- 1Institute of Urban Rail Transit, Liaoning Railway Vocational and Technical College, Jinzhou, China
- 2School of Civil Engineering, Liaoning University of Technology, Jinzhou, China
- 3Institute of Railway Engineering, Liaoning Railway Vocational and Technical College, Jinzhou, China
Constructing dams with geotextile bags filled with fine tailings can overcome the shortage of sand and gravel resources for dam construction. In addition, it can also prevent safety hazards caused by the deposition of fine tailings in tailings reservoirs. However, the geotextile bags were made of polypropylene woven fabrics, whose surface pore size can influence both the dehydration rate and the amount of tailings consolidated in geotextile bags. To optimize the construction efficiency of geotextile bag dams and improve the utilization rate of fine tailings, geotextile bags filling experiments, X-ray diffraction experiments and scanning electron microscopy experiments were performed by revealing the influence mechanism of low-cement content on the filling characteristics of geotextile bags from macro and micro perspective. The results showed that the addition of low-content cement as a curing agent effectively solved the contradiction between the permeability and the utilization rate of fine tailings. Compared with the fine tailings slurry without cement addition, when 1%, 3%, and 5% cement was added, the drainage efficiency increased by 12.90%, 23.39%, and 49.6% respectively; the sand retention ratio improved by 0.25%, 10.44%, and 13.62% respectively. Calcium silicate hydrate (C-S-H) calcium silicoaluminate hydrate (C-A-S-H), calcium carbonate, and calcium aluminate (CH) were found in the consolidated tailings. The hydration of cement within the geotextile bags formed a hydrated membrane layer that wrapped, adsorbed, and encapsulated fine tailings particles, accumulating on the surface of coarse tailings to create a composite particle structure. With the increase of cement content, the amount of hydration products mentioned above increased accordingly, and their diffraction peaks gradually enhanced, which not only contributed to effectively alleviate the surface blockage of geotextile bags but also improve the filling degree of geotextile bags, and thus increasing the effective normal stress transmitted to the contact surface of laminated geotextile bag in dam construction. Considering construction efficiency, the utilization rate of fine tailings, and construction cost, the optimal cement content was determined to be 3%. This research is expected to offer insightful and transferable experiences for dredging projects, coastline governance, soft foundation strengthening, among other applications.
1 Introduction
Tailings are solid waste materials that are generated concomitantly with the ore beneficiation processes of metal mining enterprises. Typically, they are discharged and stored in tailings reservoirs. However, they also give rise to significant safety hazards and environmental pollution risks. With advancements in mineral processing technology, the amount of tailings discharged into tailings reservoirs will prominently increase, and tailings particles will become finer and finer. Fine tailings have several disadvantages, including low mechanical strength, poor permeability, and an inability to be consolidated. Utilizing fine tailings to construct dams through traditional upstream methods will create many problems, such as difficult dam construction, high seepage lines, and poor dam stability (Zhang J. F. et al., 2023). As environmental protection consciousness increases, the exploitation of sand and gravel resources has become severely restricted. Additionally, the high transportation costs of sand and gravel will increase the construction cost of dams. Therefore, by sourcing materials locally at the tailings reservoir and using the fine tailings stored there to construct the tailings dam, not only can the transportation costs of dam-building materials be saved, but also the amount of tailings stored can be reduced, thus achieving the capacity expansion of tailings reservoirs.Damming technology of geotextile bags can solve many difficulties mentioned above. In this technique, geotextile bags are filled with fine tailings slurries, and consolidated tailings and geotextiles form high-strength composite soils (Li et al., 2023). This offers several advantages compared with traditional methods, including the recycling of fine tailings and reducing construction costs. Additionally, it will reduce the amount of accumulated tailings in tailings reservoirs, which is equivalent to improve the capacity of tailings reservoirs. Finally, dangers such as landslides, floods, seepage failure, and environmental pollution caused by the storage of fine tailings in tailings reservoirs can be prevented (Du et al., 2019). Therefore, this technology will bring significant social and economic benefits.
When permeable geotextile bags are filled by fine tailings slurries, the geosynthetics serve as a filtering medium (Sheng et al., 2024). An ideal situation is that the water is quickly dis-charged, and a maximum amount of tailings is retained inside (Bulut and Sengul, 2024). If the aperture of the geosynthetic is too large, the permeability will increase, and a certain number of fine particles will be discharged with water, decreasing the utilization ratio of fine tailings. In contrast, the utilization ratio of fine tailings will be improved, but the drainage speed will decrease, which will affect construction progress. Therefore, over-coming the conflict between permeability and utilization ratio of fine tailings is the first technical problem in the construction of geotextile bag dams (Liu et al., 2024). The fine tailings slurry filled into geotextile bags is similar to high-water-content sludge and plastic soft clay (Kim and Dinoy, 2021). To improve the construction efficiency and utilization ratio of fine tailings, the solidification technology from dredging, foundation, and subgrade engineering can be referenced (Zhang H. et al., 2023; Finklenburg et al., 2024; Tseng et al., 2024).
