Xie, L. H., Huang, W., Zhang, H. M., et al. (2017). White organic light-emitting diodes based on exciton and electroplex dual emissions. Acta Physico-Chimica Sin. 33, 1057–1064. doi:10.3866/pku.whxb201702161
Tanaka, K., Yanagida, T., Hirose, A., Yamane, H., Yoshii, R., and Chujo, Y. (2015). Synthesis and color tuning of boron diiminate conjugated polymers with aggregation-induced scintillation properties. RSC Adv. 5, 96653–96659. doi:10.1039/c5ra20459c
Tang, B. Z., Zhan, X., Yu, G., Lee, P. P. S., Liu, Y., and Zhu, D. (2001). Efficient blue emission from siloles. J. Mater. Chem. 11, 2974–2978. doi:10.1039/B102221K
Tang, L., Jin, J. K., Qin, A., Zhang Yuan, W., Mao, Y., Mei, J., et al. (2009). A fluorescent thermometer operating in aggregation-induced emission mechanism: probing thermal transitions of PNIPAM in water. Chem. Commun., 4974–4976. doi:10.1039/B907382E
Tang, B. (2024) “New aggregation-induced emission (AIE) molecule-modified polyamino cationic polymer used as fluorescent probe in preparation of nanoparticles used for preparing cell imaging reagent,” in Cell staining reagent, and antibacterial reagent.
Temsamani, J., and Vidal, P. (2004). The use of cell-penetrating peptides for drug delivery. Drug Discov. Today 9, 1012–1019. doi:10.1016/s1359-6446(04)03279-9
Tian, M., Ma, Y., and Lin, W. (2019). Fluorescent probes for the visualization of cell viability. Acc. Chem. Res. 52, 2147–2157. doi:10.1021/acs.accounts.9b00289
Tseng, N. W., Liu, J., Ng, J. C. Y., Lam, J. W. Y., Sung, H. H. Y., Williams, I. D., et al. (2012). Deciphering mechanism of aggregation-induced emission (AIE): is E–Zisomerisation involved in an AIE process? Chem. Sci. 3, 493–497. doi:10.1039/C1SC00690H
Wan, H., Peiyang, Z., Feng, W., Hongliang, J., Jun, C., Chen, D., et al. (2018). Polyacrylic esters with a “one-is-enough” effect and investigation of their AIEE behaviours and cyanide detection in aqueous solution. Polym. Chem. 9, 3893–3899. doi:10.1039/c8py00705e
Wang, K., Zhang, X., Zhang, X., Yang, B., Li, Z., Zhang, Q., et al. (2015). Red fluorescent cross-linked glycopolymer nanoparticles based on aggregation induced emission dyes for cell imaging. Polym. Chem. 6, 1360–1366. doi:10.1039/c4py01452a
Wang, L., Yang, L., and Cao, D. (2014). Application of aggregation-induced emission (AIE) systems in sensing and bioimaging. Curr. Org. Chem. 18, 1028–1049. doi:10.2174/1385272819666140407214006
Wang, W. Z., and Hu, Z. Y. (2019). Targeting peptide-based probes for molecular imaging and diagnosis. Adv. Mater. 31, e1804827. doi:10.1002/adma.201804827
Wang, Y., Chen, M., Alifu, N., Li, S., Qin, W., Qin, A., et al. (2017). Aggregation-induced emission luminogen with deep-red emission for through-skull three-photon fluorescence imaging of mouse. ACS Nano 11, 10452–10461. doi:10.1021/acsnano.7b05645
Wei, Q., Poetzsch, R., Liu, X., Komber, H., Kiriy, A., Voit, B., et al. (2016). Hyperbranched polymers with high transparency and inherent high refractive index for application in organic light-emitting diodes. Adv. Funct. Mater 26. doi:10.1002/adfm.201504914
Wu, W., Ye, S., Huang, L., Xiao, L., Fu, Y., Huang, Q., et al. (2012). A conjugated hyperbranched polymer constructed from carbazole and tetraphenylethylene moieties: convenient synthesis through one-pot “A2 + B4” Suzuki polymerization, aggregation-induced enhanced emission, and application as explosive chemosensors and PLEDs. J. Mater Chem. 22, 6374–6382. doi:10.1039/C2JM16514G
