- 1Qingdao Technology Innovation Center of Remote Sensing and Precise Measurement, School of Science, Qingdao University of Technology, Qingdao, China
- 2Zaozhuang Vocational College, Zaozhuang, China
- 3Beijing Key Laboratory of Cardiopulmonary-Cerebral Resuscitation, Emergency Department, Chao-Yang Hospital, Capital Medical University, Beijing, China
Friction, wear, and corrosion are common phenomena in both industrial and daily life, significantly impacting the performance, lifespan, and energy efficiency of equipment. To mitigate these adverse effects, current research primarily focuses on the development of novel materials, surface modification techniques, and lubricating coatings. Accurate detection and evaluation are crucial for understanding material behavior under varying conditions, which not only aids in optimizing material design but also enables timely identification and repair of potential issues, thereby extending equipment lifespan and enhancing reliability. Addressing the limitations of existing detection methods in real-time monitoring and micro-defect detection, this study proposes a polarization-based detection method to more accurately assess and optimize the friction, wear, and corrosion properties of materials. This method leverages the polarization characteristics of light to investigate the optical properties and microstructures of materials, offering advantages such as high data dimensionality, sensitivity to microstructural changes, and compatibility with existing optical techniques. We constructed a polarization detection system and employed data analysis methods, such as linear discriminant analysis, to study various samples. By using this system, we extracted specific parameters sensitive to different characteristic variables, enabling us to more accurately characterize the lubrication performance of materials and providing valuable insights for material optimization. The proposed polarization-based detection method offers a new approach for evaluating the friction, wear, and corrosion properties of materials, demonstrating potential for real-time monitoring and micro-defect detection, and is expected to enhance equipment lifespan and reliability.
1 Introduction
In modern industry and daily life, friction, wear, and corrosion are inevitable phenomena. These issues not only reduce mechanical efficiency, increase energy consumption and maintenance costs, but also may lead to equipment failure, safety hazards, and even significant economic losses. To mitigate the damage caused by friction, measures such as developing new materials, employing surface modification techniques, and applying lubricating coatings are commonly adopted. These approaches aim to enhance equipment performance and lifespan while reducing energy consumption and maintenance expenses. The development of novel materials focuses on finding substances with excellent wear resistance and corrosion resistance, such as high-strength alloys and ceramic-based composites. By altering the internal structure and chemical composition of these materials, their overall performance is improved, enabling them to maintain stable performance even in harsh working environments (Gul et al., 2024; Kalinin et al., 2019). Surface modification techniques involve the use of physical or chemical methods to create a special coating on the material’s surface or alter its microstructure. Techniques such as ion implantation (Guo et al., 2025; Sotoudeh Bagha et al., 2023) and laser cladding (Lu et al., 2025; Wang et al., 2025; Feng et al., 2025; Chen et al., 2025) are employed to enhance the hardness, wear resistance, and corrosion resistance of the material’s surface, thereby extending the service life of equipment. Lubricating coatings can form a stable and thin lubricating film on friction surfaces, effectively reducing the coefficient of friction, minimizing wear, and improving the operational efficiency and reliability of equipment (Zhang et al., 2025; Shao et al., 2024; Yang et al., 2024). Solid lubricants, due to their low evaporation rates, resistance to high and low temperatures, radiation tolerance, and corrosion resistance, have been widely used in mechanical equipment operating in extreme space environments (Song et al., 2024). Among these, MAX phase ceramic materials, such as titanium aluminum carbide (Ti3AlC2) and vanadium aluminum carbide (V2AlC), possess a thermodynamically stable nano-layered structure, which sets them apart from traditional solid lubricants and gives them unique properties. In the MAX phase formula, M represents a transition metal element, A represents a main-group element, and X represents carbon or nitrogen. On a microscopic level, MAX phases exhibit a hexagonal layered structure, consisting of alternating stacks of M6X octahedral layers and A atomic layers. This structure endows MAX phase ceramics with both the hardness of ceramics and the ductility of metals (Shuai and June 2018). Due to their inherent layered structure, MAX phase materials show great potential for research and application in the field of solid lubricants (Hai-zhong et al., 2013; Hai et al., 2013).