Cui (Han et al., 2019) performed an investigation on the characteristics of geotextile bags composed of geotextile and fine tailings, and proved that geotextile bags can obtain a higher load carrying capacity. Aydilek and Edil (2008) asserted that preventing geotextile surface clogging and reducing particle loss were the two most important issues in geotextile bags dehydration. Liu et al. (2024) studied the effects of different slurry concentrations and filling heights on the filling characteristics and consolidation process of the geotextile bags, and revealed that the balance between dewatering time and tailings consolidation presented relatively superior at a 40% concentration compared with 25% and 60% concentrations. Wu (Wu et al., 2021) conducted orthogonal experiments on hanging bags dehydrating, investigating the influence patterns of fabric pore size and clay content in filling slurry on both soil retention performance and dehydration rate. Richard Weggel et al. (2011) developed a dimensionless analysis model for the drainage of suspended geotextile bags. They proposed a data analysis procedure and then compared the measured drainage data of the formwork bags with the drainage data computed by the model. The results indicated a good match between the two sets of data. Kim and Dinoy (2021) introduced a two-dimensional consolidation solution aimed at depicting the consolidation characteristics of geotextile tubes filled with fine slurry. However, the above research findings were focused on the permeability of geotextile bags, without addressing the sand retention property. Moreover, no quantitative indicators for comprehensively evaluating both the permeability and sand retention of geotextile bags were proposed.
Sun et al. (2021) conducted experiments on the mechanical properties and microstructure of solidified sludge, proposed the difference between low-content cement solidified soil and conventional cement solidified soil, and selected silt solidifying agent based on this. Kong et al. (2024) completed the solidification treatment of dredged sediment using active MgO carbonation technology, revealing through micro-experiments the influence mechanisms of MgO dosage, moisture content, compaction degree, and consolidation time on the hydration and carbonation products. Xiao et al. (2024) incorporated 10% cement as a curing agent into granulated blast furnace slag, fly ash, and other industrial by-products to enhance the properties of loess subgrade, while analyzing the hydration mechanisms that contribute to the strength improvement of the stabilized loess. Guo et al. (2008), Guo (2007) conducted a series of studies on the curing mechanisms of stabilized soil by examining the types and quantities of curing agents, characteristics of hydration reactions, and the types, quantities, and morphologies of hydration products, using image analysis, phase analysis, and micro-structural analysis. Yang and Hu (2018) conducted SEM and XRD tests on urban sludge solidified soil at different consolidation times. The study found that the hydration reaction of the solidifying agent gradually deepened with increasing consolidation time, leading to more pronounced agglomeration of soil particles and a more evident soil framework, resulting in significantly improved strength of the solidified soil. Horpibulsuk et al. (2003) enhanced the solidification of soft clay with high moisture content through the application of low-cement-content mixtures, positing that the water-cement ratio serves as a precise indicator of the interactions between cement and clay particles. Consequently, the water-cement ratio is regarded as a critical parameter for regulating the strength of cement-stabilized soil. The above research findings, to a certain extent, demonstrate that adding an appropriate amount of cement to fine tailings slurry can promote the aggregation of fine tailings particles. This, in turn, enhances the filling compactness and improves the filling characteristics.
The existing findings offer invaluable insights for addressing the conflict between permeability and fine tailings utilization ratio in dam construction utilizing geotextile bags filled with fine tailings. Nonetheless, it is noteworthy that the particle sizes of the soils examined in these studies are typically larger than those of fine tailings. Additionally, the primary objective of incorporating cement into fine tailings slurries is to enhance their filling characteristics, which allows for a significant reduction in the cement content mixed into these slurries. Furthermore, micro-scale investigations into the early hydration of consolidated soil with low cement content remain scarce. Crucially, there is still a lack of proven indicators for quantitatively assessing the permeability and sand retention capabilities of geotextile bags.
In view of this, filling tests were conducted through a self-made system to macro-scopically explore the effects of cement content on the filling characteristics, and drainage efficiency and sand retention ratio were regarded the quantitative indexes to evaluate the filling characteristics. Microscopic morphology observations and phase identification were performed on the consolidated tailings to reveal the mechanisms by which cement content influences the permeability and utilization ratio of fine tailings. This study has theoretical and practical significance for improving the filling characteristics of fine tailings in geotextile bags and provides beneficial references for the resource utilization of fine tailings.
2 Materials and methods
2.1 Filling test of the geotextile bags
Geotextile bags, as shown in Figure 1, with sizes of 300 × 300 mm were sewn with polypropylene woven fabrics and nylon thread whose strength exceeded 180 N. A filling port with a diameter of 45 mm and a height of 100 mm was set at the top center of geotextile bags. The filling port was tightly stitched with the geotextile bag to ensure no slurry leakage at the joints. To satisfy the particle size requirements of fine tailings, the tailings chosen for this study were the dry - discharged gold tailings which were from Paishanlou Gold Mine in China. Their passing rate exceeded 80% when sieving by the 0.075 mm screen, as shown in Figure 2.
The main chemical composition of these gold tailings were described in Table 1. They were uniformly mixed with tap water to configure the filling slurry with a mass concentration of 40%, and denoted as sample S0 (Liu et al., 2024). Ordinary Portland cement (P.O42.5) was selected as curing agent. Cai et al. (2016) conducted a study on the solidification of coastal aeolian sand using cement contents of 0%, 4%, and 6%. The findings revealed that at a 4% cement content, hydration products began to adhere to the surface of the sand particles, resulting in a gradual roughening of the particle surfaces as the hydration reaction progressed. When the cement content was increased to 6%, a greater amount of hydration products enveloped the sand particles, filling the interparticle pores and enhancing the cementation between particles, which led to an agglomerated appearance of the particles. Liu (2007) investigated the factors influencing the strength of low-cement-content solidified soil in geotextile bags cofferdam projects. The experimental results demonstrated that when the cement content exceeded 6%, the strength of the solidified soil was significantly enhanced compared to the original silt soil. In the present study, the addition of a small amount of cement to fine tailings slurry aims to utilize the hydrate film layer to adhere to the fine tailings particles. This approach improves the internal pore structure of the solidified tailings and mitigates surface blockage of the geotextile, thereby enhancing water permeability and sand retention. The primary objective is not to substantially increase the strength of the solidified tailings. Consequently, the maximum cement dosage in this study has been set at 5%. Cement accounting for 1%, 3% and 5% of the mass of gold tailings was mixed into sample S0, and the uniformly mixed slurries were recorded as sample S1, S3 and S5, respectively.