Xu, R., Zhang, P., Shen, Q., Zhou, Y., Wang, Z., Xu, Y., et al. (2023). AIE nanocrystals: emerging nanolights with ultra-high brightness for biological application. Coord. Chem. Rev. 477, 214944. doi:10.1016/j.ccr.2022.214944
Xu, S., Wu, W., Cai, X., Zhang, C. J., Yuan, Y., Liang, J., et al. (2017). Highly efficient photosensitizers with aggregation-induced emission characteristics obtained through precise molecular design. Chem. Commun. 53, 8727–8730. doi:10.1039/C7CC04864E
Xu, Y. Y., Liang, W. L., Qiu, Y. S., Cespi, M., Palmieri, G. F., Mason, A. J., et al. (2016). Incorporation of a nuclear localization signal in pH responsive LAH4-L1 peptide enhances transfection and nuclear uptake of plasmid DNA. Mol. Pharm. 13, 3141–3152. doi:10.1021/acs.molpharmaceut.6b00338
Xue, B., Hou, A., Du, Y., Qi, Y., Jiang, H., Zhou, H., et al. (2023). AIE donor-dependent photosensitizer for enhance photodynamic antibacterial interface. Surfaces Interfaces 39, 102996. doi:10.1016/j.surfin.2023.102996
Yamaguchi, M., Ito, S., Hirose, A., Tanaka, K., and Chujo, Y. (2017). Control of aggregation-induced emission versus fluorescence aggregation-caused quenching by bond existence at a single site in boron pyridinoiminate complexes. Mater Chem. Front. 1, 1573–1579. doi:10.1039/C7QM00076F
Yan, L., Zhang, Y., Xu, B., and Tian, W. (2016). Fluorescent nanoparticles based on AIE fluorogens for bioimaging. Nanoscale 8, 2471–2487. doi:10.1039/c5nr05051k
Yan, Y., Jiang, L., Zhang, S., Shen, X., and Huang, C. (2022). Specific “light-up” sensor made easy: an aggregation induced emission monomer for molecular imprinting. Biosens. Bioelectron. 205, 114113. doi:10.1016/j.bios.2022.114113
Yang, J. L., Wei, J. M., Luo, F., Dai, J., Hu, J. J., Lou, X. D., et al. (2020). Enzyme-responsive peptide-based AIE bioprobes. Top. Curr. Chem. 378, 47. doi:10.1007/s41061-020-00311-9
Yang, M., Xu, D., Xi, W., Wang, L., Zheng, J., Huang, J., et al. (2013). Aggregation-induced fluorescence behavior of triphenylamine-based schiff bases: the combined effect of multiple forces. J. Org. Chem. 78, 10344–10359. doi:10.1021/jo401719c
Yang, Z., Tao, Y. U., Chen, M., Zhang, X., Jiarui, X. U., Xu, B., et al. (2009). A monomer and its polymer derived from carbazolyl triphenylethylene with aggregation-induced emission effect characteristics. Acta Polym. Sin. 009, 560–565. doi:10.3724/sp.j.1105.2009.00560
Ye, F.-Y., Hu, M., and Zheng, Y.-S. (2023). Advances and challenges of metal ions sensors based on AIE effect. Coord. Chem. Rev. 493, 215328. doi:10.1016/j.ccr.2023.215328
Ye, R., Liu, Y., Zhang, H., Su, H., Zhang, Y., Xu, L., et al. (2017). Non-conventional fluorescent biogenic and synthetic polymers without aromatic rings. Polym. Chem. 8, 1722–1727. doi:10.1039/C7PY00154A
Yin, X., Meng, F., and Wang, L. (2013). Thermosensitivity and luminescent properties of new tetraphenylethylene derivatives bearing peripheral oligo(ethylene glycol) chains. J. Mater Chem. C Mater 1, 6767–6773. doi:10.1039/C3TC31482K
Yuan, J., Xi, L., Juxiang, Y., and Zhen, L. (2022). Research progress of AIE hyperbranched polymers. J. Funct. Mater. 53, 12039–12046. doi:10.3969/j.issn.1001-9731.2022.12.006