However, as industrial equipment evolves toward higher speeds, heavier loads, and extreme environments, the performance requirements for lubricating materials are becoming increasingly demanding. To ensure the reliability of lubricating materials in practical applications, it is essential to precisely detect and characterize their microstructure, surface morphology, and tribological properties. Current detection techniques primarily include optical microscopy (Křivánková et al., 2025), electron microscopy (Peng et al., 2024), and X-ray diffraction (Chavan et al., 2024). While these methods can provide microscopic information about materials to some extent, they still have limitations in terms of real-time monitoring and detecting microscopic defects. For example, the resolution of optical microscopy is limited, making it difficult to capture nanoscale surface defects. Although electron microscopes can provide high-resolution images, the sample preparation process is cumbersome, involving steps such as sampling, fixation, cutting, grinding, and polishing. The entire process from sample collection to data analysis may take several hours to a day or even longer, resulting in low detection efficiency. Additionally, electron microscopy is unable to perform real-time online monitoring. X-ray diffraction, on the other hand, cannot directly reflect the surface morphology or tribological properties of materials. In light of these issues, the development of a rapid, high-resolution, and non-destructive detection technology is of great significance for optimizing the performance of lubricating materials and their industrial applications. This paper selects polarized light scattering as a technique to evaluate the performance of MAX phase solid lubricants. Polarized light scattering, as a non-contact detection method, has the advantages of being label-free, non-destructive, and highly sensitive to sub-wavelength microstructural changes (Hong-Hui et al., 2015), it has been widely applied in various research fields including marine environmental monitoring, biomedical studies, and astronomical observations (Wang et al., 2020; Wang et al., 2018; He et al., 2025; Qi et al., 2022). By utilizing the differences in how various materials scatter polarized light, this technique can effectively extract information about the physical properties of materials. This method does not require a complex sample preparation process and can collect polarization state information at specific angles and perform data processing in just a few minutes. It not only improves detection efficiency but also avoids the sample contamination issues common in traditional techniques. Furthermore, it enables real-time online monitoring of materials, offering significant practical value.
2 Methods and samples
The polarization state of light can be described by the four-dimensional Stokes vector S, as shown in Equation 1.
Here, I represents the total light intensity; Q is the intensity difference between 0° linear polarization and 90° linear polarization; U is the intensity difference between 45° linear polarization and 135° linear polarization; and V is the intensity difference between right-handed circular polarization and left-handed circular polarization.
The polarized light scattering process can be described by the following Equation 2, where M is expressed as the matrix in (Equation 3).
Here,
Compared to traditional optical detection methods, the polarization detection method only requires the addition of a polarizer and a detector to the existing optical path for measurement. Polarization measurement technology can provide more information about the microscopic structure of a sample and is considered a promising detection technique.
2.1 Methods
2.1.1 Simulation calculations
The polarization scattering simulation program is designed based on Mie scattering theory (Jonsson et al., 2004). It can simulate the polarization scattering process of spherical particles and outputs results including: the polarization state of scattered light and the Mueller matrix for spherical particles with different refractive indices and sizes, under varying detection directions, incident light polarization states, and medium conditions.
The specific process for simulating spherical particles is as follows: Assuming the Stokes vector of the incident light is
2.1.2 Experimental measurements
2.1.2.1 Experimental setup
The schematic diagram of the experimental setup and the photographs of the physical equipment are shown in Figures 2A,B, respectively, and it mainly consists of three parts: the incident end, the sample cell, and the detection end. The incident end is used to generate and deliver the required polarized light; the sample cell is used to hold the experimental samples; and the detection end is used to collect and measure the polarization information of the scattered light.
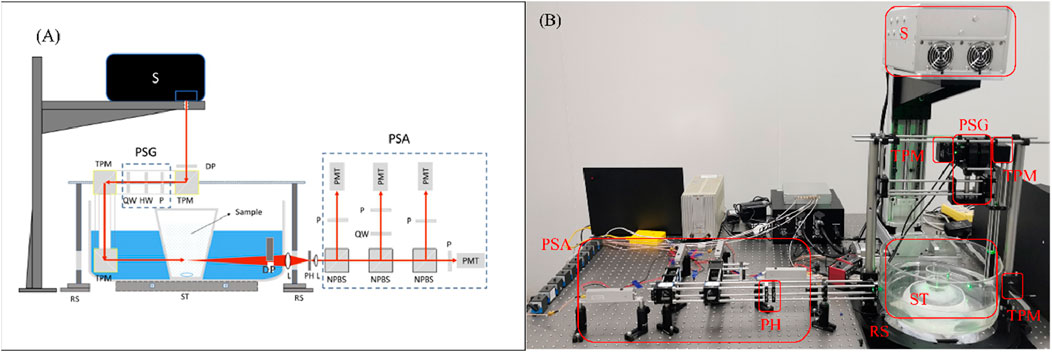
Figure 2. (A) Schematic diagram of the experimental setup. (B) Photographs of the physical equipment. S, light source; DP, aperture; TPM, reflection prism; PSG, polarization state generator; P, polarizer; HW, half-wave plate; QW, quarter-wave plate; ST, sample cell; RS, rotation stage; PH, circular pinhole; L, lens; PSA, polarization state analyzer; NPBS, non-polarizing beam splitter; PMT, photomultiplier tube.
The incident end includes a laser light source S, an aperture DP, and a polarization state generator PSG, among other components. The light emitted from the source becomes parallel light after passing through the aperture DP and then enters the PSG via a reflection prism TPM. The PSG, which is used to generate arbitrary polarization states, consists of a polarizer P, a half-wave plate HW, and a quarter-wave plate QW. By adjusting the angles of the half-wave plate and the quarter-wave plate using a motorized rotation stage, light with any desired polarization state can be obtained.
