- 1CSCEC Xinjiang Construction and Engineering (Group) Co., Ltd., Chengdu, China
- 2Key Laboratory of New Technology for Construction of Cities in Mountain Area, Chongqing University, Ministry of Education, Chongqing, China
- 3School of Civil Engineering, Chongqing University, Chongqing, China
This paper presents experimental studies on mechanical properties and micro-structures of ultra-high performance concrete (UHPC) made of recycled molybdenum tailings. The particle size distribution and chemical compositions of recycled molybdenum tailings were analysed at first. Then, compressive and flexural tests were conducted on UHPC in which different volume fractions of recycled molybdenum tailings were used to replace silica sand. The effects of recycled molybdenum tailings replacement ratio on the compressive and flexural strengths were determined through comparisons. Besides, X-ray diffraction tests and backscattered electron tests were carried out to analyse the effect of molybdenum tailings on the micro-structure of UHPC. Based on test data, an optimal replacement ratio of molybdenum tailings was determined which could significantly reduce the cost of UHPC but maintain the mechanical properties in compression. A molybdenum tailings replacement ratio of 30% was recommended to maximise the economic effect and to reduce the carbon emission.
1 Introduction
Ultra-high performance concrete (UHPC) was first proposed and developed by Larrard and Sedran (De Larrard and Sedran, 1994) in 1994. It has very high compressive strength, excellent durability, and almost zero maintenance costs. Using UHPC in structural members can significantly reduce the size, thereby reducing the emission of carbon dioxide in the cement production process (Wu et al., 2022). Compared with traditional concrete structures, the life of UHPC structures can be increased exponentially (Li et al., 2020). In recent years, UHPC has been increasingly utilised in construction practice due to its ecological and social benefits. However, the cost of UHPC is still higher than that of conventional concrete, which hinders its wide application.
A wide range of experimental tests has been conducted on UHPC, including the mix design, mixing process, and mechanical properties, to condense the micro-structure of UHPC. Yan et al. (Yan, 2010) proposed to eliminate coarse aggregates with particle sizes larger than 1 mm in UHPC. Therefore, the adhesion between the cement paste and aggregates was strengthened, and the degree of calcium hydroxide enrichment in the interfacial transition zone was reduced. Chen et al. (Chen et al., 2014) optimised the mixing procedures and suggested using steam curing to expedite hydration of the cement paste in UHPC.
The water-binder ratio is the most important factor affecting the workability and mechanical properties of UHPC. Hu et al. (Hu et al., 2018) conducted an experimental study on the performance of UHPC with the water-cement ratio ranging from 0.12 to 0.20. Test results showed that with the reduction of the water-cement ratio, the slump of the fresh UHPC decreased gradually, but the compressive strength increased. A water-cement ratio of 0.18 was also recommended based on test data. Wang et al. (Wang et al., 2012) studied the influence of cementitious materials and mineral admixtures on the workability and compressive strength of UHPC. When the ratio of cement, silica fume and slag was 1:0.2:0.4, the 90-day compressive strength of UHPC could reach 175.8 MPa after standard curing. In order to improve the properties of UHPC, different types of fibres are usually mixed into UHPC. Fibres commonly used in cementitious materials include steel fibres with the highest modulus of elasticity, glass fibres and polymer fibres (Zhang and RESEARCH PROGRESS ON HYBRID FIBER ULTRA HIGH PERFORMANCE CONCRETE, 2022). Kim et al. (Kim et al., 2011) investigated the effect of fibre length on the mechanical properties of UHPC and found that the compressive strength of UHPC was the highest when the volume fraction of fibres was 2.5%. Wang (Wang, 2019) found that with the increase of steel fibre column fraction to 2.0%, the workability of UHPC was decreased but the compressive strength was increased. The mixing process and curing method also have an impact on the performance of UHPC, especially the workability (Chen et al., 2003; Wu, 2012). Su et al. (Su et al., 2021) used high-strength sand instead of ordinary sand to mix UHPC, and by reducing the water-binder ratio, the compressive strength of UHPC reached 170 MPa after standard curing for 28 days.
Experimental tests have also been conducted on the mechanical properties of UHPC in compression and tension. It was found that the ascending branch of the stress-strain curve of UHPC is almost linear in uniaxial compression. Researchers (Guo et al., 2017; Run, 2005; Shen, 2014; Dan, 2002; Al-Hassani et al., 2015) have proposed different equations for the stress-strain curve based on test data. Yao et al. (Yao et al., 2006) found that the addition of steel fibre could significantly improve the fracture toughness of UHPC, and the fracture energy could be increased by 11 times. Existing results showed that the mechanical properties of UHPC are superior to those of conventional concrete and UHPC has a strong potential to be used in building structures. Nonetheless, it is still urgent to reduce the cost of UHPC so that its structural application can be prompted.