As shown in Figure 3, the filling slurry was thoroughly mixed in the slurry tank and subsequently pumped through the slurry pipe into the geotextile bag, which was put on a filling platform, within 10 min. The slurry that permeated through the geotextile bag was collected in a collection vessel, which was used to measure the volume of the discharged slurry. To reduce the error of test results, each sample was filled into four geotextile bags, and the average value was used. The volume of discharge was measured every 20 min, and if the discharged volume was less than 500 mL in 20 min, dehydrating was regarded as complete, and the next filling was started. The filling and consolidating processes are illustrated in Figure 4.
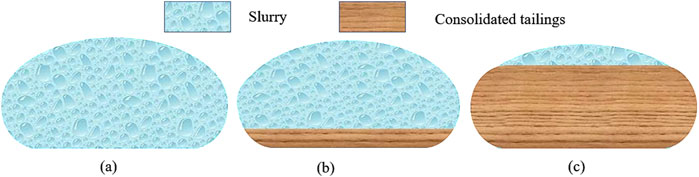
Figure 4. The filling and consolidating processes (a) The instant of the first filling (b) The deposition of tailings particles (c) The filling after several times.
Different filling ratios may lead to different mechanical properties of the composite soils formed by consolidated tailings and geosynthetics (Liu et al., 2024). If the filling ratio is too large, it may be destroyed prematurely when pressure is applied to the composite soil. However, if it is too low, the composite soil may be flattened under pressure. Then, if geotextiles do not suffer from tensile deformation or that is too low, and the lateral restriction from geosynthetics acting on fine tailings is lost, no reinforcement will be achieved. Generally, the best reinforcement effect was achieved when the volume of consolidation tailings in geotextile bags reached 85%–90% of the geotextile bag capacity (Fu et al., 2018). It was inevitable that some water was discharged even after completion of filling tests, which decreased the height of consolidated tailings inside the bags. Therefore, the optimal filling ratio in this paper was determined to be 90%. In the preparation stage, many tests were performed to explore the standard for test termination and showed that filling was complete when the discharged volume was less than 500 mL within 20 min and the height of consolidated tailings in the geotextile bag first reached 7 cm. During the tests, filing and discharged volumes were recorded for each filling. When the test was performed, the mass of composite soils was immediately measured, denoted as Mi-GB, and the time spent on the test was calculated, denoted as Ti, where i was the cement content.
2.2 Micro-experiment on consolidated tailings
Fine tailings slurries with different cement content (S0, S1, S3, and S5) were filled into geotextile bags and respectively consolidated for 20 min, 1 h, and 2 h to obtain early-stage consolidated tailings. After filling tests, the geotextile bags filled with different cement content were consolidated for 1 day, 3 days, 7 days and 28 days respectively to obtain the consolidated tailings in the middle and late hydration. The microstructural evolution of solidified tailings under different cement contents and curing periods was investigated through scanning electron microscopy (Hitachi S-3400N, Japan) and X-ray diffraction analysis (Bruker D8 ADVANCE, Germany), focusing on morphological features and phase composition. In the morphological observation, the solidified tailings samples were magnified within the range of 2,000 to 10,000 times.
3 Results of filling tests
3.1 Basic assumptions
To prepare sample S0, 2 kg of air-dried fine tailings was mixed with 3 kg of tap water in a 5 L measuring cup under uniform stirring. The volume of the tailing slurry was determined to be 3.8 L when the liquid level was stable. The densities of S0 and air-dried tailings were calculated and denoted respectively as ρ0 = 1.32 g/cm3 and ρT = 2.5 g/cm3. S1, S3, and S5 were obtained by adding 1%, 3%, and 5% cement contents into S0 with uniform stirring.
During calculation, the following assumptions were made: (1) Cement added into S0 did not increase the volume; (2) The densities of air-dried tailings was constant in discharged slurries, that was ρT = 2.5 g/cm3; (3) No evaporation of water occurred, and no cement was discharged; (4) Water consumption due to cement hydration was ignored.
3.2 Calculation process
The masses of filling slurry, filling tailings, filling water, discharged slurry and discharged tailings were denoted as Mi-FS, Mi-FT, Mi-FW, Mi-DS, and Mi-DT, respectively. Similarly, the volumes of filling slurry, discharged slurry, discharged tailings, and dis-charged water were donated as Vi-FS, Vi-DS, Vi-DT, and Vi-DW, respectively, where i was the cement content. The density of the discharged slurry was expressed as ρi-DS. During the test, these equations were as follows:
where, Vi-FS, Vi-DS, Mi-GB, and m were obtained through measurement. Equations 1–6 were used to calculate Vi-DT and Vi-DW. Then, Mi-DT was calculated by Equation 7:
The geotextile bag drainage ratio ASi, drainage efficiency BSi, sand retention ratio CSi, and theoretical water content of consolidated tailings were all be calculated using Equations 8–11:
When the filling tests were completed, the water contents of consolidated tailings in three different sedimentary depths were immediately measured, and the average was taken as the measured water content. The relationship between water content and cement content is depicted in Figure 5. The water content was negatively correlated with the cement content, and the theoretical water content was greater than the measured water content. This could have arisen due to the evaporation of water during the test. Additionally, part of the discharged water was adsorbed on the surface of geotextiles and the inner wall of the water collection vessel, which was not measured. Finally, a small amount of water was discharged from geotextile bags between weighing the composite soils to the completion of consolidated tailings sampling. Although the two water contents were different, they showed nearly the same trend. Therefore, the theoretical water content was considered to be basically identical to the measured water content, which further verified the reliability of the filling tests.