The light exiting the PSG passes through two reflection prisms TPM before entering the sample cell. The sample cell consists of a circular glass container filled with water and a small beaker containing the sample suspension. This setup is designed to minimize the influence of refraction from the beaker walls on the detection results and to facilitate the replacement of samples. The incident end is connected to a high-precision rotary stage capable of 360° rotation (a cross-sectional view is shown in the figure). By rotating the incident end, multi-angle measurements of the polarization state of the scattered light can be achieved.
In the sample solution, multiple particles may coexist, and their scattering signals can interfere with each other, leading to inaccurate measurement results. Therefore, single-particle measurements are necessary to obtain clearer scattering signals. Additionally, single-particle measurements can effectively avoid the issue in multi-particle measurements where averaging over a large number of particles may obscure the unique characteristics of individual particles, thereby overlooking some detailed information. The scattered light emerging from the sample cell passes through an aperture DP and a circular pinhole PH, and is then collimated by a lens before entering the analyzer. To achieve single-particle measurement and reduce interference from background light, we minimize the detection area. By controlling the size of the aperture and the circular pinhole, the scattering volume (the intersection between the incident and detection ends) can be effectively regulated.
Since the suspended particles in the sample are in constant motion, the polarization state of their scattered light is also continuously changing. Therefore, simultaneous measurement is required, where all four parameters of the Stokes vector can be determined in a single measurement. The polarization state analyzer PSA at the detection end is based on a mature polarization measurement method and has been improved (Wang, 2018). It has a measurement error of less than 6% and consists of three non-polarizing beam splitters NPBS, four polarizers, a quarter-wave plate, and four photomultiplier tubes PMT. After entering the analyzer, the scattered light is split by the beam splitters into four channels: the horizontal polarization channel, the left-circular polarization channel, the 45° polarization channel, and the 135° polarization channel. The light in each channel is then detected by the corresponding photomultiplier tubes. The data is collected using a multi-channel data acquisition card, and after extracting the effective pulse information, the polarization state of the light can be calculated.
2.1.2.2 Data processing
Linear Discriminant Analysis (LDA) is a classical supervised learning algorithm that projects high-dimensional data into a lower-dimensional space (Song et al., 2023). By calculating the maximum value of the between-class scatter matrix (Equation 9) and the minimum value of the within-class scatter matrix (Equation 10) in the [q u v] space, the objective function J (Equation 11) is maximized, thereby achieving the classification of multiple groups of data.
Here, C represents the data classes,
2.2 Samples
The experiment utilized three typical MAX phase ceramic materials, including 3 μm titanium silicon carbide, 10 μm titanium aluminum carbide and 24 μm vanadium aluminum carbide. The three samples were all prepared through high-temperature sintering followed by grinding and crushing, resulting in grayish-black powders with a purity of >99 wt%. The primary component of the titanium silicon carbide material is Ti3SiC2, with the weight percentages and atomic percentages of the elements as follows: C accounts for 17.41% by weight and 42.91% by atomic composition, Si accounts for 13.92% by weight and 14.67% by atomic composition, and Ti accounts for 68.67% by weight and 42.42% by atomic composition. The main component of the titanium aluminum carbide material is Ti2AlC, with the following weight percentages and atomic percentages: C accounts for 15.76% by weight and 38.89% by atomic composition, Al accounts for 18.72% by weight and 20.57% by atomic composition, and Ti accounts for 65.52% by weight and 40.54% by atomic composition. The principal component of the vanadium aluminum carbide material is V2AlC, with the weight percentages and atomic percentages as follows: C accounts for 15.26% by weight and 39.32% by atomic composition, Al accounts for 17.04% by weight and 19.55% by atomic composition, and V accounts for 67.70% by weight and 41.13% by atomic composition. All these samples were provided by Jiangsu Xianfeng Nanomaterials Technology Co., Ltd.
3 Results
3.1 Simulation results
Through the simulation program, we first investigated the effects of the polarization state of incident light and the detection direction on the polarization state of scattered light to determine the optimal polarization state of incident light and detection direction for the experiment. We selected spherical particles with different particle sizes and refractive indices for simulation. Here, we propose a criterion: each particle’s Stokes vector corresponds to a point in the three-dimensional [q, u, v] space. For particles with different particle sizes or refractive indices, the points corresponding to their Stokes vectors are distributed at different positions in this space. If, under the same polarization state of incident light and detection direction, the points corresponding to different particle sizes or refractive indices are far apart from each other, it indicates that they are well distinguished. Therefore, the average distance between scatter points can be used to characterize the effectiveness of particle recognition.