Statistical results show that 20% of molybdenum tailings are generated during the collection and processing of many non-metallic and metallic ores, with an ore volume of up to 5.97 billion each year. The use of molybdenum tailings can effectively avoid long-term storage which results in land and exchange pollution (Siddique and Jiang, 2020). The main components of UHPC are cementitious materials, sand, and fibres. The use of quartz sand and emery as fine aggregates is more expensive. Molybdenum tailings contain all kinds of rare metals after many times of treatments, of which the particle size is small, the crystal structure of the grinding is damaged, and the surface defects increase, with certain active characteristics. After grinding, molybdenum tailings can be used as a substitute for sand and gravel aggregates to prepare UHPC (Zhou et al., 2023; Yu et al., 2022). Zhou et al. (Zhou et al., 2023) replaced quartz sand with molybdenum tailings sand of UHPC and studied the effect of molybdenum tailings on the mechanical properties, workability and dry shrinkage of UHPC. Under standard maintenance conditions, with the increase volume fraction of molybdenum tailing sand, the compressive strength of UHPC at all ages decreased. With the increase of molybdenum tailings content, the resistance to chloride ion penetration and drying shrinkage performance of UHPC were improved significantly. When the molybdenum tailings content was 40%, the resistance to chloride ion penetration and drying shrinkage performance of UHPC were the best. However, existing studies mainly focused on the mechanical properties of UHPC containing molybdenum tailing, the effect of molybdenum tailing on the micro-structure of UHPC and the mechanism need further investigations.
This paper compares the physical properties and chemical compositions of silica sand and molybdenum tailings, and then uses molybdenum tailings to design UHPC partly in place of silica sand. The macroscopic mechanical properties of UHPC were studied by compressive and flexural tests, and the effects molybdenum tailings on the mechanical properties of UHPC were investigated through comparisons. Besides, the microstructure and composition were studied by X-ray diffraction (XRD) tests and backscattered electron (BSE) tests. According to the test results, the mechanism of molybdenum tailings in UHPC was identified, and the optimal replacement ratio of molybdenum tailings was determined.
2 Experimental design
2.1 Mix design of UHPC
The mix proportion of UHPC included masterbatch, steel fibre, silica sand, molybdenum tailings, and water. Among the major constituents, the masterbatch was premixed UHPC supported by a local company, and the compressive strength of the masterbatch was expected to be 120 MPa. Steel fibres were copper coated, with a length of 13 mm. In the mixing of UHPC, a water-cement ratio of 0.21 was adopted, and the volume fraction of steel fibres was determined to be 1.66%. Molybdenum tailings were used to replace silica sand in the experimental programme. The replacement ratio was varied to quantify the effect of molybdenum tailings replacement ratio on the mechanical properties and micro-structure of UHPC. Previous experimental results showed that the compressive strength of concrete increased first with increasing dosage of molybdenum tailings, and then decreased if the molybdenum tailings exceeded a certain content. An optimal replacement ratio of 30% existed which could make full use of the contribution of molybdenum tailings. Therefore, in the present study, the molybdenum tailings replacement ratio was set to be 0%, 20%, 30%, 40%, and the specific weight of each constituent at a certain replacement ratio can be found in Table 1. It should be pointed out that in the sample designation, UC represents UHPC material, and the numeral behind it denotes the ratio of molybdenum tailings in place of silica sand.
2.2 Testing programme
In this paper, two types of testing methods were conducted to analyse the effect of molybdenum tailings on the mechanical properties of UHPC. Firstly, the compressive and flexural strength were studied using cubes and prisms. The dimensions of UHPC cubes were 100 mm, determined according to GB/T50081-2019 (Ministry of Construction, 2019). UHPC prisms with dimensions of 100 mm × 100 mm × 300 mm were prepared and tested under axial compression to obtain the compressive strength and modulus of elasticity of UHPC. To measure the 28-day flexural strength of UHPC, prisms with dimensions of 100 mm × 100 mm × 400 mm were tested under four-point bending. The distance between two vertical supports was 300 mm, and two concentrated point loads were applied at the third point of the clear span. The loading rate was selected to be within the range of 0.08 MPa/s ∼ 0.10 MPa/s. Then, sieve analyses, XRD and BSE tests were performed on UHPC with and without molybdenum tailings.