3.3 Permeability analysis
The relationships between filling volume, filling time, and cement content are plotted in Figure 6. Both variables approximately linearly decreased as the cement content increased, but cement content had a relatively weak influence when the cement content was 1%. As the cement content increased, the effect turned was more obvious. Compared with S0, the filling times of S1, S3, and S5 were respectively reduced by 7.15%, 14.29%, and 28.57%, and the S1, S3, and S5 filling volumes respectively decreased by 1.5%, 10.0%, and 15.49%.
The relationships between each filling volume, each discharged volume, and time are plotted in Figures 7–10 for different cement contents. Both variables exponentially de-creased with time. The first discharge volume was larger than the latter. The first dis-charged volumes of S0, S1, S3, and S5 accounted for 29.68%, 32.13%, 36.34%, and 41.67% of total discharged volumes, respectively. The drained fluid of geotextile bags was concentrated during the first 2 h, and the sum of the discharged volumes of S0, S1, S3, and S5 respectively reached 59.32%, 69.15%, 72.36%, and 78.10% of the total dis-charged volume within 2 h. The difference between each filling volume and each discharged volume became increasingly smaller with time. During the latter filling tests (about 2 h later), the volume of each discharged gradually stabilized. The initial high slurry discharge volume is attributed to the dispersion of cement and tailings particles in the aqueous medium, coupled with the absence of fine particle blockage on the geotextile bag surface. Although the effective drainage area at the bottle diminishes as tailings consolidation, the substantial hydrostatic pressure within the bag facilitates continuous water discharge through the top and side pores. During the later filling stage, the discharge mechanism shifts. As the consolidated tailings height increases, hydrostatic pressure decreases, and water in the tailings pores is expelled by the self-weight of the consolidated tailings. Consequently, drainage transitions to a self-weight-driven process, gradually stabilizing discharge volumes. These observations suggest practical construction strategies: early-stage filling should focus on preventing surface blockage through measures like surface tapping to dislodge adhering particles, while later stages benefit from increased vertical loading, such as taking measures like stepping on the bag or preloading with heavy objects, to enhance drainage efficiency.
As shown in Figure 11, both the drainage ratio and drainage efficiency display a near-linear correlation with cement content, increasing proportionally as the cement content rises. The drainage ratio of S0 was 69.51%, and increasing the cement content to 1%, 3%, and 5% improved the drainage ratio by 4.56%, 5.50%, and 6.73%, respectively. This shows that adding cement allows more water to seep through geotextile bags, but the influence was not significant. Considering the effect of cement content on filling time, the drainage efficiency was obviously increased. Compared with S0, the drainage efficiency improved by 12.90%, 23.39%, and 49.6% at cement contents of 1%, 3%, and 5%. Therefore, compared with drainage ratio, the drainage efficiency more comprehensively reflected the permeability of geotextile bags, and was selected as the index to evaluate the permeability of geotextile bags.
3.4 Analysis of tailings utilization rate
Figure 12 shows the effect of different cement contents on the density of discharged slurry. The relationship between discharged slurry density, sand retention, and cement content are plotted in Supplementary Figure S13.
Figure 12 shows that, as the cement content increased, the discharged slurry gradually became clear, which was consistent with changes in the discharged slurry density shown in Supplementary Figure S13. When the cement content was 1%, the discharged slurry was still turbid, and the effect of the cement content on sand retention was not obvious, and possibly even negligible. With the cement content increased, sand retention remarkably improved. The sand retention ratio of S0 was 77.97%, and that of S1, S3, and S5 were improved by 0.25%, 10.44%, and 13.62%, respectively, suggesting that the sand retention ratio more directly reflected the utilization ratio of fine tailings. As evidenced in Supplementary Figure S13, the sand retention ratio demonstrated a significant improvement from 78.17% to 86.11% as the cement content increased from 1% to 3%. However, a further increase in cement content to 5% resulted in only a marginal enhancement of the sand retention ratio to 88.59%, which aligned with the observations presented in Figures 12c,d. This trend indicated that while increasing cement content up to 3% substantially improved sand retention, additional cement incorporation beyond this threshold yields diminishing returns in terms of the utilization ratio of fine tailings, while simultaneously escalating construction costs. Therefore, considering construction progress, construction cost, and the utilization ratio of fine tailings, the optimal cement content was determined to be 3%.
3.5 Hydration reaction and hydration products in filling tests
The improvement in the filling characteristics was attributed to cement hydration, but setting and hardening of cement occurs due to complex and continuous physical and chemical changes. It is often supposed that there are four stages of this process: an initial reaction period (5–10 min), an incubation period (within 1 h), a setting period (within 6 h), and a hardening period (6 h later). Considering the filling time in Table 2, the effect of the hydration reaction and hydration products on the filling characteristics will be discussed, mainly taking into account the first three stages of the hydration reaction.
The hydration products during the initial reaction period were mainly needle-shaped ettringite crystals and flaky calcium hydroxide crystals. However, a very small amount of fine fibrous C-S-H (C-S-H) was obverted (Sun et al., 2016). The hydration products may have first covered the surface of the finest tailings particles to form hydrated films which were thin and less-cohesive. This was equivalent to enlarging the diameter of fine tailings and to also retaining a part of the finest tailings particles.