3.1.1 Results for particles with different diameters
We simulated particles with diameters ranging from 1 to 26 μm (the particle sizes of all three samples fall within this range), with detection directions spanning 0°–180° in 10° intervals, refractive indices of 2.6, 2.7, and 2.8, and six fundamental polarization states of incident light: horizontal linear polarization (h), vertical linear polarization (v), 45° linear polarization (p), 135° linear polarization (m), right-handed circular polarization (r), and left-handed circular polarization (l). The results are shown in Figures 3A–C. The x-axis represents the detection direction, and the y-axis represents the average distance between scatter points. It can be observed that, under three different refractive indices, the average distances between scatter points are relatively large when the detection directions are 140°, 150°, and 160° and the incident light polarization states are p, m, r, and l. This indicates that the points are well-separated, allowing for better differentiation of particles with different diameters.
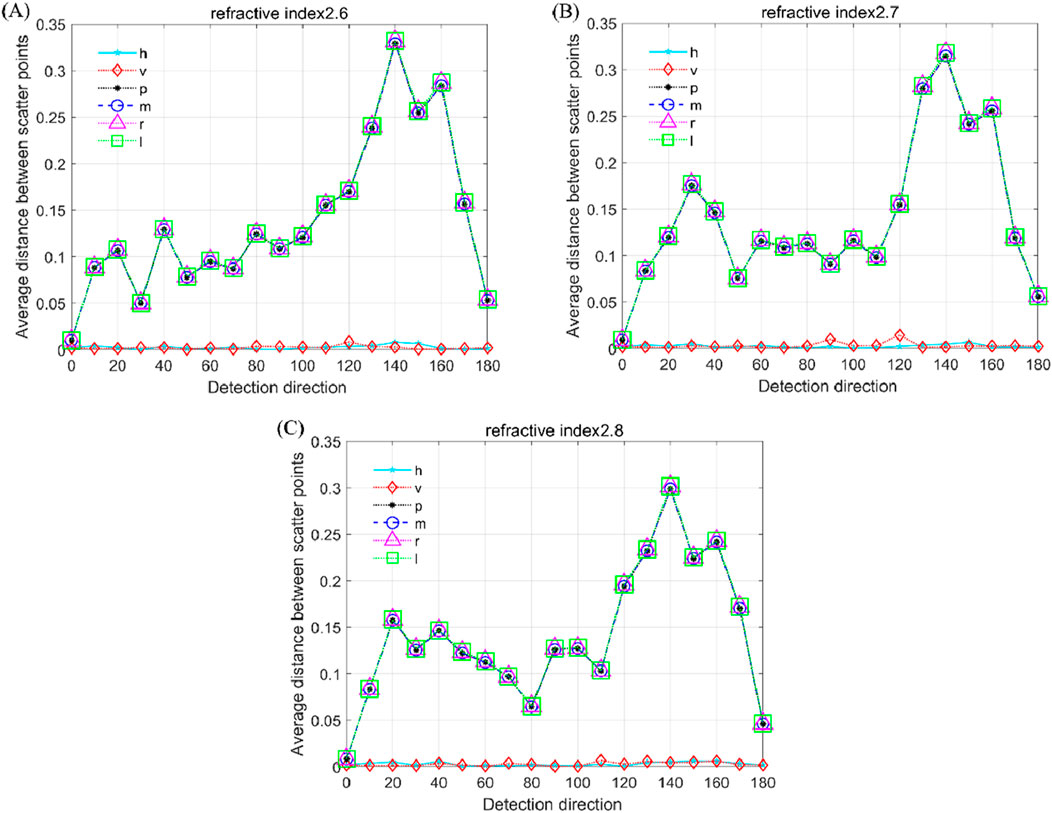
Figure 3. Average distances between scatter points of Stokes vectors for particles with different diameters. (A) Refractive index of 2.6. (B) Refractive index of 2.7. (C) Refractive index of 2.8.
3.1.2 Results for particles with different refractive indices
We simulated particles with refractive indices ranging from 2.6 to 3.1 (the refractive indices of all three samples fall within this range), with detection directions spanning 0°–180° in 10° intervals, particle diameters of 3 μm, 10 μm, and 24 μm (corresponding to the sample sizes), and six fundamental polarization states of incident light: horizontal linear polarization (h), vertical linear polarization (v), 45° linear polarization (p), 135° linear polarization (m), right-handed circular polarization (r), and left-handed circular polarization (l). The results are shown in Figures 4A–C. The x-axis represents the detection direction, and the y-axis represents the average distance between scatter points. From the figures, it can be observed that for all three particle sizes, the average distances between scatter points are relatively large when the detection directions are 140°, 150°, and 180° and the incident light polarization states are p, m, r, and l. This indicates that under these conditions, the scatter points corresponding to particles with different refractive indices are well-separated, allowing for better differentiation.