Zalmi, G. A., Jadhav, R. W., Mirgane, H. A., and V Bhosale, S. (2022). Recent advances in aggregation-induced emission active materials for sensing of biologically important molecules and drug delivery system. Molecules 27, 150. doi:10.3390/molecules27010150
Zhan, L., Chen, Z., Gong, S., Xiang, Y., Ni, F., Zeng, X., et al. (2019). A simple organic molecule realizing simultaneous TADF, RTP, AIE, and mechanoluminescence: understanding the mechanism behind the multifunctional emitter. Angew. Chem. Int. Ed. 58, 17651–17655. doi:10.1002/ANIE.201910719
Zhan, R., Pan, Y., Manghnani, P. N., and Liu, B. (2017). AIE polymers: synthesis, properties, and biological applications. Macromol. Biosci. 17. doi:10.1002/mabi.201600433
Zhang, C., Zhao, Y., Li, D., Liu, J., Han, H., He, D., et al. (2019b). Aggregation-induced emission (AIE)-active molecules bearing singlet oxygen generation activities: the tunable singlet–triplet energy gap matters. Chem. Commun. 55, 1450–1453. doi:10.1039/C8CC09230C
Zhang, C.-W., Ou, B., Jiang, S.-T., Yin, G.-Q., Chen, L.-J., Xu, L., et al. (2018). Cross-linked AIE supramolecular polymer gels with multiple stimuli-responsive behaviours constructed by hierarchical self-assembly. Polym. Chem. 9, 2021–2030. doi:10.1039/C8PY00226F
Zhang, E., Hou, X., Han, X., Wang, S., Li, H., Jiang, D., et al. (2023a). A series of AIE dyes derived from shikimic acid: the thermo/pressure dependent fluorescence and application in the sensing of arginine. Dyes Pigments 210, 111041. doi:10.1016/j.dyepig.2022.111041
Zhang, H., Zeng, J., Luo, W., Wu, H., Zeng, C., Zhang, K., et al. (2019c). Synergistic tuning of the optical and electrical performance of AIEgens with a hybridized local and charge-transfer excited state. J. Mater Chem. C Mater 7, 6359–6368. doi:10.1039/c9tc01453e
Zhang, H., Zhao, Z., McGonigal, P. R., Ye, R., Liu, S., Lam, J. W. Y., et al. (2020). Clusterization-triggered emission: uncommon luminescence from common materials. Mater. Today 32, 275–292. doi:10.1016/J.MATTOD.2019.08.010
Zhang, T. T., Yang, X. N., Hu, J. H., Luo, Y., Liu, H. J., Tao, Z., et al. (2023b). New dual-mode orthogonal tunable fluorescence systems based on cucurbit [8]uril: white light, 3D printing, and anti-counterfeit applications. Chem. Eng. J. 452, 138960. doi:10.1016/j.cej.2022.138960
Zhang, W., Chen, Q., Wu, F., Dai, J., Ding, D. F., Wu, J., et al. (2021). Peptide-based nanomaterials for gene therapy. Nanoscale Adv. 3, 302–310. doi:10.1039/d0na00899k
Zhang, X., Du, L., Zhao, W., Zhao, Z., Xiong, Y., He, X., et al. (2019a). Ultralong UV/mechano-excited room temperature phosphorescence from purely organic cluster excitons. Nat. Commun. 10 (1), 5161–5210. doi:10.1038/s41467-019-13048-x
Zhao, W., He, Z., Lam, J. W. Y., Peng, Q., Ma, H., Shuai, Z., et al. (2016). Rational molecular design for achieving persistent and efficient pure organic room-temperature phosphorescence. Chem 1, 592–602. doi:10.1016/j.chempr.2016.08.010
Zhao, W., Liu, Z., Yu, J., Lu, X., Lam, J. W. Y., Sun, J., et al. (2021). Turning on solid-state luminescence by phototriggered subtle molecular conformation variations. Adv. Mater. 33, e2006844. doi:10.1002/adma.202006844
Zhao, Z., Gao, S., Zheng, X., Zhang, P., Wu, W., Kwok, R. T. K., et al. (2018). Rational design of perylenediimide-substituted triphenylethylene to electron transporting aggregation-induced emission luminogens (AIEgens) with high mobility and near-infrared emission. Adv. Funct. Mater 28, 1705609. doi:10.1002/ADFM.201705609