The flow chart for the preparation of UHPC samples is shown in Figure 1. UHPC was mixed using a gravity mixer. Before mixing, the concrete masterbatch, silica sand, steel fibres, molybdenum tailings, and water were first weighed according to the mix design, and then put into the mixer. Then, water was added slowly to the mixer until good flowability was achieved for the concrete mixture. Discrete steel fibres were added to the concreter mixture within 20 minutes to ensure uniform dispersion of fibres in the concrete mixture. The fresh UHPC was poured into moulds and vibrated with a vibrator. The sample was covered using a plastic membrane for 24 h, and was then cured in a standard curing room with a temperature of 20°C ± 2°C and a humidity of more than 95% for expected ages.
3 Characteristics of molybdenum tailings
3.1 Particle size distribution
Before material tests, the particle size distribution of silica sand and molybdenum tailings was analysed using a particle size and shape image analyser. The analyser adopted a dynamic imaging method to test the particles in rapid motion and to analyse their size and shape, with a test range of 5–4,000 μm. Before the test, samples were taken from the two types of aggregates, with a volume of 3–4 mL. Figure 2 shows the interval and cumulative distributions of the particle sizes of silica sand and molybdenum tailings. It can be observed that the particle size distributions of silica sand and molybdenum tailings were significantly different from each other. The particle size of silica sand was generally greater than 800 μm, whereas that of molybdenum tailings was less than 800 μm. Moreover, the particle of silica sand was mainly distributed in the range of 850–950 μm and 1,100–1,200 μm, as shown in Figure 2A, which accounted for 56.8% of the total volume, and the maximum particle size was 1,400 μm. By contrast, the particle size of molybdenum tailings ranged from 100 to 300 μm which accounted for 43.5% of the total volume, with the maximum particle size of 900 μm. Hence, the particle size distribution of molybdenum tailings was more uniform than that of silica sand.
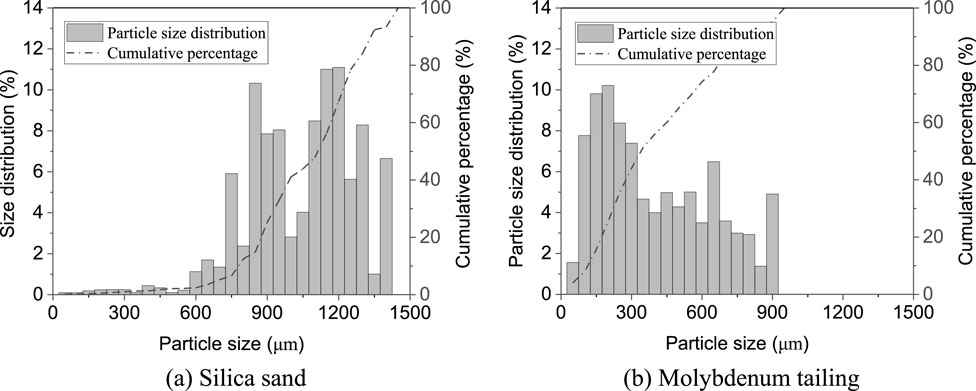
Figure 2. (A) Silica sand. (B) Molybdenum tailing. Particle size distribution of silica sand and molybdenum tailings.
Figure 3 shows the particle shape of silica sand and molybdenum tailings. In the silica sand, the particle was sharp and angular, whereas round and smooth particles existed in molybdenum tailings. Hence, molybdenum tailings with more round particles and more continuous size distribution could be used partly in place of silica sand to improve the gradation of silica sand and to improve the workability of the UHPC mixture.

Figure 3. (A) Silica sand. (B) Molybdenum tailings. Particle size distribution of silica sand and molybdenum tailings.
3.2 Mineral and chemical compositions
Molybdenum tailings are the by-product of molybdenum mining after the selection of molybdenum metal, and silica sand is mainly manufactured by using various types of magmatic, sedimentary and metamorphic rocks. Differences may exist in the mineral compositions of molybdenum tailings and silica sand. In this section, the mineral composition of molybdenum tailings and silica sand is analysed and compared with each other using X-ray diffractometer.
Before testing, the samples of molybdenum tailings and silica sand were milled and sieved. Figure 4 shows the mineral composition of molybdenum tailings and silica sand. It can be observed from Figure 4A that the main diffraction peaks of silica sand were quartz, calcite and mica, and those of molybdenum tailings were quartz, potassium feldspar and mica. Thus, the mineral compositions of the two materials were similar, which indicates that molybdenum tailings can be used in the preparation of UHPC.