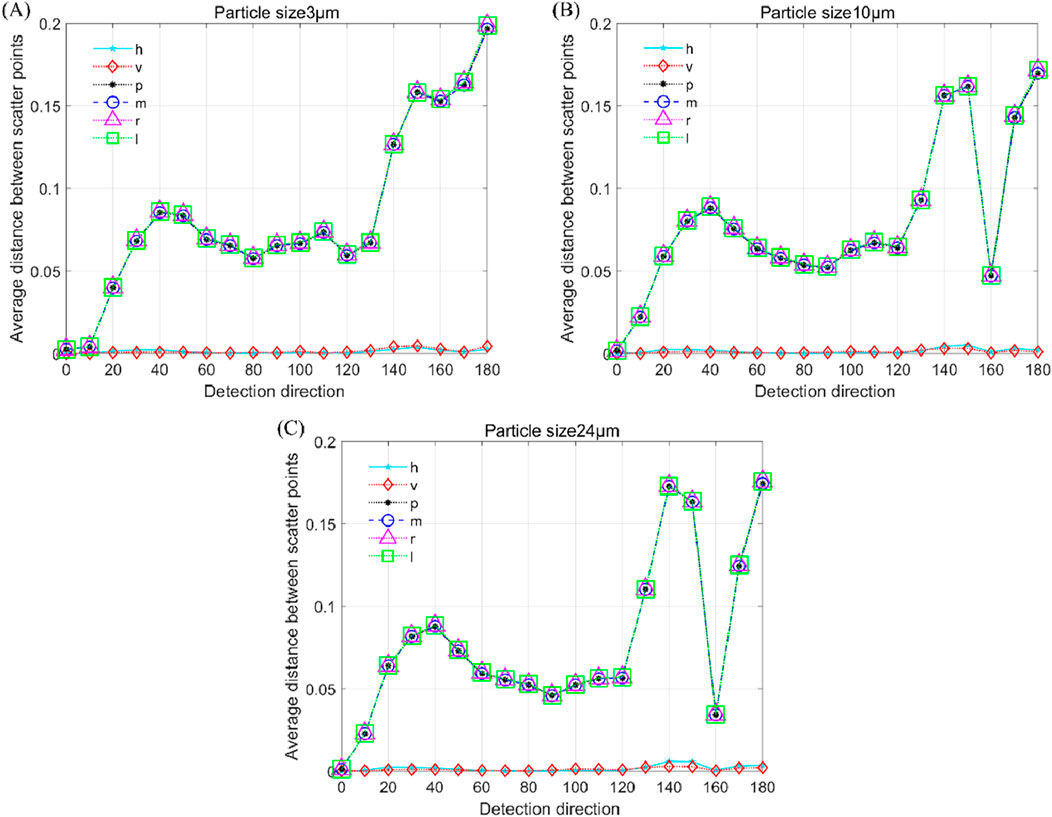
Figure 4. Average distances between scatter points of Stokes vectors for particles with different refractive indices. (A) Particle diameter of 3 μm. (B) Particle diameter of 10 μm. (C) Particle diameter of 24 μm.
Based on the simulations for particles with different diameters and refractive indices, we conclude that the optimal experimental conditions are achieved when the detection directions are 140° and 150° and the incident light polarization states are p, m, r, and l.
3.2 Experimental results
In the experiment, we performed measurements under identical conditions for three different samples. For each sample, the incident light source was set to 550 nm green light. Based on the simulation results, the polarization state of the incident light was uniformly set to 135° linear polarization, and the detection angle was set to 150°. During the experiment, to ensure the uniformity of the sample suspension concentration in the small beaker, a magnetic stirrer was placed beneath the beaker, operating at a speed of 250 revolutions per minute. Finally, we recorded the scattered light information for 2 min. From this data, we extracted valid pulses and calculated the Stokes vectors of the scattered light.
The experimental results for 3 μm titanium silicon carbide (Ti3SiC2), 10 μm titanium aluminum carbide (Ti2AlC), and 24 μm vanadium aluminum carbide (V2AlC) are shown in Figure 5. Figure 5 displays the scattering polarization distributions of the three samples at a detection angle of 150° and an incident polarization state of 135° linear polarization (m), after processing using the linear discriminant analysis method. It can be observed that the scattering components q, u and v of the three samples are distributed within certain ranges, and under these conditions, the distributions of the three samples differ, allowing them to be distinguished. This is consistent with the previous simulation results.
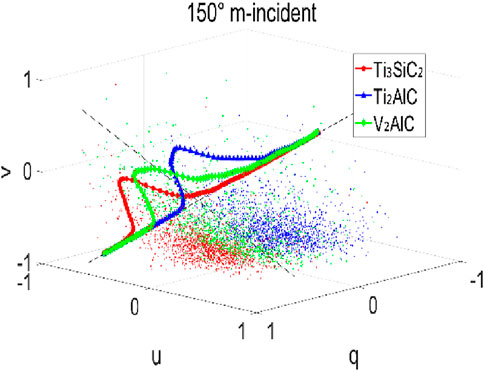
Figure 5. Scattering distributions of the three samples at a detection angle of 150° with 135° linearly polarized incident light.