When entering the incubation period, the number of hydration products gradually increased, especially C-S-H, and the hydrated films gradually thickened. Then, the distance between all particles, including tailing particles and those wrapped by hydrated films, gradually became smaller as particles were deposited. When the distance between particles was small enough, reversible agglomerated structures formed due to van der Waals forces. Furthermore, during particles settling, relative movement occurred between particles and water, and the thicker hydrated films would loosely ac-cumulate on the surface of relatively larger tailings particles in certain spots. This would cause a transition zone to appear on the surface of relatively larger tailings particles (Zhong et al., 2002). The consolidated tailings particles surrounded by the thicker hydrate films may adsorb additional finer particles, and finally form a composite particle structure (shown in Supplementary Figure S14) with a larger particle size than previous particles and contained many fine tailings particles. Different improvements in the filling characteristics were directly attributed to the appearance of composite particles. To further validate the improved filling characteristics, microscopic tests were conducted on the consolidated tailings listed in Table 3.
4 Discussion
4.1 Discussion on X-ray diffraction of consolidated tailings
Compounds such as Na2O, K2O, Al2O3 and CaO were contained in the gold tailings. The Na+ and K+ in the slurry bound with OH−, resulting in an alkaline environment in the consolidated tailings, as shown in Supplementary Figure S15. The cement particles within the geotextile bags were hydrated under the alkaline environment, which also catalyzed the hydration reactions (Li et al., 2019; Huang and Yan, 2019; Wang, 2019; Zheng, 2011), leading to the formation of calcium aluminosilicate hydrate (Gao, 2015).
The experimental results indicated that the hydration products in the consolidated tailings with a cement content of 1% and consolidation times of 20 min and 1 h were minimal and poorly crystallized. Compared to the gold tailings, no significant changes were presented in the XRD spectra. Therefore, the XRD patterns for this portion of the consolidated tailings were not shown in this paper. The XRD spectra gradually changed with the increase in cement content. The qualitative identification results of the consolidated tailings with 3% and 5% cement content after 2 h of consolidation were shown in Supplementary Figures S16, 17, respectively.
Starting from consolidating 2 h, diffraction peaks of C-S-H, calcium aluminosilicate hydrate, and calcium carbonate were detected in the consolidated tailings, with the calcium carbonate crystals present in the form of calcite. From consolidating 1 day, diffraction peaks of ettringite were detected. The diffraction peaks of hydration products were positively correlated with consolidation time, which was consistent with the findings in Reference (Ahmed, 2015). Therefore, the peak intensity of calcium carbonate continuously increased during the consolidation period. The enhancements of diffraction peaks of C-S-H and calcium aluminosilicate hydrate were primarily concentrated primarily within the first 7 days and 3 days, respectively. The diffraction peak of ettringite increased slowly at first, but rapidly after 3 days of consolidation.
Due to the existence of alkaline environment, the diffraction peak of calcium aluminosilicate hydrate at the initial stage of hydration gradually increased with the in-crease of consolidation time. As the alkaline environment diminishes, the diffraction peak of calcium aluminosilicate hydrate no longer increased. On the first day of hydration reaction, the diffraction peaks of ettringite were relatively weak, indicating a poorer degree of crystallinity. This observation aligned with the results reported in Reference (Long and Liu, 1997), indicating that alkaline conditions can inhibit the crystallization of ettringite. Consequently, only a limited quantity of poorly crystalline ettringite forms, which predominantly exists in either colloidal or microcrystalline states.
The reasons for the above characteristics in the XRD pattern of consolidated tailings were analyzed as follows:
1) At the initial stage of hydration (20 min of consolidation), the generated C-S-H, calcium aluminosilicate hydrate, calcium hydroxide, and ettringite were small in size and low in quantity, after which the hydration reaction entered a dormant phase. At 1 h of consolidation, no significant changes were presented both in the size and quantity of hydration products in the consolidated tailings. Therefore, the diffraction peaks of the hydration products were difficult to detect.
2) As the hydration reaction progresses, the size and quantity of the hydration products in the consolidated tailings gradually increased, and their crystallinity improved. Air entered the consolidated tailings through the pores on the polypropylene woven fabrics, and carbon dioxide in geotextile bags reacted with water to form carbonic acid, which then reacted with calcium hydroxide generated from the hydration of tricalcium silicate and dicalcium silicate to produce calcium carbonate. Palacios and Puertas (2006) suggested that the alkaline environment accelerated the carbonation of hydration products. Therefore, the diffraction peaks of C-S-H, calcium aluminosilicate hydrate, and calcium carbonate were detected in the consolidated tailings.
3) The factors limiting the formation of ettringite may be the alkaline environment and carbonation. Therefore, ettringite diffraction peaks were only observed after 1 day of hydration. As the hydration reaction progresses, the alkaline substances in the consolidated tailings were gradually consumed, leading to an increase in the quantity of ettringite. Multiple ettringite diffraction peaks were detected in the XRD spectra, and these peaks became more pronounced with the increase of consolidation time.
4) The practical application of this study’s findings in engineering contexts is subject to certain limitations. Firstly, the filling slurry preparation at tailings dam construction sites utilizes clarified tailings slurry recycled from the tailings pond to the concentrator. This slurry inherently contains residual tailings particles, resulting in elevated grout concentrations. Secondly, variations in metal ore types and beneficiation processes may alter the chemical composition of tailings, potentially affecting hydration reactions within geotextile bags. Future research directions will prioritize addressing these identified limitations to enhance the practical applicability of the findings.
4.2 Discussion on scanning electron microscopy of consolidated tailings
The microstructures of consolidated tailings with different cement contents and consolidation times were shown in Supplementary Figures S18–25. As the cement content and consolidation time increase, a gradual transition in the particle morphology of consolidated tailings was observed, culminating in the development of the composite particle architecture illustrated in Supplementary Figure S14. The presence of C-S-H, calcium aluminosilicate hydrate, calcium hydroxide, ettringite, and calcite in the consolidated tailings were influenced from quantity to morphology by cement content and consolidation time.