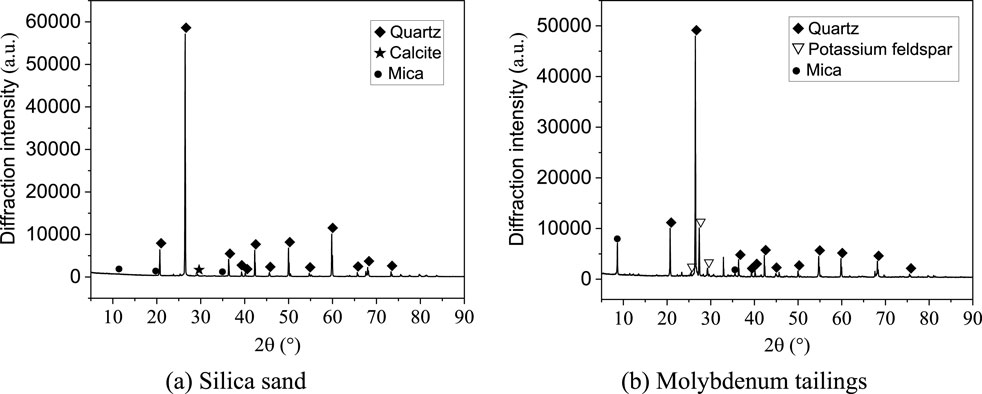
Figure 4. (A) Silica sand. (B) Molybdenum tailings. Mineral composition of silica sand and molybdenum tailings.
Besides the mineral composition, the chemical composition of molybdenum tailings and silica sand was also determined using an X-ray fluorescence spectrometer, as shown in Table 2. It can be seen from the table that the chemical composition of molybdenum tailings and silica sand was in general similar, but the quartz content in silica sand was greater than that in molybdenum tailings. As the hardness of silica sand and molybdenum tailings is mainly contributed by the quartz in the composition, it could be expected that the hardness of molybdenum tailings was less than that of silica sand. In addition, the contents of metal compounds Fe, Mg, K, Ti and Mn in molybdenum tailings were much larger than those in silica sand, but the content of metal compounds Al and Na in molybdenum tailings was slightly larger than those in silica sand.
4 Experimental results and discussions
4.1 Compressive strength of cubes
Three groups of samples were mixed at the same time to measure the compressive strength of UHPC at ages of 3-day, 7-day and 28-day, and three samples were included in each group. Compressive tests were completed using a loading rate of 1.2 MPa/s∼1.4 MPa/s. During testing, pull-out of steel fibres from UHPC matrix was observed, and spalling of UHPC occurred eventually on the surface, but the other part of the sample remained intact due to the bridging effect of steel fibres. By incorporating molybdenum tailings in UHPC, the failure mode of samples remained the same as that in Figure 5.
Table 3 summarises the compressive strength of UHPC at different ages. In the sample designation, UC denotes UHPC cubes, and the numeral behind the hyphen represents the replacement ratio of molybdenum tailings. It can be observed from the table that the compressive strength of UC cubes without molybdenum tailings increased with the increase in age. The average 3-day compressive strength of UC was 97.7 MPa. When UC was cured to an age of 7 days, the compressive strength became 104.9 MPa, only 7.4% greater than that at an age of 3 days. The 28-day compressive strength of UC could reach 111.7 MPa, about 14.3% higher than that at an age of 3 days and 6.5% greater than that at an age of 7 days, indicating that the compressive strength of UC could be developed at an early age.
When the silica sand in UHPC was replaced with molybdenum tailings at different replacement ratios, the compressive strength of UHPC with molybdenum tailings varied, as listed in Table 2. When the replacement ratio of molybdenum tailings was less than or equal to 30%, the compressive strengths at an age of 3 days, 7 days and 28 days were not significantly affected. Nonetheless, when the replacement ratio was increased to 40%, the compressive strength of UC-40 was slightly decreased to 95.5 MPa, 100.0 MPa and 108.4 MPa at an age of 3 days, 7 days and 28 days, respectively. Therefore, a replacement ratio of no more than 30% is recommended when molybdenum tailings are used for mixing UHPC.
4.2 Compressive strength of prisms
Four groups of samples with different molybdenum tailings replacement ratios were tested at the age of 28 days, and three samples were cast in each group. The loading rate was kept at 1.2 MPa/s∼1.4 MPa/s. Besides the applied load measured by a load cell, strain gauges were also mounted on the surface of prisms to quantify the modulus of elasticity.