4 Discussion
We calculated the polarization states of scattered light for spherical particles with a detection angle of 150°, incident light polarization set to 135° linear polarization, a refractive index of 2.6, and particle diameters of 1 μm, 6 μm, 11 μm, 16 μm, 21 μm, and 26 μm. The variations in q, u and v for spherical particles of different diameters are shown in Figure 6A. It can be observed from the figure that q, u and v change as the particle diameter increases, with the q-component showing more pronounced changes compared to the u and v-components. This indicates that the parameters of scattered light polarization can be used to characterize the particle size.
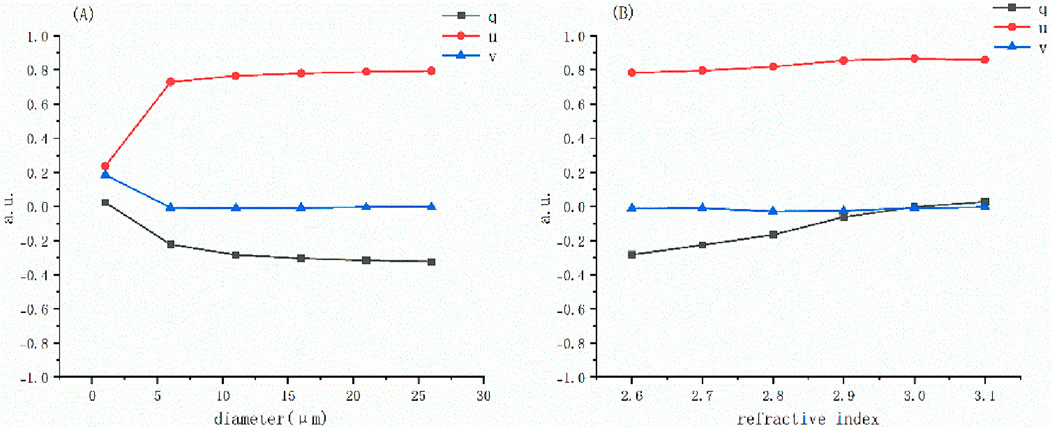
Figure 6. Variations in q, u, v of particle scattering. (A) q, u, v as a function of particle size. (B) q, u, v as a function of refractive index.
Similarly, we calculated the polarization states of scattered light for spherical particles with a detection angle of 150°, incident light polarization set to 135° linear polarization, a particle diameter of 10 μm, and refractive indices of 2.6, 2.7, 2.8, 2.9, 3.0, and 3.1. The variations in q, u and v for spherical particles with different refractive indices are shown in Figure 6B. It can be observed from the figure that the three components q, u and v exhibit certain monotonic trends as the refractive index changes. Among them, the variation in the q-component is greater than that of the u and v-components. These results indicate that the parameters of scattered light polarization can be used to characterize the refractive index of particles. Combining both cases, it can be concluded that polarization parameters can characterize the particle size and refractive index of lubricating materials. This demonstrates that the polarized light scattering method can effectively characterize the performance of lubricating materials.
5 Conclusion
This paper proposes a method based on polarized light scattering to characterize the lubrication performance of different materials. The surface morphology and microstructure of lubricating materials significantly influence their tribological properties. However, traditional characterization methods often suffer from issues such as destructiveness, insufficient resolution, and an inability to perform real-time monitoring. Polarization scattering technology, as a non-contact and non-destructive optical method, provides a new approach to addressing these challenges. We first conducted simulations to study the effects of different incident light polarization states and detection directions, using the average distance between scatter points to evaluate the effectiveness of particle recognition. The optimal characterization conditions were found to be a detection angle of 150° and an incident polarization state of 135° linear polarization. Subsequently, experimental validation was performed under the conditions obtained from the simulations, and the experimental results were consistent with the simulation outcomes. Finally, through discussion, we concluded that the polarization parameters of scattered light can effectively characterize the lubrication performance of materials. In summary, this study demonstrates the potential of using polarization scattering methods to evaluate the performance of lubricating materials. Future research may focus on establishing and quantifying the direct relationship between polarization parameters and lubrication performance, thereby promoting the application and development of polarization scattering technology in this field.
Data availability statement
The original contributions presented in the study are included in the article/supplementary material, further inquiries can be directed to the corresponding authors.
Author contributions
XL: Writing – original draft, Methodology, Investigation. JJ: Validation, Writing – review and editing. XH: Validation, Writing – review and editing. HL: Writing – review and editing, Data curation. YT: Writing – review and editing, Methodology. WM: Data curation, Writing – review and editing. XlZ: Supervision, Writing – review and editing, Methodology. ZL: Supervision, Writing – review and editing. JS: Writing – review and editing, Investigation. XuZ: Investigation, Writing – review and editing. YW: Data curation, Supervision, Writing – review and editing. XmZ: Supervision, Writing – review and editing.