In terms of the relationship with geotextile bag permeability and fine tailings utilization ratio, the early stage of hydration has a significant impact. Within 1 h of hydration (Supplementary Figures S18, 19), the number of hydration products was low and their sizes were small. Therefore, no significant differences were observed in microstructure compared to gold tailings without cement.
After 2 h of hydration (Supplementary Figure S20), composite particles composed of coarse tailings, a hydrate film layer, and fine tailings can be observed. The flocculent structure on the surface of composite particles may be calcium aluminosilicate hydrate gel or C-S-H gel. The range and thickness of the hydrate film layer gradually increased with the rise in cement content, and the particle size of the composite particles also gradually increased. With increasing cement content and consolidation time, the hydrate films exhibited progressive expansion in both its coverage of coarse tailings particles and its thickness. Concurrently, a greater number of fine tailings particles became entrapped, wrapped, and adsorbed within the hydrate films, ultimately leading to the growth of composite particle size. The deposition of these larger composite particles within the geotextile bag not only mitigated the clogging of surface pores by fine tailings particles but also enhanced interparticle pore diameter during filling. This structural modification facilitated more efficient drainage of water, thereby improving both the water permeability and sand retention capacity of the geotextile bag system. In Supplementary Figure S20c, gel aggregates with a mushroom-like, leaf-like shape can be observed, which may be calcium aluminosilicate hydrate gel generated from cement in an alkaline environment.
Supplementary Figure S21 presented a high-magnification observation of Supplementary Figure S20b, revealing the accumulation of a hydrate film layer on the surface of coarse tailings. Notably, needle-like ettringite with diameters less than 500 nm was embedded within the interwoven fibers of C-S-H. Additionally, rhombohedral calcite and C-S-H flocs were also attached to the lamellar calcium hydroxide.
At 1 day of hydration, the quantity of needle-like ettringite in consolidated tailings increased. Notably, in Supplementary Figures S2 2b, c, ettringite can be observed embedded within C-S-H flocs, forming a spatial network structure.
At 3 days of hydration, more square-shaped calcite and a spatial network structure intergrown with C-S-H and ettringite were observed. In Supplementary Figures S23b, c, it can be seen that compared to Supplementary Figure S22, the spatial network structure continued to develop, and the ettringite gradually became more robust.
Sun and Hou (2020) concluded from unconfined compressive strength tests that the increased hydration products filled the voids between the particles of consolidated tailings. In comparison with Sun’s research, which primarily examined the relationship between hydration products and void filling through strength testing, our SEM observations provide a more comprehensive and detailed characterization of the microstructural evolution across distinct hydration stages. Specifically, our analysis elucidates the temporal progression of hydration product morphology and its consequent effects on the structural configuration of tailings particles. Starting from 7 days of hydration, it became evident that the quantity and size of hydration products in consolidated tailings were influenced by cement content. At 1% cement content, the area of flocs embedded by ettringite was smaller, with fewer quantities of C-S-H and ettringite, while the particle surface profiles were still visible. When the cement content increased to 3% and 5%, the quantities of C-S-H and ettringite significantly increased, and ettringite continued to develop and become more robust. The profiles of tailings particles became difficult to discern due to the coverage by C-S-H and ettringite, and fine tailings particles were distributed among the hydration products. At 28 days of hydration, the hydration products and tailings particles cannot be clearly identified, and the clustering phenomenon was also evident among the fine tailings particles. This indicated that, aided by the hydration products, more fine tailings particles were sticking together, and the hydration products continued to harden within the consolidated tailings. Compared with previous studies, our results further confirm the long - term evolution trend of tailings microstructure under different hydration conditions.
5 Conclusion
In this paper, to optimize the construction efficiency of geotextile bag dams and improve the utilization rate of fine tailings, geotextile bags filling experiments, X-ray diffraction experiments and scanning electron microscopy experiments were per-formed through exploring the effect of cement content on filling characteristics from both macro and micro perspective. By comparing the measured water content with the theoretical water content of consolidated tailings, the reliability of the filling tests were verified, and the following conclusions were drawn:
1) The filling characteristics of geotextile bags, including their permeability and sand retention, were improved by adding cement into the fine tailing slurries. The drainage efficiency and sand retention ratio were selected as indexes to evaluate the filling characteristics. Improvements in the filling characteristics depended on the cement content, and it was generally noticed that higher cement contents showed more significant improvements. A cement content of 1% had an extremely poor effect on the filling characteristics. Increasing the cement content to 3% provided an obvious improvement in the filling characteristics. Although the drainage efficiency improved when the cement content increased from 3% to 5%, the construction cost was significantly increased, and the sand retention ratio was only slightly improved. Therefore, the optimal cement content was determined to be 3%.
2) The primary reason for the improved filling characteristics was the formation of composite particles through the hydrated films which absorbed finer tailings in the filling slurry. Since the composite particles were larger than those of the original tailings in the filling slurries, the pore blockage of geotextile bags was reduced, and the efficient drainage area increased. Therefore, smooth drainage channels were formed to improve drainage efficiency. Since some finer tailings particles were absorbed by hydrated films, the number of tailings particles discharged from geotextile bags significantly decreased. Therefore, the tradeoff between permeability and sand retention was effectively overcome.
3) The consolidated tailings exhibited alkalinity in the initial stage of hydration, which facilitated the early hydration reactions of cement, promoting the formation of early hydration products and carbonation. Scanning electron micros-copy revealed composite particles composed of coarse tailings, a hydrate film lay-er, and fine tailings, along with hydration products formed at different hydration stages. Fibrous C-S-H intertwined with some fine tailing particles in its fibrous layers, while the surface of the hydrate film layer also adhered to some fine tailings particles. These composite particles not only reduced the clogging of the polypropylene woven fabrics surface by fine tailings but also optimized the pore structure of the consolidated tailings, providing smoother drainage pathways.