UHPC prisms experienced similar failure modes to cubes. Cracking of UHPC was observed when the applied load approached the ultimate load, accompanied with pulling-out of steel fibres bridging across the crack, as shown in Figure 6. Thus, the transverse expansion of UHPC prisms was effectively constrained by the steel fibre. When the ultimate load of prisms was reached, the failure of UHPC generated a loud sound due to the sudden release of energy. Note that following the attainment of the ultimate load, the major portion of prisms remained intact, and spalling was not observed on the prism. The failure mode of UHPC prisms was not affected when the molybdenum tailings replacement ratio was varied for the prism.
The compressive strength of UHPC prisms at an age of 28 days were obtained in the test, as listed in Table 4. In the designation, UPM denotes UHPC prisms, and the numeral stands for the replacement ratio of molybdenum tailings. It can be observed that the compressive strength of prism UPM was 111.6 MPa, rather close to that of UHPC cube UC. It indicates that the effect of sample size on the compressive strength of UHPC was insignificant. With the increase in the molybdenum tailings replacement ratio to 30%, the compressive strength of prisms increased slightly to 112.8 MPa. Nevertheless, when the molybdenum tailings replacement ratio reached 40%, the compressive strength of prisms UPM-40 decreased to 108.3 MPa, 2.8 MPa lower than that of UPM without molybdenum tailings, as shown in Figure 7. Furthermore, the modulus of elasticity was not significantly affected when the replacement ratio of molybdenum tailings was 30%. Therefore, it confirms once again that a molybdenum tailings replacement ratio of 30% could provide UHPC with the highest compressive strength.
The voids in the mixture could be filled when the molybdenum tailing was used in place of silica sand and the particle gradation could be improved. As the replacement ratio of molybdenum tailing increased, the specific surface area increased as well, and more water was needed. Therefore, a molybdenum tailings replacement ratio of 30% was optimal considering the effects of voids and specific surface area.
4.3 Flexural strength of UHPC
UHPC samples developed cracks with limited widths in the tension zone during testing, and fractured suddenly when the ultimate load was reached. Steel fibres played an important role in transferring tensile stresses across cracks and arresting the rapid propagation of cracks at the post-cracking stage. Therefore, only one major crack could be observed at failure, whereas other parts of the sample remained intact, as shown in Figure 8.
With the measured load, the flexural strength of UHPC prisms can be calculated from Equation 1.
where
Table 5 summarises the 28-day flexural strength of UHPC prisms under four-point bending. In the designation, UB denotes UHPC samples in bending, and the numeral represents the replacement ratio of molybdenum tailings. It can be observed that the flexural strength of UHPC prisms fell within the range of 19.0 MPa and 19.6 MPa, and it was not considerably affected by the replacement ratio of molybdenum tailings. When the replacement ratio was 30%, the calculated flexural strength was 19.5 MPa, nearly the same as that of UHPC prism without molybdenum tailings, as can be seen in Figure 9.
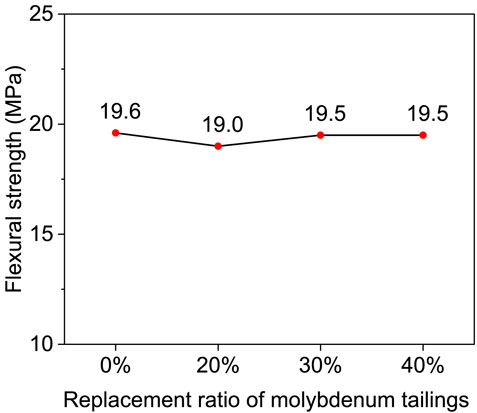
Figure 9. Effect of molybdenum tailings replacement ratio on flexural strength of UHPC prisms under four-point bending.
5 Effect of molybdenum tailings on UHPC
To gain a deep insight into the effect of molybdenum tailings on the mechanical properties of UHPC, sieve analyses, XRD and BSE tests were performed on UHPC. Detailed of test data will be discussed in the subsequent sections.
5.1 Sieve analyses of aggregates
The particle size distribution, namely, grading of aggregates, has an impact on the workability and mechanical properties of UHPC. The properties of UHPC can be greatly improved by a good grading. In this section, sieve analyses were performed on four groups of UHPC with different molybdenum tailings replacement ratios according to GB/T14684-2011 (Standardization Administration of China, 2011). A sample with a weight of 500 g was taken from UHPC and sieved for 10 min. The weight of aggregates retaining on each sieve was analysed, as shown in Figure 10. It can be observed that the particle size was generally larger than 0.6 mm for UHPC without molybdenum tailings. By contrast, the aggregate particle was better graded, with a continuous distribution within a range of 1.2 mm and 0.2 mm, when the replacement ratio of molybdenum tailings reached 30% in UHPC-30. Therefore, mixing molybdenum tailings into silica sand with a replacement ratio of 30% could effectively improve the grading of aggregates in UHPC.