Funding
The author(s) declare that financial support was received for the research and/or publication of this article. This work was supported by Natural Science Foundation of Shandong Province, China (Nos ZR2020QA078, ZR2023MD064), National Natural Science Foundation of China (No. 12005110), the Capital’s Funds for Health Improvement and Research (grant numbers: CFH 2021-1G-2031 and CFH 2024-1-1051) and Capital Health Hevelopment Research Project (No. SF 2021-1G-2031).
Conflict of interest
The authors declare that the research was conducted in the absence of any commercial or financial relationships that could be construed as a potential conflict of interest.
Generative AI statement
The author(s) declare that no Generative AI was used in the creation of this manuscript.
Publisher’s note
All claims expressed in this article are solely those of the authors and do not necessarily represent those of their affiliated organizations, or those of the publisher, the editors and the reviewers. Any product that may be evaluated in this article, or claim that may be made by its manufacturer, is not guaranteed or endorsed by the publisher.
References
Chavan, K. B., Desarada, S. V., Chaure, S., and Chaure, N. B. (2024). Study of substrate dependent microstructural properties of sputtered Mo/CZTS heterojunctions using X ray diffraction. Solid State Sci. 158, 107739. doi:10.1016/J.SOLIDSTATESCIENCES.2024.107739
Chen, H., Du, Y., Liang, Q., Ma, W., and Tu, J. (2025). Effect of double aging treatment on the tribological properties of 15-5PH coating on 17-4PH stainless steel by laser cladding. J. Mat. Res. Technol. doi:10.1016/J.JMRT.2025.02.104
Feng, M., Yang, X., Lian, G., and Chen, C. (2025). Microstructure and properties of laser cladding CoCrNi-based medium-entropy alloy enhanced by Nb. J. Mat. Res. Technol. doi:10.1016/J.JMRT.2025.02.100
Gul, O., Karsli, N. G., Gul, C., Durmus, A., and Yilmaz, T. (2024). Study of the effect of hexagonal boron nitride addition to thermoplastic polyester elastomer composites reinforced with carbon, glass and basalt fibers. Polym. Bull. 81 (17), 16219–16239. doi:10.1007/S00289-024-05471-Y
Guo, L., Wang, X., Lu, L., Cao, H., Dai, Y., Tang, K., et al. (2025). Different corrosion behaviors of Sn-based modification coatings on magnesium alloy surface via plasma-involved processes: FCVA deposition vs MEVVA ion implantation. Appl. Surf. Sci. 684, 161842. doi:10.1016/J.APSUSC.2024.161842
Hai, W., Zeng, J., Ren, S., Meng, J., and Lu, J. (2013). Tribological behavior and tribochemistry of self-mated Ti 3 SiC 2 in ethanol. Tribol. Lett. 50, 449–455. doi:10.1007/s11249-013-0142-x
Hai-zhong, W. A., Jun-ling, Z. E., Da-peng, F. E., Jin-jun, L. U., and Wei-min, L. I. (2013). Tribological behavior of Si3 N4- Ti3 SiC2 contacts lubricated by ionic liquids. Tribology 33 (06), 537–542. doi:10.16078/j.tribology.2013.06.001
He, Q., Zhan, J., Liu, X., Dong, C., Tian, D., and Fu, Q. (2025). Multispectral polarimetric bidirectional reflectance research of plant canopy. Opt. Lasers Eng. 184, 108688. doi:10.1016/J.OPTLASENG.2024.108688
Hong-Hui, H., Nan, Z., Ran, L., and Hui, M. (2015). Progresses of polarization imaging techniques and their applications in cancer detections. Prog. Biochem. Biophys. 42 (05), 419–433. doi:10.16476/j.pibb.2015.0034
Jonsson, J. C., Smith, G. B., and Niklasson, G. A. (2004). Experimental and Monte Carlo analysis of isotropic multiple Mie scattering. Opt. Commun. 240 (1-3), 9–17. doi:10.1016/j.optcom.2004.06.017
Kaiser, T., and Schweiger, G. (1993). Stable algorithm for the computation of Mie coefficients for scattered and transmitted fields of a coated sphere. Comput. Phys. 7 (6), 682–686. doi:10.1063/1.168475
Kalinin, Y. E., Kosilov, A. T., Ovdak, O. V., Kudrin, A. M., Karaeva, O. A., Kashirin, M. A., et al. (2019). Internal friction in glass fiber-and carbon fiber-reinforced composites with a T-107 matrix. Tech. Phys. 64, 535–539. doi:10.1134/S1063784219040121
Křivánková, L., Chotěborský, R., Černilová, B., and Linda, M. (2025). Analysis of grain size and distribution in Fe-BC alloy using optical microscopy and image analysis. Materials 18 (3), 596. doi:10.3390/MA18030596