4) The practical application of this study’s findings in engineering contexts is subject to certain limitations. Firstly, the filling slurry preparation at tailings dam construction sites utilizes clarified tailings slurry recycled from the tailings pond to the concentrator. This slurry inherently contains residual tailings particles, resulting in elevated slurry concentrations. Secondly, variations in metal ore types and beneficiation processes may alter the chemical composition of tailings, potentially affecting hydration reactions within geotextile bags. Future research directions will prioritize addressing these identified limitations to enhance the practical applicability of the findings.
Data availability statement
The raw data supporting the conclusions of this article will be made available by the authors, without undue reservation.
Author contributions
HL: Conceptualization, Data curation, Formal Analysis, Funding acquisition, Methodology, Project administration, Resources, Supervision, Validation, Visualization, Writing – original draft, Writing – review and editing. MS: Conceptualization, Methodology, Software, Validation, Visualization, Writing – original draft, Writing – review and editing. JZ: Formal Analysis, Investigation, Software, Writing – original draft. LP: Investigation, Validation, Writing – original draft.
Funding
The author(s) declare that financial support was received for the research and/or publication of this article. This work was supported by Scientific Research Funding Project of Education Department of Liaoning Province, China [grant numbers LJKY2020114].
Conflict of interest
The authors declare that the research was conducted in the absence of any commercial or financial relationships that could be construed as a potential conflict of interest.
Generative AI statement
The author(s) declare that no Generative AI was used in the creation of this manuscript.
Publisher’s note
All claims expressed in this article are solely those of the authors and do not necessarily represent those of their affiliated organizations, or those of the publisher, the editors and the reviewers. Any product that may be evaluated in this article, or claim that may be made by its manufacturer, is not guaranteed or endorsed by the publisher.
Supplementary material
The Supplementary Material for this article can be found online at: https://www.frontiersin.org/articles/10.3389/fmats.2025.1529905/full#supplementary-material
References
Ahmed, A. (2015). Compressive strength and microstructure of soft clay soil stabilized with recycled bassanite. Appl. Clay Sci. 0, 27–35. doi:10.1016/j.clay.2014.11.031
Aydilek, A. H., and Edil, T. B. (2008). “Remediation of highwater content geomaterials: a review of geotextile filter performance,” in In first panamerican conference on geosynthetics. China : Environmental Science, 902–910.
Bulut, A., and Sengul, T. (2024). Investigation of the dewatering process with geotextile tubes by sedimentation, one- and two-dimensional filtration test methods. Arabian J. Sci. Eng. 50, 2091–2108. doi:10.1007/s13369-024-09323-9
Cai, Y. Y., Jiang, H. C., Yu, J., Tu, B. X., and Liu, S. Y. (2016). Experimental study and mesoscopic numerical simulation of the mechanical properties of cement-solidified coastal aeolian sand. Chin. J. Geotechnical Eng. 38, 1973–1980.
Du, Y. Q., Xie, B., Mullarney, B., and Zhang, C. (2019). Deposition of fine tailing particles and profile zoning of tailings dams. Soil Mech. Found. Eng. 56, 359–365. doi:10.1007/s11204-019-09615-5
Finklenburg, B., Klopries, E. M., and Schüttrumpf, H. (2024). 2D failure mechanisms and failure modes of a new type of geotextile tubes used for river dikes. Geotext. Geomembranes 52, 690–703. doi:10.1016/j.geotexmem.2024.03.009
Fu, S., Liu, X., Liu, X., Chen, M., and Deng, Z. (2018). An experimental study of the mechanical properties of tailings filling mold bag. Hydrogeol. Eng. Geol. 45, 83–88.
Gao, K. (2015). The influence of adding nano SiO2 on the characteristics of alkali-activated inorganic polymer prepared from TFT-LCD waste glass. Yantai: Yantai University.
Guo, Y. (2007). Research on solidification and mechanical properties of silty soil. Hangzhou: Zhejiang University.
Guo, Y., Xu, R. Q., and Shao, Y. C. (2008). Study on solidification mechanism of silty soil. J. Zhejiang Univ. Eng. version. 6, 1071–1075. doi:10.3785/j.issn.1008-973X.2008.06.033
Han, Y., Cui, X., and Qie, Y. (2019). Industrial test of damming using different kinds of geofabriform filled with grade tailings. Nonferrous Met. Min. Sect. 71, 41–46.
Horpibulsuk, S., Miura, N., and Nagarag, T. S. (2003). Assessment of strength development in cement-admixed high water content clays with Abrams’law as a basis. Geotechnique 4, 439–444. doi:10.1680/geot.2003.53.4.439
Huang, L., and Yan, P. Y. (2019). The influence of alkali content on the rheological properties of fresh cement slurry. J. Chin. Ceram. Soc. 11, 1546–1553.