5.2 X-ray diffraction (XRD) tests
Experimental tests on the mechanical properties of UHPC showed that when the replacement ratio of molybdenum tailings was 30%, the mechanical properties of UHPC were optimal at an age of 28 days. Therefore, in this section, UHPCs without molybdenum tailings and with a replacement ratio of 30% were tested using XRD. Figure 11 shows the comparison between XRD results. It can be observed from the figure that after 28 days of hydration, calcium hydroxide, calcite, and a small amount of dicalcium silicate appeared in the hydration product of UHPCs. The diffraction images of the hydration products of UHPCs without molybdenum tailings and with a replacement ratio of 30% were nearly the same. It indicates that no new hydration products were generated in the UHPC with a molybdenum tailings replacement ratio of 30%. The intensity of SiO2 (quartz) peak decreased when the angle increased from 50° to 60° which means more SiO2 (quartz) was involved in the reaction. Therefore, molybdenum tailings not only acted as aggregate fillers in UHPC, but also had a limited effect on the hydration of UHPC.
5.3 Backscattered electron (BSE) tests
The mechanical properties of UHPC are affected by its micro-structure. In order to investigate the effect of molybdenum tailings on the micro-structure of UHPC samples, this section presents a comparative analysis of the microstructural morphology of UHPC samples without and with a molybdenum tailings replacement ratio of 30% at the age of 28 days. Before testing, the length, width and height of UHPC samples were taken as less than 10 mm, and the surface was smoothened and then sprayed with gold for 60s to make it electrically conductive.
Figure 12 shows the microscopic morphology of the UHPC samples without molybdenum tailings at different scales. On a 400 times scale, the aggregate, cement paste, and the interfacial transition zone between them in the UHPC sample can be clearly seen from Figure 12A. The interfacial transition zone is generally regarded as the weakest part of the concrete. However, unlike conventional concrete, UHPC contains only fine aggregates with small particle size, and the interfacial transition zone was much thinner and denser, which greatly improved the mechanical properties of UHPC. When the scale was increased to 3,000, the dense micro-structure of UHPC can be observed more clearly, as shown in Figure 12B. A large number of flocculent, lamellar, reticulated, and spherical hydration products overlapped together irregularly. These hydration products were identified as calcium-silicate-hydrate gels, calcium hydroxide, dicalcium silicate through XRD tests. The dense micro-structure strengthened the mechanical properties of UHPC greatly and also improved the durability of UHPC.
Figure 13 shows the BSE images of UHPC-30 with a molybdenum tailings replacement ratio of 30% on a scale of 30. It can be found from the comparison between two images that the content of silica sand was significantly increased in the UHPC without molybdenum tailings. In addition, a large number of pores existed in the UHPC, and some of the large pores had a diameter ranging from 0.3 mm to 0.4 mm. By contrast, when molybdenum tailings were used in UHPC, the largest pore size was reduced to less than 0.1 mm and some pores even disappeared. The largest pore size reduction was from 66.7% to 75%. The reduction in pore size reduction showed the difference in the microstructure which would increase the density of cement paste around the steel fibre and enhanced the bond strength. Therefore, it can be confirmed that molybdenum tailings acted as aggregate fillers to improve the micro-structure of UHPC-30. By using molybdenum tailings, the pore size was reduced and the mechanical properties of UHPC-30 samples were enhanced.
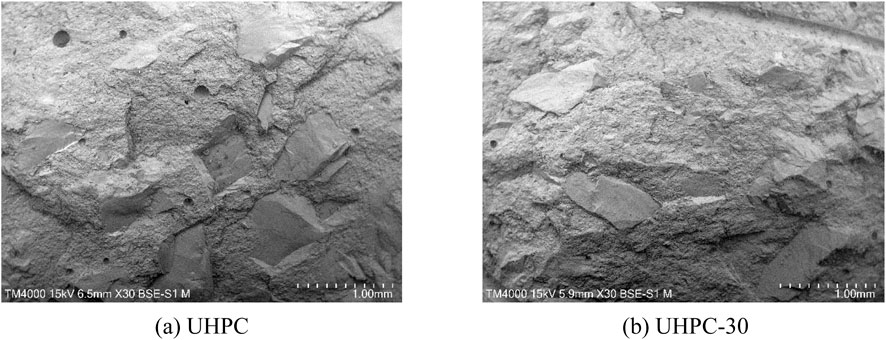
Figure 13. (A) UHPC. (B) UHPC-30. Comparison of BSE images of UHPCs without and with 30% molybdenum tailings at 30 scale.