Lu, D., Cui, X., and Zhang, J. (2025). Microstructure and properties of high entropy alloy coating obtained by laser cladding. Sci. Rep. 15 (1), 7357. doi:10.1038/S41598-025-91706-5
Peng, X., Shangguan, J., Zhang, Q., Hauwiller, M., Yu, H., Nie, Y., et al. (2024). Unveiling corrosion pathways of Sn nanocrystals through high-resolution liquid cell electron microscopy. Nano Lett. 24 (4), 1168–1175. doi:10.1021/ACS.NANOLETT.3C03913
Qi, L., Li, G., Ge, M., Zhang, J., Jiang, W., Liu, X., et al. (2022). Implementation of the polarimetry focusing telescope array observation simulator on board the X-ray timing and polarimetry observatory. Astrophys. J. 934 (2), 109. doi:10.3847/1538-4357/AC7B82
Shao, X., Wu, X., Liu, H., Wang, P., Du, C. F., Wang, L., et al. (2024). Effect of nano-particles ZnO and layered GO on high temperature tribological performance of MoS2-based heterojunction composite lubricating coating. J. Mat. Sci. 59 (42), 19855–19874. doi:10.1007/S10853-024-10303-5
Shuai, W. A., and Jun, Y. A. (2018). Progress research on the tribology of MAX phase ceramics. Tribology 38 (06), 735–746. doi:10.16078/j.tribology.2018121
Song, L., Jiang, T., Yu, H., Zhou, Y., Cao, X., Wang, P., et al. (2024). Research progress on the tribological properties of epoxy-based solid-lubricating composites. Polym. Compos. 45 (18), 16452–16466. doi:10.1002/PC.28923
Song, T., Huang, Z., and Xu, H. (2023). Linear discriminant analysis algorithm for detecting hardware trojans delay. J. Electron. Inf. Techn. 45 (01), 59–67. doi:10.11999/JEIT220389
Sotoudeh Bagha, P., Paternoster, C., Khakbiz, M., Sheibani, S., Gholami, N., and Mantovani, D. (2023). Surface modification of an absorbable bimodal Fe-Mn-Ag alloy by nitrogen plasma immersion ion implantation. Materials 16 (3), 1048. doi:10.3390/MA16031048
Wang, J., Cui, X., Zhao, Y., Zhang, Y., Jin, G., Feng, C., et al. (2025). Microstructure and corrosion performance of Fe-based composite layer doped modification with Ti6Al4V fabricated by underwater wet laser cladding. Mat. Today Commun. 44, 111875. doi:10.1016/J.MTCOMM.2025.111875
Wang, Y. (2018). A study on classification of suspended particles and marine microalgae by polarized light scattering. Beijing, China: Tsinghua University.
Wang, Y., Dai, J., Liao, R., Zhou, J., Meng, F., Yao, Y., et al. (2020). Characterization of physiological states of the suspended marine microalgae using polarized light scattering. Appl. Opt. 59 (5), 1307–1312. doi:10.1364/AO.377332
Wang, Y., Liao, R., Dai, J., Liu, Z., Xiong, Z., Zhang, T., et al. (2018). Differentiation of suspended particles by polarized light scattering at 120. Opt. Express 26 (17), 22419–22431. doi:10.1364/OE.26.022419
Yang, D., Cheng, J., Tang, Z., Chen, W., Xiao, R., Geng, Y., et al. (2024). Friction and wear properties of D-gun sprayed CrFeNiAl0. 3Ti0. 3-Ag-SrSO4 high entropy alloy matrix self-lubricating coating at elevated temperature. Tribol. Int. 200, 110151. doi:10.1016/J.TRIBOINT.2024.110151
Keywords: polarization, characterization, lubrication performance, specificity parameter, materials
Citation: Liu X, Jian J, Huang X, Li H, Tian Y, Ma W, Zhao X, Liu Z, Sun J, Zhang X, Wang Y and Zhu X (2025) Polarization scattering-based detection of lubricating materials. Front. Mater. 12:1596327. doi: 10.3389/fmats.2025.1596327
Received: 19 March 2025; Accepted: 16 April 2025;
Published: 29 April 2025.
Edited by:
Huan Liu, Tsinghua University, ChinaReviewed by:
Lingwei Ma, University of Science and Technology Beijing, ChinaXiushuo Zhang, Tsinghua University, China
Copyright © 2025 Liu, Jian, Huang, Li, Tian, Ma, Zhao, Liu, Sun, Zhang, Wang and Zhu. This is an open-access article distributed under the terms of the Creative Commons Attribution License (CC BY). The use, distribution or reproduction in other forums is permitted, provided the original author(s) and the copyright owner(s) are credited and that the original publication in this journal is cited, in accordance with accepted academic practice. No use, distribution or reproduction is permitted which does not comply with these terms.
*Correspondence: Yong Wang, d2FuZy15b25nMTNAdHNpbmdodWEub3JnLmNu; Xiaomei Zhu, emh1eG0wNzIyQDE2My5jb20=
†These authors have contributed equally to this work and share first authorship