Kim, H. J., and Dinoy, P. R. (2021). Two-dimensional consolidation analysis of geotextile tubes filled with fine-grained material. Geotext. Geomembranes 49, 1149–1164. doi:10.1016/j.geotexmem.2021.03.009
Kong, X. H., Liang, Y. P., and Cui, S. (2024). Factors influencing and mechanisms of activated MgO carbonization in the solidification of dredged sediment. J. Build. Mater. 7, 620–628. doi:10.3969/j.issn.1007-9629.2024.07.007
Li, Q. Y., Ma, G. W., and Lu, Y. L. (2023). An experimental and theoretical study on the tailings dam with geotextile bags. Sustainability 156, 1–14. doi:10.3390/su15064768
Li, Y., Wang, S. Y., and Yin, H. B. (2019). A review of the effects of alkali on hydration and hydration products of cementitious materials. Yangtze River Sci. Acad. J. 1, 127–133. doi:10.11988/ckyyb.20170736
Liu, A. M. (2007). Analysis of factors affecting the strength of low-cement-content solidified soil. Port & Waterw. Eng. 2, 24–27. doi:10.16233/j.cnki.issn1002-4972.2007.02.006
Liu, X. W., Wu, Z. M., He, H. S., and Xu, Q. (2024). An experimental study focusing on the filling process and consolidation characteristics of geotextile tubes filled with fine-grained tungsten tailings. Sustainability 16, 5270. doi:10.3390/su16125270
Long, S. Z., and Liu, C. (1997). Study on the early hydration effects of NaOH and Ca(OH)2 on the C3A-CaSO4.2H2O-H2O system. J. Chin. Ceram. Soc. 6, 635–642.
Palacios, M., and Puertas, F. (2006). Effect of carbonation on alkali-activated slag paste. J. Chin. Ceram. Soc. 10, 3211–3221. doi:10.1111/j.1551-2916.2006.01214.x
Richard Weggel, J., Jacob, D., and Douglas, G. (2011). Analysis of fluid discharge from a hanging geotextile bag. Geotext. Geomembranes 29(1): 65–73. doi:10.1016/j.geotexmem.2010.06.011
Sheng, M. Q., Zhu, Q. X., Huang, F. M., Zou, C., Gao, Y., Liu, X. X., et al. (2024). Study on the formation and dewatering process of the surface filter cake of geotextile on the lateral boundary of geotextile tubes under constant flow grouting. Front. Earth Sci. 12, 1427659. doi:10.3389/feart.2024.1427659
Sun, H. C., Wang, W. J., and Ling, D. S. (2021). Mechanical properties and microstructure of soil stabilized with low-dosage cement. J. Zhejiang Univ. 55, 530–538. doi:10.3785/j.issn.1008-973X.2021.03.013
Sun, X., and Hou, Y. (2020). Experimental investigation of the macroscopic behavior and microstructure property evolution of hardened cement consolidated tailings. Minerals 1, 6–17. doi:10.3390/min10010006
Sun, Z. P., Liu, Y., Yang, X., and Yang, H. J. (2016). Early hydration performance of three kinds of cement with different particle size. J. Build. Mater. 6, 965–968.
Tseng, I. F., Hsu, C. H., Cheng, H. C., and Chen, Y. S. (2024). Application of geotextile tubes to coastal silt mitigation: a case study in niaoyu fishing harbor. Sustainability 15, 2024. doi:10.3390/su15032024
Wang, X. P. (2019). Research on the microstructure of alkali-activated slag cement hydration C-A-S-H gel. Bull. Chin. Ceram. Scoiety 4, 1062–1067.
Wu, H. M., Tian, Z. Y., Shu, Y. M., Mo, D. H., Pei, Y., Mao, W. L., et al. (2021). Experimental study on the dewatering performance of geotextile tubes fflled with silt-sand slurry using hanging bag tests. J. Tianjin Sci. Technol. 54, 487–496. doi:10.11784/tdxbz202004075
Xiao, J., Xiang, J. J., and Liu, Z. H. (2024). Optimization of solidification agent ratios for fluid multi-source solid waste in loess and mechanisms of strength formation. J. China Highw. Eng. 9, 1–16.
Yang, A. W., and Hu, Y. (2018). Engineering characteristics and micro-mechanism of new urban sludge solidified soil. Rock Soil Mech. 1, 69–78. doi:10.16285/j.rsm.2017.2046
Zhang, H., Sun, H. L., Liu, S. J., Geng, X. Y., Deng, Y. F., Cai, Y. Q., et al. (2023b). Combined vacuum-assisted geotextile and geomembrane tubes for sludge dewatering: a theoretical switching point. Can. Geotechnical J. 61, 978–991. doi:10.1139/cgj-2022-0370
Zhang, J. F., Song, Z. F., and Geng, W. Z. (2023a). Analysis and prevention of tailings dam accidents in China. Mod. Min. 3, 6–9. doi:10.3969/j.issn.1674-6082.2023.03.002
Zheng, G. J. (2011). “Preparation of amorphous Al2O3-2SiO2 powder and study on geopolymerization reaction mechanism,” in Nanning. China: Guangxi University.
Keywords: fine tailings, geotextile bags, low cement content, filling tests, filling characteristics, microscopic experiments
Citation: Li H, Shi M, Zhang J and Peng L (2025) An experimental study on the influence of low - cement content on improved filling characteristics of geotextile bags filled with fine tailings slurry. Front. Mater. 12:1529905. doi: 10.3389/fmats.2025.1529905
Received: 19 November 2024; Accepted: 21 April 2025;
Published: 09 May 2025.
Edited by:
Jialai Wang, University of Alabama, United StatesReviewed by:
Pedro Lamas, NOVA University of Lisbon, PortugalLeila Khajenoori, University of Central Lancashire, United Kingdom
Copyright © 2025 Li, Shi, Zhang and Peng. This is an open-access article distributed under the terms of the Creative Commons Attribution License (CC BY). The use, distribution or reproduction in other forums is permitted, provided the original author(s) and the copyright owner(s) are credited and that the original publication in this journal is cited, in accordance with accepted academic practice. No use, distribution or reproduction is permitted which does not comply with these terms.
*Correspondence: Hui Li, bGgxMzQ2NDg1NzYwMUAxMjYuY29t