6 Conclusion
In this paper, UHPC samples made of molybdenum tailings were tested under compression and bending, and the compressive and flexural strengths of the sample were obtained. The effect of replacement ratio of molybdenum tailings was investigated through comparisons between test data. Moreover, the micro-structure of UHPC with different replacement ratios of molybdenum tailings was also analysed through XRD and BSE tests. The following conclusions could be drawn from the experimental results.
(1) Comparisons between silica sand and molybdenum tailings in terms of mechanical properties showed that silica sand and molybdenum tailings had similar mineral and chemical compositions. The particle size of molybdenum tailings was smaller than that of silica sand, and thus it is feasible to use molybdenum tailings as aggregates to replace silica sand to reduce the cost of UHPC.
(2) The result of axial compression tests on UHPC showed that when the replacement ratio of molybdenum tailings was less than 30%, the mechanical properties of UHPC at different ages were not adversely affected. The compressive strength of UHPC at an age of 28 days could reach 112.8 MPa, and the associated flexural strength was 19.5 MPa. Based on experimental results, a molybdenum tailings replacement ratio of 30% was recommended to maximize the economic effect and to reduce the carbon emission.
(3) The effect of molybdenum tailings on the micro-structure of UHPC was studied through sieve analyses, XRD and BSE tests. Test results showed that the use of molybdenum tailings in UHPC could reduce the pore size and make the micro-structure denser, thereby improving the mechanical properties of UHPC. The major role of molybdenum tailings in UHPC was achieved through filling effect.
(4) Considering cost, strength and workability, the replacement ratio of 30% was recommended as an optimal ratio. Even though there are many advantages to use molybdenum tailings in place of quartz sand, the long-term performance of UHPC in molybdenum tailings still needs to be studied, especially when molybdenum tailings are used on a large scale, such as bridge deck joints and UHPC-filled steel tube columns. To further investigate the application of molybdenum tailings in UHPC, the mechanical properties, workability and durability under a variety of coupling environmental conditions, such as high temperature, freezing-thaw cycles, chloride erosion and fire, need to be investigated in the future.
Data availability statement
The raw data supporting the conclusions of this article will be made available by the authors, without undue reservation.
Author contributions
XL: Formal Analysis, Writing–original draft. C-XL: Investigation, Writing–original draft. X-YZ: Data curation, Methodology, Writing–review and editing. S-BK: Funding acquisition, Methodology, Writing–review and editing.
Funding
The author(s) declare that financial support was received for the research, authorship, and/or publication of this article. The authors gratefully acknowledge the financial support provided by the Luzhou Science and Technology Program, China (Grant No.: 2022-GYF-2 and 2023GYF079) and by the Scientific and Technological Research Program of Chongqing Education Commission (Grant No.: KJQN202403440, KJZD-K202204001 and KJQN202004010).
Conflict of interest
Authors XL, and C-XL were employed by CSCEC Xinjiang Construction and Engineering (Group) Co., Ltd.
The remaining authors declare that the research was conducted in the absence of any commercial or financial relationships that could be construed as a potential conflict of interest.
Publisher’s note
All claims expressed in this article are solely those of the authors and do not necessarily represent those of their affiliated organizations, or those of the publisher, the editors and the reviewers. Any product that may be evaluated in this article, or claim that may be made by its manufacturer, is not guaranteed or endorsed by the publisher.
References
Al-Hassani, H. M., Khalil, W. I., and Danha, L. S. (2015). Proposed model for uniaxial compression behavior of reactive powder concrete. J. Babylon Univ. Eng. Sci. 23 (3), 591–606.
Chen, C., Ji, T., Huang, Q., Wu, H., Ding, Q., and Zhan, Y. (2014). Review of research on ultra-high performance concrete. J. Archit. Civ. Eng. 31 (3), 1–24.
Chen, Y., Yan, G., and An, M. (2003). Research of influential factors on compressive strength of reactive powder concrete with normal mixing technology. Railw. Eng. (3), 44–48.
Dan, B. (2002). Experiment and research on basic mechanical properties of reactive powder concrete. Changsha: Hunan university.
De Larrard, F., and Sedran, T. (1994). Optimization of ultra-high-performance concrete by the use of a packing model. Cem. Concr. Res. 24 (6), 997–1009. doi:10.1016/0008-8846(94)90022-1
Guo, X., Kang, J., and Zhu, J. (2017). Constitutive relationship of ultrahigh performance concrete under uni-axial compression. J. Southeast Univ. Nat. Sci. Ed. 47 (2), 469–376.