Zhao, Z., Lam, J. W. Y., and Tang, B. Z. (2010). Aggregation-induced emission of tetraarylethene luminogens. Curr. Org. Chem. 14, 2109–2132. doi:10.2174/138527210793351571
Zhao, Z., Lam, J. W. Y., and Tang, B. Z. (2012). Tetraphenylethene: a versatile AIE building block for the construction of efficient luminescent materials for organic light-emitting diodes. J. Mater Chem. 22, 23726–23740. doi:10.1039/C2JM31949G
Zhao, Z., Nie, H., Ge, C., Cai, Y., Xiong, Y., Qi, J., et al. (2017). Furan is superior to thiophene: a furan-cored AIEgen with remarkable chromism and OLED performance. Adv. Sci. 4, 1700005. doi:10.1002/ADVS.201700005
Zhao, Z., Zheng, X., Du, L., Xiong, Y., He, W., Gao, X., et al. (2019). Non-aromatic annulene-based aggregation-induced emission system via aromaticity reversal process. Nat. Commun. 10 (1 10), 2952–3010. doi:10.1038/s41467-019-10818-5
Zheng, X., Peng, Q., Zhu, L., Xie, Y., Huang, X., and Shuai, Z. (2016). Unraveling the aggregation effect on amorphous phase AIE luminogens: a computational study. Nanoscale 8, 15173–15180. doi:10.1039/C6NR03599J
Zheng, Z., Zhou, T., Hu, R., Huang, M., Ao, X., Chu, J., et al. (2020). A specific aggregation-induced emission-conjugated polymer enables visual monitoring of osteogenic differentiation. Bioact. Mater 5, 1018–1025. doi:10.1016/j.bioactmat.2020.06.020
Zheng, H. (2021). Preparing AIE nanoprobe useful in fluorescence imaging, comprises dissolving AIE molecule in organic solvent. in Mixing AIE solution with albumin aqueous solution and removing organic solvent in mixed solution.
Zhou, Q., Cao, B., Zhu, C., Xu, S., Gong, Y., Yuan, W. Z., et al. (2016). Clustering-triggered emission of nonconjugated polyacrylonitrile. Small 12, 6586–6592. doi:10.1002/SMLL.201601545
Zhou, X., Luo, W., Nie, H., Xu, L., Hu, R., Zhao, Z., et al. (2017). Oligo(maleic anhydride)s: a platform for unveiling the mechanism of clusteroluminescence of non-aromatic polymers. J. Mater Chem. C Mater 5, 4775–4779. doi:10.1039/C7TC00868F
Zhu, F., Fang, H., Liu, W., Li, H., Li, B., Li, Y., et al. (2022). Helical mesoporous organic-inorganic hybrid silica nanofibers prepared using a TPE-based silane for explosive detection. Mater Lett., 306.
Keywords: aggregation-induced emission molecular, luminescence, aggregation-caused quenching, application, synthesis
Citation: Pei Y, Liu L, Cao X, Zhou J and Liu C (2024) Advances in the study of AIE polymers. Front. Mater. 11:1446307. doi: 10.3389/fmats.2024.1446307
Received: 09 June 2024; Accepted: 15 July 2024;
Published: 14 August 2024.
Edited by:
Eugene Caldona, North Dakota State University, United StatesReviewed by:
Reymark Maalihan, North Dakota State University, United StatesAnas Karuth, Bridgestone, United States
Copyright © 2024 Pei, Liu, Cao, Zhou and Liu. This is an open-access article distributed under the terms of the Creative Commons Attribution License (CC BY). The use, distribution or reproduction in other forums is permitted, provided the original author(s) and the copyright owner(s) are credited and that the original publication in this journal is cited, in accordance with accepted academic practice. No use, distribution or reproduction is permitted which does not comply with these terms.
*Correspondence: Leixin Liu, bGl1bGVpeGluNTIzM0AxNjMuY29t; Jian Zhou, c3BpbmVAYWxpeXVuLmNvbQ==; Cuiyun Liu, bGl1Y3VpeXVuMjAwOC0xNzJAMTYzLmNvbQ==