Hu, A., Liang, X., Li, D., Yu, J., Shi, Q., and Li, L. (2018). Mix design method and uniaxial tensile characteristics of ultra-high performance concrete. J. Hunan Univ. Nat. Sci. Ed. 45 (3), 39–46.
Kim, D. J., Park, S. H., Ryu, G. S., and Koh, K. T. (2011). Comparative flexural behavior of hybrid ultra high performance fiber reinforced concrete with different macro fibers. Constr. Build. Mater. 25 (11), 4144–4155. doi:10.1016/j.conbuildmat.2011.04.051
Li, L., Ming, Y., Chen, P., Chen, X., and Gan, G. (2020). Research and application progress of ultra high performance concrete at home and abroad. Cem. Eng. 32 (2), 85–90.
Ministry of Construction (2019). Standard for test methods of concrete physical and mechanical properties, GB/T50081-2019. Beijing: China Architecture and Building Press.
Run, G. (2005). Study on failure criterion and constitutive relation of 200 MPa reactive powder concrete (RPC200). Beijing: Northern Jiaotong University.
Shen, T. (2014). Study on constitutive relation and structural design parameters of reactive powder concrete under uniaxial compression. Harbin: Harbin Institute of Technology.
Siddique, S., and Jiang, J. (2020). Assessment of molybdenum mine tailings as filler in cement mortar. J. Build. Eng. 101322.
Standardization Administration of China (2011). Sand for construction, GB/T14684-2011. Beijing: Standards Press of China.
Su, Y., Luo, B., Luo, Z., Huang, H., Li, J., and Wang, D. (2021). Effect of accelerators on the workability, strength, and microstructure of ultra-high-performance concrete. Materials 15 (1), 159. doi:10.3390/ma15010159
Wang, C., Yang, C., Liu, F., Wan, C., and Pu, X. (2012). Preparation of ultra-high performance concrete with common technology and materials. Cem. Concr. Compos. 34 (4), 538–544. doi:10.1016/j.cemconcomp.2011.11.005
Wang, W. (2019). Effects of the steel fibre on the ultra-high performance fibre reinforced concrete (UHPFRC). World Build. Mater. 40 (1), 13–16.
Wu, Z. (2012). “Autoclaved and non-autoclaved early strength and high strength concrete for pipe piles,” in Annual meeting of China concrete and cement products association, 29–30.
Wu, Z., Wang, L., Gao, H., Yi, B., and Li, G. (2022). Development status and engineering application of ultra-high performance concrete. Guangdong Archit. Civ. Eng. 29 (2), 90–92.
Yan, P. (2010). Development and present situation of ultra-high performance concrete(UHPC). China Concr. (9), 36–41.
Yao, Z., Zhou, J., and Zhou, R. (2006). Experimental study on fracture properties of reactive powder concrete(RPC). J. Build. Mater. 9 (6), 654–659.
Yu, R., Dong, E., Shui, Z., Qian, D., Fan, D., Wang, J., et al. (2022). Advanced utilization of molybdenum tailings in producing Ultra High-Performance Composites based on a green activation strategy. Constr. Build. Mater. 330 (127272), 127272. doi:10.1016/j.conbuildmat.2022.127272
Zhang, H.RESEARCH PROGRESS ON HYBRID FIBER ULTRA HIGH PERFORMANCE CONCRETE (2022). Low. Temp. Archit. Technol. 44 (4), 38–43.
Keywords: molybdenum tailing, ultra-high performance concrete, replacement ratio, mechanical properties, filler effect
Citation: Li X, Liu C-X, Zhao X-Y and Kang S-B (2024) Effect of recycled molybdenum tailings on mechanical properties of ultra-high-performance concrete. Front. Mater. 11:1483446. doi: 10.3389/fmats.2024.1483446
Received: 20 August 2024; Accepted: 01 November 2024;
Published: 13 November 2024.
Edited by:
Chaopeng Xie, North China University of Water Conservancy and Electric Power, ChinaReviewed by:
Amir Ali Shahmansouri, Washington State University, United StatesChuanqing Fu, Zhejiang University of Technology, China
Copyright © 2024 Li, Liu, Zhao and Kang. This is an open-access article distributed under the terms of the Creative Commons Attribution License (CC BY). The use, distribution or reproduction in other forums is permitted, provided the original author(s) and the copyright owner(s) are credited and that the original publication in this journal is cited, in accordance with accepted academic practice. No use, distribution or reproduction is permitted which does not comply with these terms.
*Correspondence: Shao-Bo Kang, a2FuZzAxMTlAY3F1LmVkdS5jbg==